- 1Department of Pediatrics, University of Cincinnati College of Medicine, Cincinnati, OH, United States
- 2The Heart Institute, Cincinnati Children’s Hospital Medical Center, Cincinnati, OH, United States
Background: Little is known about how sport and school restrictions early during the novel coronavirus 2019 (COVID-19) pandemic impacted exercise performance and body composition in youth with heart disease (HD).
Methods: A retrospective chart review was performed on all patients with HD who had serial exercise testing and body composition via bioimpedance analysis performed within 12 months before and during the COVID-19 pandemic. Formal activity restriction was noted as present or absent. Analysis was performed with a paired t-test.
Results: There were 33 patients (mean age 15.3 ± 3.4 years; 46% male) with serial testing completed (18 electrophysiologic diagnosis, 15 congenital HD). There was an increase in skeletal muscle mass (SMM) (24.1 ± 9.2–25.9 ± 9.1 kg, p < 0.0001), weight (58.7 ± 21.5–63.9 ± 22 kg, p < 0.0001), and body fat percentage (22.7 ± 9.4–24.7 ± 10.4%, p = 0.04). The results were similar when stratified by age <18 years old (n = 27) or by sex (male 16, female 17), consistent with typical pubertal changes in this predominantly adolescent population. Absolute peak VO2 increased, but this was due to somatic growth and aging as evidenced by no change in % of predicted peak VO2. There remained no difference in predicted peak VO2 when excluding patients with pre-existing activity restrictions (n = 12). Review of similar serial testing in 65 patients in the 3 years before the pandemic demonstrated equivalent findings.
Conclusions: The COVID-19 pandemic and related lifestyle changes do not appear to have had substantial negative impacts on aerobic fitness or body composition in children and young adults with HD.
1. Introduction
Concerns about cardiac damage following coronavirus disease 2019 (COVID-19) and society-wide mitigation strategies disrupted children's lives during the COVID-19 pandemic, particularly during the early stages. Policies, such as school and sport restriction, dramatically altered daily routines, leading to more school-based and leisure screen time and less time in active play with their peers (1). Decreased physical activity because of COVID-19 related restrictions has been shown in multiple studies in pediatrics (1–3). Little is known about the effect this has had on fitness and body composition in pediatric and congenital heart disease (CHD), individuals who are often already deconditioned. The only known pediatric CHD study included a combination of individuals with and without heart disease, reporting a decline in peak oxygen consumption (VO2) on serial exercise testing performed during the pandemic (4).The aims of this study were to: (1) understand how COVID-19 mitigation strategies affected fitness and also body composition in youth with heart disease, and (2) determine if children with heart disease and pre-existing exercise restrictions were more likely to have alterations in their fitness or body composition compared to without such restriction.
2. Materials and methods
This study was approved by the Cincinnati Children's Hospital Medical Center Institutional Review Board, and informed consent was not required for this study.
2.1. Patients
We identified all individuals with heart disease ≤21 years old at the time of the first test, and who underwent a routine cardiopulmonary exercise test (CPET) at Cincinnati Children's Hospital within a year before and after March 21, 2020 (i.e., the date that extensive COVID-19 mitigation strategies including school and sports cancellations were established locally). This group we defined as the “COVID lockdown” study group. Heart disease was defined as either electrocardiographic (i.e., Long QT syndrome, catecholaminergic polymorphic ventricular tachycardia) or CHD (bicuspid aortic valve, Fontan physiology, etc). We included only those for whom exercise testing was performed as routine standard of care and not secondary to cardiac symptoms or concern for deterioration (i.e., testing to assess for adequate beta-blockade if long QT patients, evaluate for serial changes in peak VO2 in CHD patients for prognostic purposes, ST segment/T wave changes in patients with left ventricular outflow tract obstruction). This was evaluated on chart review utilizing the electronic medical record. Additional exclusion criteria included a submaximal test (details below) and missing data. Additionally, to determine if any changes in fitness were secondary to COVID-19 mitigation or rather typical fitness changes in patients with heart disease, an age, sex, and diagnosis-matched historical control group was performed for those with serial CPET performed during the 3 years prior to the COVID-19 pandemic. To help account for other confounders, following initial result reporting a sensitivity analysis was performed to evaluate for differences in serial testing by excluding patients with pre-existing activity restrictions, adult patients, and those with a percent predicted peak VO2 < 80%. Lastly, all patients tested during the COVID-19 pandemic required a negative PCR test on a nasopharyngeal swab prior to their CPET.
