- Department for Pediatric Cardiology, Children’s Hospital, University of Bonn, Bonn, Germany
Purpose: For Fontan-palliated patients, altitude exposure is still a part of discussion since the extent of hypoxic pulmonary vasoconstriction potentially resulting in decreasing cardiac output (Qc), especially during physical exercise, is still unclear. We investigated the effects of normobaric hypoxia (15.2% O2) simulating 2,500 m above sea level on cardiopulmonary and metabolic parameters and the benefit of daily physical activity (PA) on hypoxic exercise capacity.
Methods: A total of 21 Fontan patients (14–31 years) and 20 healthy controls performed cardiopulmonary exercise tests on a bicycle ergometer in normoxia and hypoxia until subjective exhaustion, measuring capillary lactate (cLa) every 2 min. In between, participants underwent an activity tracking over 5 days with a triaxial accelerometer.
Results: Hypoxic exercise was well tolerated by Fontan patients, and no adverse clinical events were observed. Fontan patients showed reduced physical capacity under both conditions compared to controls (63% normoxia, 62% hypoxia), but the relative impairment due to hypoxia was similar for both (≈10%). Up to workloads of 2 W/kg oxygen uptake (O2) and heart rate (HR) developed similarly in patients and controls. cLa increased faster in relation to workload in Fontan patients, but remained significantly lower at peak workload (normoxia 3.88 ± 1.19 mmol/l vs. 7.05 ± 2.1 mmol/l; hypoxia 4.01 ± 1.12 mmol/l vs. 7.56 ± 1.82 mmol/l). Qc was diminished but could be increased similar to controls. Fontan patients with higher PA levels showed a higher O2peak in hypoxia.
Conclusion: Exercise during short-time artificial altitude exposure seems to be safe for young Fontan patients. Further studies are needed to validate longer exposure under real conditions. O2, HR, and Qc might not be a limiting factor for exercise until workloads of 2 W/kg. Higher daily PA levels might improve physical capacity under altitude conditions.
Introduction
High altitude is defined as an altitude of 2,500–3,500 m above sea level (masl). It results in reduced oxygen concentration and barometric pressure (1). As known from healthy individuals, hypoxia leads to vasoconstriction in the pulmonary arteries with the consequence of a rising pulmonary vascular resistance (PVR) in children and adults (2). For Fontan-palliated patients with a passive pulmonary perfusion due to a missing sub-pulmonary ventricle, a rising PVR was thought to lead to an impairment of pulmonary blood flow (PBF) and as a result low cardiac output (Qc) with limited mechanisms to compensate.
In Germany, every year ∼200 children are born with a complex heart defect, leading to a univentricular circulation (3). In the last four decades, a staged surgery has been established, resulting in Fontan palliation (4). This univentricular circulation currently enables a life expectancy until adulthood (5). But still for Fontan patients, life is different in many aspects. Rising up with a congenital heart defect (CHD) often means limitations in everyday life (6, 7).
Over the past decade, pediatric cardiologists and scientists have increasingly been able to establish that physical activity (PA) does not pose a danger to children with CHDs, but rather improves their physical performance (8–10). On this basis, pediatricians, as well as EMAH cardiologists, are confronted with the question of guidelines regarding all kinds of activities. Skiing, hiking, and mountain biking became popular sports for many families, but we do not yet know for sure whether in general altitude exposure alone or even PA at altitude is safe and feasible for patients with Fontan circulation.
Previous studies investigating the exercise capacity of patients after Fontan palliation under normal conditions at sea level (normoxia) showed a relevant impairment of exercise capacity with ∼60–65% of O2peak of age-matched healthy subjects (11–14). Besides cardiac function, including low Qc or chronotropic incompetence, further reasons for this impairment are multifactorial and include reduction in lung function, skeletal muscle, and endothelial dysfunction (15–18).
Studies by Garcia et al., Staempfli et al., and Takken et al. previously investigated hypoxic exposure to Fontan patients without any adverse events (19–21). Garcia analyzed data from a small group of 11 adolescent and young adult Fontan patients without matched controls, whereas Staempfli included only adult patients with single-ventricle physiology, both with a focus on PBF. Takken recently published data on teenagers and young adults, but all of them focused on peak performances. As endurance activities like hiking or skiing are not mainly absolved at peak physical capacity, this chapter focuses on differences at lower workloads as well as peak performances.
The aim of this study was to add further insights into physiological processes in Fontan patients at hypoxic exposure by investigating the impact of hypoxia on physical capacity and metabolic status, depending on daily PA. We therefore performed a study comparing performance parameters at different workloads in adolescent and young adult Fontan patients with matched healthy controls under normal conditions and in a normobaric hypoxic chamber, simulating an altitude of 2,500 masl (average height of European recreational mountain and skiing areas) and 5 days of activity tracking with a triaxial accelerometer.
Materials and methods
Participants
A total of 21 adolescent and young adult Fontan patients with a variety of underlying heart defects and 20 age, sex, and body mass index (BMI)-matched healthy controls were investigated in this study (for details, refer to Table 1).
