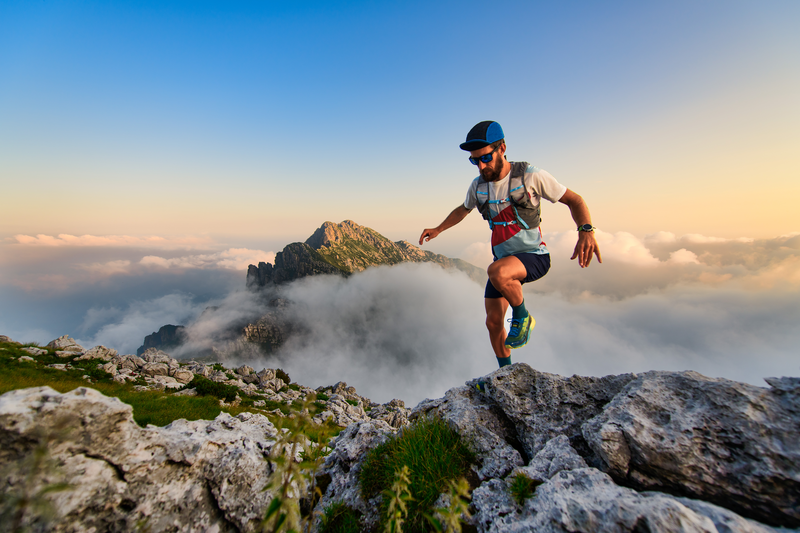
95% of researchers rate our articles as excellent or good
Learn more about the work of our research integrity team to safeguard the quality of each article we publish.
Find out more
REVIEW article
Front. Pediatr. , 29 July 2022
Sec. Children and Health
Volume 10 - 2022 | https://doi.org/10.3389/fped.2022.961509
This article is part of the Research Topic Neonatal Health in Low- and Middle-Income Countries View all 41 articles
The complications of prematurity are the leading cause of neonatal mortality worldwide, with the highest burden in the low- and middle-income countries of South Asia and Sub-Saharan Africa. A major driver of this prematurity-related neonatal mortality is respiratory distress syndrome due to immature lungs and surfactant deficiency. The World Health Organization's Every Newborn Action Plan target is for 80% of districts to have resources available to care for small and sick newborns, including premature infants with respiratory distress syndrome. Evidence-based interventions for respiratory distress syndrome management exist for the peripartum, delivery and neonatal intensive care period- however, cost, resources, and infrastructure limit their availability in low- and middle-income countries. Existing research and implementation gaps include the safe use of antenatal corticosteroid in non-tertiary settings, establishing emergency transportation services from low to high level care facilities, optimized delivery room resuscitation, provision of affordable caffeine and surfactant as well as implementing non-traditional methods of surfactant administration. There is also a need to optimize affordable continuous positive airway pressure devices able to blend oxygen, provide humidity and deliver reliable pressure. If the high prematurity-related neonatal mortality experienced in low- and middle-income countries is to be mitigated, a concerted effort by researchers, implementers and policy developers is required to address these key modalities.
Childhood mortality is predominantly driven by deaths in the neonatal period (the first 28 days of life) (1). In the last 30 years, reductions in neonatal mortality have not kept pace with those beyond the first month of life (1). Consequently, 47% or an estimated 2.4 million of all childhood deaths occur in the newborn period, with births occurring before 32 weeks gestation carrying the highest risk of death (2, 3). To focus the world's attention on needed improvements to close this gap, the Sustainable Development Goals revised targets in 2015 to reduce neonatal mortality to 12 per 1,000 live births by 2030 (4). The Every Newborn Action Plan (ENAP) identified the management of the complications of prematurity as a high-yield area for improvement critical to reducing neonatal deaths (2, 5).
Between and within-country variation in premature birth rates and prematurity-related mortality exist, with low- and middle-income countries (LMIC) carrying the highest burden (6). Over 90% of extremely preterm babies (<28 weeks) born in LMICs die within the first few days of life, while <10% of extremely preterm babies die in high-income countries (HICs) (6). Improving access to facilities capable of delivering quality neonatal care for small and sick newborns has been identified as a target of the ENAP (5). Specifically, the ENAP coverage target has called out the goal of having 80% of districts with available care for small and sick newborns (7). World Health Organization (WHO) guidelines on transforming care for small and sick newborns target key treatment modalities (Figure 1) for primary health facilities including neonatal resuscitation; secondary facilities including oxygen, continuous positive airway pressure and methylxanthines; and in addition to these, for tertiary facilities surfactant and mechanical ventilation (8).
Figure 1. Essential therapies for respiratory distress syndrome (RDS) in resource limited settings along the time course of preterm birth. Important fundamental premature care includes thermoregulation, nutrition, and management of infection. CPAP, continuous positive airway pressure.
The drivers of prematurity related mortality are multifaceted and related to the immature organ-systems of the preterm newborn. However, respiratory distress syndrome (RDS), which results from lung immaturity and surfactant deficiency, contributes about 45% of case-fatality due to prematurity in LMICs (9). Risk of RDS associated mortality is inversely related to the degree of prematurity. Given its contribution to prematurity-specific mortality, optimizing and scaling RDS specific interventions is paramount for reducing neonatal mortality at a population level, as is targeted by ENAP. In this paper, we review the pathophysiology of RDS, review RDS-specific interventions including their mode of action, available evidence that supports their use in High and LMICs and discuss research and operational gaps that exist which are specific to LMICs.
The development of RDS begins with impaired or delayed surfactant synthesis and secretion in the immature lung. Between 24 to 28 weeks of gestation, type II alveolar epithelial cells begin production of surfactant, however, this innate surfactant production is often insufficient for extra-uterine life until after 35 weeks (10). The primary function of surfactant is to reduce the surface tension of the air-liquid interface in the alveoli. When deficient, atelectasis, ventilation-perfusion mismatch, and hypoventilation ensues with resultant hypoxemia, hypercarbia, and impaired endothelial and epithelial integrity with leakage of proteinaceous exudate and formation of hyaline membranes and injury to the immature lung (11). Perinatal risk factors for developing RDS include lower gestational age and birth weight, male sex, cesarean delivery without labor, maternal diabetes, and perinatal hypoxic-ischemic events (11–13). In contrast, antenatal steroids, hypertensive disorders in pregnancy, and prolonged rupture of membranes are perinatal factors associated with a reduced risk of developing RDS (12, 14). Certain postnatal conditions including acidosis, hypothermia, hyperoxia, poor perfusion, baro and volutrauma from assisted ventilation, affect surfactant production, function, and metabolism (11).
The incidence of RDS is inversely related to gestational age. It occurs in 98% of preterm infants between 22 and 24 weeks gestation but only 25% of those with birth weight between 1,251 to 1,500 grams (15, 16). The signs of RDS are non-specific, and not all preterm infants below 34 weeks gestation presenting with respiratory distress have RDS. Examples of conditions that can mimic RDS include retained lung fluid, meconium aspiration syndrome, persistent pulmonary hypertension, and pulmonary hypoplasia (11, 17). RDS, however, clinically worsens in the first few days after birth hence, early diagnosis is important to ensure prompt treatment and transfer as necessary. RDS can increase the risk of pneumothorax and in severe cases, or where appropriate therapy is not available, can lead to respiratory failure and death (17).
In HICs, RDS is diagnosed in preterm infants who have signs including supraclavicular, intercostal, and subcostal retraction, grunting and flaring of the nares, requirement for supplemental oxygen as dictated by hypoxia from pulse oximetry, and have chest radiographic findings of diffuse haziness and air bronchograms (18). Additionally, blood gas analysis to assess for acidosis, hypoxemia, and hypercapnia may be included in the diagnostic criteria. These parameters are also used to determine the need for the initiation or escalation of respiratory support.
However, the use of chest radiographs, pulse oximetry and blood gas analysis are resource intensive and not commonly available in LRS. Hence, the most feasible assessments for RDS in LRS include objective criteria for assessing work of breathing, such as scoring systems that are simple, non-invasive, inexpensive and have shown both prognostic value and good inter-rater-reliability. Most commonly in use are the Downes score and the Silverman Andersen Respiratory Severity Score (19, 20). In one study among nurses trained to use the Silverman Andersen Score, the intra-class correlation coefficient was 0.88 (CI 0.72–0.98) (21). Similarly, in another study among nurses trained to use the Downes score reported an inter rater reliability of 0.71 (22). These scores assess work of breathing specific to the physiology of newborns such as chest wall flexibility and use of accessory respiratory muscles. Components of these scores include exam findings of work of breathing, cyanosis, and degree of tachypnea (Figure 2). Neonates are scored on each component from 0 to 2 and their total score is from 0 to 10, where 10 represents severe distress. Elevated scores correlate to an increased likelihood of requiring advanced respiratory support (23, 24). Silverman Andersen score in the 4–6 range is commonly used as a threshold for initiation, and titration of CPAP therapy given it can be serially repeated at the bedside as a type of vital sign (23, 25).
Figure 2. Scoring systems for respiratory distress syndrome. Downes and Silverman Andersen scores assign 0–2 points for each of five categories of respiratory distress (19, 20). Part of figure reprinted with permission from (25).
In the 1960s, animal studies showed corticosteroids accelerated lung maturation (26). In subsequent human trials, antenatal corticosteroid (ACS) given to the pregnant woman prior to preterm birth improved survival in premature infants primarily by reducing RDS incidence (14). Consequently, ACS became the standard of care in pregnancies between 24 0/7 weeks and 33 6/7 weeks of gestation at risk of delivery within 7 days (27–29). In high-income settings, over 80% of at-risk pregnancies receive ACS (30, 31). However, its use in low resource settings remains low and sporadic (32). Betamethasone is most commonly used in HIC, however, it is not readily available in LRS (33). Hence, WHO has recommended the use of dexamethasone as a substitute to betamethasone (27). Evidence suggests both betamethasone and dexamethasone are equivalent in the effect (34, 35).
