- 1Center for Perinatal Research, Nationwide Children's Hospital, Columbus, OH, United States
- 2Department of Pediatric Surgery, Nationwide Children’s Hospital, Columbus, OH, United States
- 3Center for Microbial Pathogenesis, Nationwide Children’s Hospital, Columbus, OH, United States
- 4Department of Pathology, Nationwide Children’s Hospital, Columbus, OH, United States
Introduction: Necrotizing enterocolitis (NEC) is a complex inflammatory disorder of the human intestine that most often occurs in premature newborns. Animal models of NEC typically use mice or rats; however, pigs have emerged as a viable alternative given their similar size, intestinal development, and physiology compared to humans. While most piglet NEC models initially administer total parenteral nutrition prior to enteral feeds, here we describe an enteral-feed only piglet model of NEC that recapitulates the microbiome abnormalities present in neonates that develop NEC and introduce a novel multifactorial definitive NEC (D-NEC) scoring system to assess disease severity.
Methods: Premature piglets were delivered via Caesarean section. Piglets in the colostrum-fed group received bovine colostrum feeds only throughout the experiment. Piglets in the formula-fed group received colostrum for the first 24 h of life, followed by Neocate Junior to induce intestinal injury. The presence of at least 3 of the following 4 criteria were required to diagnose D-NEC: (1) gross injury score ≥4 of 6; (2) histologic injury score ≥3 of 5; (3) a newly developed clinical sickness score ≥5 of 8 within the last 12 h of life; and (4) bacterial translocation to ≥2 internal organs. Quantitative reverse transcription polymerase chain reaction was performed to confirm intestinal inflammation in the small intestine and colon. 16S rRNA sequencing was performed to evaluate the intestinal microbiome.
Results: Compared to the colostrum-fed group, the formula-fed group had lower survival, higher clinical sickness scores, and more severe gross and histologic intestinal injury. There was significantly increased bacterial translocation, D-NEC, and expression of IL-1α and IL-10 in the colon of formula-fed compared to colostrum-fed piglets. Intestinal microbiome analysis of piglets with D-NEC demonstrated lower microbial diversity and increased Gammaproteobacteria and Enterobacteriaceae.
Conclusions: We have developed a clinical sickness score and a new multifactorial D-NEC scoring system to accurately evaluate an enteral feed-only piglet model of NEC. Piglets with D-NEC had microbiome changes consistent with those seen in preterm infants with NEC. This model can be used to test future novel therapies to treat and prevent this devastating disease.
1. Introduction
Necrotizing enterocolitis (NEC) is the leading cause of death due to gastrointestinal disease in premature infants, with a mortality rate as high as 20%–30% for infants requiring surgery (1–3). As described by Bell et al. (4), NEC classically presents in a susceptible preterm infant after the initiation of enteral feeds, with rapid onset of abdominal distension, feed intolerance, bloody diarrhea, and intestinal necrosis in the most severe cases (5, 6). Diagnosis is based on a multifactorial modified Bell's staging criteria, which examines systemic, abdominal, and radiographic manifestations (4). Currently, it is widely accepted that the pathophysiology underlining NEC involves gut immaturity, exaggerated inflammatory response, and bacterial translocation across the intestinal barrier (5). In addition, it has been shown that preterm infants with NEC have intestinal dysbiosis, with an overall increase in Proteobacteria, particularly Enterobacteriaceae, and a decrease in Firmicutes and Bacteroidetes (7). This has been further described in premature piglets with NEC by Touloukian et al. who demonstrated that the disease is associated with ileal dysbiosis, characterized by the overrepresentation of Clostridium species, and members of the Actinobacteria and Cyanobacteria phyla (8). This imbalance in the intestinal microbiota results in blooms of opportunistic pathogens that are known to stimulate toll-like receptor 4 (TLR4) signaling, which is a significant player in the development of this disease (9). Several studies have demonstrated that breast milk plays an important role in preventing the development of NEC through the maintenance of epithelial barrier homeostasis via growth factors, enhancing the colonization of beneficial commensal bacteria in the intestine, and by preventing the expansion of Enterobacteriaceae via maternal immunoglobulin A (IgA) (10, 11). Despite the significant progress made in our understanding of the pathophysiology of NEC, existing therapeutic strategies remain elusive, leaving clinicians unarmed against this devastating disease (2, 10, 11). It is clear that a better understanding of the disease is required for the development of definitive diagnostics and novel efficacious treatments. Hence, several animal models have been developed, with rodents being most commonly used to study NEC. Rodent models often depend on repeated exposures to different stresses to induce NEC, including administration of the Gram-negative bacterial cell wall product lipopolysaccharide (LPS), exposure to hypoxia, exposure to hypothermia, and the administration of formula feeds (12, 13). The use of rodents has multiple advantages, including their small size, ease of maintenance, short gestation, and the abundance of genetically modified animals which has allowed a substantial advancement in the comprehension of NEC pathophysiology. On the other hand, rodents display significant size, developmental and physiologic differences compared to humans, thus limiting the relevance of their use as models to study NEC (14). Therefore, premature piglets have emerged as an alternative to rodent models of NEC. In addition to their body weight being comparable to a preterm human neonate, the piglet intestine more closely resembles the human intestine histologically and physiologically (12, 13, 15, 16). Delivery of preterm piglets at 90% term gestation provides a natural brief period of hypoxia and hypothermia, similar to human deliveries (15, 17). However, unlike rodent models where the activation of TLR4 combined with hypoxic and hypothermic stresses are required for the induction of NEC, premature piglets develop NEC spontaneously upon initiation of enteral feeds, as do human premature infants (12, 15, 16). This was confirmed by Bjornvad et al. in 2008 when they characterized the intestinal changes associated with formula feeds compared to colostrum in preterm piglets (18). Formula-fed piglets had increased mucosal inflammation and loss of mucosal integrity, as is observed in premature neonates with NEC (18). Pigs are also known to have a comparable microbiome to that of humans (19–23) allowing for a more detailed analysis of changes in the microbiome with NEC. In addition, confirmation of results obtained in rodents using a large animal model of disease can be very useful in obtaining Food and Drug Administration (FDA) approval for the testing of novel therapeutic agents in humans.
Several groups have demonstrated that premature piglet models of NEC have reproducible and characteristic clinical changes and intestinal injury similar to that seen in human NEC (8, 24–26). While some groups rely solely on macroscopic damage to assess NEC severity (24, 27, 28), others have quantified the cellular and architectural changes that occur during NEC in piglets, adapting similar criteria to that used in the rat NEC model (24, 27, 28). Interestingly, a microbial analysis revealed that NEC in premature piglets is associated with ileal dysbiosis, characterized by the overrepresentation of Clostridium species, and members of the Actinobacteria and Cyanobacteria phyla (8).
Although piglets have been used to study NEC since 1972, there is still no consensus on the definition of the disease in this model (8), making it difficult to compare the results between different research groups. In addition, current pig NEC models typically utilize total parenteral nutrition (TPN) for the first 48 h of life, followed by enteral feeds (29–31). Here, we present a multifactorial definitive NEC scoring system using a simplified enteral feed-only piglet model of the disease to standardize the global injury that occurs.
2. Materials and methods
2.1. Cesarean delivery of premature piglets
All animal studies were carried out in accordance with the guidelines of the Institutional Animal Care and Use Committee (IACUC) of the Research Institute at Nationwide Children's Hospital (protocol #AR18-00062). White Yorkshire × Landrace sows were purchased from Oak Hill Genetics (Ewing, IL). Sows were acclimatized to our facility for five days before terminal cesarean section delivery, performed on gestational day E104, with full term gestation being E114. Piglets delivered at E104 are roughly equivalent to premature infants born at 32 weeks gestation (16).
Sows were sedated with an intramuscular injection of telazol (0.4–1.0 mg/kg), ketamine (1–2.5 mg/kg), and xylazine (1–2.5 mg/kg), followed by an intramuscular injection of glycopyrrolate (0.01 mg/kg). After endotracheal intubation, anesthesia was maintained throughout the cesarean section using 1%–4% isoflurane. After induction of adequate anesthesia, laparotomy was performed through a lower midline incision to expose the uterus and deliver the piglets. The umbilical cord of each piglet was milked to ensure adequate placental blood transfusion prior to cord ligation. The time from induction of anesthesia to piglet delivery was kept at a minimum to avoid excess exposure of the premature piglets to anesthesia. After all piglets were delivered (typically within 10–15 min after skin incision), the sow was euthanized with IV or intracardiac Euthasol® (1 ml/4.5 kg) and thoracotomy, and the laparotomy closed.
2.2. Piglet resuscitation
Piglets were immediately dried and resuscitated after birth by clearing the remaining mucous from the airways using suction and providing positive pressure bag-mask ventilation as needed. Once piglets showed signs of independent breathing, 1 ml of iron dextran (Vedco, Saint Joseph, MO) was administered intramuscularly to prevent iron deficiency anemia, one drop of sublingual Doxapram (WEST-WARD, Eatontown, NJ) was administered to stimulate respiration, and sublingual glucose paste (Insta-Glucose, Valeant Pharmaceuticals, Bridgewater, NJ) was provided to prevent hypoglycemia. Doxapram was re-dosed as needed to piglets that were slow to breathe independently and require additional stimulation. Once resuscitated, piglets were placed in temperature- and oxygen-controlled small animal intensive care units (Suburban Surgical Co, Wheeling, IL) to maintain a temperature of 35.5°C–39.5°C with FiO2 of 40%. Once stabilized, typically within 60 min, each piglet was weighed and sexed. A 6-French transbuccal orogastric feeding tube (Cardinal Health, Dublin, OH) was introduced into the stomach through a small puncture made with the needle in the cheek. 3-0 polypropylene sutures were used to secure the feeding tube to the cheek, snout, and forehead, with additional securement of the tubes using Elastoplast tape. After tube placement was confirmed by auscultation, piglets received 10 ml of dextrose (25 g) suspended in 500 ml Pedialyte (Abbott, Columbus, OH). Piglets from 4 separate experiments were randomized regardless of sex into two different experimental groups—the colostrum-fed group (total n = 17) and the formula-fed group (total n = 18) (Supplementary Figure S1).
2.3. Induction of NEC
Piglets were fed every 3–4 h for a total of 6–8 feeds/day. The initial volume goal of colostrum or formula feeds was 240 ml/kg/day and was decreased to 120 ml/kg/day in later experiments to avoid potential over-hydration. Oral glucose paste was administered if there was concern for hypoglycemia. Feeding volumes were scaled up over the first two days. 60% of goal volume was administered as colostrum or formula on day 1, with the remaining 40% of the goal volume achieved with Pedialyte supplementation. On day 2, 75% of goal volume was administered as colostrum or formula, with the remaining 25% of the goal volume achieved with Pedialyte. On days 3 to 5, the complete goal volume was administered as colostrum or formula.
Fresh frozen bovine colostrum (BC) was obtained from OSU Waterman Farms (Columbus, OH). The colostrum provided was medium grade in quality, between 20 and 50 mg/ml of immunoglobulins, as assessed on the farm by density measurement using a Brix Refractometer. The colostrum was diluted to a 50% concentration with distilled autoclaved water upon thawing since higher concentrations led to gastric bezoar formation. Piglets in the colostrum-fed group received colostrum for the entire experiment. In contrast, piglets in the formula-fed group received fresh BC for 24 h, followed by Neocate Jr (1.0 kcal/ml; 120 kcal/kg/day) (Nutricia, Zoetermeer, Netherlands) for the remainder of the experiment to induce intestinal injury. All milk products were prepared daily and stored in a 4°C refrigerator. Aliquots were taken before each feed and warmed for 20–30 min prior to administration.
2.4. Quantification of IgG in bovine colostrum
IgG is the major immunoglobulin present in BC (32, 33), and its use as a marker for BC quality is well-documented in the literature (32–36). To further assess colostrum quality, samples were thawed and centrifuged four times at 10,000 g for 20 min each at 4°C to remove cells and fat. BC IgG was quantified using a bovine IgG ELISA kit (Bethyl Laboratories Inc., Montgomery, TX) following the manufacturer's instructions with minor modifications. Samples were diluted to 1:500,000 and plated in triplicate on 96-well plates using 3,3′,5,5′tetramethylbenzidine (TMB) as substrate. Absorbance OD450 nm was measured using a Spectramax M2 microplate reader equipped with SoftMax Pro 5.4 software (Molecular Devices, San Jose, CA). IgG concentrations were calculated using standard curves.
