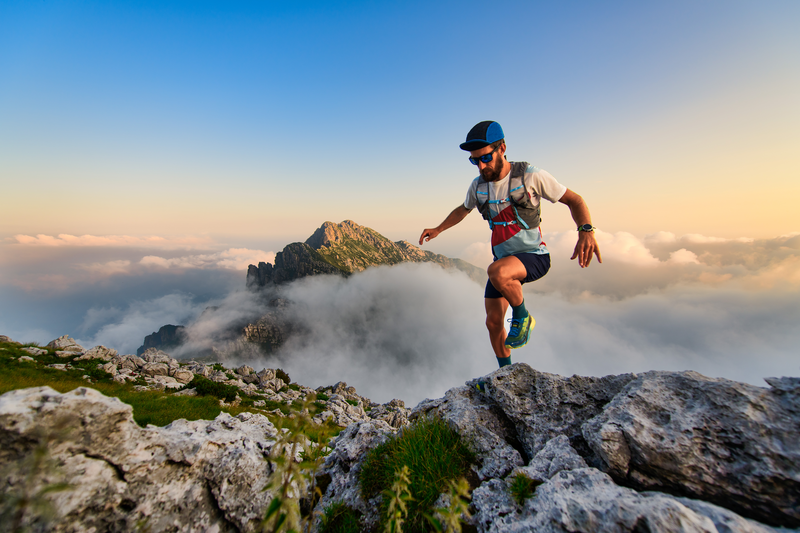
94% of researchers rate our articles as excellent or good
Learn more about the work of our research integrity team to safeguard the quality of each article we publish.
Find out more
REVIEW article
Front. Pediatr. , 02 March 2023
Sec. Pediatric Infectious Diseases
Volume 11 - 2023 | https://doi.org/10.3389/fped.2023.1115009
The purpose of this study is to review the molecular characteristics, the diagnosis, and treatment of the widespread infection of macrolide-resistant Mycoplasma pneumoniae (M. pneumoniae; MRMP) in children, thus providing a better knowledge of this infection and presenting the associated problems. Single point mutations in the V region of the 23S rRNA gene of M. pneumoniae genome are associated with macrolide resistance. P1–1, MLVA4-5-7-2, and ST3 are usually the predominated genetic types in the M. pneumoniae epidemics. The short-term two times serological IgM (or together with IgG) test in the acute stage can be used for confirmation. Combined serological testing and PCR might be a more prudent method to reduce macrolide consumption and antibiotic selective pressure in a clinical setting. Molecular methods for the detection of single-nucleotide mutations in the V region of the 23S rRNA gene can be used for the diagnosis of MRMP. The routine use of macrolide for the treatment of macrolide-sensitive Mycoplasma pneumoniae (MSMP) infections can get good effect, but the effects are limited for severe MRMP infections. Additional corticosteroids may be required for the treatment of severe MRMP infections in children in China during the era of MRMP.
Mycoplasma pneumoniae (M. pneumoniae) is a main pathogenic cause of community-acquired pneumonia (CAP) and bronchitis, which is responsible for 10%–40% of CAP (1–3). M. pneumoniae infection is a kind of self-limited disease, but the infection in some patients, especially in children, can develop serious pneumonia, bronchitis, and asthma, and even endanger their lives. Since there are no intact bacteria in pathologic lesions in severe M. pneumoniae pneumonia (MPP) patients and experimental animals in species-specific mycoplasma strains, the pathogen itself may not be a direct cause of cell injury in M. pneumoniae infections, and the theory of immunopathogenesis is proposed. The overuse and unnecessary usage of macrolides for the treatment of respiratory infections in recent years have contributed to the increase of macrolide-resistant M. pneumoniae (MRMP). Since MRMP was first isolated and described in pediatric patients in Japan (4), it spread rapidly all over the world, especially in Asian countries including China, Japan, and South Korea, where the resistance rates could reach 80%–90% (5–7). Studies found that single-nucleotide mutations in the V region of the 23S rRNA gene of M. pneumoniae genome are associated with macrolide resistance (8), and A2063G is the most common mutation, followed by A2064G. Clinical isolates of M. pneumoniae can be typed by p1 adhesion gene typing (9), multiple-locus variable-number tandem-repeat analysis (MLVA) (10, 11), and multilocus sequence typing (MLST) (12, 13). Common methods for the detection of M. pneumoniae include culture, serum special anti-M. pneumoniae antibody tests, and molecular methods. PCR or sequencing methods could be performed to identify the macrolide resistance-associated mutations within the V region of 23S rRNA gene of the M. pneumoniae genome. Macrolide is regarded as the first-line antibiotic treatment for M. pneumoniae infections in children for its low minimum inhibitory concentrations (MICs) and toxicity. Treating M. pneumoniae infections is more difficult in China because of the high macrolide-resistant rates. The routine use of azithromycin for the treatment of macrolide-sensitive M. pneumoniae (MSMP) infections can achieve a good effect, but additional corticosteroids may be required for the treatment of MRMP infections. Corticosteroid use has been reported in children of South Korea infected with MRMP or MSMP, which showed reduced disease morbidity and disease progression in MPP patients without side effects (14). Although some achievements have been made, there are some unsolved issues about MRMP. The pathogenesis of MPP and its complications remain unknown; the molecular characteristics of M. pneumoniae including the genotypes and macrolide resistance-associated single-nucleotide mutations are unclear in many parts of China and the world, and the correlation between macrolide resistance and genotypes remain controversial; the early and definite diagnosis of M. pneumoniae infection and the macrolide resistance is difficult, along with the proper additional corticosteroids treatments for MRMP infections.
M. pneumoniae is a kind of small atypical prokaryotic pathogen, with body size lager than a virus but smaller than a bacterium, and it can go through a bacterial filter and cannot be identified under an optical microscope. However, M. pneumoniae is considered a self-replicating bacterium for its ability to live outside a host cell and in the medium, and it can be inhibited or killed by antibiotics in patients with lower respiratory infections (15, 16). The growth of M. pneumoniae is very slow; thus, up to 6 weeks are required for the culture of M. pneumoniae (17). M. pneumoniae has a small genome of approximately 800 kb in size and up to 700 protein-coding genes (18–20), and the genome appears to be highly conservative between the strains (21). M. pneumoniae lacks a cell wall, and the special structure makes M. pneumoniae resistant to β-lactam antibiotic instinctively and facilitates the membrane of M. pneumoniae contact with that of the host cell directly, thus transferring and exchanging membrane components (22). M. pneumoniae is a unique human pathogen and can be transmitted through droplets between humans, and it can be transmitted among family members who live together in a house or among students with close and frequent touches in a school (23, 24). M. pneumoniae infection occurs both endemically and epidemically worldwide and it can induce upper and lower respiratory infections (21). M. pneumoniae infection can occur at any time during a year but may be more common in the summer and early autumn; an epidemic cycles at a time interval of 3–7 years (25), and each epidemic may last 1–2 years. Meteorological factors play an important role in the incidence of M. pneumoniae, and the infection rate may be positively correlated with temperature (26). The symptoms of M. pneumoniae infections include sore throat, fatigue, fever, headache, and cough that can last for weeks to months. Infected children younger than 5 years old are unlikely to develop fever but may manifest as wheezing, vomit, diarrhea, stuffy or runny nose, and sneezing (25). Extrapulmonary manifestations may be present other than the respiratory infections, affecting the skin mucous membrane, liver, kidney, and central nervous systems, such as the “Mycoplasma-induced rash and mucositis” (27).