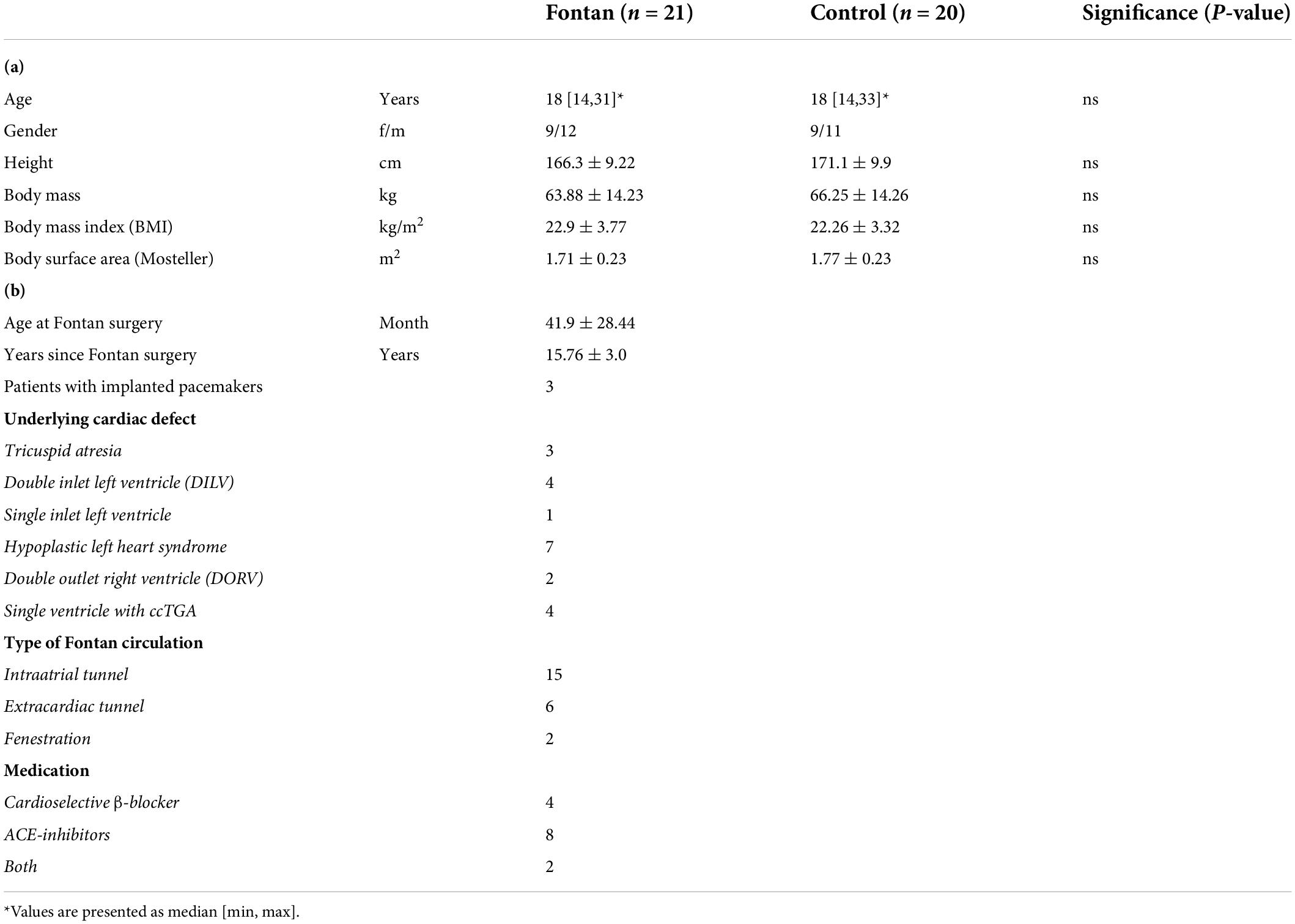
Table 1. (a) Participants’ characteristics; (b) detailed description of patients and underlying congenital heart defects.
We defined the following inclusion criteria for Fontan patients: age ≥ 14 years at the time of investigation, New York Heart Association (NYHA) class I or II, as well as mental and physical ability for cardiopulmonary exercise testing (CPET) on a sitting bicycle ergometer.
Exclusion criteria were failing Fontan [with protein-losing enteropathy (PLE) and plastic bronchitis], O2peak < 45% of predicted values, and pregnancy.
The control group consisted of nonsmokers and noncompetitive athletes without cardiovascular disorders.
Approval was obtained from the local ethics committee of the University Hospital Bonn (application number 335/14), and the study conformed with the Declaration of Helsinki.
All participants/parents gave written informed consent before participating in the study.
The study was performed at the University Children’s Hospital Bonn, and data were collected from May 2018 to October 2018.
Study procedure
Study design
Study participants had to absolve CPETs in normoxia and under hypoxic conditions since the influence of hypoxia should be demonstrated.
Therefore, 2 days of examination were necessary and a period of 2 weeks was retained between the two examination days for safe recovery (for the detailed study protocol, refer also to Figure 1).
Examination days started with baseline evaluation of metric data, blood pressure, 12-lead ECG, and oxygen saturation. For the hypoxic conditions, all participants received a peripheral venous catheter for blood samples [e.g., measurement of hemoglobin (Hb)] and safety reasons. The patients had to fulfill the same protocol on both days in normoxia and under simulated altitude.
For baseline values of physical capacity, all investigations on the first visit were done under normoxic conditions, whereas investigations on the second day were performed in a fully climatized normobaric altitude chamber (Höhenbalance, Going, Austria) with stable temperature conditions between 21°C and 22.5°C. The size of the chamber was 12 m2 and contained ∼37.5 m3 air. Also, 60 m3 air was refreshed every hour. Ambient oxygen was reduced by membrane processes to a level of 15.2% (± 0.2%) and replaced by nitrogen (84.8%) at constant air pressure (1,013 hPa) simulating an altitude of 2,500 masl. When CO2 increased over 0.2%, fresh air was ducted into the altitude chamber. Participants started with 30 min of acclimatization, sitting on a chair after entering the hypoxic environment, to ensure realistic everyday conditions during hiking and skiing with rapid ascent by gondola, starting PA more or less right away. This was followed by the setting up for CPET.
In between the two examination visits, all participants received a triaxial accelerometer that they were encouraged to wear for at least 5 out of that 14-day period to get an overview of the participant’s everyday activity levels (see below).
Exercise testing
CPET was performed on a computer-controlled bicycle ergometer in an upright position (ERG 911 Plus, Ergosana GmbH Schiller, Bitz, Germany) with a breath-by-breath gas exchange analysis, which continuously measured tidal volumes (VT), respiration rate (RR), oxygen uptake (O2), and carbon dioxide production (CO2) (Metamax 3B with MetaSoft Studio Software v. 5.4 for analysis, Cortex Biophysik GmbH, Leipzig, Germany). The highest mean of oxygen uptake of any 30 s interval during exercise was defined as O2peak.
Two different ramp protocols were chosen for either Fontan patients or controls to achieve similar loading times of ∼8–15 min. The end was defined by subjective exhaustion, according to the Borg scale ≥16 and lacking cadence ≥60 rpm. It was followed by a 5 min recovery period before finishing without load (Figure 1). For safety reasons under these special conditions, patients were not pushed further beyond their individual definition of exhaustion.
A continuous 12-lead ECG was monitored for heart rate (HR) and detection of potential arrhythmias (Custo Diagnostics, V. 5.4, Custo med GmbH, Ottobrunn, Germany). Blood pressure (BPsys, BPdia) was measured every 2 min (Tango M2, SunTech Medical Inc., Morrisville, United States). Transcutaneous oxygen saturation (SpO2) was continuously displayed by a pulse oximeter (V100, GE Healthcare, Chicago, United States). Capillary lactate probes (cLa) were taken from the earlobe and analyzed by enzymatic-amperometric measurement (LabTrend, BST Bio Sensor Technology GmbH, Berlin, Germany) within 2 h.
Impedance cardiography
Stroke volume (SV) and Qc were constantly recorded using the noninvasive thoracic impedance monitor PhysioFlow (Manatec Biomedical, Poissy, France), which was evaluated for patients with CHDs by Legendre et al. (22) and Ebrahim et al. (23). It was connected via Bluetooth to MetaSoft Studio Software. With still uncertain data regarding Fontan patients, we used this method to compare the two conditions (hypoxia and normoxia) in order to gain further clarity. The method was already used for this patient group in different studies (20, 24). As most patients have a history of veno-venous collaterals and knowing that this method can be influenced by many factors, especially shunt connections, we mainly focused on trends. Shunt volume was not quantified in more detail, and correlations with other parameters were not made.