2.5. Clinical sickness scores (CSS)
Piglets were monitored continuously throughout the experiment. Although unblinded to groups, we noted observable differences in the piglets in the two different groups. Thus, we initiated a new scoring system, the clinical sickness score (CSS), to define our observations. CSS were assigned at the time of each feed, beginning at 12 h of life. The CSS was comprised of 4 different categories: motor/tone, verbal, alertness, and body color, with scores ranging from 0 to 2 per category depending on severity, for a maximal total CSS of 8 (Table 1). This was reported as an average CSS for all piglets in each group at each time point. If a piglet was euthanized prior to the end of the experiment, that piglet was given a score of an 8 for all remaining time points of the experiment.
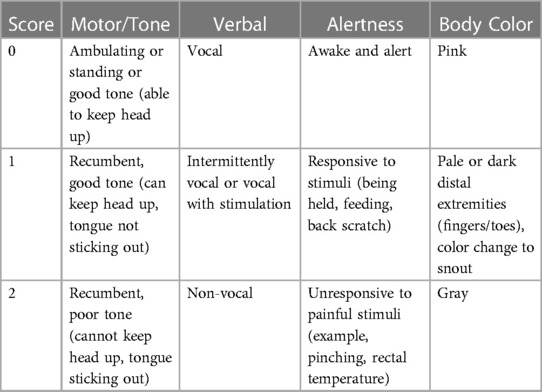
Table 1. Clinical sickness score (CSS). CSS was documented at the time of each feed (every 3–4 h) beginning at 12 h of life. Each piglet received a score of 0–2 in the four different categories (motor/tone, verbal, alertness, and body color) for a total CSS of 0–8.
2.6. Euthanasia and necropsy
In alignment with our IACUC protocol, piglets were euthanized if they lost more than 25% of their birth weight, had a single temperature >40.5°C, or were noted to have extreme lethargy. Additionally, piglets were euthanized if two of the following were observed during two consecutive feeds: loss of >20% of birth weight, significant lethargy/decreased activity, temperature >40°C, bloody diarrhea, abdominal distension, emesis, or rapid, labored, or shallow breathing. Euthanasia was performed using an intramuscular injection of ketamine (0.3–0.6 ml/kg) and xylazine (0.1–0.2 ml/kg), followed by an intraperitoneal injection of Euthasol® (1–2 ml/kg).
2.7. Gross injury scoring
After euthanasia, a midline laparotomy was performed, and the intestine examined in situ. A gross injury score was assigned to the small intestine (proximal, mid, and distal segments) and colon based on the most severely affected area assessed independently by two graders using an adaptation of a published scoring system (16, 37). Scores ranged from 1 (no injury) to 6 (most severe injury) using the criteria shown in Table 2. The highest overall score assigned to any segment was used to assign the animal to either no gross injury (grades 1–3) or gross injury (grades 4–6) (Figures 1A,B) (16, 37).
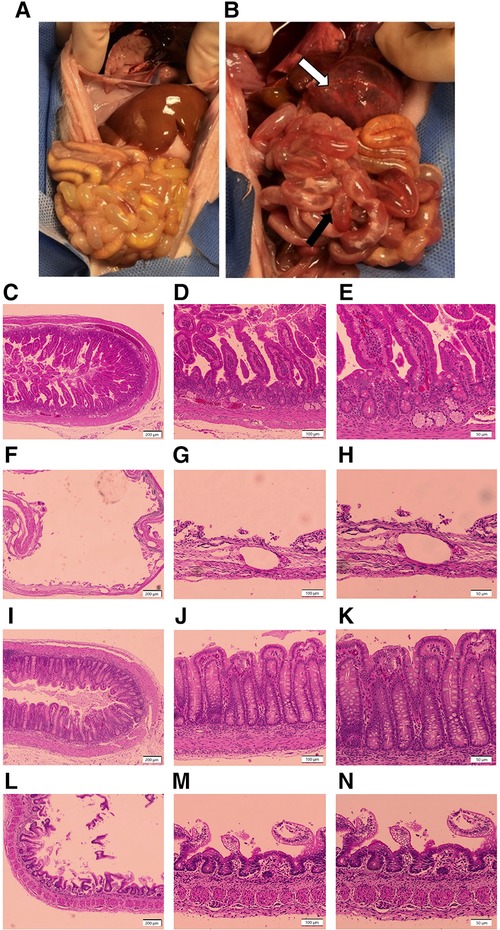
Figure 1. Representative gross and histologic intestinal images. (A,B) Gross Injury. (A) Image from a healthy piglet from the colostrum-fed group with no intestinal injury, corresponding to a gross injury score of 1; (B) image from a piglet in the formula-fed group, with extensive hemorrhage of the stomach (white arrow), and hyperemia and edema with focal areas of pneumatosis intestinalis in the mid small bowel (black arrow), corresponding to a gross injury score of a 5. (C–N) Histologic Injury. (C–E) Image of healthy small intestine from a piglet in the colostrum-fed group with no evidence of damage, corresponding to a histologic injury score of 1 at (C) 4×, (D) 10×, and (E) 20× magnification; (F–H) image of small intestine from a piglet in the formula-fed group demonstrating transmural necrosis, scant villi, and widespread pneumatosis, corresponding to a histologic injury score of 5 at (F) 4×, (G) 10×, and (H) 20× magnification; (I–K) image of healthy colon from a piglet in the colostrum-fed group with no evidence of damage, corresponding to a histologic injury score of 1 at (I) 4×, (J) 10×, and (K) 20× magnification; (L–N) image of colon from a piglet in the formula-fed group demonstrating mucosal sloughing with red blood cell infiltration, corresponding to a histologic injury score of 4 at (L) 4×, (M) 10×, and (N) 20× magnification.
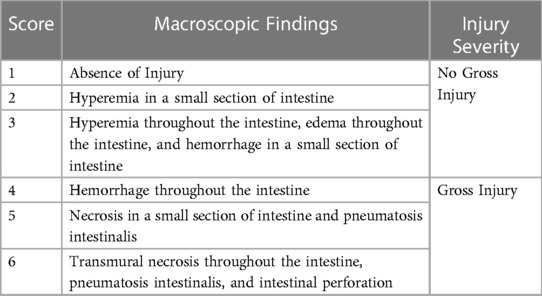
Table 2. Gross injury scoring system. Gross injury scores were assigned to the proximal small intestine, mid small intestine, distal small intestine, and colon of each animal. Scores of 1–3 were considered to have no gross injury, and scores of 4–6 were considered to have gross injury. The final score assigned to an animal was the highest score in any given section.
2.8. Histologic injury scoring
Tissue was collected from the small intestine (proximal, mid, and distal) and colon, fixed in 10% formalin (Fisher Scientific, Pittsburgh, PA) for 24 h, followed by 70% ethanol (Fisher Scientific, Waltham, MA). Samples were paraffin-embedded and stained with hematoxylin and eosin (H&E). Histologic sections were reviewed by light microscopy by at least two independent graders, including a board-certified pediatric pathologist, in a blinded fashion. Histologic injury scores were assigned based on a previously published grading system (28). Scores ranged from 1 (no damage) to 5 (transmural necrosis) (Table 3). The final score assigned to any animal was the highest score identified in any intestinal segment, with scores of 3 or above consistent with NEC. Scores were categorized as no histologic injury (grades 1–2), moderate histologic injury (grades 3–4), or severe histologic injury (grade 5) (Figures 1C–N). Images were taken using an Olympus U-Tv0.5XC-3 microscope (Tokyo, Japan).
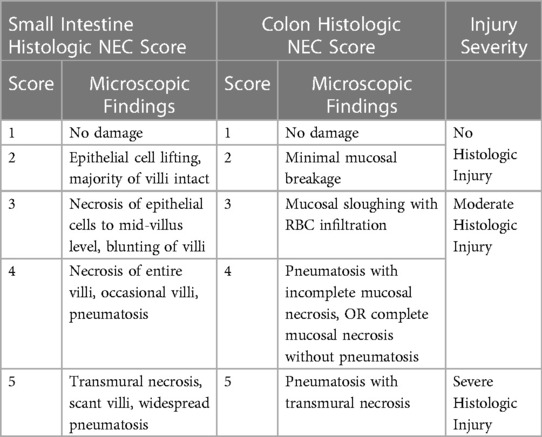
Table 3. Histologic injury scoring system. Histologic injury scores were assigned to the proximal small intestine, mid small intestine, distal small intestine, and colon of each animal. The final score assigned to an animal was the highest score in any given section. Scores of 1–2 were considered no histologic injury, scores of 3–4 were considered moderate histologic injury, and a score of 5 was considered severe histologic injury.
2.9. Bacterial translocation
Liver, spleen, and mesenteric lymph nodes were collected in a sterile fashion at the time of necropsy, snap frozen, and stored at −80°C until use. Samples were thawed on ice, weighed, and homogenized in 2 ml of PBS before plating 50 µl of the homogenized mixture on brain heart infusion (BHI) plates for overnight, aerobic incubation at 37°C. BHI is a general-purpose growth medium for culturing non-fastidious Gram-positive and Gram-negative bacteria (38). The number of colony-forming units (CFUs) for each organ was recorded after 24 h of growth and standardized per gram of tissue.
2.10. Definitive NEC (D-NEC) scoring
Piglets were determined to have D-NEC if they met at least 3 of the following four criteria: Gross injury score ≥4 of a maximum of 6, histologic injury score ≥3 of a maximum of 5, CSS ≥5 of a maximum of 8 in the last 12 h of life, and bacterial translocation of ≥1 CFU/g of tissue to two or more internal organs (liver, spleen, mesenteric lymph nodes).
2.11. RNA isolation, cDNA synthesis, and quantitative real-time PCR (qRT-PCR)
Intestinal specimens of proximal, mid, and distal small bowel and colon were collected during necropsy and stored at −80°C until use. Total RNA was isolated using the Purelink RNA mini kit (Thermo Fisher, Waltham, MA) according to the manufacturer's instructions. Samples were thawed on ice and weighed. Tissues (0.5–0.9 g) were homogenized in lysis buffer with 1.0 mm Zirconia beads (Biospec Products, Bartlesville, OK) in a TissueLyser II (Qiagen, Germantown, MD) at 30 Hz for 1 min, repeated six times. cDNA was synthesized using the Superscript IV VILO cDNA synthesis kit with ezDNAse Enzyme to remove genomic DNA (Thermo Fisher, Waltham, MA). Quantitative real-time PCR (qRT-PCR) was performed using primers for interleukin-1α (IL-1α) and IL-10, and PowerUp SYBR Green PCR Master mix (Thermo Fisher, Waltham, MA) using a QuantStudio™ 3 System (ThermoFisher, Waltham, MA) (Table 4) (39–41). Hypoxanthine-guanine phosphoribosyltransferase (HPRT) (Integrated DNA Technologies, Coralville, IA) was used as an endogenous control The cycling program consisted of a 2-minute hold stage at 50°C, a 10-minute hold stage at 95°C, followed by 40 cycles of 15 s at 95°C followed by 1-min at 60°C. Target gene expression was analyzed using the 2−ΔΔCt method.
2.12. DNA extraction and 16S rRNA gene sequencing
Intestinal contents were aseptically collected from the colon of all piglets at sacrifice and frozen at −80°C until use. Approximately 100 mg of colonic content was subjected to DNA extraction using the QIAamp DNA Mini Kit (Qiagen, Hilden, Germany) per manufacturer's instructions with slight modifications. Contents were incubated for 45 min at 37°C in lysozyme-mutanolysin buffer (pH 8.0) containing 22 mg/ml lysozyme, 0.1 U/ml mutanolysin, 20 mM TrisHCL, 1.2% Triton-x (Sigma Aldrich, St. Louis, MO), and 2 mM EDTA (Thermo Fisher Scientific, Waltham, MA), followed by homogenization for 150 s with 0.1 mm zirconia beads. Samples were incubated at 95°C for 5 min with InhibitEx Buffer, then incubated at 70°C for 10 min with Proteinase K and Buffer AL (Qiagen). Following this step, the QIAamp DNA Mini Kit isolation protocol was followed beginning with the ethanol step. DNA was quantified with the Qubit 2.0 Fluorometer (Life Technologies, Carlsbad, CA) using the dsDNA Broad Range Assay Kit and stored at −20°C prior to 16S rRNA gene sequencing.