The self-limited nature of M. pneumoniae infection often results in mild symptoms or being asymptomatic, but it may sometimes lead to dysfunction of the localized organs. The pathogenic mechanisms of M. pneumoniae include (1) activating the innate immune response and producing local cytotoxic effects through a specialized attachment to host cells and (2) leading to inflammation and airway dysfunction through producing a unique virulence known as Community-Acquired Respiratory Distress Syndrome (CARDS) toxin (25). The pathophysiology of acute lung injury and extrapulmonary manifestations, such as skin rashes, arthritis, encephalopathy, and other organ cell injuries on M. pneumoniae infection or coronavirus disease 2019 (COVID-19) are not fully understood. It is proposed that M. pneumoniae may act like a virus in the pathophysiology of the disease (28). Thus, it is possible that inflammation-inducing substances in M. pneumoniae infection are produced when pathogens are replicated within host cells like viruses. Host immune system may control these etiologic substances that originate from pathogens, including toxins such as CARDS toxin, and pathogen-associated molecular patterns (PAMPs), which lead to the release of pro-inflammatory cytokines. The substances originated from injured infected-host cells called damage-associated molecular patterns (DAMPs) can result in the elevation of inflammatory cytokines levels. The protein-homeostasis-system hypothesis proposes that the immune systems in the host control the etiological substances in M. pneumoniae-infected cells like viruses according to the size and biochemical properties (29). When these substances spread systemically and locally and bind to target organ cells, pneumonia and extrapulmonary manifestations begin due to the activation of corresponding immune cells and immune proteins. The substances produced from injured target cells such as lung cells induce further inflammation if released into the systemic circulation or near local lesions, and they could be associated with extrapulmonary manifestations (30).
As a kind of bacteriostatic agent, macrolide antibiotics act by inhibiting protein synthesis of bacteria through binding to the 50S ribosomal element (31). Macrolide is regarded as the preferred antibiotic choice for the treatment of M. pneumoniae infections than tetracyclines or quinolones especially in children because of the toxicities of the latter two. In vivo studies found that macrolide resistance-associated mutations including A2063G and A2064G could be found in 100% patients after 7–24 days of the initiation of macrolide treatment (32), and in vitro studies showed that the resistance mutations could be induced by subinhibitory concentrations of macrolide (33). Therefore, the overuse and unnecessary usage of macrolide in the past years are the main reasons for the prevalence of MRMP.
The resistance mechanisms of M. pneumoniae are mainly associated with the alteration of action targets of macrolide antibiotics. It has been identified and confirmed that the single-nucleotide mutations in domain V of the 23S rRNA gene are responsible for the macrolide resistance of M. pneumoniae (8, 34, 35). The mutations at the V region of the 23S rRNA gene decrease the affinity of macrolide to the 23S rRNA gene, and the ability of macrolides to suppress the protein synthesis of M. pneumoniae weakens, thus resulting in reduced strength of macrolides to inhibit the growth of M. pneumoniae. The mutations at sites 2063, 2064, 2067, and 2617 of 23S rRNA gene are the frequent mutations that confer macrolide resistance (9, 10, 13, 36–38), and mutations at sites 2063 and 2064 are associated with a high level of resistance, whereas the 2067 and 2617 site mutations result in a low level of resistance (35, 39). MRMP has very high MICs for 14- and 15-membered ring macrolides and moderately high MICs for 16-membered ring macrolides, and strains with the A2063G mutation have lower MICs for rokitamycin and josamycin (40).
The rates of MRMP vary across different regions of the world. The prevalence of MRMP is shown in Table 1 (11–13, 37, 41–47), and we selected and summarized the representative studies from regions of the world including America, Japan, South Korea, China, and Europe. From Table 1, it can be concluded that the prevalence of MRMP from high to low among different regions are northeastern Asia, America, and Europe. The rate of MRMP in the United States is about 10%, and it is often below 10% in Europe, whereas the rate in Asia is far higher. A2063G of the V region of the 23S rRNA gene is nearly the most frequently identified macrolide resistance-associated mutation in all regions, followed by A2064G. The data about MRMP are concentrated in America, Japan, South Korea, and European countries, and in Beijing and Shanghai of China. The lack of data about macrolide resistance in many parts of the world and China may be due to technical difficulties and cost reasons. There is no significant difference in clinical manifestation and disease severity between patients infected with MRMP and MSMP, but patients infected with MRMP may display prolonged hospitalization, febrile and coughing days, and antibiotic treatment course; more MRMP-infected patients experienced a change of antibiotic prescription under the traditional antibiotic treatments (48–50). Children with MRMP infections could appear with higher leukocyte counts and C-reactive protein (CRP) compared with patients infected with MSMP (51), but other studies suggested that MRMP is unlikely to be associated with laboratory and radiographic severity (48, 52); the contradictions might have resulted from the different subjects selected or the different time points in which the examinations were performed.
The most frequently performed methods for the genetic typing of M. pneumoniae include p1 adhesion gene restriction fragment length polymorphism (RFLP) analysis, MLVA, and MLST. The gene p1 is a kind of adhesion gene, which is the major factor that determines the virulence of M. pneumoniae (53). The P1 protein can cause allergy by inducing the production of P1-specific IgE (54). RepMP2/3 and RepMP4 are two repeated elements of the p1 gene, and M. pneumoniae strains can be classified into two major types (P1 subtype 1 and subtype 2, P1–1 and P1–2) based on the RFLP of the two regions (55–57). The regions can be combined with similar regions outside the p1 gene, thus generating the V1, V2a, V2b, V2c, and V2d variants (58). MLVA includes the analysis of five tandem repeated regions, which are named Mpn1, Mpn13, Mpn14, Mpn15, and Mpn16. Due to the instability of Mpn1, it might be more reliable to use the four-locus analysis (excluding Mpn1) to identify M. pneumonia (59). MLVA may be only useful for the identification or comparison of strains that are collected from relatively limited area and over a short period (60). MLST is performed through the sequencing of the eight housekeeping genes (ppa, pgm, gyrB, gmk, glyA, atpA, arcC, and adk) of M. pneumoniae, and M. pneumoniae can be typed based on the polymorphisms of the house keeping genes (61). MLST has higher discriminatory power than the frequently used four-locus MLVA typing and p1 gene RFLP methods (23).