Activity tracking
Between both examination visits, participants received a triaxial accelerometer, Actigraph wGT3x-BT (Actigraph LLC., Pensacola, United States), which inter alia was validated for reliable data by Lee et al. (25) and Chu et al. (26). The accelerometer was worn for at least 5 days, including one weekend day. The detailed analysis process was recently described (27).
Briefly, activity data with a sampling rate of 30 Hz were included when a minimum wear time of 330 min per day at five different days was reached.
Analysis was performed using the ActiLife 6 software (V.6.13.3, Actigraph LLC, Pensacole, United States). Different activity intensity categories were built by analyzing counts per minute with an epoch length of 10 s using the cutoff points by Freedson et al. and moderate or higher intensities were summarized as moderate-to-vigorous PA (MVPA) (28).
Statistics
Statistical analysis was performed with GraphPad Prism (v.9.2.0, GraphPad Software, San Diego, CA, United States).
First, variables were tested for normal distribution using D’Agostino and Pearson normality test.
For investigation of normal distributed patients’ characteristics, Student’s unpaired t-test was performed.
To detect differences at rest and peak exercise under different conditions (normoxia vs. hypoxia) within and between groups, values were tested with one-way repeated-measures analysis of variance (ANOVA) and for post hoc analysis Tukey’s multiple comparison test was used.
Parameters at specific workloads between Fontan patients and controls and development of parameters within groups were compared using two-way ANOVA, followed again by Tukey’s multiple comparison test.
Correlation analysis between parameters was done with simple linear regression. To measure the correlation strength, Pearson’s correlation coefficient was applied.
Quantitative data are presented in mean ± standard deviation, unless otherwise described.
Level of significance was defined for p-values ≤ 0.05 (two-sided).
Results
Clinical outcome
All Fontan patients and matched controls could manage hypoxic conditions and exercise testing without any adverse events. During recovery in normoxia, one Fontan patient with known Kent bundle had an atrioventricular reentry tachycardia, requiring adenosine therapy.
One patient could not participate in the normoxia examinations due to time constraints, but was included in the calculations of the hypoxia results. The rest of the participants finished CPET under both conditions.
All relevant parameters at rest and peak exercise are listed in Table 2.
Resting state
Significant differences at rest between normoxia and hypoxia in Fontan patients were only seen for SpO2 (Table 2).
In simulated altitude, the mean of SpO2 was 5 points lower than in normoxia. This decrease was also observed in controls even though the absolute SpO2 level was significantly higher under both conditions in this group. Furthermore, Fontan patients had significantly higher Hb.
HR in hypoxia was reduced in both groups compared to values in normoxia even though this effect was not statistically significant. A difference between Fontan patients and controls could not be seen neither in normoxia nor hypoxia.
Tidal volume increased under hypoxic conditions in both groups, while respiratory rate was similar and differences between Fontan patients and controls were not detected. Nevertheless, Fontan patients showed reduced respiration efficiency since E/CO2 was significantly increased compared to controls.
O2 and O2/kg were increased in hypoxia, even though the effect was not statistically significant. Values for Fontan patients and controls were similar.
SV measured by impedance cardiography was significantly reduced in Fontan patients under both conditions compared to controls, with no difference within the Fontan group in normoxia and hypoxia.
In none of the groups, baseline capillary lactate was increased after exposure to hypoxia.
Peak exercise
Fontan patients achieved a peak workload (Ppeak) of 1.8 Watts/kilo bodyweight (W/kg) in normoxia compared to 1.6 W/kg in hypoxia. Under hypoxic conditions, O2peak decreased by ∼10% compared to normoxic values, corresponding to workloads. In contrast, controls showed a similar decrease (p = 0.453).
SpO2peak was significantly lower in Fontan patients in normoxia and hypoxia with a comparable decline on a higher level in controls.
Comparable to the resting state, E/CO2 was higher in the Fontan group, again showing a less efficient gas exchange also under peak exercise. The maximal oxygen pulse (O2/HR) did not vary within groups but differed significantly between Fontan patients and controls.
Arteriovenous oxygen difference (a-v)DO2 estimated by impedance cardiography did not show differences neither at maximum exhaustion in the Fontan group nor between groups, even though Fontan patients showed a trend for increasing (a-v)DO2 values in hypoxia.
SVpeak and Qcpeak showed reduced values compared to controls. Hypoxia did not lead to impaired Qcpeak in both groups. Furthermore, hypoxic exercise led to reduced SVpeak and Qcpeak in controls. For this, a statistically significant difference to the Fontan group could not be found [p(SV) = 0.6064, p(Qc)> 0.9999].
Lactate levels were significantly lower in Fontan patients at O2peak with an incipient hyperacidity in the blood gas analysis mainly in the control group but also in Fontan patients, corresponding with a higher decline in base excess (BE).
Submaximal exercise
To achieve a better comparability of selected data, development of parameters during increasing workloads in W/kg, which is common for pediatric patients, is presented in Figure 2.