Colonic content DNA samples were submitted to the Genomic Services Core at the Institute for Genomic Medicine at Nationwide Children's Hospital (Columbus, OH) for library preparation and high-throughput sequencing. Paired-end (300 nt forward and reverse) sequences of the V4 hypervariable region of the 16S rRNA gene (515F-806R) were generated by Illumina MiSeq. Quantitative Insights Into Microbial Ecology (QIIME) 2.0 (42) and DADA2 (43) were utilized for downstream amplicon processing, quality control, diversity analyses, and taxonomic assignment using a trained classifier built from the ribosomal RNA database SILVAv138 (44, 45). For quality control, sequences were truncated from the 3′ end to 280 nt (forward reads) or 250 nt (reverse reads) to achieve a quality score of at least 20, and the first 20 nt were trimmed from the 5′ end of both forward and reverse reads as these early positions may contain errors. Sequences that did not meet these criteria were discarded. After quality control, taxa denoted as eukaryotic or “unassigned” were also removed. Subsequent diversity analyses were carried out at a sequencing depth of 18,755 sequences per sample. Evenness, richness (number of observed species), and Shannon's Diversity Index (46) were calculated to measure alpha diversity within samples on the basis of feeding regimen and occurrence of NEC. The weighted UniFrac distance matrix (47) was applied to measure beta diversity, and the EMPeror software package (48) was used to construct three-dimensional principal coordinate (PCoA) plots to visualize differences based on feeding regimen and occurrence of NEC. Relative abundance was calculated for the class, family, and genus taxonomic levels on an individual sample basis as well as abundances within feeding regimen and occurrence of NEC.
2.13. Statistical analyses
All statistical analyses were performed using GraphPad Prism, Version 9 (GraphPad Software, Inc. La Jolla, CA). Nonparametric analysis between groups was performed using the Mann–Whitney U-test. Survival data were analyzed using a log-rank (Mantel-Cox) test.
Differences in bacterial community composition were assessed by comparing alpha and beta diversity between feeding regimens (i.e., colostrum fed vs. formula fed) and between pigs that developed NEC vs. those with No NEC collapsed across the two feeding regimens. Evenness, richness, and Shannon Diversity Index alpha diversity metrics used the Kruskal-Wallis test for pairwise comparisons. Differences in beta diversity weighted UniFrac distances were analyzed by permutational multivariate analysis of variance (PERMANOVA) with 999 randomizations of the data. Relative abundance data were analyzed using the Kruskal-Wallis test for pairwise comparisons, and taxa that were present in fewer than 10% of samples (present in fewer than 3/26 samples) were omitted from abundance analyses. The statistical alpha level was set to 0.05 for all analyses.
3. Results
3.1. Bovine colostrum feeding prevents the early death of premature piglets
In preliminary experiments, piglets that were exclusively fed with commercial human formulas were found to have a rapid deterioration of their health with early death within the first 24 h of life. We investigated the use of Sow Colostrum Replacer (APS LaBelle, Phoenix, AZ), but this had a minor effect on preventing early death. Unlike infants, piglets do not undergo maternal-placental transfer of immunoglobulins and instead depend almost exclusively on postnatal transfer of maternal IgG via colostrum (13, 49–52). We ultimately chose bovine colostrum because of its high levels of beneficial immunoglobulins to help protect against early animal mortality, and human breast milk or porcine colostrum were not available in the volumes needed. Piglets subsequently received BC for 24 h prior to the introduction of formula feeding to induce NEC. This led to a significant improvement in early mortality, however, the effect was variable depending upon the lot of BC used. Based on this, we investigated the IgG content of the different BC lots by ELISA. Consistent with previous reports, we found that the efficacy of the colostrum in preventing early death was dependent upon its IgG content (33). We measured the IgG content in 8 different batches of colostrum from 7 sows. The range of IgG varied widely, from 1.123 mg/ml to 94.898 mg/ml. We found that colostrum with IgG levels greater than 5 mg/ml reduced early death (data not shown). Thus, for all subsequent experiments, we used colostrum that contained a minimum of 5 mg/ml of IgG.
In addition to the use of BC, we found that rapid piglet delivery to decrease anesthesia exposure time, the use of oxygen and temperature-controlled incubators, and the administration of iron dextran to prevent iron deficiency anemia, doxapram to simulate respiration, and sublingual glucose to prevent hypoglycemia significantly improved piglet survival during the first 24 h of life.
3.2. Formula feeding adversely affects intestinal health in premature piglets
Piglets in the formula-fed group had increased all-cause mortality compared to piglets in the colostrum-fed group, regardless of sex (Figure 2A). Furthermore, piglets in the formula-fed group lost significantly more weight compared to piglets in the colostrum-fed group (−9.9% vs. +5.3%, p = 0.0001) (Figure 2B) and had significantly higher CSS than piglets in the colostrum-fed group (Figure 3A).
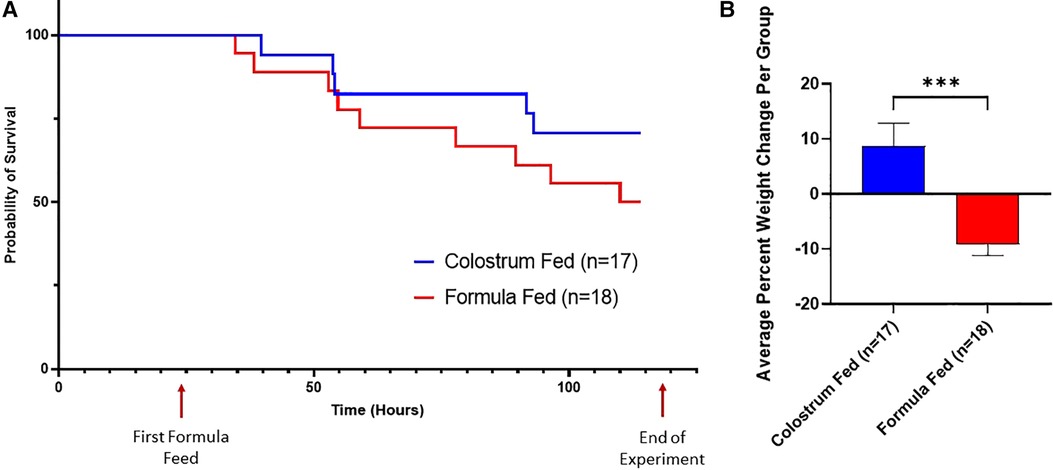
Figure 2. Survival curves and average percent weight change. (A) Survival Curves. These survival curves depict all-cause mortality (NEC plus non-NEC deaths) over the course of the experiment. (B) Weight Change. The percent weight change per piglet was averaged for each group. Variability of sample means was reported as a standard error of the mean (SEM) and statistical significance level was set to α = 0.05. Statistical analysis was carried out using the Mann–Whitney U-test (***p = 0.0001).
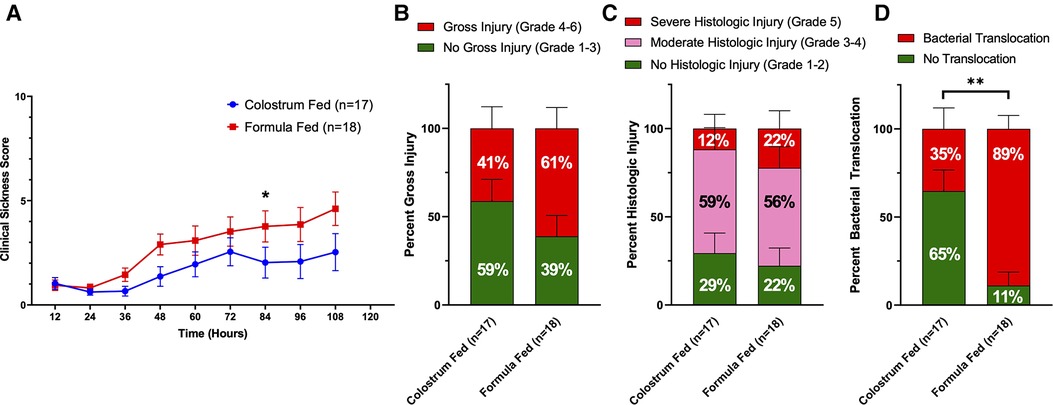
Figure 3. Components of D-NEC score. (A) Clinical Sickness Scores (CSS). Each data point represents the average CSS for all piglets in that group. If a piglet was euthanized prior to the end of the experiment, that piglet was given a score of an 8 for all remaining time points of the experiment; (B) Gross Injury Scores; (C) Histologic Injury Scores; (D) Bacterial Translocation. Variability of sample means was reported as a standard error of the mean (SEM) and statistical significance level was set to α = 0.05. Statistical analyses for gross injury score, histologic injury score, and bacterial translocation were carried out using Sidak’s multiple comparison test (***p < 0.001). p values were calculated at each timepoint for CSS using the Mann–Whitney U-test (*p = 0.05).
Upon necropsy, we found that 61% (11 of 18) of animals in the formula-fed group had gross evidence of intestinal injury consistent with NEC, compared to 41% (7 of 17) of animals in the colostrum-fed group (Figure 3B). The highest degree of gross injury in both groups was seen in the mid-small bowel, with an average gross injury score of 3.3 in the formula-fed group and 2.5 in the colostrum-fed group. The lowest degree of gross injury in both groups was seen in the proximal small bowel, with an average score of 1.9 in the formula-fed group and 1.7 in the colostrum-fed group (Supplementary Figure S2). While these findings were not statistically significant, average gross injury in all sections of bowel was greater in the formula-fed group than the colostrum-fed group.
Histological examination of the intestine revealed that 78% (14 of 18) of animals in the formula-fed group had histologic intestinal injury consistent with NEC, with 56% having moderate NEC and 22% having severe NEC (Figure 3C). 71% (12 of 17) of animals in the colostrum-fed group had histologic injury consistent with NEC, with 59% having moderate NEC and 12% having severe NEC. In both groups, the mid-small bowel was found to have the highest degree of histologic injury, with an average score of 3.2 in the formula-fed group and 2.9 in the colostrum-fed group (Supplementary Figure S2). While these findings were not statistically significant, average histologic injury in all sections of bowel was greater in the formula-fed group than the colostrum-fed group.
Since the intestinal injury that occurs with NEC results in gut barrier failure, we next assessed intestinal barrier function by detecting bacterial translocation to the liver, spleen, and mesenteric lymph nodes. Bacterial translocation was detected in 89% of piglets in the formula-fed group compared to only 35% of piglets in the colostrum-fed group (p = 0.0016) (Figure 3D).
3.3. Formula feeding increases Definitive NEC (D-NEC), increases death due to NEC, and promotes colonic inflammation in premature piglets
D-NEC criteria were met if a piglet met at least 3 of the following 4 criteria: gross injury score of ≥4, histologic injury score of ≥3, CSS of ≥5 in the last 12 h of life, or bacterial translocation to ≥2 internal organs (liver, spleen, mesenteric lymph nodes). 77% (12 of 18) of animals in the formula-fed group had D-NEC compared to 24% (4 of 17) of animals in the colostrum-fed group (p = 0.0176) (Figure 4A). This was not affected by the sex of the piglet.