Informed by models and strong evidence from both high, and upper middle-income countries that suggested ACS may prevent prematurity-related neonatal mortality (36–38), the National Institutes of Child Health and Human Development sponsored the population-based ACS cluster-randomized trial (39). This trial was conducted in peri-urban and rural settings in geographical clusters in Argentina, Guatemala, India, Kenya, Pakistan and Zambia (39). Women assessed to have signs of preterm labor, preterm premature rupture of membranes, pre-eclampsia or eclampsia, or obstetric hemorrhage were included. The primary outcome was 28-day neonatal mortality among infants less than the 5th percentile for birthweight—a proxy for preterm birth as gestational age could not be reliably determined (39). The proportion of infants with birth weight <5th percentile was 5% (2,362/48,219) and 4% (2,094/51,523) in the intervention and control group, respectively. The results of this trial showed no benefit on mortality in neonates with birth weights <5th percentile, increased risk of mortality in neonates >2,500 g, and an increased incidence of maternal infection (39). Notable variations in the study outcome occurred by study region. Among African sites included in the trial, ACS was associated with increased risk of mortality among neonates with birth weights <5th percentile (39). Further sub-regional analysis to evaluate why better outcomes were observed in some regions and not others, suggested for example, that better obstetric and neonatal care may have been associated with the improved outcomes observed in Guatemala (40).
After this study, the WHO issued guarded recommendations for ACS to be used in health facilities capable of assessing gestational age, diagnosing maternal infection, and providing emergent obstetric and preterm neonatal care, including resuscitation, respiratory, thermal, and nutritional support (27). Subsequently, the Antenatal Corticosteroids for Improving Outcomes in preterm Newborns (ACTION-1) Trial-sponsored by the WHO-randomized pregnant women between 26 0/7 weeks and 33 6/7 weeks of gestation at centers capable of gestational age assessment, who were at risk for preterm birth to either dexamethasone or placebo. This trial showed ACS resulted in a 16% and 12% risk reduction in neonatal mortality and stillbirths (41). To evaluate the impact of ACS on late preterm infants or those >2,500 g, the ACTION-2 trial was conducted in pregnant women between 34 0/7 weeks and 36 6/7 weeks of gestation who were at risk of preterm labor (42). The results from the ACTION-2 trial, showed ACS did not increase the risk of mortality and reduced the need for resuscitation by 62% (42).
Taken together, these trials on ACS among pregnant women in LMICs at risk of preterm delivery indicate that with close monitoring and the provision of neonatal interventions for preterm newborns, ACS has the potential to substantially reduce prematurity-related mortality. However, a significant proportion of deliveries in LMICs still occur in resource limited settings where the quality of care required to reap the benefits of ACS may be unavailable. Research on how to effectively monitor women at risk of preterm delivery, optimize neonatal resuscitation and increase availability of level two newborn care including respiratory support with limited resources as recommended by WHO is required. Also, investments and research into optimizing the referral and safe transportation systems to higher-level facilities for the at-risk mother, would increase access to ACS.
While desirable, in utero transfer of pregnancies at risk for preterm delivery to a center capable of providing high-quality obstetric and neonatal care is not always feasible (43, 44). WHO recommends small or sick newborns receive a timely referral through integrated newborn service pathways with continuity of care, including during transport (45). In high resource countries the practice of inter-healthcare facility transportation of critically ill neonates continues to expand and has evolved into mobile ICUs capable of delivering state-of-the-art critical care outside the NICU, thus maintaining or improving the continuum of care (46). Anticipating the need for transfer early, appropriate preparation for transfer, and ongoing high-quality care during transfer, are the cornerstones of quality neonatal transport systems (44). Maintaining early supportive care is especially important for the treatment of RDS including use of oxygen and CPAP. However, the availability and quality of referral systems in LMICs are limited (47). In a study from Nigeria that examined 411 neonatal transports, no referral information was available upon presentation to the tertiary referral center. In that study, only 4% arrived by ambulance, 0.7% in a transport incubator, and 7% were accompanied by a health professional (48). This pattern is common in most published observational studies from other countries as well (47, 49). Of the few studies from South Africa where medical services are more established, limitations in availability of resources and effective communication between facilities were limitations of the transport systems (46, 50).
Safe and timely inter-facility transport of small and sick newborn infants including those with RDS is critical to maintain the continuum of care from the referring to the referral hospital. National commitments, investments, and research into optimizing and examining the effect of inter-facility transport on neonatal mortality are critical for further improvements in prematurity-related mortality and morbidity.
Initial respiratory support for some premature infants with RDS requires careful bag mask ventilation to establish lung functional residual capacity. In anticipation of a preterm delivery, appropriate preparation includes availability of a working self-inflating bag and appropriately sized facemask, as well as an emergently available provider trained in resuscitation to focus on the newborn. Helping Babies Breath is a low cost, well established program to train providers to perform these key interventions (5, 51, 52). Implementation of this program has been shown to reduce intrapartum-related stillbirths and 1-day neonatal mortality rate (53). Challenges for scale up of HBB, however, include limited time for training, retention of trained staff, and learners subsequently translating simulation skills into consistent behavioral change (54). Research suggests low dose, high frequency refresher strategies are associated with best retention (54). While the HBB program addresses resuscitation for the majority of births globally, the program is not targeted toward care of the preterm infant.
Tertiary facilities in LMIC may have greater capacity for resuscitation of the premature newborn than what is presented in HBB. Where resources allow, use of the Neonatal Resuscitation Program provides additional training for the initial management of preterm newborns, such as emergent endotracheal intubation and continuous positive airway pressure (CPAP) (55).
The Neonatal Resuscitation Program recommends the use of CPAP when respiratory distress persists after initial resuscitation despite establishment of a normal heart rate and spontaneous respiration (55). This use of CPAP shortly after delivery reduces the risk of subsequent intubation, surfactant use, and ventilator days and is a useful therapy where equipment and expertise are available. CPAP use among preterm infants does increase the risk of pneumothorax, so should be used with caution, and where availability of staff and resources to manage this complication are available (56–59).
The primary consequence of RDS (surfactant deficiency) is alveolar collapse and the loss of functional residual capacity. In spontaneously breathing infants with RDS, CPAP provides continuous distending pressure to the airway and lungs (45, 60). This pressure provides the driving force to overcome the elastic, flow-resistive, and inertial resistance of the respiratory system and restore functional residual capacity (61). The continuous pressure applied via a nasal interface enhances lung inflation, decreases work of breathing and is associated with decreased mortality due to RDS (60, 62). CPAP also decreases the risk of chronic lung disease, one of the major sequelae of RDS that often requires the baby receive pulmonary care up to or beyond term-corrected age (63).
CPAP devices can be grouped into two broad categories based on the method of pressure generation. Devices that use an adaptive flip valve located at the nasal interface to generate CPAP are termed variable flow devices (64). Devices that generate pressure by preventing gas egress from the circuit, because of an expiratory limb resistance or a titratable PEEP valve, are termed continuous flow devices (64). One form of continuous flow CPAP device is one where the expiratory limb is submerged in liquid with the depth of insertion coinciding with the pressure in the circuit. These “bubble CPAP” devices result in less failure of CPAP and can be generally made at a lower cost than other forms of CPAP (65). Most CPAP devices available in HIC are expensive, ranging from US$2000 to US$6000—not including the cost of consumables (66, 67).
Evidence from HIC suggest that compared to supplemental oxygen, the use of CPAP is an effective treatment for preterm infants with RDS (68). A 2020 Cochrane systematic review of CPAP vs. supplemental oxygen and the effect on treatment failure and death included five studies and 322 preterm infants with RDS from HICs. In this review, treatment with CPAP significantly lowered the risk of death, or use of mechanical ventilation [typical risk ratio (RR) 0.64, 95% confidence interval (CI) 0.50 to 0.82; typical risk difference (RD) −0.19, 95% CI −0.28 to −0.09] (68).
The cost of CPAP devices, unavailability of consumables and spare parts, maintenance needs, and dependence on electricity have limited the availability and use of commercialized CPAP devices in LMICs (67, 69). To fill this gap, multiple low-cost CPAP devices have emerged ranging from those crafted locally by providers at the bedside, to lower-cost commercialized devices (70–81). In comparison with commercial device available in HIC settings, the improvised devices lack some features raising questions regarding their efficacy and safety (72, 82–84). Specifically, the improvised devices often lack heated humidity relying only ambient humidity. The available tubing in low resource settings for assembly of CPAP are for standard nasal cannula which are of narrower bore than those typically used with commercialized CPAP circuits (85). The nasal cannula interface used are also not specifically designed to transmit pressure from CPAP and may attenuate the delivered pressure (85–87). Despite the lack of available reliable CPAP devices, a systematic review of 21 observational quasi randomized and observational studies using improvised CPAP devices concluded that the introduction of CPAP improved neonatal survival (81). Pooled data from four of the observational studies showed 66% reduction in in-hospital mortality among preterm neonates following introduction of CPAP (odds ratio 0.34, 95% confidence interval 0.14–0.82) (81).
In 2020, UNICEF published a target product profile to align CPAP innovators and other stakeholders to the most important performance and operational characteristics, as well as target pricing to aid in the development of effective and safe CPAP devices that can be scaled (88). Excerpts from this profile are shown in Table 1. Some technical characteristics for optimal CPAP devices include the ability to produce CPAP pressure between 5 and 8 cm H2O, provide humidification, blend oxygen, and have flow capacity of 0–10 L/min. Table 2 compares the characteristics of different categories of CPAP devices available in LMIC to the highest cost devices which fulfill the requirement of UNICEF's CPAP target product profile (89).
Table 1. Features from the UNICEF target product profile for neonatal continuous positive airway pressure for use in low- and middle-income countries (88).
The only published LMIC randomized trial of CPAP among neonates was conducted in Tanzania, in which 48 preterm infants with birth weight >1,000 g with RDS were randomized to receive CPAP via the Pumani device (25 subjects) or oxygen (23 subjects). The study found that survival to hospital discharge in the CPAP and oxygen groups respectively was not statistically significant [68 vs. 47.8%, crude OR 2.3 (95% CI 0.72–7.49)]. However, in the per-protocol analysis a more significant number of subjects in the CPAP group survived to hospital discharge 77.2 vs. 47.8% (crude OR 3.7, 95% CI 1.02–13.47). The study reported no pneumothoraxes, but that bleeding from the nose was more common in the CPAP group (94).