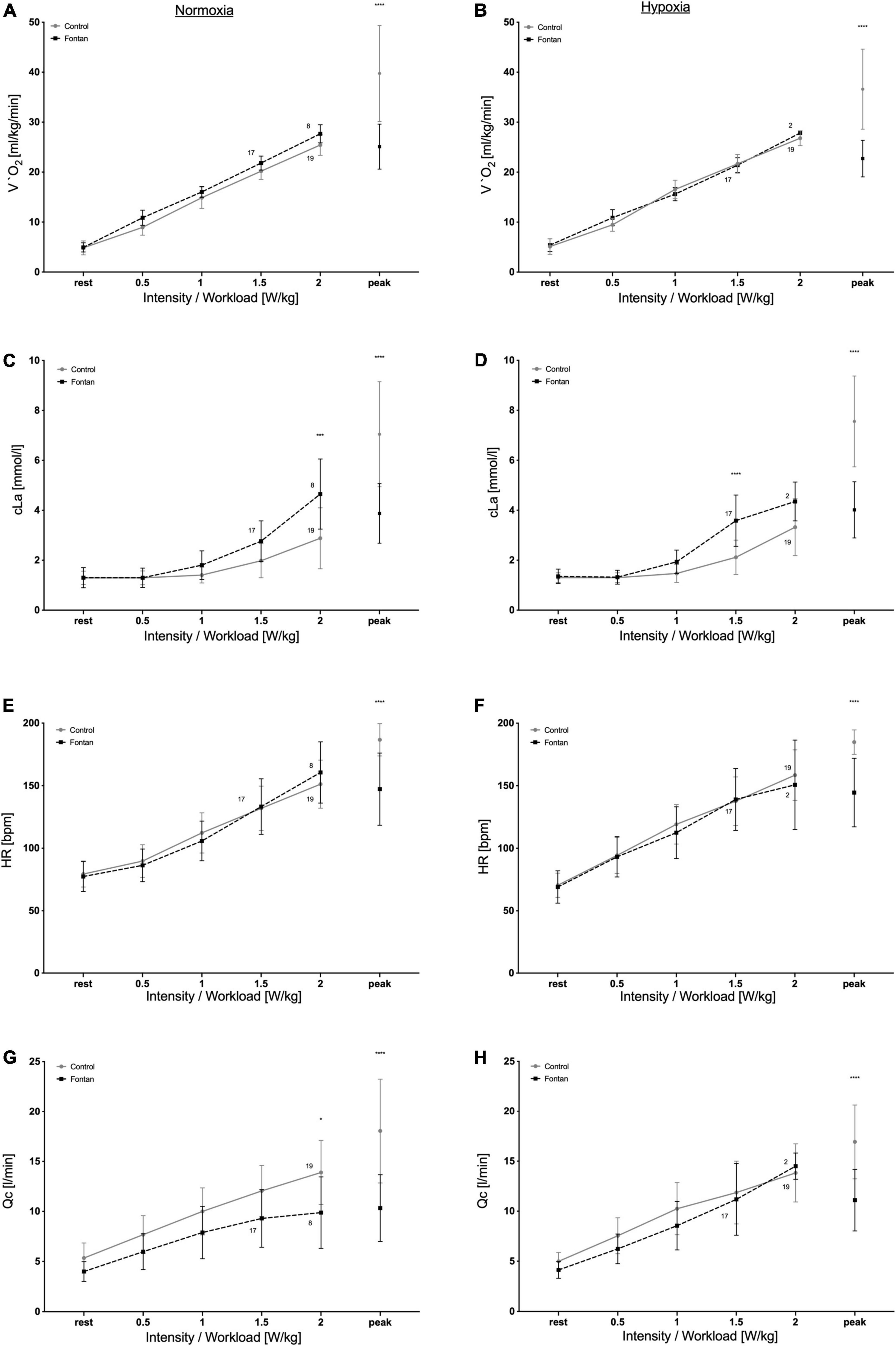
Figure 2. Development of cardiopulmonary and metabolic parameters during exercise in normoxia (left side) and hypoxia (right side). Fontan patients showed similar development of parameters except cLa, which already increased at lower workloads. Numbers show numbers of participants reaching the different workloads. (A,B) Development of O2, (C,D) development of cLa, (E,F) development of HR, and (G,H) development of Qc. Asterisks represent significance level: *p < 0.05; ***p < 0.001; ****p < 0.0001.
Only a minority of the Fontan patients reached workloads of 2 W/kg (8/21 in normoxia respective 2/21 in hypoxia).
O2 showed a similar increase until 2 W/kg (Figures 2A,B), with even slightly higher values in Fontan patients at similar workloads in normoxia, whereas in hypoxia values showed no differences. Correspondingly, HR increased parallel to O2 with no difference between groups, except for HRpeak.
In contrast, cLa showed a steeper increase already at workloads of 1 W/kg compared to controls, even though O2 was similar (Figures 2C,D) under both conditions. While in normoxia, this difference was significant for values at workloads of 2 W/kg, in hypoxia a statistically significant difference was already seen for 1.5 W/kg.
Additionally, Qc showed a trend for reduced values in the patient group, even though the difference was not significant (Figures 2G,H). Interestingly, these developments were mainly seen in normoxia, whereas under hypoxic conditions Qc especially for workloads of 1.5 and 2 W/kg was similar.
Moderate-to-vigorous physical activity and hypoxic physical capacity
A total of 18 Fontan patients and 16 controls fulfilled the criteria for analysis with sufficient wear time.
Differences in MVPA levels between Fontan patients and controls were not found, but both groups showed alarmingly low activity levels overall. With only 27% of Fontan teenagers and 33% of control teenagers, only a small proportion of this group reached the current WHO activity recommendations of 60 min MVPA/day, as recently shown (27).
In addition, a positive significant correlation could be demonstrated for MVPA with O2peak as well as for MVPA with Ppeak in hypoxia in Fontan patients (Figure 3).
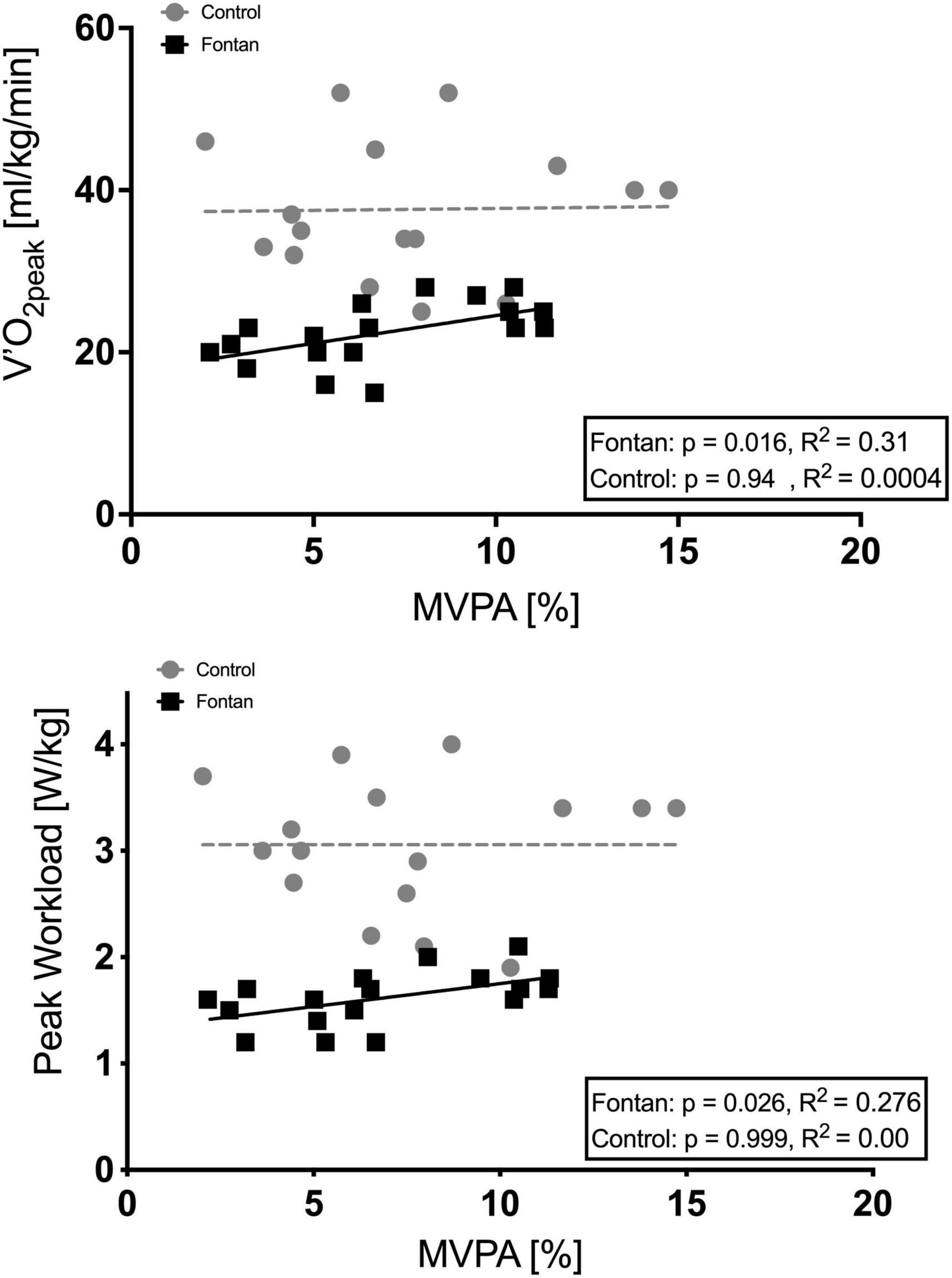
Figure 3. Correlation of MVPA (as percentage of time spent in MVPA) during everyday life with O2peak and MVPA with Ppeak in hypoxia. A significant correlation was detected in Fontan patients.