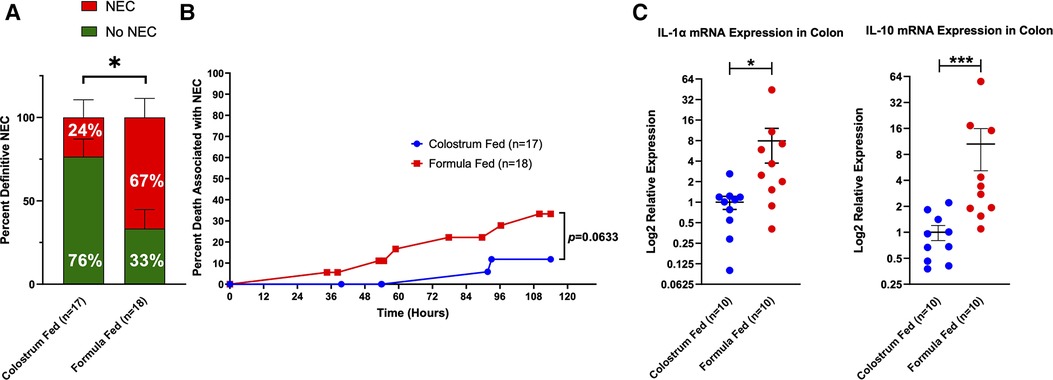
Figure 4. Definitive NEC (D-NEC) incidence, percent death associated with NEC, and colonic inflammation. (A) Incidence of D-NEC. Statistical analysis was carried out using the Mann–Whitney U-test (*p = 0.0176); (B) Percent Death Associated with NEC. Each data point represents death of a piglet that was determined to have D-NEC. Piglets that survived to the end of the experiment and were found to have D-NEC were not included in this graph; (C) Colonic Inflammation. Expression levels of IL-1α and IL-10 mRNA in the colon were determined by qRT-PCR. Data were analyzed using the Mann–Whitney U-test (*p = 0.0115, ***p = 0.0005). Variability of sample means was reported as a standard error of the mean (SEM) and statistical significance was set to an α = 0.05.
Piglets in the formula-fed group tended to die during the experiment due to D-NEC more frequently than piglets in the colostrum-fed group (33.3% vs. 11.8%) (Figure 4B). qRT-PCR was performed on samples of the mid and distal small bowel and colon from 10 piglets in each group. There was significantly increased mRNA expression for IL-1α (p = 0.0115) and IL-10 (p = 0.0005) in colon specimens from the formula-fed group compared to the colostrum-fed group (Figure 4C).
3.4. Formula feeding and NEC in premature piglets are associated with reduced diversity of the intestinal microbiome
To determine the influence of the feeding regimen and the impact of NEC on colonic microbial diversity within samples, alpha diversity was assessed based on evenness, richness, and Shannon Diversity Index, which accounts for both evenness and richness. Formula-fed piglets had a lower mean evenness score than colostrum-fed piglets (p = 0.054) (Figure 5A), as well as a significant decrease in richness (p = 0.002) (Figure 5B). Consistent with the observations of evenness and richness, the overall diversity within samples as denoted by the Shannon Diversity Index was significantly lower in the formula-fed relative to the colostrum-fed piglets (p = 0.003) (Figure 5C). When collapsed across feeding types, piglets that developed NEC during the experimental period had significantly reduced evenness (p = 0.012) and richness (p = 0.038) within colonic microbial communities relative to piglets that did not develop NEC (Figures 5D,E). Similarly, the Shannon Diversity Index was significantly reduced in piglets that developed NEC (p = 0.006) (Figure 5F). Analogous significant results were obtained when other alpha diversity measures were evaluated, including ACE, and Simpson Diversity Indices, but not Faith Phylogenetic Diversity (Supplementary Figure S3).
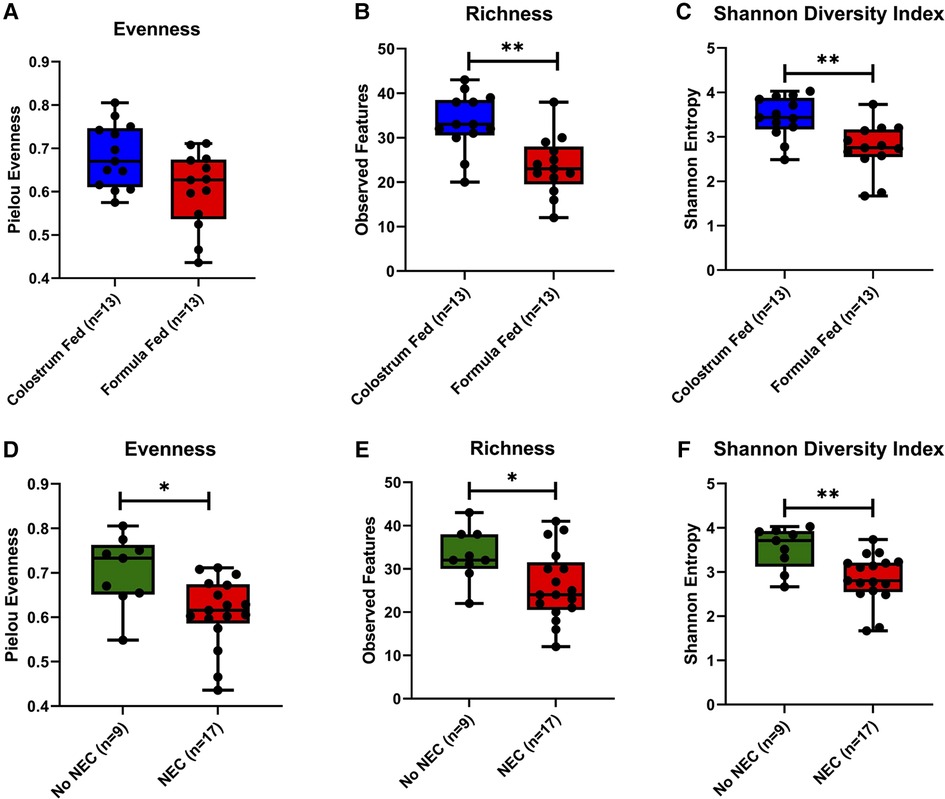
Figure 5. Microbial alpha diversity in piglet colonic contents. (A,D) Evenness within feeding regimen (p = 0.054) or occurrence of NEC (p = 0.012). (B,E) Richness (number of observed features) within feeding regimen (p = 0.002) or occurrence of NEC (p = 0.038). (C,F) Shannon Diversity Index within feeding regimen (p = 0.003) or occurrence of NEC (p = 0.006). Pairwise comparisons between groups were made using the Kruskal-Wallis test (p < 0.05). Data are represented as box and whisker plots that denote minimum, maximum, and interquartile range values.
The weighted UniFrac distance matrix was constructed to characterize changes in the community and phylogenetic composition. Both feeding regimens (p = 0.019) and NEC (p = 0.002) were associated with significant changes in colonic content beta diversity. Importantly, a notable amount of clustering was associated with a positive NEC diagnosis (Figure 6). Thus, feeding regimen and NEC exerted independent influences on the bacterial composition within the colonic environment resulting in phylogenetically distinct microbial profiles. Comparable results were observed upon assessment of additional beta diversity metrics, including Bray-Curtis dissimilarity and Jaccard distances, but no differences were observed for the unweighted UniFrac distance matrix (Supplementary Figure S4).
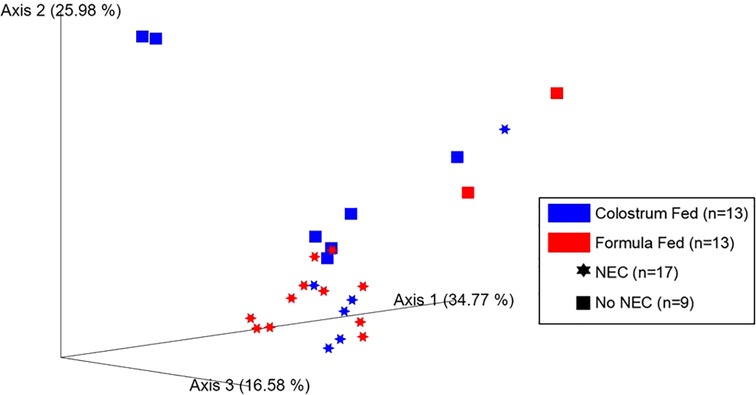
Figure 6. Microbial beta diversity in piglet colonic contents. Principal coordinate analysis (PCoA) plot of beta diversity clustering based on feeding regimen (p = 0.019, PERMANOVA using 999 randomizations of the data) and occurrence of NEC (p = 0.002, PERMANOVA using 999 randomizations of the data) derived from the weighted UniFrac distance matrix.
3.5. NEC in piglets is associated with a significant change in microbial communities in the intestine
The relative abundances of specific bacterial taxa (at the class, family, and genus levels) were compared between the formula-fed and the colostrum-fed piglets, and between piglets that had or had not developed NEC (collapsed across the two feeding regimens). Taxa relative abundance bar plots were also generated at each of the previously described phylogenetic levels on an individual sample basis for feeding regimen (Supplementary Figures S5A–C) and occurrence of NEC (Supplementary Figures S6A–C).
Class level taxa were primarily dominated by Bacilli, Gammaproteobacteria, and Clostridia (Figures 7A,B). While there were no differences in the relative abundances of bacterial classes based on feeding regimen, there was a significant increase in the relative abundance of Gammaproteobacteria in piglets with NEC relative to piglets without NEC (p = 0.038) (Figure 7C). Enterobacteriaceae, Clostridiaceae, and Enterococcaceae predominated at the family classification level (Figures 8A,B). However, significant changes in the relative abundances of bacterial families occurred based on the two experimental factors (Figures 8C,D). The relative abundance of Lactobacillaceae was surprisingly higher in formula fed piglets than in colostrum fed piglets (p = 0.017). In contrast, colostrum-fed piglets had significantly increased relative abundance of Lachnospiraceae (p < 0.001), Peptostreptococcaceae (p < 0.001), Ruminococcaceae (p = 0.030), Moraxellaceae (p = 0.040) and Pseudomonadaceae (p = 0.001), (Figure 8C). Further, Lachnospiraceae (p = 0.013) and Peptostreptococcaceae (p = 0.047) were significantly more abundant in piglets without NEC. However, in piglets that developed NEC, Clostridiaceae (p = 0.029), and, importantly, Enterobacteriaceae (p = 0.01) abundances were significantly elevated relative to healthy piglets (Figure 8D). Similar to family level taxonomy, the most prevalent genera were similar when assessing based on feeding regimen or occurrence of NEC (Figures 9A,B). However, the relative abundances of bacterial genera differed between the two factors (Figures 9C,D). While ten genera were significantly different based on feeding (Figure 9C), the most notable was Lactobacillus (p = 0.017), which was elevated in formula-fed piglets, thus recapitulating family findings. Epulopiscium (p < 0.001), Pseudomonas (p = 0.001), Pygmaiobacter (p = 0.030), and Lactococcus (p = 0.001) were increased in colostrum-fed piglets (Figure 9C). Epulopiscium was also significantly higher in piglets without NEC (p = 0.013) (Figure 9D). In piglets that were positive for NEC, there was a significant increase in the abundance of Clostridium sensu stricto 1 (p = 0.025), Clostridium sensu stricto 2 (p = 0.021) and Clostridium sensu stricto 13 (p = 0.017) (Figure 9D). Additionally, an undefined genus of Enterobacteriaceae was increased in NEC-positive piglets with a trend towards significance (p = 0.056). Collectively, taxonomic abundance data indicate that colostrum and formula feeding differentially influence pioneer colonization within the colonic environment. Additionally, this pioneer colonization may, to an extent, also affect the distribution and prevalence of taxa seen here to be associated with the onset of NEC.