The typing methods, the most prevalent reported types, and the correlations between different genetic types and macrolide resistance in studies are shown in Table 1. According to Table 1, P1–1 type predominated in most districts and periods, except the P1–2 predominance during 2012–2018 in America and during 2011–2017 in Japan, and the equal predominance of P1–1 and P1–2 during 2016–2020 in Switzerland. The two studies in America revealed a type shift from P1–1 to P1–2, which might be due to changes of human immunity, such as during the prevalence of P1–1, the human immunity against it strengthened (60). From Table 1, it can be summarized that MLVA4-5-7-2 and MLVA3-5-6-2 are the most prevalent reported MLVA types. In the two American studies, the MLVA types changed from MLVA4-5-7-2 during 2006–2013 to MLVA3-5-6-2 during 2012–2018, which revealed a MLVA type shift. ST3 predominated in most studies except the ST14 predominance in Switzerland from 2016 to 2020. The prevalence of MRMP and the genotypes between children and adults may be different, and the study by Yan et al. demonstrated that more diverse genotypes and a higher prevalence of macrolide resistance-associated mutations were found in the pediatric specimens (62). Further studies are needed to explore the molecular differences of M. pneumoniae infections between adult and pediatric population.
Controversial opinions existed regarding the correlations between different genetic types and macrolide resistance, and final conclusion has not been achieved yet. According to Table 1, the correlation between different genetic types and macrolide resistance can be established in Asian countries such as China, Japan, and South Korea, whereas few correlations existed in America and European countries, which might be due to the higher prevalence of MRMP in China and Japan whereas the lower prevalence of MRMP in America and European countries. In China, Japan, and South Korea, the prevalence of P1–1, ST3, and MLVA4-5-7-2 types are always correlated with MRMP (63). Periodic genotype shifts of the p1 gene in Japan from 2006 to 2019 found a decreased rate of MRMP and P1–1 type simultaneously (60), which can be speculated that the reduction of MRMP might be caused by the prevalence of P1–2-type M. pneumoniae. Controversial opinions suggest that the prevalence of MRMP is correlated with the use of macrolides but not genotypes for the different macrolide resistance rates of P1–1-type M. pneumoniae across different regions (42).
The methods for the diagnosis of M. pneumoniae include culture, PCR assays, and serologic tests. The advantages and disadvantages of each diagnostic method are shown in Table 2. The PPLO (pleuropneumonia-like organisms) broth supplemented with nutrients is often used for the culture of M. pneumoniae. The growth of M. pneumoniae is slow, and the diagnosis is time consuming, so it is not suitable for the early diagnosis and treatment of M. pneumoniae infection. On the other hand, the culture of M. pneumoniae requires high conditions of medium, and thus the positive rate is low. PCR assays include the detection of DNA and RNA, and the most frequently used method for the detection of DNA is the real-time PCR targeting the adhesion p1 gene of M. pneumoniae (64). The median duration of persistence of M. pneumoniae DNA in the body is 7 weeks after the onset of M. pneumoniae infection disease, and the period cannot be shortened even if adequate antibiotic treatment is given (65). Therefore, the M. pneumoniae DNA can be positive even after the symptoms of M. pneumoniae infection disappear, whereas M. pneumoniae RNA can be cleared quickly after the death of M. pneumoniae, and the detection of RNA can be negative after the recovery of the infection symptoms. The problem of the false-positive possibility of M. pneumoniae infection due to the persistence of M. pneumoniae DNA can be avoided by using RNA detection. M. pneumoniae RNA detection is capable of distinguishing recent and past infections; thus, it can be used to evaluate the therapeutic effect and the prognosis of the disease (66). There are many asymptomatic infected patients during M. pneumoniae epidemics and some co-infected patients. Thus, carriers and co-infected patients can be PCR positive (false positive), and it is necessary to identify the asymptomatic infection of M. pneumoniae and distinguish the real cause of symptoms. A serological test is the most widely used method for the diagnosis of M. pneumoniae infection currently. The main targets for the serological test are the special anti-M. pneumoniae IgG and IgM. The production of IgM requires 1–2 weeks, and it can exist positively a long time of over 1 year. A sensitivity of approximately 70%–80% and a specificity of 90% could be achieved by the perfect IgM test (67). The sensitivity of M. pneumoniae IgM test is higher than that of IgA for the diagnosis of M. pneumoniae-related pneumonia in school-age children and adolescents, and it is interesting to find that the rates of IgM and IgA are positively associated with the febrile days before hospitalization (68). In a clinical setting, because the PCR results much depend on the specimen collection process, PCR-positive patients at presentation are far less than single IgM-positive patients, and PCR-negative patients, especially lately presented, are common over 20%–50% of study subjects (69). There are no confirmative diagnostic laboratory tools for M. pneumoniae infection in the early stage, and single IgM-positive and/or single PCR tests are not confirmative for diagnosis of M. pneumoniae infection. Paired sera tests could provide a more accurate diagnosis of M. pneumoniae infection, and the fourfold increase of IgG from the acute to the recovery stage is regarded as the gold standard (70). The positive rates of paired sera IgG tests could be over 80% (71). The limitation of paired sera IgG test is that it can be only used for retrospective diagnosis and could not be applied for the early diagnosis. The long-time existence of IgM in the body can result in false positivity, and IgM serology may lead to false positive due to limited assay performance and age/host-dependent characteristics or false-negative results early in disease course and after reinfection (72). Chang et al. demonstrated that IgM showed poor sensitivity and positive predictive value, so the interpretation of IgM should be done with caution (73). Serological test only reflects the host immune response to M. pneumoniae (69). Serological changes, including IgM, IgG, and IgA, begin to appear after clinically manifesting such as fever and pneumonia. Thus, short-term two times serological IgM (or together with IgG) test in the acute stage is needed for confirmation of M. pneumoniae infection, and its clinical application is easy during hospitalization (74). Considering the advantages and disadvantages of serological and PCR tests, combined serological testing and PCR might be a more prudent method to reduce macrolide consumption and antibiotic selective pressure in a clinical setting.