Discussion
To the best of our knowledge, this is the first study showing the development of cardiopulmonary and metabolic parameters over different workloads during hypoxic exercise in Fontan patients and matched controls and thus complements important information to previous studies.
The Fontan circulation depends on a passive pulmonary perfusion since a sub-pulmonary ventricle is lacking. Therefore, factors increasing PVR such as hypoxic vasoconstriction are expected to be dangerous for these patients or at least have a further negative impact on physical capacity.
Compared to controlsO2peak and Ppeak in normoxia were impaired in Fontan patients, which is in line with previous findings (8, 13). With 63% of the control value for O2peak/kg in normoxia, respectively, 62% in hypoxia physical capacity stayed equal, so that the loss of physical capacity induced by hypoxia was similar between the groups. In Fontan patients and controls, O2peak decreased (10% in Fontan patients, 7.6% in controls) with no significant difference in effect size. In previous studies, a loss of 1% of O2peak was shown for every additional 100 m at altitudes higher than 1,500 masl for healthy individuals, resulting in an expected loss of O2peak of 10% at an altitude of 2,500 masl (29, 30). Consequently, hypoxia had a similar impact on this parameter in Fontan patients with no higher limitations, even though peak performance in Fontan patients was impaired. This result is compatible with the results of Staempfli et al. and Takken et al. but in contrast to these studies we could not demonstrate a significantly higher impact of hypoxia on controls (19, 20).
Furthermore, none of the patients showed any clinical symptoms during hypoxia exposure, even though SpO2 was significantly reduced at all-time points. The decline at SpO2peak was comparable between both groups under both conditions, which was also shown by Staempfli et al. (19) and Takken et al. (20).
Short maximal hypoxic exercise at altitudes of 2,500 masl therefore seems to be well tolerated by these patients. This finding is underlined by the fact that hypoxic pulmonary vasoconstriction (PVC) resulting in increasing PVR, which was expected to lead to reduced preload and disproportionally impairment in physical capacity, could not be seen.
This finding is also underlined by an increase in SV and Qc in both groups in normoxia and hypoxia (Table 2). As described earlier, this data obtained by impedance cardiography must be considered critically as shunt connections in particular can have a considerable influence on the method. As this study was intended to provide a general statement for Fontan patients, shunt connections were deliberately not an exclusion criterion. Thus, the absolute values of SV and Qc may be subject to greater deviations, while the trend development certainly allows for reliable information.
Furthermore, cLa did not increase at rest after 30 min of acclimatization and setting up for CPET in hypoxia and showed significant lower peak values in Fontan patients under both conditions, which might be explained by sufficient hypoxia tolerance as well as further cardiopulmonary capacities. We assume that O2max was not reached by most participants, primarily because of muscular exhaustion of the legs, which was the main reason for termination of CPET. This might also explain the demonstrated low absolute cLapeak, especially in Fontan patients, since expectable cLapeak at O2max is reported to reach values of > 8–10 mmol/L (31). Corresponding to cLapeak, pH and BE were significantly reduced in controls compared to Fontan patients, which could also be explained by higher peak exercise in controls. Factors such as uncertainty in the unfamiliar environment and a lack of body awareness may play an additional role here. Many patients are not used to regular sport activities and show a low PA level so that they might not be used to the feeling of physical exhaustion (32).
In addition, physical exercise usually leads to an increase in (a-v)DO2 in healthy individuals since more oxygen is used for energy supply in the muscles. In our study, absolute (a-v)DO2 was lower compared to previously reported ranges from Ogawa et al. for healthy individuals under both conditions (33) with similar values for Fontan patients and controls. Again, the low (a-v)DO2 could be explained by a lack of objective cardiopulmonary exhaustion, but since values show similar results in normoxia and hypoxia in Fontan patients, an adequate oxygen supply at the point of subjective exhaustion under hypoxic conditions can be postulated. In the study of Takken et al. Fontan patients also showed reduced (a-v)DO2, but overall values were greater in his cohort, which could be explained by higher peak power. Furthermore, Takken described a decline under hypoxia, which is also in contrast to our findings (20), but in both cases (no difference vs. a decline compared to normoxia) the consequence is similar: oxygen supply is sufficient even under hypoxic conditions and low SpO2 with no rise in (a-v)DO2. Consequently, it can be speculated that Fontan patients are adapted to lower SpO2, which might be explained by significantly higher Hb, which is common and could also be proven in our collective (Table 2). Here, too, the influence of the potentially present shunt connections on the method must be taken into account since the (a-v) DO2 is also determined by impedance cardiography.
Previous altitude studies with Fontan patients only describe and compare parameters at peak exercise, but comparability to healthy controls is therefore only possible to a limited extent since Fontan patients only reach significantly lower Ppeak (19–21). As skiing and hiking are popular sports for adolescents and young adults, it becomes an issue for Fontan patients as well as they want to be a recognized member of their peer groups and catch up with their classmates. Because these leisure activities are predominantly in the aerobic range, the focus of the evaluation was placed on the areas of light and moderate PA. Hence, this study demonstrates important insights into the development of cardiopulmonary and metabolic parameters below O2peak (Figure 2).