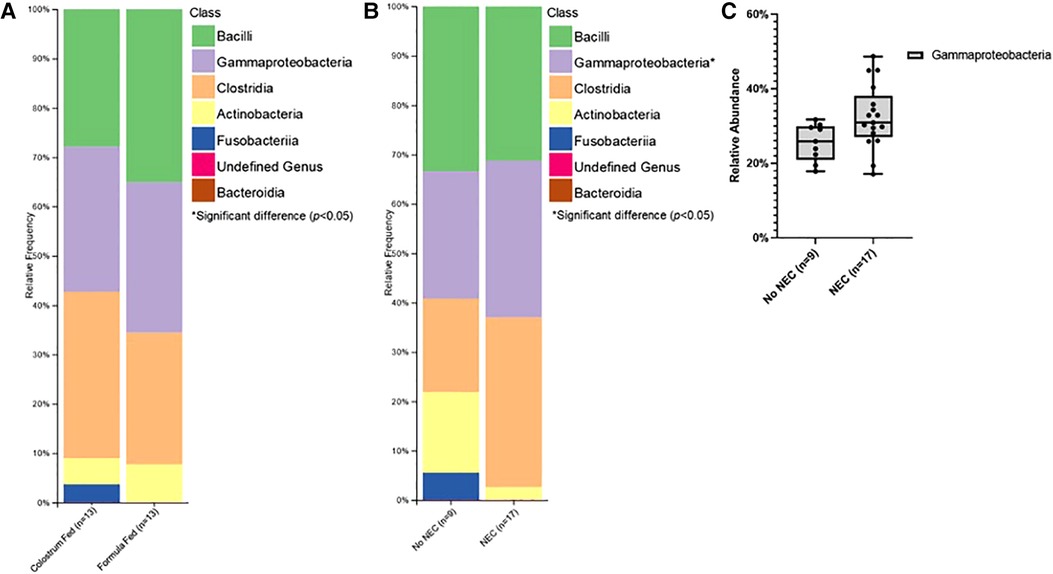
Figure 7. Class level taxonomic abundance. Class level taxa distribution within (A) feeding regimen and (B) occurrence of NEC. Bars represent mean relative percentage of each corresponding bacterial class. (C) Distribution of significantly different classes within incidence of NEC as box and whisker plots that denote minimum, maximum, and interquartile range values. For all relative abundance data, pairwise comparisons between groups were made using the Kruskal-Wallis test (p < 0.05).
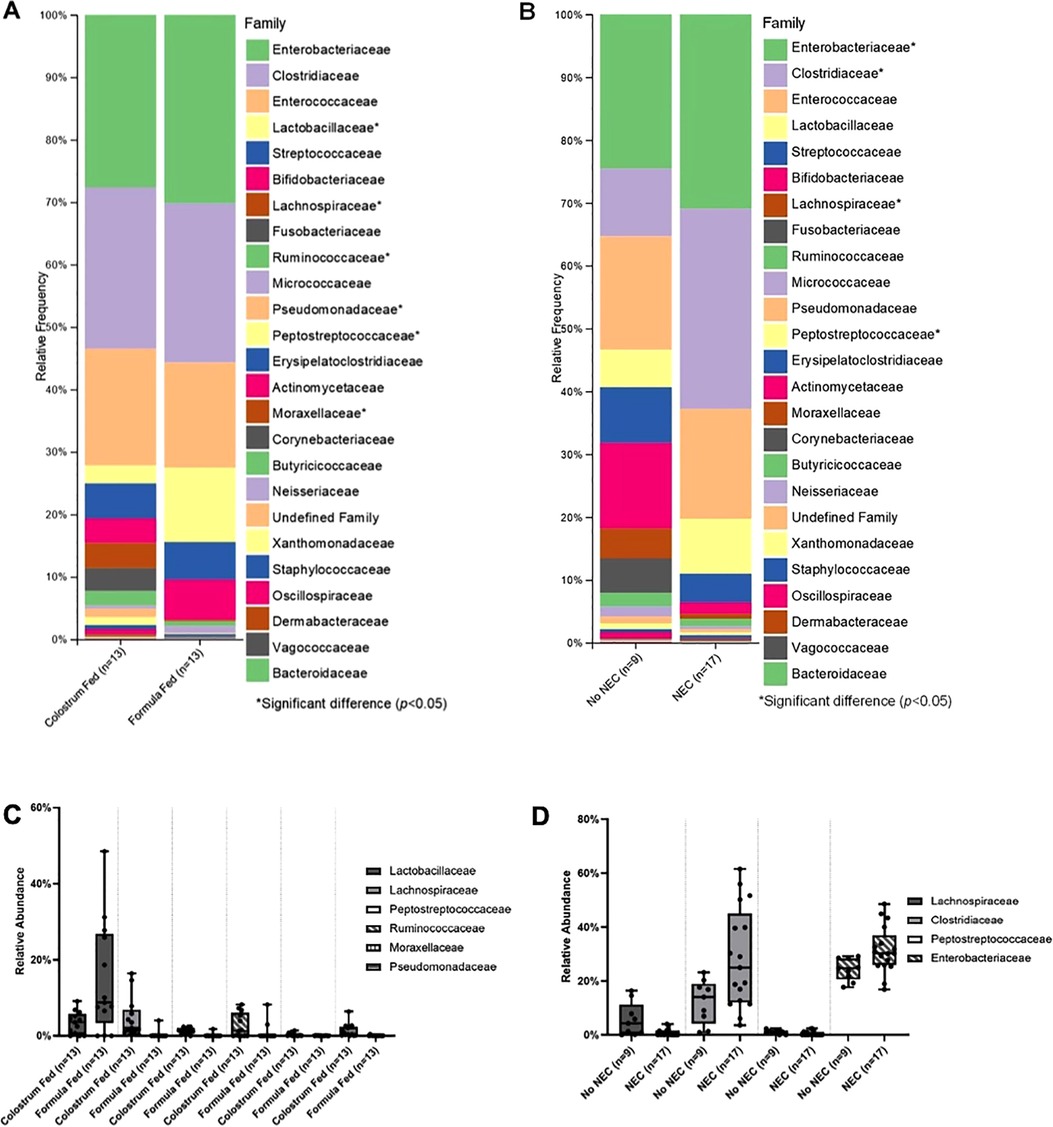
Figure 8. Family level taxonomic abundance. Family level taxa distribution within (A) feeding regimen and (B) occurrence of NEC. Bars represent mean relative percentage of each corresponding bacterial family. Distribution of significantly different families within (C) feeding regimen and (D) occurrence of NEC as box and whisker plots that denote minimum, maximum, and interquartile range values. For all relative abundance data, pairwise comparisons between groups were made using the Kruskal-Wallis test (p < 0.05).
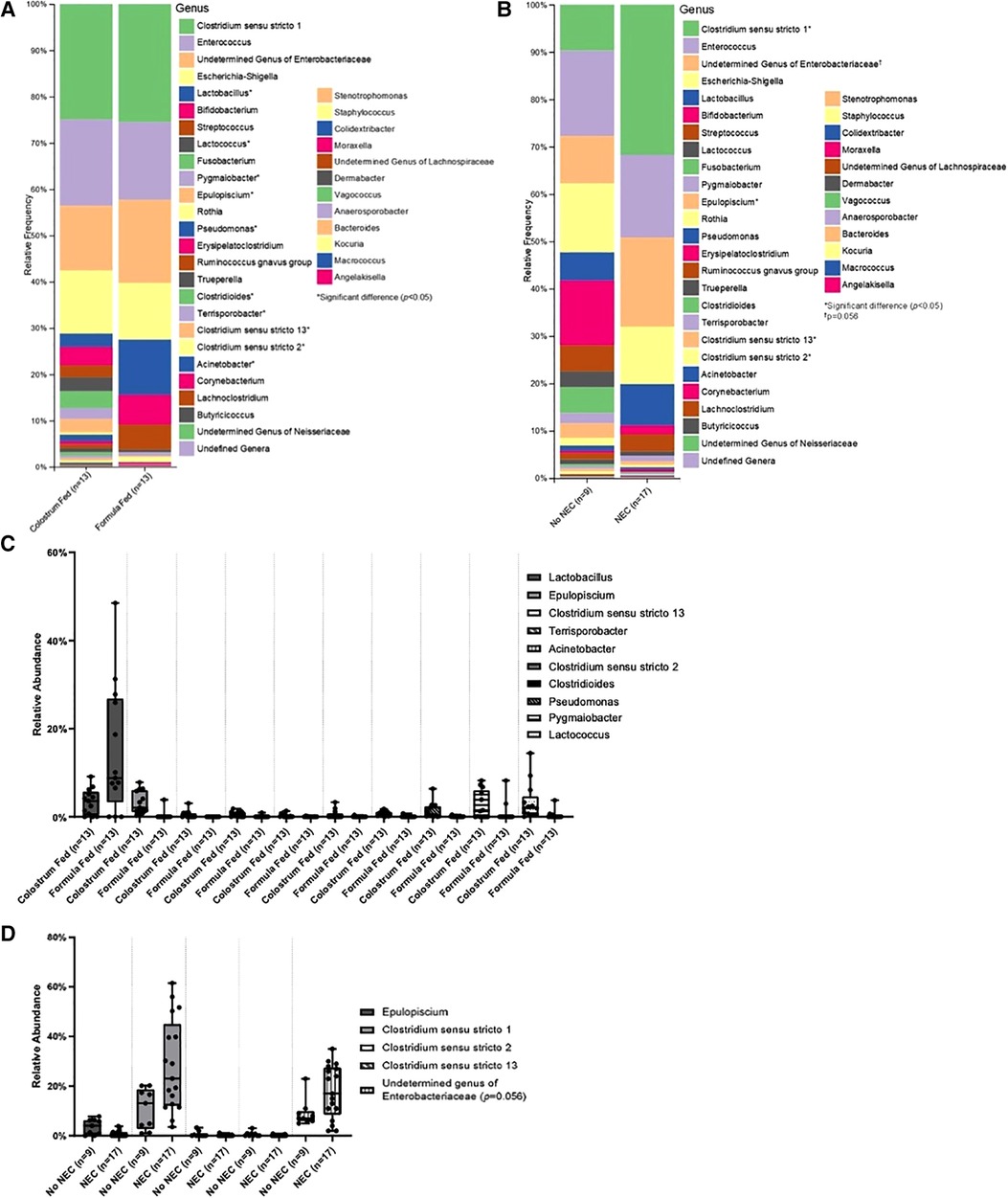
Figure 9. Genus level taxonomic abundance. Genus level taxa distribution within (A) feeding regimen and (B) occurrence of NEC. Bars represent mean relative percentage of each corresponding bacterial genera. Distribution of significantly different genera within (C) feeding regimen and (D) occurrence of NEC as box and whisker plots that denote minimum, maximum, and interquartile range values. For all relative abundance data, pairwise comparisons between groups were made using the Kruskal-Wallis test (p < 0.05).
4. Discussion
Given the complexity of NEC pathophysiology, the development of animal models emulating different facets of the human disease is a critical step towards better disease understanding, and a prerequisite to efficient preclinical drug testing. Here, we describe a new model of NEC using premature piglets that are exclusively orally fed, eliminating the need for TPN, and that utilizes BC during the initial 24 h. Our preliminary studies revealed that exclusive formula feeding of premature piglets resulted in rapid mortality within the first 24 h of life, limiting the possibility of testing novel therapeutic strategies in laboratory settings. Given the importance of maternal antibodies in the protection against NEC, we then used BC to confer transient protection during the first hours of life. The subsequent transition from colostrum to formula resulted in the development of intestinal injury that is similar to human NEC. However, the protection conferred by BC allows for improved early survival and the use of our model in testing potential novel therapeutics. Unlike the commonly used rodent models, where a combination of TLR4 activation and hypoxic stress are required for the induction of the disease, NEC occurs spontaneously with formula feeding in the piglet model, making it more physiologically relevant. Interestingly, despite a significant reduction in NEC incidence with colostrum-only feeding, some animals in this group still developed the disease. It is possible that this is partially due to the use of BC as opposed to porcine colostrum. Although it does not typically occur this quickly, this is also observed with humans, where despite a substantial decrease in NEC incidence observed with exclusive breastfeeding, some infants can still develop the disease, likely due to etiologies that are still obscure (10, 11).
