- 1Développement, Adaptation et Handicap Laboratory (DevAH), Université de Lorraine, Nancy, France
- 2Aix-Marseille University, CNRS, ISM, Marseille, France
- 3CHRU, Maternité Régionale, Nancy, France
- 4CNRS, LPNC, Université Grenoble Alpes, Université Savoie Mont Blanc, Grenoble, France
School-aged prematurely born children (PC) have a higher risk of academic difficulties, which may be partly explained by attention difficulties. It has been suggested that children’s attentional performance might be influenced by their body posture and spontaneous body motion. The aim of this study (ClinicalTrials.gov – NCT 03125447) was to test the influence of three body mobility conditions on the three functions of attention (alertness, orienting, and executive control) among school-aged PC vs. term-born children (TC). Notably, 21 PC and 21 TC performed the Attention Network Test for Children in three body mobility conditions, namely, sitting and standing imposed fixed postures and a free-to-move condition. The children’s median reaction times were compared between trials (1) with and without alerting cues, (2) with valid and invalid orienting cues, and (3) with and without distracting information, to calculate the performance of alertness, orienting, and executive control, respectively. Results showed that with distracting information, PC exhibited significantly slower responses in the standing-still posture than in the sitting-still posture (1,077 ± 240 vs. 1,175 ± 273 ms, p < 0.05), but not TC. No difference was observed with the free-to-move condition. PC and TC did not significantly differ in alertness or orienting, regardless of body mobility condition. These data suggest that PC must use executive resources to stand still and maintain position, which impairs their performance during executive tasks. We speculate that these results may be related to less developed postural control and motor inhibition in PC.
Introduction
Prematurely born children (PC) carry a risk of adverse long-term outcomes (1–3) including a high risk of cognitive impairment at school age (1, 4). Academic difficulties are more frequent among PC than in term-born children (TC), as revealed by lower reading, spelling, and mathematics performance, and greater needs for special education (5–7). These academic difficulties are sustained throughout schooling, suggesting that it is difficult to catch up from early learning delays (5, 8).
Learning difficulties in PC are partly explained by poorer executive functions and attention abilities (9–13). In fact, PC exhibit lower performance in attention skills during childhood (14–21). Three attentional functions have been described: alertness, orienting, and executive control (22). Alertness refers to the achievement and maintenance of an appropriate level of vigilance. It is divided into the general control of arousal (tonic alertness) during a task and the increase in response readiness following an acute external cueing (phasic alertness) (23). Although this function strongly developed at preschool age (24), at 6 years of age, tonic and phasic alertness have not reached the adult level yet (25). Orienting refers to the ability to select and prioritize information from the environment (23). Some studies indicate that automatic attention shifting is mature at 6 years, (25) while others suggest later development (26, 27). Finally, executive control, also termed inhibition, corresponds to the ability to detect errors, resolve conflicts, and resist distraction during goal-oriented behaviors. This develops strongly in preschool-aged children and continues to advance throughout childhood (19, 28–30).
The efficiency of each attentional function may be separately assessed using the Attention Network Test (ANT), (31) which has been adapted for children (ANT-c) (25). Studies have used this test to describe attentional functions during childhood among TC (32) and PC (14, 15, 17). While overall response time and accuracy may be reduced in PC compared with TC (14, 15) due to more lapses of attention (14), the ANT-c highlights that prematurity may have different effects on each attentional function (14, 15, 17, 19). In fact, while PC and TC of 5–7 years of age exhibit no significant differences in phasic alertness (14–17) or exogenous orienting (14, 15), PC show poorer executive performance (15, 17). This suggests that PC have greater difficulties in maintaining attention for a long time and resisting distraction in class.