Other available evidence on the benefits of CPAP from LRS include three trials conducted in children who were between 1 month to 5 years of age with diagnoses other than RDS. The first trial conducted in Ghana was a cross over trial between two secondary hospitals and included over 2,000 subjects with clinical signs of respiratory distress. The primary outcome measure was all-cause mortality at 2 weeks after enrollment. The study found that 3% (26/1,021) patients in the CPAP group, and 4% (44/1,160) patients in the control group died [relative risk (RR) of mortality 0.67, 95% CI 0.42–1.08; p = 0.11] (95). The second was conducted in Bangladesh and randomized children with a diagnosis of severe pneumonia and hypoxemia to receive oxygen therapy by either bubble CPAP, standard low-flow nasal cannula (2 L/min), or high-flow nasal cannula (2 L/kg per min up to the maximum of 12 L/min). Significantly fewer children in the bubble CPAP group had treatment failure than in the low-flow oxygen therapy group (relative risk 0.27, 99.7% CI 0.07–0.99; p = 0.0026) (96). The third trial was conducted in Malawi, and 323 children were randomly assigned to oxygen and 321 to bCPAP. The results showed that CPAP was associated with increased risk of mortality compared with oxygen therapy, 53 (17%) of 321 vs. 35 (11%) of 323 (relative risk 1.52; 95% CI 1.02–2.27; p = 0.036) (97).
The lack of standard equipment and high-quality trials have limited access to CPAP among preterm neonates with RDS in LRS. There are also practical barriers to the widespread use of CPAP in LMICs. In a systematic study on facilitators and barriers to implementation of CPAP in LMICs, the authors reported staff shortage and high staff turnover limited the uptake and use of CPAP (76). The study also reported that parents were resistant to the use of CPAP because of local beliefs that oxygen use led to poor outcomes (76).
Supplemental oxygen is a key RDS treatment modality whether used through nasal cannula, CPAP or mechanical ventilation (98). However, oxygen use among neonates in particular can have deleterious effects and current evidence is that titration of fraction of inspired oxygen to achieve saturations as measured by pulse oximetry between 90 and 95% provides the optimal balance between the therapeutic benefits of oxygen and the risk of oxygen toxicity (45). When used in excess, oxygen can cause oxidative injury to a premature baby's lungs, eyes and brain (99). Use of 100% oxygen with premature infants is a major risk factor for the development of retinopathy of prematurity (ROP) which can lead to visual impairment and even blindness among survivors (45). This is especially important in CPAP and mechanical ventilation where all of the baby's inspired gas is from the respiratory circuit and there is no entrainment of room air around a nasal interface or mask. Most low resource facilities treating newborns with CPAP use 100% oxygen because they do not have a source of compressed air to blend with oxygen (73, 74, 100). The well-intentioned use of oxygen therapy to save preterm newborn lives in LRS could lead to an epidemic of ROP-related blindness in sub-Saharan Africa, as already suggested in Latin America, South Africa, India and China (101–104). Methods to ensure safe use of oxygen, with blending of air to optimize the fraction of inspired oxygen are urgently needed (66). Recent WHO guidelines for the care of small and sick newborns recommend retinal exams for preterm infants to detect ROP and efforts to scale up this screening are underway (45, 105). Unfortunately, ophthalmologists trained in these exams are sparsely distributed (106).
Several options exist for the provision of blended oxygen to newborns with varying cost and availability (Table 3). High resource settings and some tertiary facilities in LRS use precision oxygen blenders; however, these are expensive and require high pressure sources of air and oxygen flow. Some medium cost commercialized CPAP devices designed for lower resource settings include on-board air compressors. When used in combination with an oxygen source, these devices can provide flow with oxygen concentrations ranging from 21 to 100% when available (92–94).
To address the remaining gap in safe oxygen therapy, low-cost modalities to blend oxygen and air are in development. One type of device entrains room air into a flow of oxygen via the Bernoulli principle and therefore does not require compressed air (77, 107, 108). Initial studies of these devices are in progress and show promise in their efficacy, portability, cost, and usability. This entrained air mechanism, however, is limited to providing fraction of inspired oxygen in pressurized flow of less than ~30% because of the pressure drop associated with increasing entrainment of room air (107).
An important consideration for provision of blended oxygen is the increased importance it places on pulse oximetry and measurement of patient saturations to guide blending at the bedside. Pulse oximetry is included as a recommendation in the WHO standards for care of the small newborns and is best used continuously to allow frequent detection of low or high saturations and resultant adjustment in oxygen concentration (45). However, oximetry devices are expensive and remain a limitation for safe provision of CPAP and oxygen (74, 111).
In addition to RDS, apnea of prematurity (AOP) commonly affects premature infants. It is defined as cessation of breathing with hypoxia and bradycardia that last more than 15 s (112). The severity and frequency of AOP are inversely related to the degree of prematurity (112, 113). Methylxanthines—aminophylline, theophylline, and caffeine citrate (caffeine)—are the mainstay pharmacologic treatments for AOP used adjunctively with positive pressure ventilation (114). The pharmacological effects of methylxanthine include (i) stimulation of the respiratory center in the medulla; (ii) increased sensitivity to carbon dioxide; (iii) increased skeletal muscle tone; (iv) enhanced diaphragmatic contractility; (v) increased minute ventilation; (vi) increased metabolic rate; and (vii) increased oxygen consumption (115–117). Caffeine and aminophylline are equivalent in reducing events of AOP (118). However, caffeine has a wider therapeutic index, longer half-life that allows once-daily administration, does not require drug-level monitoring, and has a better side effect profile—causing less tachycardia and feeding intolerance (118, 119). Furthermore, compared to placebo, caffeine shortens ventilator days, reduces the risk of developing bronchopulmonary dysplasia, improves neurodevelopment at 18–24 months, and is cost-effective (120–123). Furthermore, theophylline therapy has been associated with seizures and hypokalemia in neonates (124).
Caffeine for AOP treatment first appeared in the 2009 WHO essential drug list (125). Despite this, the use of caffeine to treat preterm babies in low resource settings has not achieved scale (126). In a survey of 55 clinicians from 13 countries in sub-Saharan Africa, only six countries used caffeine, and often inconsistently (126). In a review of 11 studies on caffeine for premature infants, the only studies from LMICs were from India (127). The reasons for the unavailability of caffeine in SSA are complex, including high drug prices, stock outs, and the drugs is not obtainable for purchase in some countries (126). Additionally, there may be less demand for caffeine as knowledge of greater safety over aminophylline may not be pervasive. Furthermore, the patient population where the benefits of methylxanthines are greatest (very-to-extreme preterm infants), have very poor survival in LMICs. The availability of overall care for these patients varies greatly between these low resource sites and that in HIC where the caffeine trials were conducted (120, 128).
Exogenous surfactant replacement therapy is the definitive pharmacologic treatment for RDS (129). Used adjunctively with invasive or non-invasive ventilation, the use of surfactant reduces RDS specific neonatal mortality (130). WHO recommends that small and sick neonates be assessed for surfactant deficiency, and treatment provided within 2 h of birth (45). Exogenous surfactant preparations include synthetic surfactants and natural surfactants derived from animal sources. Early trials suggested that natural surfactants are more efficacious than synthetic preparations, with the benefit attributed to the proteins in natural preparations (131). However, synthetic surfactants can be produced at lower cost, and newer synthetic surfactants are being developed and evaluated, showing promise for their equivalency to natural surfactants (132, 133).
Surfactant use is limited in low resource settings; particularly, sub-Saharan Africa (74, 134). In a systematic review on the use of surfactant in LMICs, of 38 relevant studies, none were from Sub-Saharan Africa (SSA) (135). In a survey involving respondents from 49 African countries, surfactant was available in 33% and 39% of the most well-equipped public and private hospitals, respectively (74). Potential explanations for the limited use of surfactant in LMICs includes unavailability, cost, and the perception that surfactant therapy must occur in conjunction with mechanical ventilation (MV) which may not be available or feasible (74). In the survey of African respondents, the cost of a vial of surfactant varied from <US$200 to over US$500.
Traditional surfactant replacement therapy involves instillation via endotracheal tube, which requires significant skill on the part of the provider, and adjunctive use of MV. However, given the association of MV with adverse outcomes (136–138), in current neonatal practice, clinicians strive to limit or avoid mechanical ventilator use. This change in practice has led to innovative ways to administer exogenous surfactant in minimally invasive ways. Examples of these techniques include dosing surfactant via a thin catheter, a laryngeal mask airway (LMA), or using nebulized or aerosolized surfactant. LMAs, of note, are generally designed for use among neonates >2,000 g, and studies on surfactant instilled via LMA have not included preterm newborns <1,250 g (139).
Evidence from high-income settings indicates that instillation of surfactant through a thin catheter (140, 141) or LMA method (142, 143) can prevent the need for intubation when compared to treatment with continuous positive airway pressure alone. When pooled in a meta-analysis, these minimally invasive techniques (administration via LMA or thin catheter) are associated with a 47% risk reduction in CPAP failure, when compared with CPAP alone (141–144) (Figure 3). Surfactant instillation via thin catheter is included in the 2019 European consensus guidelines on the management of respiratory distress syndrome (145, 146). Data from the German Neonatal Network indicated that over 50% of surfactant replacement therapy occurs with the thin catheter method (147). Results on the efficacy and feasibility of nebulized surfactant is emerging (148). In a recent randomized controlled trial, nebulized surfactant did not differ from CPAP alone in preventing CPAP failure, however with advances in nebulization/aerosolization devices and surfactant formulations, this modality may have promise (149).
Figure 3. Forest plot of comparison of CPAP plus minimally invasive surfactant administration via thin catheter or laryngeal mask airway (LMA) vs. CPAP only. The outcome of interest was CPAP failure as determined by need for intubation. These minimally invasive techniques are associated with a 47% risk reduction in CPAP failure (142–144, 150).
Data from a survey conducted among 49 African countries on the availability of neonatal respiratory care showed that 11% (4/35) of NICUs capable of providing surfactant replacement therapy did so with the thin catheter method (74). There are no randomized trials originating from a LMIC on the use of minimally invasive surfactant administration. The only available study from Sub Saharan Africa is an observational study from Asaba, Nigeria that compared mortality among preterm infants with respiratory distress treated with CPAP who were administered surfactant via thin catheter compared with those who did not receive surfactant. Eligibility included parent's ability to pay for the surfactant, resulting in only 25% (n = 51) of neonates to be able to receive the medication. The study reported a significant reduction in mortality with surfactant administration only among neonates with birth weight below 28 weeks and 1 kg birthweight [50% (13/26) vs. 65% (35/54)] (151). Further studies on less invasive strategies for administration of exogenous surfactant in LRS are urgently needed.