According to Center for Disease Control (CDC) data from 2017, the prevalence of infants receiving any breast milk in 48 states and the District of Columbia was 83.9% overall and varied by gestational age (53). Looking at specific age groups, 71.3% of extremely preterm infants (20–27 weeks), 76.0% of early preterm infants (28–33 weeks), 77.3% of late preterm infants (34–36 weeks), and 84.6% of term infants (≥37 weeks) received breast milk (53). Many preterm infants struggle to gain weight appropriately after birth due to immaturity of the gastrointestinal tract, feeding difficulties (i.e., immature sucking movements, low breastmilk supply), and a lack of appropriate hepatic glycogen stores (54, 55). Thus, to ensure that these infants receive adequate nutrition during this time, TPN is administered via a central venous catheter. Despite the high percentage of preterm infants receiving any breast milk, the incidence of proven or severe NEC in the United States is estimated to be approximately 1–3 per 1,000 live births (56). Unfortunately, NEC frequently occurs in susceptible preterm infants following the administration of enteral feeds. Studies conducted in premature piglets suggest that maltodextrin in human infant formulas is partly responsible for intestinal injury during NEC (57, 58). Thus, initial TPN administration followed by initiation of enteral feeds has been used in several established models of NEC in swine (16, 18, 24, 25, 31, 59, 60). Often, these studies continue the administration of TPN at lower rates while simultaneously administering enteral feeds for 18–120 h (18, 29–31, 37, 59–63). On the other hand, other investigators have developed piglet NEC models using enteral feeds exclusively for NEC induction (64–66). Good et al. used cultured enteric bacteria isolated from a patient with surgical NEC to induce intestinal lesions in neonatal mice and premature piglets (65). Similarly, Roy et al. directly administered fermented formula through a catheter inserted through a gastrostomy directly into the duodenum resulting in NEC-like disease within 6 h in piglets (66). Regardless of the model, the initiation of enteral feeds seems to trigger NEC, leading to rapid fatalities with as high as a 70% mortality rate within 48 h following the initial administration of formula (18, 24–26, 31). The severity of the disease and the subsequent rapid mortality in these models drastically limit the window of therapeutic intervention in the context of investigational studies aiming to assess the efficacy of novel treatments, making our model a potentially better option. In addition, while breast milk is known to be protective against NEC due to the presence of maternal antibodies, there are still some infants that exclusively receive breast milk and still develop NEC. Our model mimics these conditions, as we still observed some NEC in piglets in the colostrum-fed group, despite receiving beneficial antibodies from colostrum administration.
Traditionally, the severity of NEC in piglets has been assessed using gross injury scoring which depicts macroscopic damage of the intestine, or histologic injury scoring, or a combination of both (18, 24–26, 29–31, 37, 59, 61–63, 65, 68). During our study, it was common to find piglets with high gross injury and high histologic injury scores that appeared clinically healthy throughout the entire experiment and did not show bacterial translocation to internal organs. Moreover, the histologic injury score did not reflect the extent of damage throughout the length of the intestine, as the tissue specimens collected for histological assessment, as in previous studies using piglet NEC models, were obtained from the areas of the intestine displaying the most severe macroscopic lesions (24–26, 31, 64, 65, 68). Therefore, we developed a new D-NEC score that entailed a clinical sickness scoring system and assessment of bacterial translocation (5, 67). This scoring system also reduces the likelihood of attributing death during the experiment to other potential etiologies common in premature piglets, such as lung immaturity, inadequate thermoregulation, and sepsis (16). A similar approach using a multi-component scoring system that included gross injury, radiographic findings on abdominal x-ray after euthanasia, and pre-mortem clinical signs of NEC was used by Azcarate-Peril et al. (64). A multi-component scoring system is also reminiscent of that first described by Bell and used by Neonatologists to diagnose patients with NEC (4). In agreement with previous reports, our new scoring system was further strengthened by the quantification of inflammatory factors which revealed a significant upregulation of IL-1α and IL-10 in the colon of animals that developed NEC (68–76).
Despite multiple gaps in our understanding of NEC etiology and pathophysiology, the contribution of the microbiome to the development of NEC, initially suggested by Claud and Walker (77), has been widely documented in the last decade (78–82). Several studies have clearly established that intestinal bacterial diversity in premature infants is inherent to gestational age, breastfeeding, and the use of antibiotics (83, 84). A decrease in microbial diversity is often associated with the onset of NEC (77, 81, 85–87). Our data revealed that prolonged formula feeding decreases bacterial diversity, similar to the findings in premature human infants (88). Furthermore, we found that NEC, whether in the colostrum-fed or formula-fed group, was associated with low microbial diversity. Interestingly, we also showed that the development of NEC in our model is associated with increased Gammaproteobacteria and Enterobacteriaceae. This observation is in line with previous reports showing that NEC is consistently associated with the enrichment of Proteobacteria and Enterobacteriaceae in preterm infant cohorts (77, 85, 88, 89). Findings that Gram-negative bacteria dominate the gut microbiota of preterm infants are consistent with the hypothesis that increased Proteobacteria is directly linked to the development of NEC through the activation of TLR4 (11, 14, 90, 91). Indeed, it is widely accepted that the premature intestine is more prone to TLR4-driven inflammation, which is likely a primary cause of NEC (11, 14, 90, 91). Our data also revealed that NEC was associated with a significant increase in Clostridiaceae abundance (64, 87, 92). Despite the absence of a consensus regarding the association with NEC, a few studies have similarly shown an increase of Clostridiaceae in NEC patients (85, 86, 89). We hypothesize that formula-feeding in our model more strongly drives microbial colonization profiles associated with NEC, and it is this altered microbial colonization that contributes TLR-4 driven inflammation. While our model displayed similarities with microbial changes observed in NEC patients, it also showed some differences. In contrast with the significant decrease of Firmicutes described in premature babies diagnosed with NEC (9), our piglets that developed NEC had a significantly higher relative abundance of Firmicutes than the control animals (data not shown). It is worth noting that it is challenging to identify the microbiome changes that are exclusively associated with human NEC, since administering antibiotics to premature infants is a broadly used practice in neonatal intensive care units. Therefore, antibiotic therapy cannot be excluded as a causative factor of some of the changes observed in microbiome analyses in NEC patients (93). It is also possible that the differences observed between human and swine NEC are specific to each species.
Our model relies on the transitional protection conferred by colostrum prior to formula feeding. Although at a much lower incidence, injury in the colostrum-fed group is still observed. This possibly occurs because we do not use TPN for supplemental nutrition to allow for delayed introduction of enterally-administered colostrum. As a control group, this allows us to discern the baseline incidence of NEC in piglets receiving enteral colostrum only, similar to infants receiving enteral breast milk only. Given the batch-to-batch differences of antibody titers in colostrum, it is imperative to assess the quality of the colostrum prior to use. This variability in the concentration of antibodies in breast milk is also observed in humans (94). Furthermore, we cannot definitively ascertain the nutritional and caloric content of BC, as can be done with Neocate Junior infant formula.
In conclusion, we have developed a new enteral feed-only model of NEC in premature piglets with an initial period of BC administration to improve survival and have introduced a novel multifactorial D-NEC scoring system. Similar to humans, premature piglets in this model spontaneously develop a NEC-like disease upon initiation of enteral formula feeds. NEC in this model is characterized by the impairment of the epithelial barrier leading to bacterial translocation and a deleterious inflammatory response. We have also demonstrated that our model recapitulates the main features of microbial dysbiosis seen in NEC patients. Moving forward, this model can be used for pre-clinical testing of novel therapies for NEC in order to facilitate the transition of these novel therapies from bench to bedside.
Data availability statement
The datasets presented in this study can be found in online repositories. The names of the repository/repositories and accession number(s) can be found here: 16S rRNA sequencing data is available in Sequence Read Archive (SRA) under BioProject accession number PRJNA922570.
Ethics statement
The animal study was reviewed and approved by The Institutional Animal Care and Use Committee (IACUC). Abigail Wexner Research Institute at Nationwide Children's Hospital.
Author contributions
MR, SW, NS, YW, AA, BM, SG, MB and GB designed the study. MR, SW, NS, AD, YW, SV, ZD, NP, JW, BM, and GB performed the experiments. MR, SW, NS, AD, YW, SV, MC, BM, SG, MB, and GB analyzed the data. MR, SW, AD, BM, GB wrote the manuscript. All authors contributed to the article and approved the submitted version.
Funding
This work was supported by NIH R44GM122130-02 (GB, SG, MB), by the Research Institute at Nationwide Children's Hospital (GB), and by the Department of Surgery at Nationwide Children's Hospital (GB).
Acknowledgments
We are grateful for the excellent veterinary assistance provided by Laurie Goodchild, Raphael Malbrue, Carmen Arsuaga, and the Animal Resources Core staff at Nationwide Children's Hospital in the care of the animals. We thank Pamela Mar, Rita Shelby, Jeffrey Galley, Brandon Rodgers, Grace Janzow, Grace Hamilton, Shivani Deshpande, and Maria Basista for assistance with early model development.
Conflict of interest
GB, SG, and MB are scientific founders of Scioto Biosciences, Inc. (Indianapolis, IN). The remaining authors declare that the research was conducted in the absence of any commercial or financial relationships that could be construed as a potential conflict of interest.
Publisher's note
All claims expressed in this article are solely those of the authors and do not necessarily represent those of their affiliated organizations, or those of the publisher, the editors and the reviewers. Any product that may be evaluated in this article, or claim that may be made by its manufacturer, is not guaranteed or endorsed by the publisher.
Supplementary material
The Supplementary Material for this article can be found online at: https://www.frontiersin.org/articles/10.3389/fped.2023.1126552/full#supplementary-material.