2.2. Study measures
2.2.1. Baseline data
We extracted demographic and other baseline data from the electronic medical record including age, sex, height, weight, race, medications, cardiac pacemaker, and documented sport restrictions.
2.2.2. Bioimpedance assessment
Anthropometric data were measured by Bioimpedance assessment (BIA) (InBody370; InBody, Cerritos, CA, United States) immediately before the CPET, as previously described (5). Bioelectrical impedance analysis (BIA) is part of our local standard of care prior to CPET and is an alternative approach to measure body composition with good agreement to dual energy x-ray absorptiometry (6, 7).
2.2.3. CPET
Exercise testing was performed on a stationary cycle ergometer (Corival; Lode; Groningen, The Netherlands) with an individualized incremental ramp protocol. The rate of increase was chosen by experienced clinical exercise physiologists based on the patient's body size and expected fitness, targeting an exercise duration of approximately 10 min. Cardiopulmonary responses to exercise were assessed breath-by-breath (Ultima CardiO2; MGC Diagnostics; Saint Paul, MN, United States). Criteria for a maximal effort exercise test were that 2 of the following 3 criteria were met: respiratory exchange ratio >1.10; maximal heart rate greater >85% of the age-predicted maximum (220 - age in years); or maximal Rating of Perceived Exertion ≥18 on a 6–20 scale (8). Predicted peak VO2 was calculated per Wasserman et al. and Cooper et al. (9, 10). In adult patients with a body mass index (BMI) <18 and >25, the underweight and overweight regression equations were used, respectively (9).
2.3. Statistics
Descriptive normally distributed data are presented as mean ± standard deviation. Differences between serial tests for both the COVID-19 lockdown and historical control groups were assessed using a paired t-test. To determine if there was a difference in the baseline fitness testing of both the control and COVID groups, a 2-sided t-test was performed with each group's initial CPET results. All presented p-values are two-tailed (where applicable) and differences and associations were considered significant when p < 0.05. Statistical analyses were performed using JMP®, Version 15 (SAS Institute Inc., Cary, NC).
3. Results
There were a total of 48 patients who underwent serial testing, with the first test performed within 12 months before and the second test performed within 12 months after the COVID-19 lockdown. There were 15 patients excluded (6 tested because of concern for clinical decompensation, 6 submaximal effort tests, 3 missing data). There were 33 remaining patients (mean age 15.3 ± 3.4 years at the time of the first test; 46% male) who were included in the analysis. Underlying cardiac diagnoses are presented in Table 1. Primary diagnosis was related to electrophysiologic disease in 18 patients, while 15 had structural heart disease. Of note, 36% (12/33) were restricted from sports before COVID-19 with the reason for sports restriction being concern about ventricular arrhythmia in 10 patients and severe left ventricular outflow tract obstruction in 2 patients. In the COVID group, 58% (19/33) were prescribed beta-blockers. None had a cardiac pacemaker, had medication changes, or experienced unexpected arrhythmias during the test.