School-aged children have a substantial urge for mobility (33) and are sometimes described as “hyperactive” even in the absence of significant attention disorders, which is thus different from attention-deficit/hyperactivity disorder syndrome. Additionally, this excessive motor activity may be transient (34) during childhood, raising questions about its role in young children. Notably, children’s motor activity does not always disturb attention functions, as revealed by the improvement of tonic alertness and executive control following physical activities (35, 36). The relationship between motor and cognitive activities strongly depends on the body posture, the motor activity, and the cognitive function studied. For example, adults have performed better on arithmetic tasks in a sitting posture than in a standing posture, whereas they performed better on memory tasks while walking (37). Regarding the function of attention, alertness (38, 39), and to a lesser extent executive control (40–43), but not orienting (38), may be improved in a standing compared with a sitting posture. We also previously demonstrated that in TC of 6–7 years of age, standing may improve executive control compared with a sitting posture (32). Spontaneous body motions and adopting a standing/upright posture may enable children to increase cortical arousal (40, 44–46). This beneficial influence of changing posture (38, 40) or of physical activity (35, 36) on attention suggests that the classic “sitting still posture” at school may not be optimal for children’s attention. Free mobility might be more effective than an imposed mobility or posture, as suggested by the increased memory performance when children walked at their preferred speed, but not at an imposed speed (47).
In this study, the primary aim was to determine the influence of posture and free mobility on the three attentional functions in PC vs. TC. We hypothesized that adopting free mobility, as compared with imposed postures, might help PC improve their attention performance. Additionally, we hypothesized that a standing posture would improve attention performance, as compared with a sitting posture.
Materials and methods
This prospective controlled study was performed at the Pediatric Outpatient Unit of the level III Maternity Hospital of Nancy. It was conducted in accordance with the Declaration of Helsinki and approved by the Comité de Protection des Personnes Sud-Est III Ethics Committee (2017–010 B). The method of this study has already been documented, (48) and TC behavior was previously analyzed (32).
In brief, inclusion criteria were PC with gestational age (GA) of <34 weeks, aged between 6 and 7 years. These children were included in a routine regional follow-up program. They were thoroughly followed yearly for neurodevelopment and motor ability. Only infants without disability or previously diagnosed neurodevelopment delay were involved in this study. They were attending normal schools at their appropriate grade level.
Children with any neurological, cognitive, developmental, or motor disorders preventing the realization of the tests were excluded. Exclusion criteria included any motor impairment, visual refractory impairment, strabismus, visuospatial difficulties not corrected by orthoptic therapy, daltonism, not corrected hearing loss, suspected or diagnosed attention deficit hyperactivity disorder, or autism spectrum disorder.
To recruit TC of equal age, parents were informed about the study through leaflets. Sociodemographic, perinatal, morbidity, anthropometric data, and visual acuity were recorded by a certified trained pediatrician during a clinical examination. Since PC are usually considered to have a learning ability delay of approximately 1 year, we previously determined (48) that we would need 24 children in each group to demonstrate some catch-up in PC attention performance related to body mobility condition, with sitting posture as the reference, with an alpha risk of 0.00625 (Bonferroni correction for the number of tests) and a power of 0.80 (Power and Precision V4, Biostat Inc., Englewood, NJ, United States 2001). Additionally, as a secondary evaluation of the effect of postnatal age, we stratified the children into two groups according to the median age of our population.
Materials and design
The attention network test for children
Children were instructed to click as quickly as possible on the right or left button of a mouse, depending on whether a target fish was facing left or right (25). This target could appear above or below a central cross, alone or surrounded by flanking fishes pointing toward the same (congruent) or the opposite (incongruent) direction. Furthermore, it could appear suddenly (no cue) or be preceded by one of three equiprobable warning cues (an asterisk appearing 150 ms before the fish): a spatial cue or a center cue orienting attention toward the location of the upcoming target or the central cross, respectively, or a double cue giving no directional information.