References
1. Caplan MS. Neonatal necrotizing enterocolitis. Introduction. Semin Perinatol. (2008) 32:69. doi: 10.1053/j.semperi.2008.02.001
2. Gephart SM, McGrath JM, Effken JA, Halpern MD. Necrotizing enterocolitis risk: state of the science. Adv Neonatal Care. (2012) 12:77–87; quiz 88–9. doi: 10.1097/ANC.0b013e31824cee94
3. Meister AL, Doheny KK, Travagli RA. Necrotizing enterocolitis: it's not all in the gut. Exp Biol Med (Maywood). (2020) 245:85–95. doi: 10.1177/1535370219891971
4. Bell MJ, Ternberg JL, Feigin RD, Keating JP, Marshall R, Barton L, et al. Neonatal necrotizing enterocolitis. Therapeutic decisions based upon clinical staging. Ann Surg. (1978) 187:1–7. doi: 10.1097/00000658-197801000-00001
5. Hackam D, Caplan M. Necrotizing enterocolitis: pathophysiology from a historical context. Semin Pediatr Surg. (2018) 27:11–8. doi: 10.1053/j.sempedsurg.2017.11.003
6. Kovler ML, Sodhi CP, Hackam DJ. Precision-based modeling approaches for necrotizing enterocolitis. Dis Model Mech. (2020) 13(6):dmm044388. doi: 10.1242/dmm.044388
7. Thänert R, Keen EC, Dantas G, Warner BB, Tarr PI. Necrotizing enterocolitis and the microbiome: current status and future directions. J Infect Dis. (2021) 223:S257–63. doi: 10.1093/infdis/jiaa604
8. Touloukian RJ, Posch JN, Spencer R. The pathogenesis of ischemic gastroenterocolitis of the neonate: selective gut mucosal ischemia in asphyxiated neonatal piglets. J Pediatr Surg. (1972) 7:194–205. doi: 10.1016/0022-3468(72)90496-4
9. Pammi M, Cope J, Tarr PI, Warner BB, Morrow AL, Mai V, et al. Intestinal dysbiosis in preterm infants preceding necrotizing enterocolitis: a systematic review and meta-analysis. Microbiome. (2017) 5:31. doi: 10.1186/s40168-017-0248-8
10. Pisano C, Galley J, Elbahrawy M, Wang Y, Farrell A, Brigstock D, et al. Human breast milk-derived extracellular vesicles in the protection against experimental necrotizing enterocolitis. J Pediatr Surg. (2020) 55:54–8. doi: 10.1016/j.jpedsurg.2019.09.052
11. Good M, Sodhi CP, Egan CE, Afrazi A, Jia H, Yamaguchi Y, et al. Breast milk protects against the development of necrotizing enterocolitis through inhibition of toll-like receptor 4 in the intestinal epithelium via activation of the epidermal growth factor receptor. Mucosal Immunol. (2015) 8:1166–79. doi: 10.1038/mi.2015.30
12. Lu P, Sodhi CP, Jia H, Shaffiey S, Good M, Branca MF, et al. Animal models of gastrointestinal and liver diseases. Animal models of necrotizing enterocolitis: pathophysiology, translational relevance, and challenges. Am J Physiol Gastrointest Liver Physiol. (2014) 306:G917–28. doi: 10.1152/ajpgi.00422.2013
13. Sangild PT. Gut responses to enteral nutrition in preterm infants and animals. Exp Biol Med (Maywood). (2006) 231:1695–711. doi: 10.1177/153537020623101106
14. Leaphart CL, Cavallo J, Gribar SC, Cetin S, Li J, Branca MF, et al. A critical role for TLR4 in the pathogenesis of necrotizing enterocolitis by modulating intestinal injury and repair. J Immunol. (2007) 179:4808–20. doi: 10.4049/jimmunol.179.7.4808
15. Sangild PT, Siggers RH, Schmidt M, Elnif J, Bjornvad CR, Thymann T, et al. Diet- and colonization-dependent intestinal dysfunction predisposes to necrotizing enterocolitis in preterm pigs. Gastroenterology. (2006) 130:1776–92. doi: 10.1053/j.gastro.2006.02.026
16. Sangild PT, Thymann T, Schmidt M, Stoll B, Burrin DG, Buddington RK. Invited review: the preterm pig as a model in pediatric gastroenterology. J Anim Sci. (2013) 91:4713–29. doi: 10.2527/jas.2013-6359
17. Lopez CM, Sampah MES, Duess JW, Ishiyama A, Ahmad R, Sodhi CP, et al. Models of necrotizing enterocolitis. Semin Perinatol. (2022) 151695. doi: 10.1016/j.semperi.2022.151695
18. Bjornvad CR, Thymann T, Deutz NE, Burrin DG, Jensen SK, Jensen BB, et al. Enteral feeding induces diet-dependent mucosal dysfunction, bacterial proliferation, and necrotizing enterocolitis in preterm pigs on parenteral nutrition. Am J Physiol Gastrointest Liver Physiol. (2008) 295:G1092–1103. doi: 10.1152/ajpgi.00414.2007
19. Patterson JK, Lei XG, Miller DD. The pig as an experimental model for elucidating the mechanisms governing dietary influence on mineral absorption. Exp Biol Med (Maywood). (2008) 233:651–64. doi: 10.3181/0709-MR-262
20. Pang X, Hua X, Yang Q, Ding D, Che C, Cui L, et al. Inter-species transplantation of gut microbiota from human to pigs. ISME J. (2007) 1:156–62. doi: 10.1038/ismej.2007.23
21. Heinritz SN, Mosenthin R, Weiss E. Use of pigs as a potential model for research into dietary modulation of the human gut microbiota. Nutr Res Rev. (2013) 26:191–209. doi: 10.1017/S0954422413000152
22. Leser TD, Amenuvor JZ, Jensen TK, Lindecrona RH, Boye M, Møller K. Culture-independent analysis of gut bacteria: the pig gastrointestinal tract microbiota revisited. Appl Environ Microbiol. (2002) 68:673–90. doi: 10.1128/AEM.68.2.673-690.2002
23. Gonzalez LM, Moeser AJ, Blikslager AT. Porcine models of digestive disease: the future of large animal translational research. Transl Res. (2015) 166:12–27. doi: 10.1016/j.trsl.2015.01.004
24. Robinson JL, Smith VA, Stoll B, Agarwal U, Premkumar MH, Lau P, et al. Prematurity reduces citrulline-arginine-nitric oxide production and precedes the onset of necrotizing enterocolitis in piglets. Am J Physiol Gastrointest Liver Physiol. (2018) 315:G638–49. doi: 10.1152/ajpgi.00198.2018
25. Ghoneim N, Bauchart-Thevret C, Oosterloo B, Stoll B, Kulkarni M, de Pipaon MS, et al. Delayed initiation but not gradual advancement of enteral formula feeding reduces the incidence of necrotizing enterocolitis (NEC) in preterm pigs. PLoS One. (2014) 9:e106888. doi: 10.1371/journal.pone.0106888
26. Gay AN, Lazar DA, Stoll B, Naik-Mathuria B, Mushin OP, Rodriguez MA, et al. Near-infrared spectroscopy measurement of abdominal tissue oxygenation is a useful indicator of intestinal blood flow and necrotizing enterocolitis in premature piglets. J Pediatr Surg. (2011) 46:1034–40. doi: 10.1016/j.jpedsurg.2011.03.025
27. Caplan MS, Hedlund E, Adler L, Hsueh W. Role of asphyxia and feeding in a neonatal rat model of necrotizing enterocolitis. Pediatr Pathol. (1994) 14:1017–28. doi: 10.3109/15513819409037698
28. Benight NM, Stoll B, Olutoye OO, Holst JJ, Burrin DG. GLP-2 delays but does not prevent the onset of necrotizing enterocolitis in preterm pigs. J Pediatr Gastroenterol Nutr. (2013) 56:623–30. doi: 10.1097/MPG.0b013e318286891e
29. Jensen ML, Sangild PT, Lykke M, Schmidt M, Boye M, Jensen BB, et al. Similar efficacy of human banked milk and bovine colostrum to decrease incidence of necrotizing enterocolitis in preterm piglets. Am J Physiol Regul Integr Comp Physiol. (2013) 305:R4–R12. doi: 10.1152/ajpregu.00094.2013
30. Cilieborg MS, Thymann T, Siggers R, Boye M, Bering SB, Jensen BB, et al. The incidence of necrotizing enterocolitis is increased following probiotic administration to preterm pigs. J Nutr. (2011) 141:223–30. doi: 10.3945/jn.110.128561
31. Zamora IJ, Stoll B, Ethun CG, Sheikh F, Yu L, Burrin DG, et al. Low abdominal NIRS values and elevated plasma intestinal fatty acid-binding protein in a premature piglet model of necrotizing enterocolitis. PLoS One. (2015) 10:e0125437. doi: 10.1371/journal.pone.0125437
32. Ahmann J, Steinhoff-Wagner J, Büscher W. Determining immunoglobulin content of bovine colostrum and factors affecting the outcome: a review. Animals (Basel). (2021) 11(12):3587. doi: 10.3390/ani11123587
33. Stelwagen K, Carpenter E, Haigh B, Hodgkinson A, Wheeler TT. Immune components of bovine colostrum and milk. J Anim Sci. (2009) 87:3–9. doi: 10.2527/jas.2008-1377
34. Zobel G, Rodriguez-Sanchez R, Hea SY, Weatherall A, Sargent R. Validation of brix refractometers and a hydrometer for measuring the quality of caprine colostrum. J Dairy Sci. (2020) 103:9277–89. doi: 10.3168/jds.2020-18165
35. Bartens M-C, Drillich M, Rychli K, Iwersen M, Arnholdt T, Meyer L, et al. Assessment of different methods to estimate bovine colostrum quality on farm. N Z Vet J. (2016) 64:263–7. doi: 10.1080/00480169.2016.1184109
36. Westhoff TA, Womack SJ, Overton TR, Ryan CM, Mann S. Epidemiology of bovine colostrum production in New York Holstein herds: cow, management, and environmental factors. J Dairy Sci. In Press, Corrected Proof. doi: 10.3168/jds.2022-22447
37. Yan X, Sangild PT, Peng Y, Li Y, Bering SB, Pan X. Supplementary bovine colostrum feedings to formula-fed preterm pigs improve gut function and reduce necrotizing enterocolitis. J Pediatr Gastroenterol Nutr. (2021) 73:e39–46. doi: 10.1097/MPG.0000000000003147
38. Ciftci I, Ozdemir M, Aktan M, Aslan K. Bacterial translocation and intestinal injury in experimental necrotizing enterocolitis model. Bratisl Lek Listy. (2012) 113:206–10. doi: 10.4149/bll_2012_047
39. Vigors S, O’Doherty Jv, Kelly AK, O’Shea CJ, Sweeney T. The effect of divergence in feed efficiency on the intestinal Microbiota and the intestinal immune response in both unchallenged and lipopolysaccharide challenged ileal and colonic explants. PLoS One. (2016) 11:e0148145. doi: 10.1371/journal.pone.0148145
40. Liu H, Feye KM, Nguyen YT, Rakhshandeh A, Loving CL, Dekkers JCM, et al. Acute systemic inflammatory response to lipopolysaccharide stimulation in pigs divergently selected for residual feed intake. BMC Genom. (2019) 20:728. doi: 10.1186/s12864-019-6127-x
41. Wang S, Wang B, He H, Sun A, Guo C. A new set of reference housekeeping genes for the normalization RT-qPCR data from the intestine of piglets during weaning. PLoS One. (2018) 13:e0204583. doi: 10.1371/journal.pone.0204583
42. Bolyen E, Rideout JR, Dillon MR, Bokulich NA, Abnet CC, Al-Ghalith GA, et al. Reproducible, interactive, scalable and extensible microbiome data science using QIIME 2. Nat Biotechnol. (2019) 37:852–7. doi: 10.1038/s41587-019-0209-9
43. Callahan BJ, McMurdie PJ, Rosen MJ, Han AW, Johnson AJA, Holmes SP. DADA2: high-resolution sample inference from illumina amplicon data. Nat Methods. (2016) 13:581–3. doi: 10.1038/nmeth.3869
44. Quast C, Pruesse E, Yilmaz P, Gerken J, Schweer T, Yarza P, et al. The SILVA ribosomal RNA gene database project: improved data processing and web-based tools. Nucleic Acids Res. (2013) 41:D590–6. doi: 10.1093/nar/gks1219
45. Bokulich NA, Kaehler BD, Rideout JR, Dillon M, Bolyen E, Knight R, et al. Optimizing taxonomic classification of marker-gene amplicon sequences with QIIME 2’s q2-feature-classifier plugin. Microbiome. (2018) 6:90. doi: 10.1186/s40168-018-0470-z
46. Shannon CE. A mathematical theory of communication. Bell Syst Tech J. (1948) 27:379–423. doi: 10.1002/j.1538-7305.1948.tb01338.x
47. Lozupone CA, Hamady M, Kelley ST, Knight R. Quantitative and qualitative beta diversity measures lead to different insights into factors that structure microbial communities. Appl Environ Microbiol. (2007) 73:1576–85. doi: 10.1128/AEM.01996-06
48. Vázquez-Baeza Y, Pirrung M, Gonzalez A, Knight R. EMPeror: a tool for visualizing high-throughput microbial community data. Gigascience. (2013) 2:16. doi: 10.1186/2047-217X-2-16
49. Sangild PT, Vonderohe C, Melendez Hebib V, Burrin DG. Potential benefits of bovine colostrum in pediatric nutrition and health. Nutrients. (2021) 13(8):2551. doi: 10.3390/nu13082551
50. Sangild PT. Uptake of colostral immunoglobulins by the compromised newborn farm animal. Acta Vet Scand Suppl. (2003) 98:105–22. doi: 10.1186/1751-0147-44-s1-s105
51. Van de Perre P. Transfer of antibody via mother’s milk. Vaccine. (2003) 21:3374–6. doi: 10.1016/s0264-410x(03)00336-0