Exposition to acute hypoxia leads to reduced oxygen supply in the lungs, resulting in hypoxemia with the consequence of increasing ventilation, HR, and Qc within minutes (34). Interestingly, in this study we found a trend of reduced HR and respiratory rate in hypoxia, which could be explained by our study protocol, since all participants started examinations in normoxia and therefore might have been more agitated, as described above.
After starting CPET, important exercise parameters, such as O2, HR, and Qc, showed similar increases and the repeatedly discussed chronotropic incompetence could not be demonstrated up to workloads of 2.0 W/kg. This is in line with the findings by Claessen et al. and Hedlund et al., who also demonstrated regular HR response and speculated that reduced HRpeak in Fontan patients might be an expression of a physiological reaction to maintain SV and Qc within the context of Frank–Starling mechanism, because of reduced preload (35, 36). In addition, due to the numerous operations, many Fontan patients have a lack of responsiveness to modulation by the autonomic nervous system and an impaired sinus node function, both with the consequence of a limited HR variability and HR reserve (13, 37, 38). Here, similar to previous studies, Fontan patients showed significantly lower HRpeak compared to controls, but these values were measured at significant lower Ppeak (39). Therefore, a statement to reduced preload resulting in impaired HRpeak is not possible because exercise response to increasing workloads was adequate for study participants.
As performance limitations of Fontan patients are still a part of discussion (40, 41), this study demonstrated the good ability of cardiopulmonary adaptation to both exercise and hypoxia in these patients with no differences to controls. A reason for the significant earlier termination of CPETs could not be explained by cardiopulmonary parameters, except cLa.
Even though O2 was similar for workloads until 2.0 W/kg, cLa increased earlier according to workloads in the Fontan group than in healthy subjects, but stayed significantly lower at Ppeak in normoxia as well as in hypoxia (Figure 2). A possible explanation might be the differences in muscle metabolism, muscle blood flow, and/or muscle fiber composition compared to controls, complemented by significantly reduced SpO2. Cordina et al. demonstrated reduced skeletal muscle mass and a poorer aerobic muscle capacity because of impaired phosphocreatine resynthesis (18). Furthermore, Turquetto et al. reported decreased muscle volume and blunted blood supply (42). All explanations could lead to reduced exercise tolerance with increased anaerobic energy consumption.
Anyhow, the earlier termination at significantly lower cLapeak could be due to lower training status and poorer tolerance of muscle soreness during increasing lactate levels since previous studies showed lower levels of PA than recommended for the majority of Fontan patients and reduced participation in sports club activities, as mentioned above (32, 43). In addition, Fontan patients often avoid activities in vigorous intensity because of concerns about cardiopulmonary tolerance, arrhythmic events or restrictions imposed by parents and doctors, as shown by Rhodes et al. (44). In this study, we did not find significant differences between MVPA in Fontan patients and controls, but in both groups MVPA was alarmingly low and a positive correlation was demonstrated for MVPA with exercise parameters such as O2peak or with cLa (27). In addition, we could demonstrate that the frequency of MVPA during everyday life activities has a positive impact on hypoxic exercise tolerance in Fontan patients. This is certainly an important finding, which should be considered when counseling Fontan patients, especially when it comes to active stays in the mountains. These results become even more important because recently published studies claim reduced activity levels during the corona pandemic, especially in children with CHD (45). A promotion for regular PA and a regular training should therefore be implemented in a multimodal concept for long-term care of Fontan patients to maintain and improve exercise capacity, quality of life, and survival.
Consequently, since the development of important cardiopulmonary parameters like Qc, HR, and O2 during hypoxic exercise is similar in Fontan patients and healthy controls and even short but exhausting exercise at 15.2% O2 is well-tolerated, the induced hypoxia does not seem to cause a disproportionate increase in PVC, leading to a relevant reduction in PBF. The continuous, non-pulsatile blood flow in pulmonary arteries might result in endothelial dysfunction and/or vascular remodeling, with lack of PVC as already discussed by Turquetto et al. (42) and Kodama et al. (43). Nevertheless, further studies are needed to investigate PBF and pulmonary artery pressure (PAP) under hypoxic conditions since all the demonstrated results only measure PBF indirectly.
Conclusion
In conclusion, normobaric hypoxia (15.2% O2) combined with short-term exercise is well-tolerated by “healthy” Fontan patients. Although peak exercise is diminished, altitude conditions have a similar impact on physical capacity compared to controls. Important exercise parameters such as O2, HR, SV, or Qc indicate a comparable development in both groups until workloads of 2.0 W/kg and consequently reasons for exercise limitations could not be demonstrated for cardiopulmonary parameters. Nevertheless, cLa showed a significant earlier increase, which might be explained by impairments in skeletal musculature and/or poor training status. Physicians and parents should therefore promote PA since higher MVPA levels even during everyday life might be important to maintain and improve physical capacity also for exercise at altitude, quality of life, and long-term survival.
Limitations
In this study as well as in previous studies by Staempfli et al., Takken et al., and Garcia et al. only the effects of acute hypoxic exercise were tested. Recommendations for trips with overnight stays at altitude therefore cannot be derived and need further investigation, especially since respiration while sleeping cannot be controlled and might result in dangerous desaturation.
The small sample size with restriction to Fontan patients with NYHA class I–II does not allow a general advice for all Fontan patients. Underlying CHD leading to a Fontan circulation varies vast and also health status is very different between patients. Therefore, individualized investigations for altitude tolerance should be recommended before altitude exposure.
The majority of Fontan patients quit CPET because of muscle exhaustion, and therefore might not have reached their individual maximal physical capacity. Nevertheless, values of Ppeak and O2peak as well as HRpeak are in line with previous studies.
PBF and PVC were only measured indirectly by Qc or O2. Therefore, measurement of PAP would be essential for a better understanding of PVC and the adaptation to hypoxia.
Due to the study design, all participants had to start with the examination in normoxia, which might have led to differences in some of the investigated parameters due to excitement and uncertainty. Nevertheless, during exercise this effect becomes less important.
This study was performed in a normobaric altitude chamber where effects might be different. Millet et al. demonstrated different physical reactions for normobaric and hypobaric hypoxia (46). Still, normobaric hypoxia is used in many studies because it represents a good approximation to reality and enables a high level of safety for the patients. In real altitude, factors like humidity and temperature fluctuations have to be considered, but the impact of these issues was not the topic of investigation and might play an additional role during stays in the mountains.