Oxygen therapy with CPAP for RDS is the most prevalent form of respiratory support in LMIC (74, 100). However, in a systematic review that included eight observational studies from LMIC on the use of CPAP and enrolling patients of varied degrees of prematurity, 20–40% of infants with RDS failed CPAP treatment in the absence of surfactant therapy (81). These patients failing CPAP require surfactant administration and mechanical ventilation (MV) for improved survival. In a survey on available respiratory support modalities for RDS from Africa, of the 49 countries with at least one respondent, only 49% of the most well-equipped government hospitals and 59% of the most well-equipped private hospitals located in capital cities used MV (74). A survey from Nigeria suggests sparse availability and lack of capacity to use MV devices where available (152, 153). We speculate that the findings of this survey can be generalized to other LMICs. Most global recommendations and predictive models of interventions to improve prematurity related mortality in LMICs do not include MV, only CPAP and there is a need to elucidate the residual mortality from RDS which could be averted with MV (45, 154).
The cost of MV and lack of capacity to use MV are deterrents to its use (74). MV is not a standalone intervention; medications like surfactant, caffeine, and antenatal steroids improve outcomes of neonates managed on MV. Furthermore, the use of blended oxygen, oximetry, arterial blood gas, mobile chest x-rays, respiratory therapists, neonatal nurses, biomedical technicians, and pharmacologic support are critical for safe and effective use of MV but are limited in LMIC. MV is currently not taught and not incorporated into undergraduate training of medical and nursing schools in several LMICs (74, 155). Furthermore, postgraduate clinical exposure and training on MV is limited; hence knowledge of MV among the essential workforce is deficient. Data on MV's safety and efficacy in LMIC is currently lacking; this area needs further exploration.
During the COVID-19 pandemic, the lack of, and need for MV generated awareness of its necessity in LMICs among patient populations outside of the neonatal period (156–158). The pandemic also sparked the production of more basic, compact, and affordable MV devices by academic institutions and industry (159–161). The increased access to, and availability of MV in some centers may increase its use in newborn respiratory support which should be done with caution.
Adequate nutrition is critical for the continued growth and development of the premature respiratory system. Premature infants are at risk of insensitive water losses and nutritional failure by virtue of their dermal and intestinal immaturity. Liberal or excessive fluid administration is associated with poor respiratory outcomes in preterm infants (162), and optimal early nutrition is correlated with a better pulmonary outcome (163). Hence careful consideration of fluid management, and optimizing nutrition in premature infants is essential to RDS management.
To achieve the sustainable developmental goals targets, small and sick newborns need high-quality inpatient care at the right time and in the right place (8). Transformative RDS therapies, which are standard of care in high resource settings (HRS), significantly reduce prematurity-related mortality. However, 40–60 years after these interventions were proven effective, about 2.4 million small and sick newborns born in LMICs will die this year because of lack of access. The resource limitations in LMICs that affect medication and equipment availability and the ancillary care required to reap their benefits are poor or non-existent. Consequently, our knowledge on the impact of these RDS-specific interventions in LRS is limited. Important questions that remain include:
1. how women at risk of preterm labor in rural communities can be treated safely with ACS and have the desired pregnancy outcome?
2. how can low-cost CPAP devices be optimized to be as effective and safe as the gold standard devices?
3. how can providers learn and maintain direct laryngoscopy skills and the complexities of MV?
4. how can the cost of essential medications like surfactant, caffeine, and devices like CPAP and MV be made more affordable, so they are accessible to providers in LMICs?
To bridge this knowledge gap, thoughtful research designs are required. Research needs to focus equally on implementation as well as evidence generation, taking into context the resource limitations of the clinical setting (164). These type of research studies will allow accurate determination of the extent to which timely and effective neonatal transport, thermoregulation, nutritional support, hypoglycemia management, infection control, and provider-to-patient ratios affect the benefits of these evidence-based RDS-specific therapies. Also, the use of telemedicine proved beneficial during the COVID-19 pandemic where face to face physician patient interaction was limited. Indeed, telemedicine has been used to provide simulation training effectively and feasibly for neonatal resuscitation skills (165, 166). Opportunities to leverage telemedicine in overcoming some of the highlighted barriers is an area for further exploration.
Most global recommendations and predictive models of interventions to improve prematurity-related mortality in LMICs indicate that the greatest benefit will only be achieved with a comprehensive approach to implementing these evidence-based interventions (45, 154). Critical to the impact of any RDS-specific interventions are the core elements of neonatal care including thermoregulation, KMC, safe and adequate transportation to facilities capable of providing high-quality care, infection management, fluid and nutritional support, and workforce availability and capacity. Meeting the ENAP coverage target for care of small and sick newborns in facilities that can provide basic RDS-specific management like optimal resuscitation and CPAP in 80% districts will require considerable investment. The target will be more attainable with further development of low cost, safe and reliable RDS-specific drugs and devices; broader availability consumable parts, standardization of training, guidelines for optimal and safe use, in addition to adequate staffing to provide this level of care (7, 75, 167). To accelerate the development of solutions essential to make the global coverage target feasible, governments may consider providing incentives for local and international biomedical companies to enable them to safely and effectively produce and market their products locally. These incentives could include tax exemptions, streamlined in-country product registration and evaluation by regulatory bodies.
Respiratory distress syndrome is a major driver of prematurity related neonatal mortality. High quality trials conducted in HRS have shown interventions to be effective in reducing the RDS specific neonatal mortality. However, these standard of care interventions in HRS have sparse and sporadic coverage in LRS. When tested in LRS these interventions have not consistently had the same beneficial effect, likely due to the lack of critical ancillary services and core neonatal care practices. To achieve a neonatal mortality rate as low as 12 per 1,000 live births in year 2030 (8), guided by high quality implementation and effectiveness research, considerable scale up of RDS-specific interventions bundled with core neonatal care practices are needed.
OE, MB, and AH conceptualized the review. Initial draft by OE, IO, and AH with revisions by MB. All authors contributed to the article and approved the submitted version.
The authors declare that the research was conducted in the absence of any commercial or financial relationships that could be construed as a potential conflict of interest.
The handling Editor declared a shared committee with one of the authors MB.
All claims expressed in this article are solely those of the authors and do not necessarily represent those of their affiliated organizations, or those of the publisher, the editors and the reviewers. Any product that may be evaluated in this article, or claim that may be made by its manufacturer, is not guaranteed or endorsed by the publisher.
1. United Nations Inter-agency Group for Child Mortality Estimation (UN IGME). Levels & Trends in Child Mortality Report 2020. New York: United Nations Children's Fund (2020).
2. Perin J, Mulick A, Yeung D, Villavicencio F, Lopez G, Strong KL, et al. Global, regional, and national causes of under-5 mortality in 2000-19: an updated systematic analysis with implications for the Sustainable Development Goals. Lancet Child Adolesc Health. (2021) 6:106–15. doi: 10.1016/S2352-4642(21)00311-4
3. World Health Organization. Newborns: Improving Survival and Well-Being. Geneva: World Health Organization (2019). Available online at: https://www.who.int/news-room/fact-sheets/detail/newborns-reducing-mortality (accessed May 30, 2022).
4. United Nations. Transforming Our World: The 2030 Agenda for Sustainable Development A/RES/70/1. United Nations (2015).
5. World Health Organization UNICEF. Every Newborn Progress Report. WHO (2019). Available online at: https://www.who.int/initiatives#:~:text=Every%20Newborn%20Action%20Plan
6. Blencowe H, Cousens S, Oestergaard MZ, Chou D, Moller AB, Narwal R, et al. National, regional, and worldwide estimates of preterm birth rates in the year 2010 with time trends since 1990 for selected countries: a systematic analysis and implications. Lancet. (2012) 379:2162–72. doi: 10.1016/S0140-6736(12)60820-4
7. UNICEF World Health Organization. Ending Preventable Newborn Deaths and Stillbirths by 2030. Geneva: UNICEF (2020). Available online at: https://www.unicef.org/reports/ending-preventable-newborn-deaths-stillbirths-quality-health-coverage-2020-2025
8. World Health Organization. Survive and Thrive: Transforming Care for Every Small and Sick Newborn. Geneva: World Health Organization (2018). Available online at: https://apps.who.int/iris/bitstream/handle/10665/276655/WHO-FWC-MCA-18.11-eng.pdf?ua=1
9. Muhe LM, McClure EM, Nigussie AK, Mekasha A, Worku B, Worku A, et al. Major causes of death in preterm infants in selected hospitals in Ethiopia (SIP): a prospective, cross-sectional, observational study. Lancet Global Health. (2019) 7:e1130–e8. doi: 10.1016/S2214-109X(19)30220-7
10. Nkadi PO, Merritt TA, Pillers DA. An overview of pulmonary surfactant in the neonate: genetics, metabolism, and the role of surfactant in health and disease. Mol Genet Metab. (2009) 97:95–101. doi: 10.1016/j.ymgme.2009.01.015
11. Martin R, Fanaroff AA, Walsh M. Fanaroff and Martin's Neonatal-Perinatal Medicine. Amsterdam: Elsevier (2019) 1159–73.
12. Anadkat JS, Kuzniewicz MW, Chaudhari BP, Cole FS, Hamvas A. Increased risk for respiratory distress among white, male, late preterm and term infants. J Perinatol. (2012) 32:780–5. doi: 10.1038/jp.2011.191
13. Jain L. Respiratory morbidity in late-preterm infants: prevention is better than cure! Am J Perinatol. (2008) 25:75–8. doi: 10.1055/s-2007-1022471
14. McGoldrick E, Stewart F, Parker R, Dalziel SR. Antenatal corticosteroids for accelerating fetal lung maturation for women at risk of preterm birth. Cochrane Database Syst Rev. (2020) 12:Cd004454. doi: 10.1002/14651858.CD004454.pub4
15. Fanaroff AA, Stoll BJ, Wright LL, Carlo WA, Ehrenkranz RA, Stark AR, et al. Trends in neonatal morbidity and mortality for very low birthweight infants. Am J Obstet Gynecol. (2007) 196:147.e1–8. doi: 10.1016/j.ajog.2006.09.014
16. Stoll BJ, Hansen NI, Bell EF, Shankaran S, Laptook AR, Walsh MC, et al. Neonatal outcomes of extremely preterm infants from the NICHD Neonatal Research Network. Pediatrics. (2010) 126:443–56. doi: 10.1542/peds.2009-2959
17. Warren J, Anderson J. Core concepts respiratory distress syndrome. Neoreviews. (2009) 1:e351–61. doi: 10.1542/neo.10-7-e351
18. Jobe A. Surfactant for respiratory distress syndrome. Neoreviews. (2014) 1:e236–45. doi: 10.1542/neo.15-6-e236
19. Downes JJ, Vidyasagar D, Boggs TR. Jr., Morrow 3rd GM. Respiratory distress syndrome of newborn infants. I. New clinical scoring system (RDS score) with acid-base and blood-gas correlations. Clin Pediatr. (1970) 9:325–31. doi: 10.1177/000992287000900607
20. Silverman WA, Andersen DH. A controlled clinical trial of effects of water mist on obstructive respiratory signs, death rate and necropsy findings among premature infants. Pediatrics. (1956) 17:1–10.