The macrolide resistance of M. pneumoniae can be determined by the drug susceptibility experiment and molecular methods currently. Drug susceptibility experiment based on the broth microdilution method can not only determine the macrolide resistance of M. pneumoniae but also test the exact minimum inhibitory concentration. However, drug susceptibility experiment requires the culture of M. pneumoniae, so it is not suitable for the clinical practice due to the long process to the final diagnosis. Molecular methods for the detection of single-nucleotide mutations in the V region of the 23S rRNA gene of M. pneumoniae can be used for the rapid diagnosis of MRMP. The most widely used molecular method for the detection of MRMP can be performed by the amplification of the V region of the 23S rRNA gene; the products are then purified and sequenced, and finally the sequence results are blasted with the corresponding sequence of the reference standard strain M129 signed in NCBI to identify the nucleotide mutations associated with macrolide resistance. Coexistence of MRMP and MSMP can be found in a single case (75), and the determination of the ratio of MRMP/MSMP may be valuable for the diagnosis. Pyrosequencing is the only method for the quantification of MRMP and MSMP within a clinical specimen (76). Melting curve analyses based on PCR assay could reliably distinguish MRMP isolates when compared to MRMP and MSMP controls included in the run due to the slightly higher melting temperature of the amplicon caused by the substitution of A to G at positions 2063 or 2064(77). The melting curve analysis can be an efficient method to identify macrolide resistance, and the analysis can be completed within 1 h (76). The melting curve analysis can be practical for the fast identification of the A2063G and A2064G transitions, which usually cover over 90% of macrolide resistance mutations, and these two kinds of mutations usually confer high-level macrolide resistance, representing valuable clinical significance.
M. pneumoniae is instinctively resistant to β-lactam antibiotics, and macrolides, tetracyclines, and fluoroquinolones are effective selections for the treatments. Considering the risks of the antibiotics for the human body, macrolides can be used for both children and adults, tetracyclines can be used for elder children and adults, and fluoroquinolones for the adults only. The emergence of MRMP infections has made the antibiotic treatment more complicated. Macrolide possesses anti-inflammatory and immunoregulatory functions, enabling it effective in the treatment of some mild MRMP-infected cases. For severe cases infected with MRMP that showed poor effects during the treatments with macrolides, alternative antibiotic treatments have been explored. Japan Society of Pediatric Pulmonology/Japanese Society for Pediatric Infectious Diseases published guidelines for recommending tosufloxacin as a second-line drug when patients remain febrile for 48–72 h following the administration of macrolides (78). Doxycycline has been used as an alternative and is not likely to cause visible teeth staining or enamel hypoplasia in young children for short periods of treatments (79). An attempt of treatment of MPP cases infected by MRMP with levofloxacin has been made, which proved to be safe and effective, the clinical symptoms and radiological manifestations improved significantly, and no side effects were observed (80). There may be a long way for the exploration of safe and effective alternative antibiotic treatments, and surveillance should be given to the possible emergence of clinical tetracyclines or fluoroquinolones resistant strains, due to the fact that resistant strains have been induced in vitro for both antibiotic with the associated mutations (81–83).
Since MPP, including refractory MPP or MRMP pneumonia, is a self-limited disease, the host hyperimmune reaction against insults from M. pneumoniae infection is responsible for lung cell injury. The immune reaction of the host before the peak of inflammation (pro-inflammatory cytokines may be involved in this stage) may be involved in tissue cell injury, and immune reaction after the peak of inflammatory may be involved in tissue cell repair (anti-inflammatory cytokines may be involved in the convalescent stage); the intensity of systemic inflammation during this process is reflected in laboratory parameters, such as WBC, and differential, CRP, LDH, and immune proteins, such as IL-6 and other cytokines and chemokines. The interleukin-6/interleukin-10 ratio is an effective biomarker for discriminating M. pneumoniae pneumonia from respiratory syncytial virus pneumonia (84). The severity of lung injury in M. pneumoniae infection may be associated with the extent of host immune reaction against the amount of etiologic or inflammation-inducing substances in the acute stage. Thus, early control of this process is critical in immune modulator (corticosteroid) treatment of M. pneumoniae infection (85). Also, early control of lung injuries from initial hyperactive immune reactions is crucial for reduction of morbidity and prevention of pneumonia progression and complications in patients with MPP94. In the era of macrolide-resistant M. pneumoniae strains and based on immunopathogenesis of M. pneumoniae, now early immune modulators, including corticosteroids, are reliable to use for M. pneumoniae (86, 87). The effect of immune modulators (corticosteroid and/or intravenous immunoglobulin) on immune cells is also dose-dependent, and the dose of corticosteroid use can be adjusted according to severity of the disease. Thus, it is possible that patients with severe MPP have more severe immune disturbance and respond to the higher-dose immune modulators because of same immune pathogenesis of mild and severe MPP. The open problem now is the optimal time, dose, and schedule of immune modulator therapy based on the severity of the disease and the need for alternative antimicrobial drugs may not be that urgent (88).
M. pneumoniae infection is a main cause of CAP in children, and MRMP spreads as a result of the overuse and unnecessary usage of macrolides. The pathogenic mechanism of M. pneumoniae infection is mainly associated with the hyperimmune response of the host to the substances from the infection, thus causing lung injury and extrapulmonary manifestations. Macrolide resistance of M. pneumoniae is mainly associated with nucleotide transition mutations in domain V of the 23S rRNA gene, and A2063G is the most prevalent mutation, followed by A2064G. P1–1, MLVA4-5-7-2, and ST3 are usually the predominated types in the M. pneumoniae epidemics, but genotype shifts may occur through different time periods. The correlation between genotypes and MRMP still remains controversial. Short-term two times serological IgM (or together with IgG) test in the acute stage can be used for confirmation. The identification of MRMP can be performed by the detection of single-nucleotide mutations within the 23S rRNA gene. The routine use of macrolide for the treatment of MSMP infections can get good effect, but the effects are limited for severe MRMP infections. Early immune modulator (corticosteroid and/or intravenous immunoglobulin) treatment is crucial for controlling the immune reaction-associated cell injuries, thus reducing morbidity and preventing pneumonia progression and complications. Additional corticosteroids may be required for the treatment of severe MRMP infections in children of China during the era of MRMP.
ML contributed to definition of intellectual content, literature search, date acquisition, data analysis, manuscript preparation, and manuscript editing. JY and JZ contributed to the conception, design, manuscript editing, and manuscript review. All authors contributed to the article and approved the submitted version.
The authors declare that the research was conducted in the absence of any commercial or financial relationships that could be construed as a potential conflict of interest.
All claims expressed in this article are solely those of the authors and do not necessarily represent those of their affiliated organizations, or those of the publisher, the editors and the reviewers. Any product that may be evaluated in this article, or claim that may be made by its manufacturer, is not guaranteed or endorsed by the publisher.