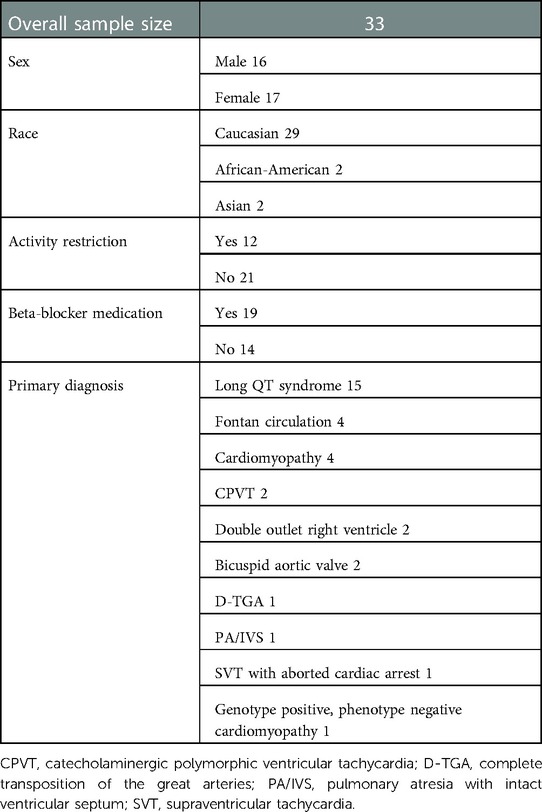
Table 1. Demographic data for the young cardiology patients with serial cardiopulmonary exercise tests in the 12 months before and after the COVID-19 lockdown.
There was no significant difference in sport restriction and beta-blocker usage between the post COVID-19 lockdown and historical control groups. No patients were diagnosed with COVID-19 prior to their exercise test.
In the COVID-19 lockdown group, the mean time between tests was 1.1 years, ranging from 0.6 to 1.5 years. There was an increase in weight (58.7 ± 21.5–63.9 ± 22 kg, p < 0.0001), skeletal muscle mass (24.1 ± 9.2–25.9 ± 9.1 kg, p < 0.0001), and body fat percentage (22.7 ± 9.4–24.7 ± 10.4%, p = 0.04) without significant change in height (165.5 ± 16.4–167 cm ± 14.2 cm, p = 0.1) or body mass index (21.7 ± 4.8 vs. 22.5 ± 5.5 kg/m2, p = 0.2) (Table 2).
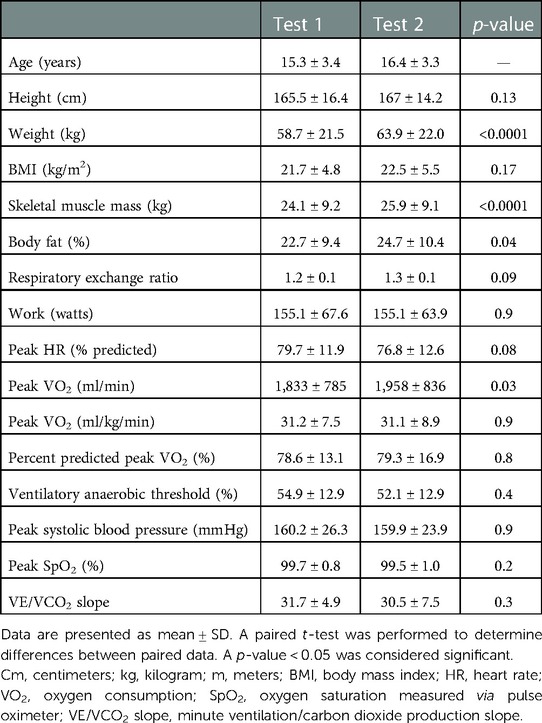
Table 2. Results of serial testing of anthropometric data as measured by bioimpedance analysis and cardiopulmonary exercise testing in 33 pediatric and congenital heart disease patients before and during the COVID-19 lockdown.