Body mobility condition
Children performed the ANT-c in three pseudo-randomized body mobility conditions: (i) sitting-still posture, (ii) standing-still posture, and (iii) free-to-move condition. The ANT-c was generated using the E-Prime software (version 3.0 professional; Psychological Software Tools®, Sharpsburg PA, United States) and was projected with a head-mounted display (iWear Video Headphones, The Way In®, Vuzix Corporation, New York, United States) to keep the child’s eyes and the target constant, in any body mobility condition. Children’s movements were video-recorded, reported on a standardized evaluation grid, and independently analyzed by JR and HC. The general amount of movements (Mov) was calculated for each body mobility condition (32).
For each body mobility condition, a block of 48 trials was performed, with 3 min of rest between blocks. The experiment lasted approximately 45 min. Children’s success and reaction times were recorded for each trial.
Data reduction and statistical analyses
Normally distributed data are presented as mean values and standard deviation (M ± SD); others as the median and interquartile range (Med [IQR]). A chi-square test was used to compare categorical variables between PC and TC.
For each child, we calculated the general success rate and the general median reaction time for correct responses (General RT) to determine the overall performance. The Mann-Whitney U tests were then performed on the general success rate to compare PC and TC in each body mobility condition, and a Friedman test was used to assess the effect of body mobility condition. In addition, mixed ANOVAs were performed on General RT, with the body mobility condition as a within-subject factor and prematurity (PC and TC) as a between-subject factor.
For each child, we also calculated the median reaction time (MedRT) for correct responses for the trials with the same condition of cue (no cue, double cue, center cue, or spatial cue) or the same condition of targets (congruent or incongruent). Then, the children’s performance in challenging trials (i.e., no cue, center cue, and incongruent trials) was compared with control trials (i.e., double cue, spatial cue, and congruent trials) for alertness, orienting, and executive control, respectively. Three mixed ANOVA were performed on MedRT (one for each attentional function), with prematurity as a between-subject factor and body mobility condition and challenging vs. control trials as within-subject factors. For these analyses, the children’s postnatal age was added as a covariate (49).
Additionally, to better evaluate the effect of development, we divided the children into two age groups separated by the medians of PC and TC, i.e., postnatal age of < 80 months vs. ≥ 80 months. They were evaluated by mixed ANOVA on MedRT, with the body mobility condition and the executive control targets as within-subject factors and the age group as a between-subject factor.
For all analyses, post hoc tests were conducted using Tukey’s honestly significant difference method.
Results
Results among TC have already been described (32). The results among PC are presented below.
A total of 24 PC and 25 TC were eligible for this study. Three PC and three TC were unable to complete the experiment, and one TC made 51% of errors. Overall, 21 PC and 21 TC were included in our analyses. One PC responded correctly only once on all incongruent trials, despite further explanations and training. This child was included for analyses of alertness and orienting because her success rate was consistent with the group in all trials when incongruent trials were excluded. If some trials were missing, due to technical issues (N = 2) or children’s non-compliance (N = 2, range of missing trials rate: 5–26%), general success rate, GeneralRT, and MedRT were calculated from the remaining trials.
Table 1 summarizes the children’s characteristics. No significant differences were observed between the two groups, except for parents’ employment, as the mothers of PC were more likely to be unemployed or employees/workers than the mothers of TC (p = 0.002).