1. Morozumi M, Takahashi T, Ubukata K. Macrolide-resistant Mycoplasma pneumoniae: characteristics of isolates and clinical aspects of community-acquired pneumonia. J Infect Chemother. (2010) 16(2):78–86. doi: 10.1007/s10156-009-0021-4
2. Hammerschlag MR. Mycoplasma pneumoniae infections. Curr Opin Infect Dis. (2001) 14(2):181–6. doi: 10.1097/00001432-200104000-00012
3. McIntosh K. Community-acquired pneumonia in children. N Engl J Med. (2002) 346(6):429–37. doi: 10.1056/NEJMra011994
4. Waites KB, Talkington DF. Mycoplasma pneumoniae and its role as a human pathogen. Clin Microbiol Rev. (2004) 17(4):697–728. doi: 10.1128/CMR.17.4.697-728.2004
5. Hong KB, Choi EH, Lee HJ, Lee SY, Cho EY, Choi JH, et al. Macrolide resistance of Mycoplasma pneumoniae, South Korea, 2000-2011. Emerg Infect Dis. (2013) 19(8):1281–4. doi: 10.3201/eid1908.121455
6. Yoon IA, Hong KB, Lee HJ, Yun KW, Park JY, Choi YH, et al. Radiologic findings as a determinant and no effect of macrolide resistance on clinical course of Mycoplasma pneumoniae pneumonia. BMC Infect Dis. (2017) 17(1):402. doi: 10.1186/s12879-017-2500-z
7. Yamazaki T, Kenri T. Epidemiology of Mycoplasma pneumoniae infections in Japan and therapeutic strategies for macrolide-resistant M. pneumoniae. Front Microbiol. (2016) 7:693. doi: 10.3389/fmicb.2016.00693
8. Liu X, Jiang Y, Chen X, Li J, Shi D, Xin D. Drug resistance mechanisms of Mycoplasma pneumoniae to macrolide antibiotics. Biomed Res Int. (2014) 2014:320801. doi: 10.1155/2014/320801
9. Zhao F, Liu J, Shi W, Huang F, Liu L, Zhao S, et al. Antimicrobial susceptibility and genotyping of Mycoplasma pneumoniae isolates in Beijing, China, from 2014 to 2016. Antimicrob Resist Infect Control. (2019) 8:18. doi: 10.1186/s13756-019-0469-7
10. Zhao F, Li J, Liu J, Guan X, Gong J, Liu L, et al. Antimicrobial susceptibility and molecular characteristics of Mycoplasma pneumoniae isolates across different regions of China. Antimicrob Resist Infect Control. (2019) 8:143. doi: 10.1186/s13756-019-0576-5
11. Sun H, Xue G, Yan C, Li S, Zhao H, Feng Y, et al. Changes in molecular characteristics of Mycoplasma pneumoniae in clinical specimens from children in Beijing between 2003 and 2015. PLoS One. (2017) 12(1):e0170253. doi: 10.1371/journal.pone.0170253
12. Hung HM, Chuang CH, Chen YY, Liao WC, Li SW, Chang IY, et al. Clonal spread of macrolide-resistant Mycoplasma pneumoniae sequence type-3 and type-17 with recombination on non-P1 adhesin among children in Taiwan. Clin Microbiol Infect. (2021) 27(8):1169.e1–e6. doi: 10.1016/j.cmi.2020.09.035
13. Lee JK, Lee JH, Lee H, Ahn YM, Eun BW, Cho EY, et al. Clonal expansion of macrolide-resistant sequence type 3 Mycoplasma pneumoniae, South Korea. Emerg Infect Dis. (2018) 24(8):1465–71. doi: 10.3201/eid2408.180081
14. Yang EA, Kang HM, Rhim JW, Kang JH, Lee KY. Early corticosteroid therapy for Mycoplasma pneumoniae pneumonia irrespective of used antibiotics in children. J Clin Med. (2019) 8(5):726. doi: 10.3390/jcm8050726
15. Chanock RM, Cook MK, Fox HH, Parrott RH, Huebner RJ. Serologic evidence of infection with Eaton agent in lower respiratory illness in childhood. N Engl J Med. (1960) 262:648–54. doi: 10.1056/NEJM196003312621303
16. Chanock RM, Hayflick L, Barile MF. Growth on artificial medium of an agent associated with atypical pneumonia and its identification as a PPLO. Proc Natl Acad Sci U S A. (1962) 48:41–9. doi: 10.1073/pnas.48.1.41
17. Razin S, Yogev D, Naot Y. Molecular biology and pathogenicity of mycoplasmas. Microbiol Mol Biol Rev. (1998) 62(4):1094–156. doi: 10.1128/MMBR.62.4.1094-1156.1998
18. Güell M, van Noort V, Yus E, Chen WH, Leigh-Bell J, Michalodimitrakis K, et al. Transcriptome complexity in a genome-reduced bacterium. Science. (2009) 326(5957):1268–71. doi: 10.1126/science.1176951
19. Lluch-Senar M, Cozzuto L, Cano J, Delgado J, Llórens-Rico V, Pereyre S, et al. Comparative “-omics” in Mycoplasma pneumoniae clinical isolates reveals key virulence factors. PLoS One. (2015) 10(9):e0137354. doi: 10.1371/journal.pone.0137354
20. Xiao L, Ptacek T, Osborne JD, Crabb DM, Simmons WL, Lefkowitz EJ, et al. Comparative genome analysis of Mycoplasma pneumoniae. BMC Genomics. (2015) 16(1):610. doi: 10.1186/s12864-015-1801-0
21. Waites KB, Xiao L, Liu Y, Balish MF, Atkinson TP. Mycoplasma pneumoniae from the respiratory tract and beyond. Clin Microbiol Rev. (2017) 30(3):747–809. doi: 10.1128/CMR.00114-16
22. Lee H, Yun KW, Lee HJ, Choi EH. Antimicrobial therapy of macrolide-resistant Mycoplasma pneumoniae pneumonia in children. Expert Rev Anti Infect Ther. (2018) 16(1):23–34. doi: 10.1080/14787210.2018.1414599
23. Miyashita N, Kawai Y, Akaike H, Teranishi H, Ouchi K, Okimoto N. Transmission of macrolide-resistant Mycoplasma pneumoniae within a family. J Infect Chemother. (2013) 19(6):1196–201. doi: 10.1007/s10156-013-0604-y
24. Zhang WZ, Zhang SJ, Wang QY, Li YD, Jing HB, Hu GY, et al. Outbreak of macrolide-resistant Mycoplasma pneumoniae in a primary school in Beijing, China in 2018. BMC Infect Dis. (2019) 19(1):871. doi: 10.1186/s12879-019-4473-6
25. Centers for Disease and Prevention (CDC). Mycoplasma pneumoniae infections. Atlanta: National Center for Immunization and Respiratory Diseases Division of Bacterial Diseases (2020). Available at: https://www.cdc.gov/pneumonia/atypical/mycoplasma/surv-reporting.html (Accessed January 31, 2023).