There was an increase in absolute peak VO2 (1,832.9 ± 784.8–1,958.2 ± 835.9 ml/min, p = 0.03), but no change in peak VO2 when expressed as a percent of predicted values or when indexed to body mass (78.6 ± 13.1–79.3 ± 16.9%, p = 0.8; 31.2 ± 7.5–31.1 ± 8.9 ml/kg/min, p = 0.9). There was no significant change between tests in any of the other exercise variables (Table 2).
When further evaluating the COVID-19 lockdown group, body composition and CPET differences by sex are provided in Table 3. Of note, male patients had an increase in weight (68.9 ± 24–74.6 ± 24.2 kg, p = 0.02) and skeletal muscle mass (29.5 ± 9.1–32.7 ± 8.3 kg, p = 0.002) without a significant change in adiposity (18.9 ± 10–18.8 ± 9.5% body fat, p = 0.8) or height (176.1 ± 12.2–176.4 cm ± 11.9 cm, p = 0.9). Female patients had an increase in height (155.4 ± 13.3–158.2 cm ± 10.2 cm, p = 0.008), weight (49 ± 13.253.8 ± 14 kg, p < 0.0001), skeletal muscle mass (18.7 ± 5.4–19.8 ± 4.2 kg, p = 0.005), and body fat (26.4 ± 7.3–29.8 ± 8.4%, p = 0.03). Male patients had a significant difference between absolute peak VO2 but not peak VO2 indexed to weight or as a percent of predicted values. There were no other significant differences based on sex.
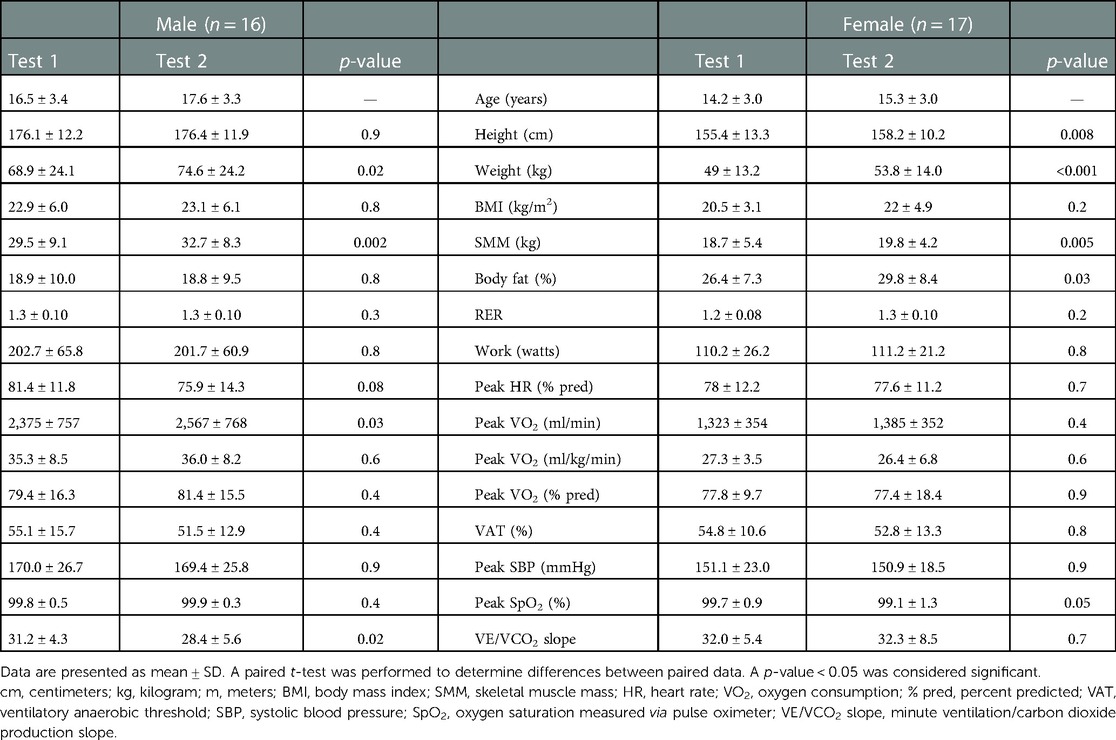
Table 3. Differences in anthropometric and exercise testing data by sex in the pediatric and congenital heart disease patients tested before and during the COVID-19 lockdown.