Data availability statement
The datasets presented in this article are not readily available because the ethical approval and the informed consent of the participants and the parents in this study do not cover any transfer of raw sensitive data to external sites. Therefore, the sensitive data supporting the findings of this study are only available on reasonable request from the institutional study nurse of the Department for Paediatric Cardiology of the University Hospital Bonn, who is in charge for data administration. Requests to access the datasets should be directed to Caroline von dem Bussche, Q2Fyb2xpbmUudm9uX2RlbV9CdXNzY2hlQHVrYm9ubg==.
Ethics statement
The studies involving human participants were reviewed and approved by the Local Ethics Committee of the University Hospital Bonn (application number: 335/14). Written informed consent to participate in this study was provided by the patients/participants’ legal guardian.
Author contributions
NM, JH, UH, and JB: conceptualization. NM, TJ, and JH: formal analysis. NM, UH, TJ, and JH: investigation. NM and JH: writing—original draft. UH and JB: writing—review and editing. All authors contributed to the article and approved the submitted version.
Funding
This study was supported by the “KinderHerz- Innovationspreis NRW 2016.”
Acknowledgments
We would like to thank Ute Baur, study nurse, for indispensable support during the study.
Conflict of interest
The authors declare that the research was conducted in the absence of any commercial or financial relationships that could be construed as a potential conflict of interest.
Publisher’s note
All claims expressed in this article are solely those of the authors and do not necessarily represent those of their affiliated organizations, or those of the publisher, the editors and the reviewers. Any product that may be evaluated in this article, or claim that may be made by its manufacturer, is not guaranteed or endorsed by the publisher.
References
1. Naeije R. Physiological adaptation of the cardiovascular system to high altitude. Prog Cardiovasc Dis. (2010) 52:456–66.
2. Allemann Y, Stuber T, de Marchi SF, Rexhaj E, Sartori C, Scherrer U, et al. Pulmonary artery pressure and cardiac function in children and adolescents after rapid ascent to 3,450 m. Am J Physiol Heart Circ Physiol. (2012) 302:H2646–53. doi: 10.1152/ajpheart.00053.2012
3. Lindinger A, Schwedler G, Hense HW. Prevalence of congenital heart defects in newborns in Germany: Results of the first registration year of the PAN Study (July 2006 to June 2007). Klin Padiatr. (2010) 222:321–6. doi: 10.1055/s-0030-1254155
4. Gargiulo GD, Bassareo PP, Careddu L, Egidy-Assenza G, Angeli E, Calcaterra G. What have we learnt 50 years after the first Fontan procedure? J Cardiovasc Med (Hagerstown). (2020) 21:349–58. doi: 10.2459/JCM.0000000000000951
5. Holbein CE, Veldtman GR, Moons P, Kovacs AH, Luyckx K, Apers S, et al. Perceived health mediates effects of physical activity on quality of life in patients with a Fontan circulation. Am J Cardiol. (2019) 124:144–50. doi: 10.1016/j.amjcard.2019.03.039
6. Howell HB, Zaccario M, Kazmi SH, Desai P, Sklamberg FE, Mally P. Neurodevelopmental outcomes of children with congenital heart disease: A review. Curr Probl Pediatr Adolesc Health Care. (2019) 49:100685.
7. Clift P, Celermajer D. Managing adult Fontan patients: Where do we stand? Eur Respir Rev. (2016) 25:438–50. doi: 10.1183/16000617.0091-2016
8. Sutherland N, Jones B, d’Udekem Y. Should we recommend exercise after the Fontan procedure? Heart Lung Circ. (2015) 24:753–68.
9. Cordina R, d’Udekem Y. Long-lasting benefits of exercise for those living with a Fontan circulation. Curr Opin Cardiol. (2019) 34:79–86. doi: 10.1097/HCO.0000000000000582
10. Takken T, Hulzebos HJ, Blank AC, Tacken MH, Helders PJ, Strengers JL. Exercise prescription for patients with a Fontan circulation: Current evidence and future directions. Neth Heart J. (2007) 15:142–7. doi: 10.1007/BF03085970
11. Goldberg DJ, Avitabile CM, McBride MG, Paridon SM. Exercise capacity in the Fontan circulation. Cardiol Young. (2013) 23:824–30.
12. Joshi VM, Carey A, Simpson P, Paridon SM. Exercise performance following repair of hypoplastic left heart syndrome: A comparison with other types of Fontan patients. Pediatr Cardiol. (1997) 18:357–60. doi: 10.1007/s002469900199
13. Takken T, Tacken MH, Blank AC, Hulzebos EH, Strengers JL, Helders PJ. Exercise limitation in patients with Fontan circulation: A review. J Cardiovasc Med (Hagerstown). (2007) 8:775–81.
14. Rybicka J, Dobrowolski P, Marczak M, Niewiadomska J, Kowalczyk M, Hoffman P, et al. Single ventricle systolic function and cardiopulmonary exercise performance in adult patients with Fontan circulation. Int J Cardiol. (2020) 321:75–80.
15. Brassard P, Bedard E, Jobin J, Rodes-Cabau J, Poirier P. Exercise capacity and impact of exercise training in patients after a Fontan procedure: A review. Can J Cardiol. (2006) 22:489–95. doi: 10.1016/s0828-282x(06)70266-5
16. Greutmann M, Le TL, Tobler D, Biaggi P, Oechslin EN, Silversides CK, et al. Generalised muscle weakness in young adults with congenital heart disease. Heart. (2011) 97:1164–8.
17. Turquetto ALR, Caneo LF, Agostinho DR, Oliveira PA, Lopes M, Trevizan PF, et al. Impaired pulmonary function is an additional potential mechanism for the reduction of functional capacity in clinically stable Fontan patients. Pediatr Cardiol. (2017) 38:981–90. doi: 10.1007/s00246-017-1606-9
18. Cordina R, O’Meagher S, Gould H, Rae C, Kemp G, Pasco JA, et al. Skeletal muscle abnormalities and exercise capacity in adults with a Fontan circulation. Heart. (2013) 99:1530–4.
19. Staempfli R, Schmid JP, Schenker S, Eser P, Trachsel LD, Deluigi C, et al. Cardiopulmonary adaptation to short-term high altitude exposure in adult Fontan patients. Heart. (2016) 102:1296–301. doi: 10.1136/heartjnl-2016-309682
20. Takken T, Evertse A, de Waard F, Spoorenburg M, Kuijpers M, Schroer C, et al. Exercise responses in children and adults with a Fontan circulation at simulated altitude. Congenit Heart Dis. (2019) 14:1005–12. doi: 10.1111/chd.12850
21. Garcia JA, McMinn SB, Zuckerman JH, Fixler DE, Levine BD. The role of the right ventricle during hypobaric hypoxic exercise: Insights from patients after the Fontan operation. Med Sci Sports Exerc. (1999) 31:269–76. doi: 10.1097/00005768-199902000-00011
22. Legendre A, Bonnet D, Bosquet L. Reliability of peak exercise stroke volume assessment by impedance cardiography in patients with residual right outflow tract lesions after congenital heart disease repair. Pediatr Cardiol. (2018) 39:45–50. doi: 10.1007/s00246-017-1725-3
23. Ebrahim M, Hegde S, Printz B, Abcede M, Proudfoot JA, Davis C. Evaluation of impedance cardiography for measurement of stroke volume in congenital heart disease. Pediatr Cardiol. (2016) 37:1453–7.