26. Tian DD, Jiang R, Chen XJ, Ye Q. Meteorological factors on the incidence of MP and RSV pneumonia in children. PLoS One. (2017) 12(3):e0173409. doi: 10.1371/journal.pone.0173409
27. Canavan TN, Mathes EF, Frieden I, Shinkai K. Mycoplasma pneumoniae-induced rash and mucositis as a syndrome distinct from Stevens-Johnson syndrome and erythema multiforme: a systematic review. J Am Acad Dermatol. (2015) 72(2):239–45. doi: 10.1016/j.jaad.2014.06.026
28. Lee KY. Pediatric respiratory infections by Mycoplasma pneumoniae. Expert Rev Anti Infect Ther. (2008) 6(4):509–21. doi: 10.1586/14787210.6.4.509
29. Lee KY. Common immunopathogenesis of central nervous system diseases: the protein-homeostasis-system hypothesis. Cell Biosci. (2022) 12(1):184. doi: 10.1186/s13578-022-00920-5
30. Youn YS, Lee KY. Mycoplasma pneumoniae pneumonia in children. Korean J Pediatr. (2012) 55(2):42–7. doi: 10.3345/kjp.2012.55.2.42
31. Macrolide antibiotics. In LiverTox: clinical and research information on drug-induced liver injury. Bethesda, MD: National Institute of Diabetes and Digestive and Kidney Diseases (2017).
32. Suzuki Y, Shimotai Y, Itagaki T, Seto J, Ikeda T, Yahagi K, et al. Development of macrolide resistance-associated mutations after macrolide treatment in children infected with Mycoplasma pneumoniae. J Med Microbiol. (2017) 66(11):1531–8. doi: 10.1099/jmm.0.000582
33. Okazaki N, Narita M, Yamada S, Izumikawa K, Umetsu M, Kenri T, et al. Characteristics of macrolide-resistant Mycoplasma pneumoniae strains isolated from patients and induced with erythromycin in vitro. Microbiol Immunol. (2001) 45:617–20. doi: 10.1111/j.1348-0421.2001.tb01293.x
34. Yang HJ, Song DJ, Shim JY. Mechanism of resistance acquisition and treatment of macrolide-resistant Mycoplasma pneumoniae pneumonia in children. Korean J Pediatr. (2017) 60(6):167–74. doi: 10.3345/kjp.2017.60.6.167
35. Lucier TS, Heitzman K, Liu SK, Hu PC. Transition mutations in the 23S rRNA of erythromycin-resistant isolates of Mycoplasma pneumoniae. Antimicrob Agents Chemother. (1995) 39:2770–3. doi: 10.1128/AAC.39.12.2770
36. Suzuki Y, Seto J, Shimotai Y, Ikeda T, Yahagi K, Mizuta K, et al. Development of an endpoint genotyping assay to detect the Mycoplasma pneumoniae 23S rRNA gene and distinguish the existence of macrolide resistance-associated mutations at position 2063. J Microbiol Methods. (2016) 131:130–4. doi: 10.1016/j.mimet.2016.10.017
37. Ando M, Morozumi M, Adachi Y, Ubukata K, Iwata S. Multilocus sequence typing of Mycoplasma pneumoniae, Japan, 2002–2016. Emerg Infect Dis. (2018) 24(10):1895–901. doi: 10.3201/eid2410.171194
38. Diaz MH, Benitez AJ, Cross KE, Hicks LA, Kutty P, Bramley AM, et al. Molecular detection and characterization of Mycoplasma pneumoniae among patients hospitalized with community-acquired pneumonia in the United States. Open Forum Infect Dis. (2015) 2(3):ofv106. doi: 10.1093/ofid/ofv106
39. Matsuoka M, Narita M, Okazaki N, Ohya H, Yamazaki T, Ouchi K, et al. Characterization and molecular analysis of macrolideresistant Mycoplasma pneumoniae clinical isolates obtained in Japan. Antimicrob Agents Chemother. (2004) 48:4624–30. doi: 10.1128/AAC.48.12.4624-4630.2004
40. Matsubara K, Morozumi M, Okada T, Matsushima T, Komiyama O, Shoji M, et al. A comparative clinical study of macrolide-sensitive and macrolide-resistant Mycoplasma pneumoniae infections in pediatric patients. J Infect Chemother. (2009) 15(6):380–3. doi: 10.1007/s10156-009-0715-7
41. Diaz MH, Benitez AJ, Winchell JM. Investigations of Mycoplasma pneumoniae infections in the United States: trends in molecular typing and macrolide resistance from 2006 to 2013. J Clin Microbiol. (2015) 53(1):124–30. doi: 10.1128/JCM.02597-14
42. Xiao L, Ratliff AE, Crabb DM, Mixon E, Qin X, Selvarangan R, et al. Molecular characterization of Mycoplasma pneumoniae isolates in the United States from 2012 to 2018. J Clin Microbiol. (2020) 58(10):e00710–20. doi: 10.1128/JCM.00710-20
43. Katsukawa C, Kenri T, Shibayama K, Takahashi K. Genetic characterization of Mycoplasma pneumoniae isolated in Osaka between 2011 and 2017: decreased detection rate of macrolide-resistance and increase of p1 gene type 2 lineage strains. PLoS One. (2019) 14(1):e0209938. doi: 10.1371/journal.pone.0209938
44. Dou HW, Tian XJ, Xin L, Wei R, Zhou W, Wang H, et al. Mycoplasma pneumoniae macrolide resistance and MLVA typing in children in Beijing, China, in 2016: is it relevant? Biomed Environ Sci. (2020) 33(12):916–24. doi: 10.3967/bes2020.125
45. Lu CY, Yen TY, Chang LY, Liau YJ, Liu HH, Huang LM. Multiple-locus variable-number tandem-repeat analysis (MLVA) of macrolide-susceptible and -resistant Mycoplasma pneumoniae in children in Taiwan. J Formos Med Assoc. (2020) 119(10):1539–45. doi: 10.1016/j.jfma.2019.12.008
46. Rivaya B, Jordana-Lluch E, Fernández-Rivas G, Molinos S, Campos R, Méndez-Hernández M, et al. Macrolide resistance and molecular typing of Mycoplasma pneumoniae infections during a 4 year period in Spain. J Antimicrob Chemother. (2020) 75(10):2752–9. doi: 10.1093/jac/dkaa256
47. Meyer Sauteur PM, Pánisová E, Seiler M, Theiler M, Berger C, Dumke R. Mycoplasma pneumoniae genotypes and clinical outcome in children. J Clin Microbiol. (2021) 59(7):e0074821. doi: 10.1128/JCM.00748-21
48. Cardinale F, Chironna M, Chinellato I, Principi N, Esposito S. Clinical relevance of Mycoplasma pneumoniae macrolide resistance in children. J Clin Microbiol. (2013) 51(2):723–4. doi: 10.1128/JCM.02840-12