When comparing with the cohort that had testing in the years prior to the COVID-19 pandemic (historical control) (N = 65; mean age 14.9 ± 3.3 years at the first test; 49% male), there was no difference in the sex, diagnoses, age, or size between groups. On comparing baseline CPET results between the COVID-19 lockdown and historical control groups, there were no significant differences in any of the CPET parameters studied. In the historical control group, there was no significant change in absolute peak VO2 (1,826.8 ± 696.8–1,866.7 ± 655.6 ml/min, p = 0.4) or percent predicted peak VO2 (78.3 ± 15.3–75.9 ± 17.7%, p = 0.2), though there was a decrease in peak heart rate (82.1 ± 11.4–78.1 ± 14.0%, p < 0.001). The ventilatory anaerobic threshold was lower on serial testing in the cohort with testing before the pandemic (54.3 ± 13.2–50.9 ± 14.6, p = 0.02) (Supplementary Table S1).
3.1. Sensitivity analysis
Excluding the 12 patients with pre-existing activity restrictions, the remaining 21 patients in the COVID lockdown group also experienced no change in percent predicted peak VO2. There was no significant change in the percent predicted peak VO2 between tests, although the absolute values trended towards abnormal in both the activity restricted (77.2 ± 8.3–76.8 ± 15.1%, p = 0.9) and not restricted (79.4 ± 15.4–80.8 ± 18.1%, p = 0.7) groups. Skeletal muscle mass increased in both those who did and did not have an activity restriction, but those who were restricted had a smaller increase (restricted 24.7 ± 7.9–26.5 ± 7.4 kg, p = 0.02; not restricted 23.7 ± 10.1–26.3 ± 10.2 kg, p = 0.0006). Neither group had a significant increase in body fat percentage >2% (restricted 24.3 ± 7.9–26.3 ± 9.3%, p = 0.3; not restricted 21.7 ± 10.3–23.7 ± 11.1%, p = 0.07).
Results were similar after excluding the 7 patients in the COVID lockdown group who were >18 years old at the initial test (Supplementary Table S2). The 7 patients >18 years old had a significant decrease in total work (221.4 ± 47.1–207.3 ± 49.4 watts, p = 0.04), but there was no change in other CPET variables. When removing patients with a peak VO2 < 80%, there remained no difference in percent predicted peak VO2 in those with more normal fitness (89.6 ± 11.2 vs. 89.3 ± 16.4, p = 0.9). Lastly, the groups with a primary electrophysiologic diagnosis were also similar to those with a structural heart disease diagnosis in terms of change in percent predicted peak VO2.
4. Discussion
Findings from this observational study revealed no significant change in the aerobic capacity or body composition, beyond those associated with puberty, from before to after the COVID-19 pandemic and related societal lockdown. This is notable, as there has previously been concern that COVID mitigation could affect exercise habits in this population (1, 11). There were no differences in fitness when comparing serial testing separated by either sex, activity restriction, or in the matched paired historical control group. There were changes in body composition seen between tests, but this was likely secondary to normal pubertal maturation in a largely pediatric cohort.