21. Hedstrom AB, Faino AV, Batra M. The Silverman Andersen respiratory severity score in the delivery room predicts subsequent intubation in very preterm neonates. Acta Paediatr. (2020) 110:1450–1. doi: 10.1111/apa.15671
22. Shashidhar A, Suman Rao P, Jose J. Downes score vs Silverman Anderson score for assessment of respiratory distress in preterm newborns. Pediatric Oncall. (2016) 13:66–8. doi: 10.7199/ped.oncall.2016.30
23. Tagare A, Kadam S, Vaidya U, Pandit A, Patole S. Bubble CPAP versus ventilator CPAP in preterm neonates with early onset respiratory distress—a randomized controlled trial. J Trop Pediatr. (2013) 59:113–9. doi: 10.1093/tropej/fms061
24. Hedstrom AB, Gove NE, Mayock DE, Batra M. Performance of the Silverman Andersen Respiratory Severity Score in predicting PCO2 and respiratory support in newborns: a prospective cohort study. J Perinatol. (2018) 38:505–11. doi: 10.1038/s41372-018-0049-3
25. McAdams RM, Hedstrom AB, DiBlasi RM, Mant JE, Nyonyintono J, Otai CD, et al. Implementation of bubble CPAP in a rural Ugandan Neonatal ICU. Respir Care. (2015) 60:437–45. doi: 10.4187/respcare.03438
26. Liggins GC. Premature delivery of foetal lambs infused with glucocorticoids. J Endocrinol. (1969) 45:515–23. doi: 10.1677/joe.0.0450515
27. World Health Organization. WHO Recommendations on Interventions to Improve Preterm Birth Outcomes. Report No.: 9789241508988. Geneva: World Health Organization (2015).
28. Committee Opinion No. 713: Antenatal corticosteroid therapy for fetal maturation. Obstet Gynecol. (2017) 130:e102–9. doi: 10.1097/AOG.0000000000002237
29. Norman J, Shennan A, Jacobsson B, Stock SJ. FIGO good practice recommendations on the use of prenatal corticosteroids to improve outcomes and minimize harm in babies born preterm. Int J Gynaecol Obstet. (2021) 155:26–30. doi: 10.1002/ijgo.13836
30. Carlo WA, McDonald SA, Fanaroff AA, Vohr BR, Stoll BJ, Ehrenkranz RA, et al. Association of antenatal corticosteroids with mortality and neurodevelopmental outcomes among infants born at 22 to 25 weeks' gestation. JAMA. (2011) 306:2348–58. doi: 10.1001/jama.2011.1752
31. Norman M, Piedvache A, Børch K, Huusom LD, Bonamy AE, Howell EA, et al. Association of short antenatal corticosteroid administration-to-birth intervals with survival and morbidity among very preterm infants: results from the EPICE cohort. JAMA Pediatr. (2017) 171:678–86. doi: 10.1001/jamapediatrics.2017.0602
32. Jobe AH, Kemp MW, Kamath-Rayne B, Schmidt AF. Antenatal corticosteroids for low and middle income countries. Semin Perinatol. (2019) 43:241–6. doi: 10.1053/j.semperi.2019.03.012
33. Jobe AH, Kemp M, Schmidt A, Takahashi T, Newnham J, Milad M. Antenatal corticosteroids: a reappraisal of the drug formulation and dose. Pediatr Res. (2021) 89:318–25. doi: 10.1038/s41390-020-01249-w
34. Crowther CA, Ashwood P, Andersen CC, Middleton PF, Tran T, Doyle LW, et al. Maternal intramuscular dexamethasone versus betamethasone before preterm birth (ASTEROID): a multicentre, double-blind, randomised controlled trial. Lancet Child Adolesc Health. (2019) 3:769–80. doi: 10.1016/S2352-4642(19)30292-5
35. Brownfoot FC, Gagliardi DI, Bain E, Middleton P, Crowther CA. Different corticosteroids and regimens for accelerating fetal lung maturation for women at risk of preterm birth. Cochrane Database Syst Rev. (2013) 2013:Cd006764. doi: 10.1002/14651858.CD006764.pub3
36. Vogel JP, Souza JP, Gülmezoglu AM, Mori R, Lumbiganon P, Qureshi Z, et al. Use of antenatal corticosteroids and tocolytic drugs in preterm births in 29 countries: an analysis of the WHO Multicountry Survey on Maternal and Newborn Health. Lancet. (2014) 384:1869–77. doi: 10.1016/S0140-6736(14)60580-8
37. Roberts D, Dalziel S. Antenatal corticosteroids for accelerating fetal lung maturation for women at risk of preterm birth. Cochrane Database Syst Rev. (2006) 2006:Cd004454. doi: 10.1002/14651858.CD004454.pub2
38. Bhutta ZA, Das JK, Bahl R, Lawn JE, Salam RA, Paul VK, et al. Can available interventions end preventable deaths in mothers, newborn babies, and stillbirths, and at what cost? Lancet. (2014) 384:347–70. doi: 10.1016/S0140-6736(14)60792-3
39. Althabe F, Belizan JM, McClure EM, Hemingway-Foday J, Berrueta M, Mazzoni A, et al. A population-based, multifaceted strategy to implement antenatal corticosteroid treatment versus standard care for the reduction of neonatal mortality due to preterm birth in low-income and middle-income countries: the ACT cluster-randomised trial. Lancet. (2015) 385:629–39. doi: 10.1016/S0140-6736(14)61651-2
40. Garces A, McClure EM, Figueroa L, Pineda S, Hambidge KM, Krebs NF, et al. A multi-faceted intervention including antenatal corticosteroids to reduce neonatal mortality associated with preterm birth: a case study from the Guatemalan Western Highlands. Reprod Health. (2016) 13:63. doi: 10.1186/s12978-016-0178-0
41. Oladapo OT, Vogel JP, Piaggio G, Nguyen MH, Althabe F, Gülmezoglu AM, et al. Antenatal dexamethasone for early preterm birth in low-resource countries. N Engl J Med. (2020) 383:2514–25. doi: 10.1056/NEJMoa2022398
42. WHO Action Trials Collaborators. Antenatal dexamethasone for late preterm birth: a multi-centre, two-arm, parallel, double-blind, placebo-controlled, randomized trial. EClinicalMedicine. (2022) 44:101285. doi: 10.1016/j.eclinm.2022.101285
43. Gage AD, Carnes F, Blossom J, Aluvaala J, Amatya A, Mahat K, et al. In low- and middle-income countries, is delivery in high-quality obstetric facilities geographically feasible? Health Aff (Millwood). (2019) 38:1576–84. doi: 10.1377/hlthaff.2018.05397
44. Fowlie PW, Booth P, Skeoch CH. Moving the preterm infant. BMJ. (2004) 329:904–6. doi: 10.1136/bmj.329.7471.904
45. World Health Organization. Standards for Improving the Quality of Care for Small and Sick Newborns in Health Facilities. Geneva: World Health Organization (2020).
46. Ashokcoomar P, Bhagwan R. The neonatal transfer process through the lens of neonatologists at public hospitals in South Africa. Health SA. (2022) 27:1617. doi: 10.4102/hsag.v27i0.1617
47. Niermeyer S, Domek JD. Neonatal Transport in Developing Country Settings: A Systematic Review. Montevideo: Pan American Health Organization (2016).
48. Abdulraheem MA, Tongo OO, Orimadegun AE, Akinbami OF. Neonatal transport practices in Ibadan, Nigeria. Pan Afr Med J. (2016) 24:216. doi: 10.11604/pamj.2016.24.216.8651
49. Okonkwo I, Abhulimhen-Iyoha B, Okolo A. Newborn transport practices: influence on newborn survival in <city>Benin City</city>,Nigeria. Am J Pediatrics. (2020) 6:346–52. doi: 10.11648/j.ajp.20200603.39
50. Ashokcoomar P, Naidoo R. An analysis of inter-healthcare facility transfer of neonates within the eThekwini Health District of KwaZulu-Natal, South Africa. S Afr Med J. (2016) 106:514–8. doi: 10.7196/SAMJ.2016.v106i5.8554
51. Kamath-Rayne BD, Thukral A, Visick MK, Schoen E, Amick E, Deorari A, et al. Helping babies breathe, second edition: a model for strengthening educational programs to increase global newborn survival. Glob Health Sci Pract. (2018) 6:538–51. doi: 10.9745/GHSP-D-18-00147
52. World Health Organization. Essential Newborn Care Course—Interim Version of Second Edition. Geneva: World Health Organization (2022). Available online at: https://www.who.int/tools/essential-newborn-care-training-course (accessed May 30, 2022).
53. Versantvoort JMD, Kleinhout MY, Ockhuijsen HDL, Bloemenkamp K, de Vries WB, van den Hoogen A. Helping babies breathe and its effects on intrapartum-related stillbirths and neonatal mortality in low-resource settings: a systematic review. Arch Dis Child. (2020) 105:127–33. doi: 10.1136/archdischild-2018-316319
54. Morris SM, Fratt EM, Rodriguez J, Ruman A, Wibecan L, Nelson BD. Implementation of the helping babies breathe training program: a systematic review. Pediatrics. (2020) 146:e20193938. doi: 10.1542/peds.2019-3938
55. American Academy of Pediatrics. Textbook of neonatal resuscitation, 8th Edition. 6th ed. In: Weiner G, Zaichkin J, editors. Elk Grove Village, IL: American Academy of Pediatrics (2021). xiii, 328 p. 61.