49. Suzuki S, Yamazaki T, Narita M, Okazaki N, Suzuki I, Andoh T, et al. Clinical evaluation of macrolide-resistant Mycoplasma pneumoniae. Antimicrob Agents Chemother. (2006) 50(2):709–12. doi: 10.1128/AAC.50.2.709-712.2006
50. Chen YC, Hsu WY, Chang TH. Macrolide-resistant Mycoplasma pneumoniae infections in pediatric community-acquired pneumonia. Emerg Infect Dis. (2020) 26(7):1382–91. doi: 10.3201/eid2607.200017
51. Shi L, Chen L, Yu J, Lin M, He S. Clinical analysis of Mycoplasma pneumoniae pneumonia with 23SrRNA A2063G gene mutation in 24 cases. Chin Pediatr Emerg Med. (2017) 24(03):205–9 (in Chinese). doi: 10.3760/cma.j.issn.1673-4912.2017.03.000
52. Kim JH, Kim JY, Yoo CH, Seo WH, Yoo Y, Song DJ, et al. Macrolide resistance and its impacts on M. pneumoniae pneumonia in children: comparison of two recent epidemics in Korea. Allergy Asthma Immunol Res. (2017) 9(4):340–6. doi: 10.4168/aair.2017.9.4.340
53. Dumke R, Catrein I, Herrmann R, Jacobs E. Preference, adaptation and survival of Mycoplasma pneumoniae subtypes in an animal model. Int J Med Microbiol. (2004) 294:149–55. doi: 10.1016/j.ijmm.2004.06.020
54. Ye Q, Mao JH, Shu Q, Shang SQ. Mycoplasma pneumoniae induces allergy by producing P1-specific immunoglobulin E. Ann Allergy Asthma Immunol. (2018) 121(1):90–7. doi: 10.1016/j.anai.2018.03.014
55. Cousin-Allery A, Charron A, de Barbeyrac B, Fremy G, Skov Jensen J, Renaudin H, et al. Molecular typing of Mycoplasma pneumoniae strains by PCR-based methods and pulsed-field gel electrophoresis. Application to French and Danish isolates. Epidemiol Infect. (2000) 124(1):103–11. doi: 10.1017/S0950268899003313
56. Dumke R, Catrein I, Pirkil E, Herrmann R, Jacobs E. Subtyping of Mycoplasma pneumoniae isolates based on extended genome sequencing and on expression profiles. Int J Med Microbiol IJMM. (2003) 292(7-8):513–25. doi: 10.1078/1438-4221-00231
57. Dallo SF, Horton JR, Su CJ, Baseman JB. Restriction fragment length polymorphism in the cytadhesin P1 gene of human clinical isolates of Mycoplasma pneumoniae. Infect Immun. (1990) 58(6):2017–20. doi: 10.1128/iai.58.6.2017-2020.1990
58. Copete AR, Aguilar YA, Rueda ZV, Vélez LA. Genotyping and macrolide resistance of Mycoplasma pneumoniae identified in children with community-acquired pneumonia in Medellín, Colombia. Int J Infect Dis. (2018) 66:113–20. doi: 10.1016/j.ijid.2017.11.019
59. Sun H, Xue G, Yan C, Li S, Cao L, Yuan Y, et al. Multiple-locus variable-number tandem-repeat analysis of Mycoplasma pneumoniae clinical specimens and proposal for amendment of MLVA nomenclature. PLoS One. (2013) 8(5):e64607. doi: 10.1371/journal.pone.0064607
60. Kenri T, Suzuki M, Sekizuka T, Ohya H, Oda Y, Yamazaki T, et al. Periodic genotype shifts in clinically prevalent Mycoplasma pneumoniae strains in Japan. Front Cell Infect Microbiol. (2020) 10:385. doi: 10.3389/fcimb.2020.00385
61. Brown RJ, Holden MT, Spiller OB, Chalker VJ. Development of a multilocus sequence typing scheme for molecular typing of Mycoplasma pneumoniae. J Clin Microbiol. (2015) 53(10):3195–203. doi: 10.1128/JCM.01301-15
62. Yan C, Yang H, Sun H, Zhao H, Feng Y, Xue G, et al. Diversity in genotype distribution of Mycoplasma pneumoniae obtained from children and adults. Jpn J Infect Dis. (2020) 73(1):14–8. doi: 10.7883/yoken.JJID.2019.037
63. Cho HK. Consideration in treatment decisions for refractory Mycoplasma pneumoniae pneumonia. Clin Exp Pediatr. (2021) 64(9):459–67. doi: 10.3345/cep.2020.01305
64. Dumke R, Schurwanz N, Lenz M, Schuppler M, Lück C, Jacobs E. Sensitive detection of Mycoplasma pneumoniae in human respiratory tract samples by optimized real-time PCR approach. J Clin Microbiol. (2007) 45(8):2726–30. doi: 10.1128/JCM.00321-07
65. Nilsson AC, Björkman P, Persson K. Polymerase chain reaction is superior to serology for the diagnosis of acute Mycoplasma pneumoniae infection and reveals a high rate of persistent infection. BMC Microbiol. (2008) 8:93. doi: 10.1186/1471-2180-8-93
66. Zhao H, Yan C, Feng Y, Xue G, Li S, Cui J, et al. Comparison RNA with DNA detection assay in diagnosis of Mycoplasma pneumoniae infection. Chin J Appl Clin Pediatr. (2021) 36(10):736–9 (in Chinese). doi: 10.3760/cma.j.cn101070-20200306-00333
67. Beersma MF, Dirven K, van Dam AP, Templeton KE, Claas EC, Goossens H. Evaluation of 12 commercial tests and the complement fixation test for Mycoplasma pneumoniae-specific immunoglobulin G (IgG) and IgM antibodies, with PCR used as the “gold standard”. J Clin Microbiol. (2005) 43:2277–85. doi: 10.1128/JCM.43.5.2277-2285.2005
68. Lee WJ, Huang EY, Tsai CM, Kuo KC, Huang YC, Hsieh KS, et al. Role of serum Mycoplasma pneumoniae IgA, IgM, and IgG in the diagnosis of Mycoplasma pneumoniae-related pneumonia in school-age children and adolescents. Clin Vaccine Immunol. (2017) 24(1):e00471–16. doi: 10.1128/CVI.00471-16
69. Jeon HE, Kang HM, Yang EA, Han HY, Han SB, Rhim JW, et al. Early confirmation of Mycoplasma pneumoniae infection by two short-term serologic IgM examination. Diagnostics. (2021) 11(2):353. doi: 10.3390/diagnostics11020353
70. Gavranich JB, Chang AB. Antibiotics for community acquired lower respiratory tract infections (LRTI) secondary to Mycoplasma pneumoniae in children. Cochrane Database Syst Rev. (2005) (3):CD004875. doi: 10.1002/14651858.CD004875.pub2
71. Talkington DF, Shott S, Fallon MT, Schwartz SB, Thacker WL. Analysis of eight commercial enzyme immunoassay tests for detection of antibodies to Mycoplasma pneumoniae in human serum. Clin Diagn Lab Immunol. (2004) 11:862–7. doi: 10.1128/CDLI.11.5.862-867.2004