There have been reports of decreased physical activity related to COVID-19 mitigation strategies both in healthy children and in those with heart disease (1, 11). Whether or not our cohort engaged in less physical activity is unknown, but their objective measures of fitness did not change, which is the opposite of what was reported by Burstein DS et al. (4). This may mean many of the patients were already deconditioned at the time of the initial CPET, as evidenced by an average peak VO2 of ∼78% of predicted, compared to ∼96% in the Burstein DS et al. cohort (4). Additionally, the lack of change in fitness may be secondary to pre-existing activity restrictions applied by the patients' medical teams, although the lack of change in VO2 in those not activity restricted argues against this. Lastly, this may mean that the introduction of lockdown measures did not alter their physical activity as they were already sedentary and deconditioned, which is not unusual for a typical congenital heart disease cohort. Prior to COVID-19, youth with congenital heart disease were shown to be more sedentary than their peers (12, 13). Thus, the introduction of lockdown measures may not have resulted in a significant change in their physical activity levels. This is further supported by the lack of change in the already low peak VO2 on serial testing in the pre-COVID control group.
This study continues to highlight the need to encourage physical activity in youth with congenital heart disease. While the absence of direct negative effects from the lockdown on fitness in children with heart disease is reassuring, this population, both in our cohort and others, has been shown to be significantly deconditioned (14–16). This is meaningful, since decreased peak VO2 has both negative prognostic and functional implications (17–19). Recreational and competitive activities should be encouraged in this population with an eye towards the risk: benefit calculation, balancing the concerns for sudden cardiac arrest during athletics, and the long-term morbidity and mortality that arises from sedentary behaviors. For individuals so severely deconditioned that participation in organized sports would be impractical, exercise prescription and structured cardiac rehabilitation should be encouraged to improve cardiopulmonary fitness (20).
While this study focused on evaluating the effect of COVID-19 mitigation strategies on the body composition of children and young adults with heart disease, body composition changed during the study period in a pattern that is likely consistent with normal pubertal changes (21). Increase in both skeletal muscle mass and body fat percentage results in an increase in total weight in a pubertal population, which was seen in our cohort. The increase in the total weight for the males was largely driven by increases in skeletal muscle mass while the increase in weight for the females was largely due to increased adiposity; this is in keeping with typical sex-specific pubertal body composition changes (21). These changes remained when the 7 patients older than 18 years-old were removed from the analysis, again supporting expected somatic growth as the likely explanation. The lack of significant change in predicted peak VO2 in this cohort further supports that these body composition changes were most likely related to normal, expected growth. Unfortunately, secondary to the retrospective design of this study we do not have specific information on the exact pubertal stage of these patients. This should be taken into account for future pediatric studies involving serial body composition measurements.
There were other limitations to this analysis. The available sample size limits statistical power, and a subset of the null findings may represent false negative findings, particularly those with a small effect size. The comparison group lacked body composition data, as the InBody Bioimpedance scale was not widely used in our laboratory until early 2019; thus it is unknown if the changes in body composition in the study cohort is reproduced in the age, sex, and diagnosis-matched cohort. Additionally, questions on specific exercise habits and sports participation are not routinely asked by all of the providers in our hospital. Additional sampling bias may also exist secondary to the retrospective design of the study.
5. Conclusion
The COVID-19 pandemic and related lifestyle changes do not appear to have had an substantial negative impact on aerobic fitness in children and young adults with heart disease. While this is largely reassuring, these finding may be in part related to pre-existing chronic deconditioning, pre-limited participation in organized sport, or sampling bias.
Data availability statement
The raw data supporting the conclusions of this article will be made available by the authors, without undue reservation.
Ethics statement
The studies involving human participants were reviewed and approved by Cincinnati Children's Hospital IRB. Written informed consent from the participants’ legal guardian/next of kin was not required to participate in this study in accordance with the national legislation and the institutional requirements. Written informed consent was not obtained from the minor(s)’ legal guardian/next of kin for the publication of any potentially identifiable images or data included in this article.
Author contributions
All authors had full control of the design of the study, methods used, outcome parameters, analysis of data, and production of the written report.
Funding
Drs Powell and Opotowsky were supported by the Cincinnati Children’s Hospital Heart Institute Research Core (HIRC).
Acknowledgments
The authors would like to thank the Exercise Laboratory staff at Cincinnati Children's Hospital for their fantastic work and dedication to our patients during the COVID-19 pandemic.