56. Dunn MS, Kaempf J, de Klerk A, de Klerk R, Reilly M, Howard D, et al. Randomized trial comparing 3 approaches to the initial respiratory management of preterm neonates. Pediatrics. (2011) 128:e1069–76. doi: 10.1542/peds.2010-3848
57. Morley CJ, Davis PG, Doyle LW, Brion LP, Hascoet JM, Carlin JB. Nasal CPAP or intubation at birth for very preterm infants. N Engl J Med. (2008) 358:700–8. doi: 10.1056/NEJMoa072788
58. Smithhart W, Wyckoff MH, Kapadia V, Jaleel M, Kakkilaya V, Brown LS, et al. Delivery room continuous positive airway pressure and pneumothorax. Pediatrics. (2019) 144:1–8. doi: 10.1542/peds.2019-0756
59. Eunice Kennedy Shriver NICHDN, Carlo WA, Walsh MC, Rich W, Gantz MG, et al. Early CPAP versus surfactant in extremely preterm infants. N Engl J Med. (2010) 362:1970–9. doi: 10.1056/NEJMoa0911783
60. Subramaniam P, Ho JJ, Davis PG. Prophylactic or very early initiation of continuous positive airway pressure (CPAP) for preterm infants. Cochrane Database Syst Rev. (2021) 10:CD001243. doi: 10.1002/14651858.CD001243.pub4
61. Gupta S, Donn SM. Continuous positive airway pressure: physiology and comparison of devices. Semin Fetal Neonatal Med. (2016) 21:204–11. doi: 10.1016/j.siny.2016.02.009
62. Lam R, Schilling D, Scottoline B, Platteau A, Niederhausen M, Lund KC, et al. The effect of extended continuous positive airway pressure on changes in lung volumes in stable premature infants: a randomized controlled trial. J Pediatr. (2020) 217:66–72 e1. doi: 10.1016/j.jpeds.2019.07.074
63. Abiramalatha T, Ramaswamy VV, Bandyopadhyay T, Somanath SH, Shaik NB, Pullattayil AK, et al. Interventions to prevent bronchopulmonary dysplasia in preterm neonates: an umbrella review of systematic reviews and meta-analyses. JAMA Pediatr. (2022) 176:502–16. doi: 10.1001/jamapediatrics.2021.6619
64. Courtney SE, Barrington KJ. Continuous positive airway pressure and noninvasive ventilation. Clin Perinatol. (2007) 34:73–92, vi. doi: 10.1016/j.clp.2006.12.008
65. Bharadwaj SK, Alonazi A, Banfield L, Dutta S, Mukerji A. Bubble versus other continuous positive airway pressure forms: a systematic review and meta-analysis. Arch Dis Child Fetal Neonatal Ed. (2020) 105:526–31. doi: 10.1136/archdischild-2019-318165
66. Jensen EA, Chaudhary A, Bhutta ZA, Kirpalani H. Non-invasive respiratory support for infants in low- and middle-income countries. Semin Fetal Neonatal Med. (2016) 21:181–8. doi: 10.1016/j.siny.2016.02.003
67. Ekhaguere OA, Mairami AB, Kirpalani H. Risk and benefits of bubble continuous positive airway pressure for neonatal and childhood respiratory diseases in low- and middle-income countries. Paediatr Respir Rev. (2019) 29:31–6. doi: 10.1016/j.prrv.2018.04.004
68. Ho JJ, Subramaniam P, Davis PG. Continuous positive airway pressure (CPAP) for respiratory distress in preterm infants. Cochrane Database Syst Rev. (2020) 10:CD002271. doi: 10.1002/14651858.CD002975.pub2
69. Dewez JE, van den Broek N. Continuous positive airway pressure (CPAP) to treat respiratory distress in newborns in low- and middle-income countries. Trop Doct. (2017) 47:19–22. doi: 10.1177/0049475516630210
70. MTTS. Dolphin CPAP (2017). Available online at: http://www.mtts-asia.com/dolphin-cpap/ (accessed May 30, 2022).
71. Phoenix Medical Systems (P) Ltd. NCPAP 300 Chennai, India (2020). Available online at: https://www.phoenixmedicalsystems.com/infant-care/respiratory/nasal-cpap-unit-300/ (accessed May 30, 2022).
72. Kasali B, Gururaj A, Batra M. Newborn care technology investments for LMIC settings: a CPAP approach. BMJ Innov. (2021) 7:519–22. doi: 10.1136/bmjinnov-2020-000598
73. Okonkwo IR, Aneji C, Ekhaguere OA, Eyo-Ita EU, Okolo AA. Cost implication of CPAP use in low resource settings, surmounting the oxygen administration challenge. J Matern Fetal Neonatal Med. (2021) 2021:1–7. doi: 10.1080/14767058.2021.1949278
74. Tooke L, Ehret DEY, Okolo A, Dlamini-Nqeketo S, Joolay Y, Minto'o S, et al. Limited resources restrict the provision of adequate neonatal respiratory care in the countries of Africa. Acta Paediatr. (2021) 111:275–83. doi: 10.1111/apa.16050
75. Dada S, Ashworth H, Sobitschka A, Raguveer V, Sharma R, Hamilton RL, et al. Experiences with implementation of continuous positive airway pressure for neonates and infants in low-resource settings: a scoping review. PLoS ONE. (2021) 16:e0252718. doi: 10.1371/journal.pone.0252718
76. Kinshella MW, Walker CR, Hiwa T, Vidler M, Nyondo-Mipando AL, Dube Q, et al. Barriers and facilitators to implementing bubble CPAP to improve neonatal health in sub-Saharan Africa: a systematic review. Public Health Rev. (2020) 41:6. doi: 10.1186/s40985-020-00124-7
77. Coffey PS, Wollen A. Nonclinical bench performance testing of a very low-cost nonelectric bubble continuous positive airway pressure (bCPAP) and blenders device designed for newborn respiratory support. Med Dev Evid Res. (2022) 15:187–97. doi: 10.2147/MDER.S318218
78. Dundek ML, Ng EK, DiBlasi RM, Poli JA, Burke TF. Evaluation of a bubble cpap system for low resource settings. Respir Care. (2021) 66:1572–81. doi: 10.4187/respcare.08948
79. Aneji C, Hartman T, Olutunde O, Okonkwo I, Ewumwen E, Adetiloye O, et al. Implementing bubble continuous positive airway pressure in a lower middle-income country: a Nigerian experience. Pan Afr Med J. (2020) 37:10. doi: 10.11604/pamj.2020.37.10.24911
80. Kawaza K, Machen HE, Brown J, Mwanza Z, Iniguez S, Gest A, et al. Efficacy of a low-cost bubble CPAP system in treatment of respiratory distress in a neonatal ward in Malawi. PLoS ONE. (2014) 9:e86327. doi: 10.1371/journal.pone.0086327
81. Thukral A, Sankar MJ, Chandrasekaran A, Agarwal R, Paul VK. Efficacy and safety of CPAP in low- and middle-income countries. J Perinatol. (2016) 36 Suppl 1:S21–8. doi: 10.1038/jp.2016.29
82. Wilson PT. Bubble CPAP in resource-poor settings: friend or foe? Lancet Respir Med. (2019) 7:923–5. doi: 10.1016/S2213-2600(19)30261-9
83. World Health Organization. Oxygen Therapy for Children: A Manual for Health Workers. Geneva, Switzerland: WHO (2016).
84. Ettinger NA, Serazin N, Nguyen R, Werdenberg J, Huibers M, Torrey S. Testing positive pressure delivered from commercial and WHO-style pediatric bubble CPAP devices. BMC Pediatr. (2021) 21:1–10. doi: 10.1186/s12887-021-03006-2
85. Baldursdottir S, Falk M, Donaldsson S, Jonsson B, Drevhammar T. Basic principles of neonatal bubble CPAP: effects on CPAP delivery and imposed work of breathing when altering the original design. Arch Dis Child Fetal Neonatal Ed. (2020) 105:550–4. doi: 10.1136/archdischild-2019-318073
86. Bailes SA, Firestone KS, Dunn DK, McNinch NL, Brown MF, Volsko TA. Evaluating the effect of flow and interface type on pressures delivered with bubble CPAP in a simulated model. Respir Care. (2016) 61:333–9. doi: 10.4187/respcare.04251
87. Volsko TA, Fedor K, Amadei J, Chatburn RL. High flow through a nasal cannula and CPAP effect in a simulated infant model. Respir Care. (2011) 56:1893–900. doi: 10.4187/respcare.01204
88. UNICEF NEST360. Target Product Profile CPAP- Respiratory Support Chicago (2020). Available online at: https://www.unicef.org/supply/media/2896/file/CPAP-TPP.pdf (accessed May 30, 2022).
89. Fisher Paykel Healthcare. Bubble CPAP System Panmure, New Zealand (2022). Available online at: https://www.fphcare.com/us/hospital/infant-respiratory/cpap/bubble-cpap-system/ (accessed May 30, 2022).
90. Bennett DJ, Carroll RW, Kacmarek RM. Evaluation of a low-cost bubble CPAP system designed for resource-limited settings. Respir Care. (2018) 63:395–403. doi: 10.4187/respcare.05762
91. Carns J, Kawaza K, Liaghati-Mobarhan S, Asibon A, Quinn MK, Chalira A, et al. Neonatal CPAP for respiratory distress across Malawi and mortality. Pediatrics. (2019) 144:e20190668. doi: 10.1542/peds.2019-0668
92. Diamedica (UK) Limited. Diamedica Baby CPAP England (2015). Available online at: http://www.diamedica.co.uk/english/product_details.cfm?id=2697 (accessed May 30, 2022).