24. Legendre A, Guillot A, Ladouceur M, Bonnet D. Usefulness of stroke volume monitoring during upright ramp incremental cycle exercise in young patients with Fontan circulation. Int J Cardiol. (2017) 227:625–30. doi: 10.1016/j.ijcard.2016.10.087
25. Lee JA, Williams SM, Brown DD, Laurson KR. Concurrent validation of the Actigraph gt3x+, Polar Active accelerometer, Omron HJ-720 and Yamax Digiwalker SW-701 pedometer step counts in lab-based and free-living settings. J Sports Sci. (2015) 33:991–1000. doi: 10.1080/02640414.2014.981848
26. Chu AH, Ng SH, Paknezhad M, Gauterin A, Koh D, Brown MS, et al. Comparison of wrist-worn Fitbit Flex and waist-worn ActiGraph for measuring steps in free-living adults. PLoS One. (2017) 12:e0172535. doi: 10.1371/journal.pone.0172535
27. Härtel JA, Herberg U, Jung T, Winkler C, Breuer J, Müller N. Physical activity and heart rate monitoring in Fontan patients - Should we recommend activities in higher intensities? PLoS One. (2020) 15:e0228255. doi: 10.1371/journal.pone.0228255
28. Freedson P, Pober D, Janz KF. Calibration of accelerometer output for children. Med Sci Sports Exerc. (2005) 37:S523–30.
30. Buskirk ER, Kollias J, Akers RF, Prokop EK, Reategui EP. Maximal performance at altitude and on return from altitude in conditioned runners. J Appl Physiol. (1967) 23:259–66.
31. Kroidl RF, Schwarz S, Lehnigk B, Fritsch J. Kursbuch spiroergometrie. 3 ed. Stuttgart: Georg Thieme Verlag KG (2015). p. 330–54.
32. McCrindle BW, Williams RV, Mital S, Clark BJ, Russell JL, Klein G, et al. Physical activity levels in children and adolescents are reduced after the Fontan procedure, independent of exercise capacity, and are associated with lower perceived general health. Arch Dis Child. (2007) 92:509–14. doi: 10.1136/adc.2006.105239
33. Ogawa T, Spina RJ, Martin WH III, Kohrt WM, Schechtman KB, Holloszy JO, et al. Effects of aging, sex, and physical training on cardiovascular responses to exercise. Circulation. (1992) 86:494–503.
35. Claessen G, La Gerche A, Van De Bruaene A, Claeys M, Willems R, Dymarkowski S, et al. Heart rate reserve in Fontan patients: Chronotropic incompetence or hemodynamic limitation? J Am Heart Assoc. (2019) 8:e012008.
36. Hedlund ER, Soderstrom L, Lundell B. Appropriate heart rate during exercise in Fontan patients. Cardiol Young. (2020) 30:674–80.
37. Okolska M, Lach J, Matusik PT, Pajak J, Mroczek T, Podolec P, et al. Heart rate variability and its associations with organ complications in adults after Fontan operation. J Clin Med. (2021) 10:4492.
38. Harteveld LM, Blom NA, Terol Espinosa de Los Monteros C, van Dijk JG, Kuipers IM, Rammeloo LAJ, et al. Determinants of exercise limitation in contemporary paediatric Fontan patients with an extra cardiac conduit. Int J Cardiol. (2021) 341:31–8. doi: 10.1016/j.ijcard.2021.08.012
39. Diller GP, Okonko DO, Uebing A, Dimopoulos K, Bayne S, Sutton R, et al. Impaired heart rate response to exercise in adult patients with a systemic right ventricle or univentricular circulation: Prevalence, relation to exercise, and potential therapeutic implications. Int J Cardiol. (2009) 134:59–66.
40. La Gerche A, Gewillig M. What limits cardiac performance during exercise in normal subjects and in healthy Fontan patients? Int J Pediatr. (2010) 2010:791291.
41. Driscoll DJ. Cardiorespiratory responses to exercise after the Fontan operation. Circulation. (1990) 81:2016–7.
42. Turquetto ALR, Dos Santos MR, Sayegh ALC, de Souza FR, Agostinho DR, de Oliveira PA, et al. Blunted peripheral blood supply and underdeveloped skeletal muscle in Fontan patients: The impact on functional capacity. Int J Cardiol. (2018) 271:54–9. doi: 10.1016/j.ijcard.2018.05.096
43. Kodama Y, Koga K, Kuraoka A, Ishikawa Y, Nakamura M, Sagawa K, et al. Efficacy of sports club activities on exercise tolerance among japanese middle and high school children and adolescents after Fontan procedure. Pediatr Cardiol. (2018) 39:1339–45. doi: 10.1007/s00246-018-1900-1
44. Rhodes J, Curran TJ, Camil L, Rabideau N, Fulton DR, Gauthier NS, et al. Sustained effects of cardiac rehabilitation in children with serious congenital heart disease. Pediatrics. (2006) 118:e586–93. doi: 10.1542/peds.2006-0264
45. Hemphill NM, Kuan MTY, Harris KC. Reduced physical activity during COVID-19 pandemic in children with congenital heart disease. Can J Cardiol. (2020) 36:1130–4.
Keywords: Fontan patients, artificial altitude, exercise capacity, physical activity, hemodynamic adaptation
Citation: Müller N, Herberg U, Jung T, Breuer J and Härtel JA (2022) Adequate exercise response at artificial altitude in Fontan patients. Front. Pediatr. 10:947433. doi: 10.3389/fped.2022.947433
Received: 18 May 2022; Accepted: 21 July 2022;
Published: 18 August 2022.
Edited by:
Jaspal Dua, Liverpool Heart and Chest Hospital NHS Trust, United KingdomReviewed by:
Tim Takken, University Medical Center Utrecht, NetherlandsHideo Ohuchi, National Cerebral and Cardiovascular Center, Japan
Copyright © 2022 Müller, Herberg, Jung, Breuer and Härtel. This is an open-access article distributed under the terms of the Creative Commons Attribution License (CC BY). The use, distribution or reproduction in other forums is permitted, provided the original author(s) and the copyright owner(s) are credited and that the original publication in this journal is cited, in accordance with accepted academic practice. No use, distribution or reproduction is permitted which does not comply with these terms.
*Correspondence: Nicole Müller, bmljb2xlLm11ZWxsZXJAdWtib25uLmRl