Conflict of interest
The authors declare that the research was conducted in the absence of any commercial or financial relationships that could be construed as a potential conflict of interest.
The handling editor [DAW] declared a past co-authorship with the author(s) [AWP, WAM, SGW, ARO, SKK].
Publisher's note
All claims expressed in this article are solely those of the authors and do not necessarily represent those of their affiliated organizations, or those of the publisher, the editors and the reviewers. Any product that may be evaluated in this article, or claim that may be made by its manufacturer, is not guaranteed or endorsed by the publisher.
Supplementary material
The Supplementary Material for this article can be found online at: https://www.frontiersin.org/articles/10.3389/fped.2023.1088972/full#supplementary-material.
References
1. Dunton GF, Do B, Wang SD. Early effects of the COVID-19 pandemic on physical activity and sedentary behavior in children living in the U.S. BMC Public Health. (2020) 20(1):1351. doi: 10.1186/s12889-020-09429-3
2. Kouis P, Michanikou A, Anagnostopoulou P, Galanakis E, Michaelidou E, Dimitriou H, et al. Use of wearable sensors to assess compliance of asthmatic children in response to lockdown measures for the COVID-19 epidemic. Sci Rep. (2021) 11(1):5895. doi: 10.1038/s41598-021-85358-4
3. Zachurzok A, Wójcik M, Gawlik A, Starzyk JB, Mazur A. An attempt to assess the impact of pandemic restrictions on the lifestyle, diet, and body mass index of children with endocrine diseases-preliminary results. Nutrients. (2021) 14(1):156. doi: 10.3390/nu14010156
4. Burstein DS, Edelson J, O'Malley S, McBride MG, Stephens P, Paridon S, Brothers JA. Cardiopulmonary exercise performance in the pediatric and young adult population before and during the COVID-19 pandemic. Pediatr Cardiol. (2022) 43(8):1832–7. doi: 10.1007/s00246-022-02920-1
5. Powell AW, Wittekind SG, Alsaied T, Lubert AM, Chin C, Veldtman GR, et al. Body composition and exercise performance in youth with a Fontan circulation: a bio-impedance based study. J Am Heart Assoc. (2020) 9(24):e018345. doi: 10.1161/JAHA.120.018345
6. Ling CH, de Craen AJ, Slagboom PE, Gunn DA, Stokkel MP, Westendorp RG, et al. Accuracy of direct segmental multi-frequency bioimpedance analysis in the assessment of total body and segmental body composition in middle-aged adult population. Clin Nutr. (2011) 30(5):610–5. doi: 10.1016/j.clnu.2011.04.001
7. Kriemler S, Puder J, Zahner L, Roth R, Braun-Fahrländer C, Bedogni G. Cross-validation of bioelectrical impedance analysis for the assessment of body composition in a representative sample of 6- to 13-year-old children. Eur J Clin Nutr. (2009) 63(5):619–26. doi: 10.1038/ejcn.2008.19
9. Wasserman K, Hansen JE, Sue DY, Casaburi R, Whipp BJ. Principles of exercise testing and interpretation: Including pathophysiology and clinical applications. 3rd edn. Philadelphia, Pa: Lippincott, Williams & Wilkins (1999).