52. Simister NE. Placental transport of immunoglobulin G. Vaccine. (2003) 21:3365–9. doi: 10.1016/s0264-410x(03)00334-7
53. Chiang Kv, Sharma AJ, Nelson JM, Olson CK, Perrine CG. Receipt of breast milk by gestational age — united States, 2017. MMWR Morb Mortal Wkly Rep. (2019) 68:489–93. doi: 10.15585/mmwr.mm6822a1
54. Greer FR. Feeding the premature infant in the 20th century. J Nutr. (2001) 131:426S–30S. doi: 10.1093/jn/131.2.426S
55. Asadi S, Bloomfield FH, Harding JE. Nutrition in late preterm infants. Semin Perinatol. (2019) 43:151160. doi: 10.1053/j.semperi.2019.06.008
56. Stoll BJ, Hansen NI, Bell EF, Walsh MC, Carlo WA, Shankaran S, et al. Trends in care practices, morbidity, and mortality of extremely preterm neonates, 1993–2012. JAMA. (2015) 314:1039–51. doi: 10.1001/jama.2015.10244
57. Buddington RK, Davis SL, Buddington KK. The risk of necrotizing enterocolitis differs among preterm pigs fed formulas with either lactose or maltodextrin. J Pediatr Gastroenterol Nutr. (2018) 66:e61–6. doi: 10.1097/MPG.0000000000001707
58. Thymann T, Møller HK, Stoll B, Støy ACF, Buddington RK, Bering SB, et al. Carbohydrate maldigestion induces necrotizing enterocolitis in preterm pigs. Am J Physiol Gastrointest Liver Physiol. (2009) 297:G1115–25. doi: 10.1152/ajpgi.00261.2009
59. Sangild PT, Petersen YM, Schmidt M, Elnif J, Petersen TK, Buddington RK, et al. Preterm birth affects the intestinal response to parenteral and enteral nutrition in newborn pigs. J Nutr. (2002) 132:2673–81. doi: 10.1093/jn/132.9.2673
60. Cilieborg MS, Boye M, Thymann T, Jensen BB, Sangild PT. Diet-dependent effects of minimal enteral nutrition on intestinal function and necrotizing enterocolitis in preterm pigs. J Parenter Enteral Nutr. (2011) 35:32–42. doi: 10.1177/0148607110377206
61. Kappel SS, Sangild PT, Hilsted L, Hartmann B, Thymann T, Aunsholt L. Gastric residual to predict necrotizing enterocolitis in preterm piglets as models for infants. J Parenter Enteral Nutr. (2021) 45:87–93. doi: 10.1002/jpen.1814
62. Jiang Y-N, Muk T, Stensballe A, Nguyen DN, Sangild PT, Jiang P-P. Early protein markers of necrotizing enterocolitis in plasma of preterm pigs exposed to antibiotics. Front Immunol. (2020) 11:565862. doi: 10.3389/fimmu.2020.565862
63. Sun J, Pan X, Christiansen LI, Yuan X-L, Skovgaard K, Chatterton DEW, et al. Necrotizing enterocolitis is associated with acute brain responses in preterm pigs. J Neuroinflammation. (2018) 15:180. doi: 10.1186/s12974-018-1201-x
64. Azcarate-Peril MA, Foster DM, Cadenas MB, Stone MR, Jacobi SK, Stauffer SH, et al. Acute necrotizing enterocolitis of preterm piglets is characterized by dysbiosis of ileal mucosa-associated bacteria. Gut Microbes. (2011) 2(4):234–43. doi: 10.4161/gmic.2.4.16332
65. Good M, Sodhi CP, Ozolek JA, Buck RH, Goehring KC, Thomas DL, et al. Lactobacillus rhamnosus HN001 decreases the severity of necrotizing enterocolitis in neonatal mice and preterm piglets: evidence in mice for a role of TLR9. Am J Physiol Gastrointest Liver Physiol. (2014) 306:G1021–32. doi: 10.1152/ajpgi.00452.2013
66. Roy SK, Meng Q, Sadowitz BD, Kollisch-Singule M, Yepuri N, Satalin J, et al. Enteral administration of bacteria fermented formula in newborn piglets: a high fidelity model for necrotizing enterocolitis (NEC). PLoS One. (2018) 13:e0201172. doi: 10.1371/journal.pone.0201172
67. Neu J, Walker WA. Necrotizing enterocolitis. N Engl J Med. (2011) 364:255–64. doi: 10.1056/NEJMra1005408
68. di Lorenzo M, Bass J, Krantis A. An intraluminal model of necrotizing enterocolitis in the developing neonatal piglet. J Pediatr Surg. (1995) 30:1138–42. doi: 10.1016/0022-3468(95)90006-3
69. Cannon JG, Tompkins RG, Gelfand JA, Michie HR, Stanford GG, van der Meer JW, et al. Circulating interleukin-1 and tumor necrosis factor in septic shock and experimental endotoxin fever. J Infect Dis. (1990) 161:79–84. doi: 10.1093/infdis/161.1.79
70. Siggers J, Sangild PT, Jensen TK, Siggers RH, Skovgaard K, Støy ACF, et al. Transition from parenteral to enteral nutrition induces immediate diet-dependent gut histological and immunological responses in preterm neonates. Am J Physiol Gastrointest Liver Physiol. (2011) 301:G435–45. doi: 10.1152/ajpgi.00400.2010
71. Cho SX, Berger PJ, Nold-Petry CA, Nold MF. The immunological landscape in necrotising enterocolitis. Expert Rev Mol Med. (2016) 18:e12. doi: 10.1017/erm.2016.13
72. Ng PC, Li K, Wong RPO, Chui K, Wong E, Li G, et al. Proinflammatory and anti-inflammatory cytokine responses in preterm infants with systemic infections. Arch Dis Child Fetal Neonatal Ed. (2003) 88:F209–13. doi: 10.1136/fn.88.3.f209
73. Hui L, Dai Y, Guo Z, Zhang J, Zheng F, Bian X, et al. Immunoregulation effects of different γδT cells and toll-like receptor signaling pathways in neonatal necrotizing enterocolitis. Medicine (Baltimore). (2017) 96:e6077. doi: 10.1097/MD.0000000000006077
74. Emami CN, Chokshi N, Wang J, Hunter C, Guner Y, Goth K, et al. Role of interleukin-10 in the pathogenesis of necrotizing enterocolitis. Am J Surg. (2012) 203:428–35. doi: 10.1016/j.amjsurg.2011.08.016
75. Hunter CJ, Singamsetty VK, Chokshi NK, Boyle P, Camerini V, Grishin Av, et al. Enterobacter sakazakii enhances epithelial cell injury by inducing apoptosis in a rat model of necrotizing enterocolitis. J Infect Dis. (2008) 198:586–93. doi: 10.1086/590186
76. Edelson MB, Bagwell CE, Rozycki HJ. Circulating pro- and counterinflammatory cytokine levels and severity in necrotizing enterocolitis. Pediatrics. (1999) 103:766–71. doi: 10.1542/peds.103.4.766
77. Claud EC, Walker WA. Hypothesis: inappropriate colonization of the premature intestine can cause neonatal necrotizing enterocolitis. FASEB J. (2001) 15:1398–403. doi: 10.1096/fj.00-0833hyp
78. Brower-Sinning R, Zhong D, Good M, Firek B, Baker R, Sodhi CP, et al. Mucosa-associated bacterial diversity in necrotizing enterocolitis. PLoS One. (2014) 9:e105046. doi: 10.1371/journal.pone.0105046
79. Warner BB, Deych E, Zhou Y, Hall-Moore C, Weinstock GM, Sodergren E, et al. Gut bacteria dysbiosis and necrotising enterocolitis in very low birthweight infants: a prospective case-control study. Lancet. (2016) 387:1928–36. doi: 10.1016/S0140-6736(16)00081-7
80. Morrow AL, Lagomarcino AJ, Schibler KR, Taft DH, Yu Z, Wang B, et al. Early microbial and metabolomic signatures predict later onset of necrotizing enterocolitis in preterm infants. Microbiome. (2013) 1:13. doi: 10.1186/2049-2618-1-13
81. Dobbler PT, Procianoy RS, Mai V, Silveira RC, Corso AL, Rojas BS, et al. Low microbial diversity and abnormal microbial succession is associated with necrotizing enterocolitis in preterm infants. Front Microbiol. (2017) 8:2243. doi: 10.3389/fmicb.2017.02243
82. Olm MR, Bhattacharya N, Crits-Christoph A, Firek BA, Baker R, Song YS, et al. Necrotizing enterocolitis is preceded by increased gut bacterial replication, Klebsiella, and fimbriae-encoding bacteria. Sci Adv. (2019) 5:eaax5727. doi: 10.1126/sciadv.aax5727
83. la Rosa PS, Warner BB, Zhou Y, Weinstock GM, Sodergren E, Hall-Moore CM, et al. Patterned progression of bacterial populations in the premature infant gut. Proc Natl Acad Sci U S A. (2014) 111:12522–7. doi: 10.1073/pnas.1409497111
84. Gregory KE, Samuel BS, Houghteling P, Shan G, Ausubel FM, Sadreyev RI, et al. Influence of maternal breast milk ingestion on acquisition of the intestinal microbiome in preterm infants. Microbiome. (2016) 4:68. doi: 10.1186/s40168-016-0214-x
85. Fu X, Li S, Jiang Y, Hu X, Wu H. Necrotizing enterocolitis and intestinal microbiota: the timing of disease and combined effects of multiple species. Front Pediatr. (2021) 9:657349. doi: 10.3389/fped.2021.657349
86. Rozé J-C, Ancel P-Y, Lepage P, Martin-Marchand L, al Nabhani Z, Delannoy J, et al. Nutritional strategies and gut microbiota composition as risk factors for necrotizing enterocolitis in very-preterm infants. Am J Clin Nutr. (2017) 106:821–30. doi: 10.3945/ajcn.117.152967
87. Støy ACF, Mølbak L, Delègue CL, Thymann T, Sangild PT, Heegaard PMH, et al. Necrotizing enterocolitis in preterm pigs is associated with increased density of intestinal mucosa-associated bacteria including clostridium perfringens. Neonatology. (2015) 108:188–95. doi: 10.1159/000431280
88. Maradiaga N, Aldridge B, Zeineldin M, Lowe J. Gastrointestinal microbiota and mucosal immune gene expression in neonatal pigs reared in a cross-fostering model. Microb Pathog. (2018) 121:27–39. doi: 10.1016/j.micpath.2018.05.007
89. Zhou Y, Shan G, Sodergren E, Weinstock G, Walker WA, Gregory KE. Longitudinal analysis of the premature infant intestinal microbiome prior to necrotizing enterocolitis: a case-control study. PLoS One. (2015) 10:e0118632. doi: 10.1371/journal.pone.0118632
90. Mihi B, Good M. Impact of toll-like receptor 4 signaling in necrotizing enterocolitis: the state of the science. Clin Perinatol. (2019) 46:145–57. doi: 10.1016/j.clp.2018.09.007
91. Arciero J, Bard Ermentrout G, Siggers R, Afrazi A, Hackam D, Vodovotz Y, et al. Modeling the interactions of bacteria and toll-like receptor-mediated inflammation in necrotizing enterocolitis. J Theor Biol. (2013) 321:83–99. doi: 10.1016/j.jtbi.2012.12.002
92. Cilieborg MS, Boye M, Mølbak L, Thymann T, Sangild PT. Preterm birth and necrotizing enterocolitis alter gut colonization in pigs. Pediatr Res. (2011) 69:10–6. doi: 10.1203/PDR.0b013e3181ff2a89
93. Greenwood C, Morrow AL, Lagomarcino AJ, Altaye M, Taft DH, Yu Z, et al. Early empiric antibiotic use in preterm infants is associated with lower bacterial diversity and higher relative abundance of Enterobacter. J Pediatr. (2014) 165:23–9. doi: 10.1016/j.jpeds.2014.01.010
Keywords: necrotizing enterocolitis (NEC), piglet, enteral feed, microbiome, colostrum
Citation: Ragan MV, Wala SJ, Sajankila N, Duff AF, Wang Y, Volpe SG, Al-Hadidi A, Dumbauld Z, Purayil N, Wickham J, Conces MR, Mihi B, Goodman SD, Bailey MT and Besner GE (2023) Development of a novel definitive scoring system for an enteral feed-only model of necrotizing enterocolitis in piglets. Front. Pediatr. 11:1126552. doi: 10.3389/fped.2023.1126552
Received: 18 December 2022; Accepted: 28 March 2023;
Published: 17 April 2023.
Edited by:
Flavia Indrio, University of Foggia, ItalyReviewed by:
Colin Martin, University of Alabama at Birmingham, United StatesSteven James McElroy, UC Davis Health, United States
© 2023 Ragan, Wala, Sajankila, Duff, Wang, Volpe, Al-Hadidi, Dumbauld, Purayil, Wickham, Conces, Mihi, Goodman, Bailey and Besner. This is an open-access article distributed under the terms of the Creative Commons Attribution License (CC BY). The use, distribution or reproduction in other forums is permitted, provided the original author(s) and the copyright owner(s) are credited and that the original publication in this journal is cited, in accordance with accepted academic practice. No use, distribution or reproduction is permitted which does not comply with these terms.
*Correspondence: Gail E. Besner Z2FpbC5iZXNuZXJAbmF0aW9ud2lkZWNoaWxkcmVucy5vcmc=
†These authors have contributed equally to this work and share first authorship
Specialty Section: This article was submitted to Pediatric Gastroenterology, Hepatology and Nutrition, a section of the journal Frontiers in Pediatrics
Abbreviations BC, bovine colostrum; D-NEC, definitive necrotizing enterocolitis; CSS, clinical sickness score; NEC, necrotizing enterocolitis.