93. Amadi HO, Okonkwo IR, Abioye IO, Abubakar AL, Olateju EK, Adesina CT, et al. A new low-cost commercial bubble CPAP (bCPAP) machine compared with a traditional bCPAP device in Nigeria. Paediatr Int Child Health. (2019) 39:184–92. doi: 10.1080/20469047.2019.1598125
94. Mwatha AB, Mahande M, Olomi R, John B, Philemon R. Treatment outcomes of Pumani bubble-CPAP versus oxygen therapy among preterm babies presenting with respiratory distress at a tertiary hospital in Tanzania-Randomised trial. PLoS ONE. (2020) 15:e0235031. doi: 10.1371/journal.pone.0235031
95. Wilson PT, Baiden F, Brooks JC, Morris MC, Giessler K, Punguyire D, et al. Continuous positive airway pressure for children with undifferentiated respiratory distress in Ghana: an open-label, cluster, crossover trial. Lancet Global Health. (2017) 5:e615–e23. doi: 10.1016/S2214-109X(17)30145-6
96. Chisti MJ, Salam MA, Smith JH, Ahmed T, Pietroni MAC, Shahunja KM, et al. Bubble continuous positive airway pressure for children with severe pneumonia and hypoxaemia in Bangladesh: AN open, randomised controlled trial. Lancet. (2015) 386:1057–65. doi: 10.1016/S0140-6736(15)60249-5
97. McCollum ED, Mvalo T, Eckerle M, Smith AG, Kondowe D, Makonokaya D, et al. Bubble continuous positive airway pressure for children with high-risk conditions and severe pneumonia in Malawi: an open label, randomised, controlled trial. Lancet Respir Med. (2019) 7:964–74. doi: 10.1016/S2213-2600(19)30243-7
98. World Health Organization (WHO). Model List of Essential Medicines for Children-−8th List. Geneva: World Health Organization (2021).
99. Perez M, Robbins ME, Revhaug C, Saugstad OD. Oxygen radical disease in the newborn, revisited: oxidative stress and disease in the newborn period. Free Radic Biol Med. (2019) 142:61–72. doi: 10.1016/j.freeradbiomed.2019.03.035
100. Herrod SK, Stevenson A, Vaucher YE, Lambert SR, Isenberg SJ, Yap VL, et al. Oxygen management among infants in neonatal units in sub-Saharan Africa: a cross-sectional survey. J Perinatol. (2021) 41:2631–8. doi: 10.1038/s41372-021-01040-7
101. Vinekar A, Dogra M, Azad RV, Gilbert C, Gopal L, Trese M. The changing scenario of retinopathy of prematurity in middle and low income countries: unique solutions for unique problems. Indian J Ophthalmol. (2019) 67:717–9. doi: 10.4103/ijo.IJO_496_19
102. Blencowe H, Moxon S, Gilbert C. Update on blindness due to retinopathy of prematurity globally and in India. Indian Pediatr. (2016) 53 Suppl 2:S89–92.
103. Limburg H, Gilbert C, Hon DN, Dung NC, Hoang TH. Prevalence and causes of blindness in children in Vietnam. Ophthalmology. (2012) 119:355–61. doi: 10.1016/j.ophtha.2011.07.037
104. Visser Kift E, Freeman N, Cook C, Myer L. Retinopathy of prematurity screening criteria and workload implications at Tygerberg Children's Hospital, South Africa: a cross-sectional study. S Afr Med J. (2016) 106:10358. doi: 10.7196/SAMJ.2016.v106i6.10358
105. Ademola-Popoola DS, Fajolu IB, Gilbert C, Olusanya BA, Onakpoya OH, Ezisi CN, et al. Strengthening retinopathy of prematurity screening and treatment services in Nigeria: a case study of activities, challenges and outcomes 2017–2020. BMJ Open Ophthalmol. (2021) 6:e000645. doi: 10.1136/bmjophth-2020-000645
106. Wang D, Duke R, Chan RP, Campbell JP. Retinopathy of prematurity in Africa: a systematic review. Ophthalmic Epidemiol. (2019) 26:223–30. doi: 10.1080/09286586.2019.1585885
107. Mollazadeh-Moghaddam K, Burke TF, Dundek M, Yeung SH, Sharma R, Ravi R, et al. A low-cost venturi ambient air-oxygen blender for neonatal oxygen therapy. Acad J Pediatr Neonatol. (2020) 8:48–56. doi: 10.19080/AJPN.2020.08.555808
108. Floersch J, Hauschildt E, Keester A, Poganski S, Tran K, Slusher T, et al. A low-resource oxygen blender prototype for use in modified bubble CPAP circuits. J Med Dev. (2020) 14:4045899. doi: 10.1115/1.4045899
109. PrecisionMedical. Air-Oxygen Blender. (2022). Available online at: https://www.precisionmedical.com/hospital/blenders/air-oxygen/c-25/c-96/p-1062 (accessed May 30, 2022).
110. Bio-Med Devices. Oxygen Blenders from Bio-Med Devices. (2022). Available online at: https://www.mercurymed.com/product/oxygen-blender/ (accessed May 30, 2022).
111. Simkovich SM, Underhill LJ, Kirby MA, Crocker ME, Goodman D, McCracken JP, et al. Resources and geographic access to care for severe pediatric pneumonia in four resource-limited settings. Am J Respir Crit Care Med. (2022) 205:183–97.
112. Barrington K, Finer N. The natural history of the appearance of apnea of prematurity. Pediatr Res. (1991) 29:372–5. doi: 10.1038/pr.1991.72500
113. Henderson-Smart DJ. The effect of gestational age on the incidence and duration of recurrent apnoea in newborn babies. Aust Paediatr J. (1981) 17:273–6. doi: 10.1111/j.1440-1754.1981.tb01957.x
114. Henderson-Smart DJ, Subramaniam P, Davis PG. Continuous positive airway pressure versus theophylline for apnea in preterm infants. Cochrane Database Syst Rev. (2001) 2001:CD001072. doi: 10.1002/14651858.CD001072
115. Aranda JV, Chemtob S, Laudignon N, Sasyniuk BI. Pharmacologic effects of theophylline in the newborn. J Allergy Clin Immunol. (1986) 78:773–80. doi: 10.1016/0091-6749(86)90060-6
116. Kraaijenga JV, Hutten GJ, de Jongh FH, van Kaam AH. The effect of caffeine on diaphragmatic activity and tidal volume in preterm infants. J Pediatr. (2015) 167:70–5. doi: 10.1016/j.jpeds.2015.04.040
117. Aranda JV, Turmen T, Davis J, Trippenbach T, Grondin D, Zinman R, et al. Effect of caffeine on control of breathing in infantile apnea. J Pediatr. (1983) 103:975–8. doi: 10.1016/S0022-3476(83)80735-5
118. Henderson-Smart DJ, Steer PA. Caffeine versus theophylline for apnea in preterm infants. Cochrane Database Syst Rev. (2010) 2010:Cd000273. doi: 10.1002/14651858.CD000273.pub2
120. Schmidt B, Roberts RS, Davis P, Doyle LW, Barrington KJ, Ohlsson A, et al. Caffeine therapy for apnea of prematurity. N Engl J Med. (2006) 354:2112–21. doi: 10.1056/NEJMoa054065
121. Schmidt B, Roberts RS, Davis P, Doyle LW, Barrington KJ, Ohlsson A, et al. Long-term effects of caffeine therapy for apnea of prematurity. N Engl J Med. (2007) 357:1893–902. doi: 10.1056/NEJMoa073679
122. Mürner-Lavanchy IM, Doyle LW, Schmidt B, Roberts RS, Asztalos EV, Costantini L, et al. Neurobehavioral outcomes 11 years after neonatal caffeine therapy for apnea of prematurity. Pediatrics. (2018) 141:e20174047. doi: 10.1542/peds.2017-4047
123. Dukhovny D, Lorch SA, Schmidt B, Doyle LW, Kok JH, Roberts RS, et al. Economic evaluation of caffeine for apnea of prematurity. Pediatrics. (2011) 127:e146–55. doi: 10.1542/peds.2010-1014
124. Hascoet JM, Hamon I, Boutroy MJ. Risks and benefits of therapies for apnoea in premature infants. Drug Saf. (2000) 23:363–79. doi: 10.2165/00002018-200023050-00002
125. WHO. Model List of Essential Medicines (2009). Available online at: http://apps.who.int/iris/bitstream/handle/10665/70642/a95055_eng.pdf?sequence=1 (accessed May 30, 2022).
126. Ekhaguere OA, Ayede AI, Ezeaka CV. Is caffeine available and affordable in low and middle-income countries? A survey in sub-Saharan Africa. Semin Fetal Neonatal Med. (2020) 25:101182. doi: 10.1016/j.siny.2020.101182
127. Nabwera HM, Ekhaguere OA, Kirpalani H, Burgoine K, Ezeaka CV, Otieno W, et al. Caffeine for the care of preterm infants in sub-Saharan Africa: a missed opportunity? BMJ Glob Health. (2021) 6:e007682. doi: 10.1136/bmjgh-2021-007682
128. Alhersh E, Abushanab D, Al-Shaibi S, Al-Badriyeh D. Caffeine for the treatment of apnea in the neonatal intensive care unit: a systematic overview of meta-analyses. Paediatr Drugs. (2020) 22:399–408. doi: 10.1007/s40272-020-00404-4
129. Engle WA. Surfactant-replacement therapy for respiratory distress in the preterm and term neonate. Pediatrics. (2008) 121:419–32. doi: 10.1542/peds.2007-3283
130. Schwartz RM, Luby AM, Scanlon JW, Kellogg RJ. Effect of surfactant on morbidity, mortality, and resource use in newborn infants weighing 500 to 1500 g. N Engl J Med. (1994) 330:1476–80. doi: 10.1056/NEJM199405263302102
131. Ardell S, Pfister RH, Soll R. Animal derived surfactant extract versus protein free synthetic surfactant for the prevention and treatment of respiratory distress syndrome. Cochrane Database Syst Rev. (2015) 2015:Cd000144. doi: 10.1002/14651858.CD000144.pub3
132. Ramanathan R, Biniwale M, Sekar K, Hanna N, Golombek S, Bhatia J, et al. Synthetic surfactant CHF5633 compared with poractant alfa in the treatment of neonatal respiratory distress syndrome: a multicenter, double-blind, randomized, controlled clinical trial. J Pediatr. (2020) 225:90–6.e1. doi: 10.1016/j.jpeds.2020.06.024
133. Jeon GW. Surfactant preparations for preterm infants with respiratory distress syndrome: past, present, and future. Korean J Pediatr. (2019) 62:155–61. doi: 10.3345/kjp.2018.07185
134. Okonkwo IR, Okolo AA. The scope and extent of exogenous surfactant utilization in Nigerian health care facilities: benefits of its regular use to outcome of premature babies. J Matern Fetal Neonatal Med. (2020) 33:1276–81.