72. Meyer Sauteur PM, Pánisová E, Bachmann LM, Ambroggio L, Berger C. Evaluation of IgM lateral flow assay as a screening tool for Mycoplasma pneumoniae infection in childhood pneumonia. J Clin Microbiol. (2020) 58(11):e01498–20. doi: 10.1128/JCM.01498-20
73. Chang HY, Chang LY, Shao PL, Lee PI, Chen JM, Lee CY, et al. Comparison of real-time polymerase chain reaction and serological tests for the confirmation of Mycoplasma pneumoniae infection in children with clinical diagnosis of atypical pneumonia. J Microbiol Immunol Infect. (2014) 47(2):137–44. doi: 10.1016/j.jmii.2013.03.015
74. Lee SC, Youn YS, Rhim JW, Kang JH, Lee KY. Early serologic diagnosis of Mycoplasma pneumoniae pneumonia: an observational study on changes in titers of specific-IgM antibodies and cold agglutinins. Medicine. (2016) 95(19):e3605. doi: 10.1097/MD.0000000000003605
75. Li J, Liu L, He L, Meng F, Zhang J, Zhao F. First report of macrolide-resistant and -susceptible Mycoplasma pneumoniae clinical strains isolated from a single case. J Glob Antimicrob Resist. (2021) 24:228–32. doi: 10.1016/j.jgar.2020.12.019
76. Chan KH, To KK, Chan BW, Li CP, Chiu SS, Yuen KY, et al. Comparison of pyrosequencing, Sanger sequencing, and melting curve analysis for detection of low-frequency macrolide-resistant Mycoplasma pneumoniae quasispecies in respiratory specimens. J Clin Microbiol. (2013) 51(8):2592–8. doi: 10.1128/JCM.00785-13
77. Diaz MH, Winchell JM. The evolution of advanced molecular diagnostics for the detection and characterization of Mycoplasma pneumoniae. Front Microbiol. (2016) 7:232. doi: 10.3389/fmicb.2016.00232
78. Uehara S, Sunakawa K, Eguchi H, Ouchi K, Okada K, Kurosaki T, et al. Japanese Guidelines for the Management of Respiratory Infectious Diseases in Children 2007 with focus on pneumonia. Pediatr Int. (2011) 53(2):264–76. doi: 10.1111/j.1442-200X.2010.03316.x
79. Volovitz B, Shkap R, Amir J, Calderon S, Varsano I, Nussinovitch M. Absence of tooth staining with doxycycline treatment in young children. Clin Pediatr. (2007) 46(2):121–6. doi: 10.1177/0009922806290026
80. Dai FF, Liu FQ, Chen X, Yang J, Wang K, Guo CY. The treatment of macrolide-resistant Mycoplasma pneumoniae pneumonia in children. J Clin Pharm Ther. (2021) 46(3):705–10. doi: 10.1111/jcpt.13331
81. Bébéar C, Pereyre S, Peuchant O. Mycoplasma pneumoniae: susceptibility and resistance to antibiotics. Future Microbiol. (2011) 6(4):423–31. doi: 10.2217/fmb.11.18
82. Gruson D, Pereyre S, Renaudin H, Charron A, Bébéar C, Bébéar CM. In vitro development of resistance to six and four fluoroquinolones in Mycoplasma pneumoniae and Mycoplasma hominis, respectively. Antimicrob Agents Chemother. (2005) 49(3):1190–3. doi: 10.1128/AAC.49.3.1190-1193.2005
83. Dégrange S, Renaudin H, Charron A, Pereyre S, Bébéar C, Bébéar CM. Reduced susceptibility to tetracyclines is associated in vitro with the presence of 16S rRNA mutations in Mycoplasma hominis and Mycoplasma pneumoniae. J Antimicrob Chemother. (2008) 61(6):1390–2. doi: 10.1093/jac/dkn118
84. Zhou JM, Ye Q. Utility of assessing cytokine levels for the differential diagnosis of pneumonia in a pediatric population. Pediatr Crit Care Med. (2017) 18(4):e162–6. doi: 10.1097/PCC.0000000000001092
85. Rhim JW, Kang JH, Lee KY. Etiological and pathophysiological enigmas of severe coronavirus disease 2019, multisystem inflammatory syndrome in children, and Kawasaki disease. Clin Exp Pediatr. (2022) 65(4):153–66. doi: 10.3345/cep.2021.01270
86. Lee KY, Rhim JW, Kang JH. Immunopathogenesis of COVID-19 and early immunomodulators. Clin Exp Pediatr. (2020) 63(7):239–50. doi: 10.3345/cep.2020.00759
87. Youn YS, Lee SC, Rhim JW, Shin MS, Kang JH, Lee KY. Early additional immune-modulators for Mycoplasma pneumoniae pneumonia in children: an observation study. Infect Chemother. (2014) 46(4):239–47. doi: 10.3947/ic.2014.46.4.239
Keywords:
Citation: Leng M, Yang J and Zhou J (2023) The molecular characteristics, diagnosis, and treatment of macrolide-resistant
Received: 3 December 2022; Accepted: 13 February 2023;
Published: 2 March 2023.
Edited by:
Ramos Amador Jose T., Complutense University of Madrid, SpainReviewed by:
Anil Tapisiz, Gazi University, Türkiye© 2023 Leng, Yang and Zhou. This is an open-access article distributed under the terms of the Creative Commons Attribution License (CC BY). The use, distribution or reproduction in other forums is permitted, provided the original author(s) and the copyright owner(s) are credited and that the original publication in this journal is cited, in accordance with accepted academic practice. No use, distribution or reproduction is permitted which does not comply with these terms.
*Correspondence: Junmei Yang eWFuZ2p1bm1laTc2ODNAMTYzLmNvbQ==
Specialty Section: This article was submitted to Pediatric Infectious Diseases, a section of the journal Frontiers in Pediatrics
Disclaimer: All claims expressed in this article are solely those of the authors and do not necessarily represent those of their affiliated organizations, or those of the publisher, the editors and the reviewers. Any product that may be evaluated in this article or claim that may be made by its manufacturer is not guaranteed or endorsed by the publisher.
Research integrity at Frontiers
Learn more about the work of our research integrity team to safeguard the quality of each article we publish.