10. Cooper DM, Weiler-Ravell D, Whipp BJ, Wasserman K. Aerobic parameters of exercise as a function of body size during growth in children. J Appl Physiol. (1984) 56:628–34. doi: 10.1152/jappl.1984.56.3.628
11. Hemphill NM, Kuan MTY, Harris KC. Reduced physical activity during COVID-19 pandemic in children with congenital heart disease. Can J Cardiol. (2020) 36(7):1130–4. doi: 10.1016/j.cjca.2020.04.038
12. White DA, Willis EA, Panchangam C, Teson KM, Watson JS, Birnbaum BF, et al. Physical activity patterns in children and adolescents with heart disease. Pediatr Exerc Sci. (2020) 32(4):233–40. doi: 10.1123/pes.2020-0073
13. Moschovi D, Kapetanakis EI, Sfyridis PG, Rammos S, Mavrikaki E. Physical activity levels and self-efficacy of Greek children with congenital heart disease compared to their healthy peers. Hellenic J Cardiol. (2020) 61(3):180–6. doi: 10.1016/j.hjc.2019.01.002
14. Tran D, Maiorana A, Ayer J, Lubans DR, Davis GM, Celermajer DS, et al. Recommendations for exercise in adolescents and adults with congenital heart disease. Prog Cardiovasc Dis. (2020) 63(3):350–66. doi: 10.1016/j.pcad.2020.03.002
15. McCrindle BW, Williams RV, Mital S, Clark BJ, Russell JL, Klein G, et al. Physical activity levels in children and adolescents are reduced after the Fontan procedure, independent of exercise capacity, and are associated with lower perceived general health. Arch Dis Childhood. (2007) 92:509–14. doi: 10.1136/adc.2006.105239
16. Massin MM, Hövels-Gürich HH, Gérard P, Seghaye MC. Physical activity patterns of children after neonatal arterial switch operation. Ann Thorac Surg. (2006) 81(2):665–70. doi: 10.1016/j.athoracsur.2005.07.034
17. Diller GP, Dimopoulos K, Okonko D, Li W, Babu-Narayan SV, Broberg CS, et al. Exercise intolerance in adult congenital heart disease: comparative severity, correlates, and prognostic implication. Circulation. (2005) 112(6):828–35. doi: 10.1161/CIRCULATIONAHA.104.529800
18. Inuzuka R, Diller GP, Borgia F, Benson L, Tay ELW, Alonso-Gonzalez R, et al. Comprehensive use of cardiopulmonary exercise testing identifies adults with congenital heart disease at increased mortality risk in the medium term. Circulation. (2012) 125(2):250–9. doi: 10.1161/CIRCULATIONAHA.111.058719
19. Jacobsen RM, Ginde S, Mussatto K, Neubauer J, Earing M, Danduran M. Can a home-based cardiac physical activity program improve the physical function quality of life in children with fontan circulation? Congenit Heart Dis. (2016) 11(2):175–82. doi: 10.1111/chd.12330
20. Khoury M, Cordina R. Exercise training for people living with fontan circulation: an underutilized intervention. Can J Cardiol. (2022) 38(7):1012–23. doi: 10.1016/j.cjca.2022.01.008
Keywords: COVID-19, exercise changes, body composition, cardiopulmonary exercise test (CPET), congenital heart disaese
Citation: Powell AW, Mays WA, Wittekind SG, Chin C, Knecht SK, Lang SM and Opotowsky AR (2023) Stable fitness during COVID-19: Results of serial testing in a cohort of youth with heart disease. Front. Pediatr. 11:1088972. doi: 10.3389/fped.2023.1088972
Received: 3 November 2022; Accepted: 2 February 2023;
Published: 20 February 2023.
Edited by:
David Alexander White, Children's Mercy Hospital, United StatesReviewed by:
Danielle Burstein, University of Vermont, United StatesAimee Layton, Columbia University, United States
© 2023 Powell, Mays, Wittekind, Chin, Knecht, Lang and Opotowsky. This is an open-access article distributed under the terms of the Creative Commons Attribution License (CC BY). The use, distribution or reproduction in other forums is permitted, provided the original author(s) and the copyright owner(s) are credited and that the original publication in this journal is cited, in accordance with accepted academic practice. No use, distribution or reproduction is permitted which does not comply with these terms.
*Correspondence: Adam W. Powell QWRhbS5Qb3dlbGxAY2NobWMub3Jn
Specialty Section: This article was submitted to Pediatric Cardiology, a section of the journal Frontiers in Pediatrics