135. Sankar MJ, Gupta N, Jain K, Agarwal R, Paul VK. Efficacy and safety of surfactant replacement therapy for preterm neonates with respiratory distress syndrome in low- and middle-income countries: a systematic review. J Perinatol. (2016) 36 Suppl 1:S36–48. doi: 10.1038/jp.2016.31
136. Thébaud B, Goss KN, Laughon M, Whitsett JA, Abman SH, Steinhorn RH, et al. Bronchopulmonary dysplasia. Nat Rev Dis Primers. (2019) 5:78. doi: 10.1038/s41572-019-0127-7
137. Jensen EA, DeMauro SB, Kornhauser M, Aghai ZH, Greenspan JS, Dysart KC. Effects of multiple ventilation courses and duration of mechanical ventilation on respiratory outcomes in extremely low-birth-weight infants. JAMA Pediatr. (2015) 169:1011–7. doi: 10.1001/jamapediatrics.2015.2401
138. Higgins RD, Jobe AH, Koso-Thomas M, Bancalari E, Viscardi RM, Hartert TV, et al. Bronchopulmonary dysplasia: executive summary of a workshop. J Pediatr. (2018) 197:300–8. doi: 10.1016/j.jpeds.2018.01.043
139. Calevo MG, Veronese N, Cavallin F, Paola C, Micaglio M, Trevisanuto D. Supraglottic airway devices for surfactant treatment: systematic review and meta-analysis. J Perinatol. (2018) 39:173–83. doi: 10.1038/s41372-018-0281-x
140. Cao ZL, Pan JJ, Shen X, Zhou XY, Cheng R, Zhou XG, et al. Less invasive surfactant administration in preterm infants with respiratory distress syndrome-an updated meta-analysis. J Chin Med Assoc. (2020) 83:170–9. doi: 10.1097/JCMA.0000000000000228
141. Dargaville PA, Kamlin COF, Orsini F, Wang X, De Paoli AG, Kanmaz Kutman HG, et al. Effect of minimally invasive surfactant therapy vs sham treatment on death or bronchopulmonary dysplasia in preterm infants with respiratory distress syndrome: the OPTIMIST-a randomized clinical trial. JAMA. (2021) 326:2478–87. doi: 10.1001/jama.2022.2929
142. Roberts KD, Brown R, Lampland AL, Leone TA, Rudser KD, Finer NN, et al. Laryngeal mask airway for surfactant administration in neonates: a randomized, controlled trial. J Pediatr. (2018) 193:40–6.e1. doi: 10.1016/j.jpeds.2017.09.068
143. Attridge JT, Stewart C, Stukenborg GJ, Kattwinkel J. Administration of rescue surfactant by laryngeal mask airway: lessons from a pilot trial. Am J Perinatol. (2013) 30:201–6. doi: 10.1055/s-0032-1323592
144. Göpel W, Kribs A, Ziegler A, Laux R, Hoehn T, Wieg C, et al. Avoidance of mechanical ventilation by surfactant treatment of spontaneously breathing preterm infants (AMV): an open-label, randomised, controlled trial. Lancet. (2011) 378:1627–34. doi: 10.1016/S0140-6736(11)60986-0
145. Sweet DG, Carnielli V, Greisen G, Hallman M, Ozek E, Te Pas A, et al. European consensus guidelines on the management of respiratory distress syndrome-−2019 update. Neonatology. (2019) 115:432–50. doi: 10.1159/000499361
146. Reynolds P, Bustani P, Darby C, Fernandez Alvarez JR, Fox G, Jones S, et al. Less-invasive surfactant administration for neonatal respiratory distress syndrome: a consensus guideline. Neonatology. (2021) 118:586–92. doi: 10.1159/000518396
147. Herting E, Härtel C, Göpel W. Less invasive surfactant administration (LISA): chances and limitations. Arch Dis Child Fetal Neonatal Ed. (2019) 104:F655–f9. doi: 10.1136/archdischild-2018-316557
148. Rong H, Bao Y, Wen Z, Chen X, Chen C, Li F. Nebulized versus invasively delivered surfactant therapy for neonatal respiratory distress syndrome: a systematic review and meta-analysis. Medicine. (2020) 99:e23113. doi: 10.1097/MD.0000000000023113
149. Dani C, Talosi G, Piccinno A, Ginocchio VM, Balla G, Lavizzari A, et al. A randomized, controlled trial to investigate the efficacy of nebulized poractant alfa in premature babies with respiratory distress syndrome. J Pediatr. (2022) 40–47.e5. doi: 10.1016/j.jpeds.2022.02.054
150. Dargaville PA, Kamlin COF, Orsini F, Wang X, De Paoli AG, Kanmaz Kutman HG, et al. Effect of minimally invasive surfactant therapy vs sham treatment on death or bronchopulmonary dysplasia in preterm infants with respiratory distress syndrome: the OPTIMIST—a randomized clinical trial. JAMA. (2021) 326:2478–87.
151. Ajanwaenyi J, Bamidele O, Osim C, Salami O, Umukoro C, Idaboh T, et al. The minimal invasive surfactant therapy: experience from a low resource setting. J Matern Fetal Neonatal Med. (2021) 2021:1–7. doi: 10.1080/14767058.2021.1875438
152. Okonkwo IR, Abhulimhen-Iyoha BI, Okolo AA. Scope of neonatal care services in major Nigerian Hospitals. Niger J Paed. (2016) 43:8–13. doi: 10.4314/njp.v43i1.2
153. Okolo AA, Okonkwo IR, Ideh RC. Challenges and opportunities for neonatal respiratory support in Nigeria: a case for regionalisation of care. Niger J Paediatr. (2016) 43:64–9. doi: 10.4314/njp.v43i2.1
154. Griffin JB, McClure EM, Kamath-Rayne BD, Hepler BM, Rouse DJ, Jobe AH, et al. Interventions to reduce neonatal mortality: a mathematical model to evaluate impact of interventions in sub-Saharan Africa. Acta Paediatr. (2017) 106:1286–95. doi: 10.1111/apa.13853
155. Kamath BD, Macguire ER, McClure EM, Goldenberg RL, Jobe AH. Neonatal mortality from respiratory distress syndrome: lessons for low-resource countries. Pediatrics. (2011) 127:1139–46. doi: 10.1542/peds.2010-3212
156. Ranney ML, Griffeth V, Jha AK. Critical supply shortages—the need for ventilators and personal protective equipment during the COVID-19 pandemic. N Engl J Med. (2020) 382:e41. doi: 10.1056/NEJMp2006141
157. McMahon DE, Peters GA, Ivers LC, Freeman EE. Global resource shortages during COVID-19: Bad news for low-income countries. PLoS Negl Trop Dis. (2020) 14:e0008412. doi: 10.1371/journal.pntd.0008412
158. World Health Organization. COVID-19—WHO Boosts Nigeria's Response to COVID-19, Donates 26 Ventilators and 3560 Pulse Oximeters (2021). Available online at: https://www.afro.who.int/news/covid-19-who-boosts-nigerias-response-covid-19-donates-26-ventilators-and-3560-pulse-oximeters (accessed August 06, 2021).
159. Nachiappan N, Koo JM, Chockalingam N, Scott TE. A low-cost field ventilator: an urgent global need. Health Sci Rep. (2021) 4:e349. doi: 10.1002/hsr2.349
160. Technology Exchange Lab. Ventilators (2022). Available online at: https://www.techxlab.org/solutions/categories/medical/ventilators/ (accessed May 30, 2022).
161. LaChance J, Schottdorf M, Zajdel TJ, Saunders JL, Dvali S, Marshall C, et al. PVP1-The People's Ventilator Project: a fully open, low-cost, pressure-controlled ventilator research platform compatible with adult and pediatric uses. PLoS ONE. (2022) 17:e0266810. doi: 10.1371/journal.pone.0266810
162. Bell EF, Acarregui MJ. Restricted versus liberal water intake for preventing morbidity and mortality in preterm infants. Cochrane Database Syst Rev. (2014) 2014:CD000503. doi: 10.1002/14651858.CD000503.pub3
163. Thiess T, Lauer T, Woesler A, Neusius J, Stehle S, Zimmer KP, et al. Correlation of early nutritional supply and development of bronchopulmonary dysplasia in preterm infants <1,000 g. Front Pediatr. (2021) 9:741365. doi: 10.3389/fped.2021.741365
164. Curran GM, Bauer M, Mittman B, Pyne JM, Stetler C. Effectiveness-implementation hybrid designs: combining elements of clinical effectiveness and implementation research to enhance public health impact. Med Care. (2012) 50:217–26. doi: 10.1097/MLR.0b013e3182408812
165. Portnoy J, Waller M, Elliott T. Telemedicine in the era of COVID-19. J Allergy Clin Immunol Pract. (2020) 8:1489–91. doi: 10.1016/j.jaip.2020.03.008
166. Umoren R, Bucher S, Hippe DS, Ezenwa BN, Fajolu IB, Okwako FM, et al. eHBB: a randomised controlled trial of virtual reality or video for neonatal resuscitation refresher training in healthcare workers in resource-scarce settings. BMJ Open. (2021) 11:e048506. doi: 10.1136/bmjopen-2020-048506
Keywords: respiratory distress syndrome (RDS), low- and middle-income countries, treatment, surfactant, continuous positive airway pressure (CPAP), low resource, prematurity
Citation: Ekhaguere OA, Okonkwo IR, Batra M and Hedstrom AB (2022) Respiratory distress syndrome management in resource limited settings—Current evidence and opportunities in 2022. Front. Pediatr. 10:961509. doi: 10.3389/fped.2022.961509
Received: 04 June 2022; Accepted: 30 June 2022;
Published: 29 July 2022.
Edited by:
Andrew Steenhoff, Children's Hospital of Philadelphia, United StatesReviewed by:
Peter Cooper, University of the Witwatersrand, South AfricaCopyright © 2022 Ekhaguere, Okonkwo, Batra and Hedstrom. This is an open-access article distributed under the terms of the Creative Commons Attribution License (CC BY). The use, distribution or reproduction in other forums is permitted, provided the original author(s) and the copyright owner(s) are credited and that the original publication in this journal is cited, in accordance with accepted academic practice. No use, distribution or reproduction is permitted which does not comply with these terms.
*Correspondence: Anna B. Hedstrom, aGVkc3Ryb21AdXcuZWR1
Disclaimer: All claims expressed in this article are solely those of the authors and do not necessarily represent those of their affiliated organizations, or those of the publisher, the editors and the reviewers. Any product that may be evaluated in this article or claim that may be made by its manufacturer is not guaranteed or endorsed by the publisher.
Research integrity at Frontiers
Learn more about the work of our research integrity team to safeguard the quality of each article we publish.