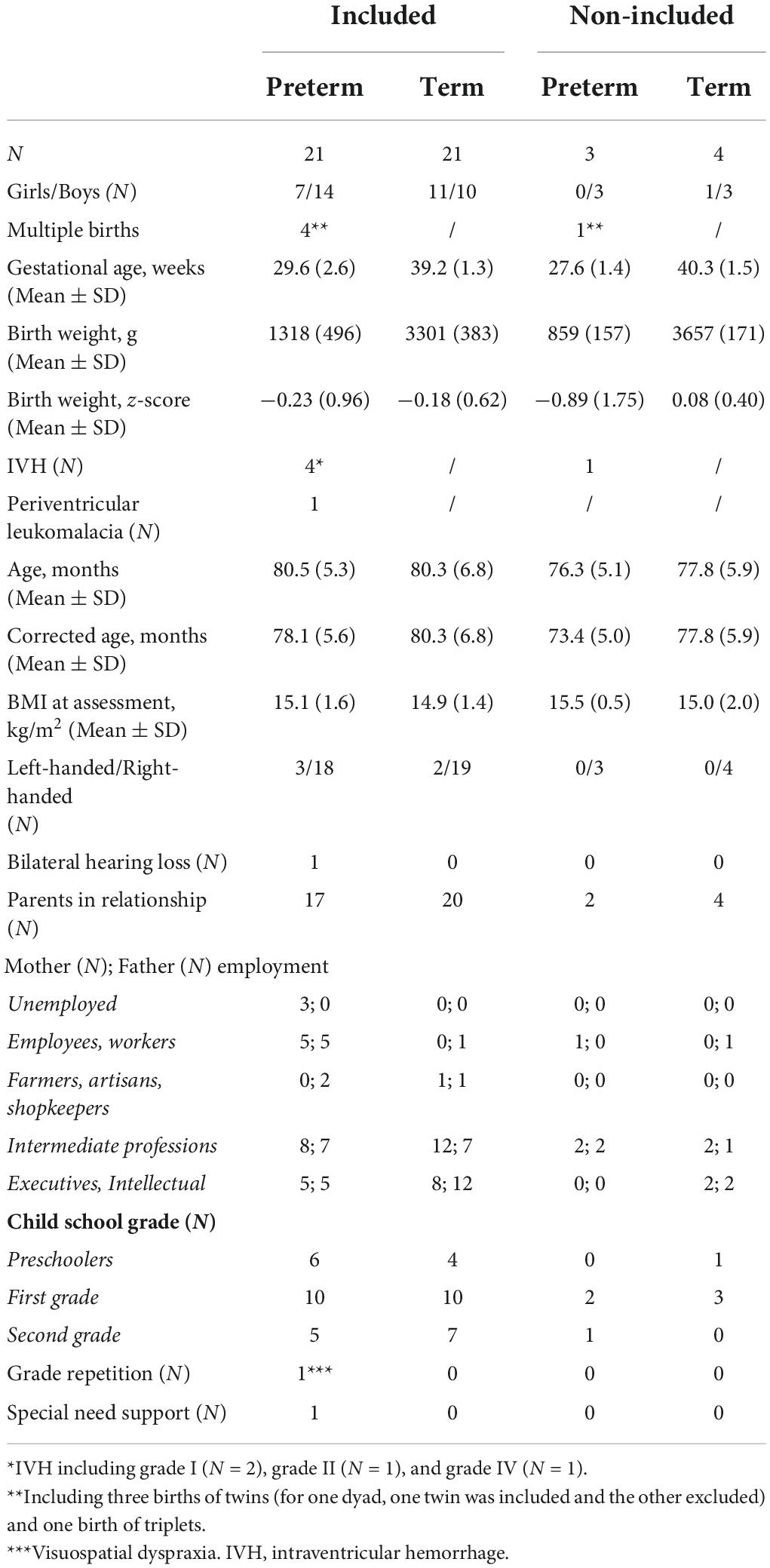
Table 1. Characteristics of preterm-born children and term-born children (included vs. non-included).
Overall performance (General RT and accuracy)
PC and TC did not differ in the general success rate, either for the sitting-still posture (95.8 [8.3] vs. 95.8 [8.3]%, p = 0.68), the standing-still posture (92.7 [11.0] vs. 95.8 [4.2]%, p = 0.12), or the free-to-move condition (91.7 [7.3] vs. 93.8 [4.2]%, p = 0.22). We also did not observe differences between the body mobility conditions. Table 2 presents the general RT for TC and PC. We found no main effect of prematurity (p = 0.17), body mobility (p = 0.89), or prematurity*body mobility interaction (p = 0.80).
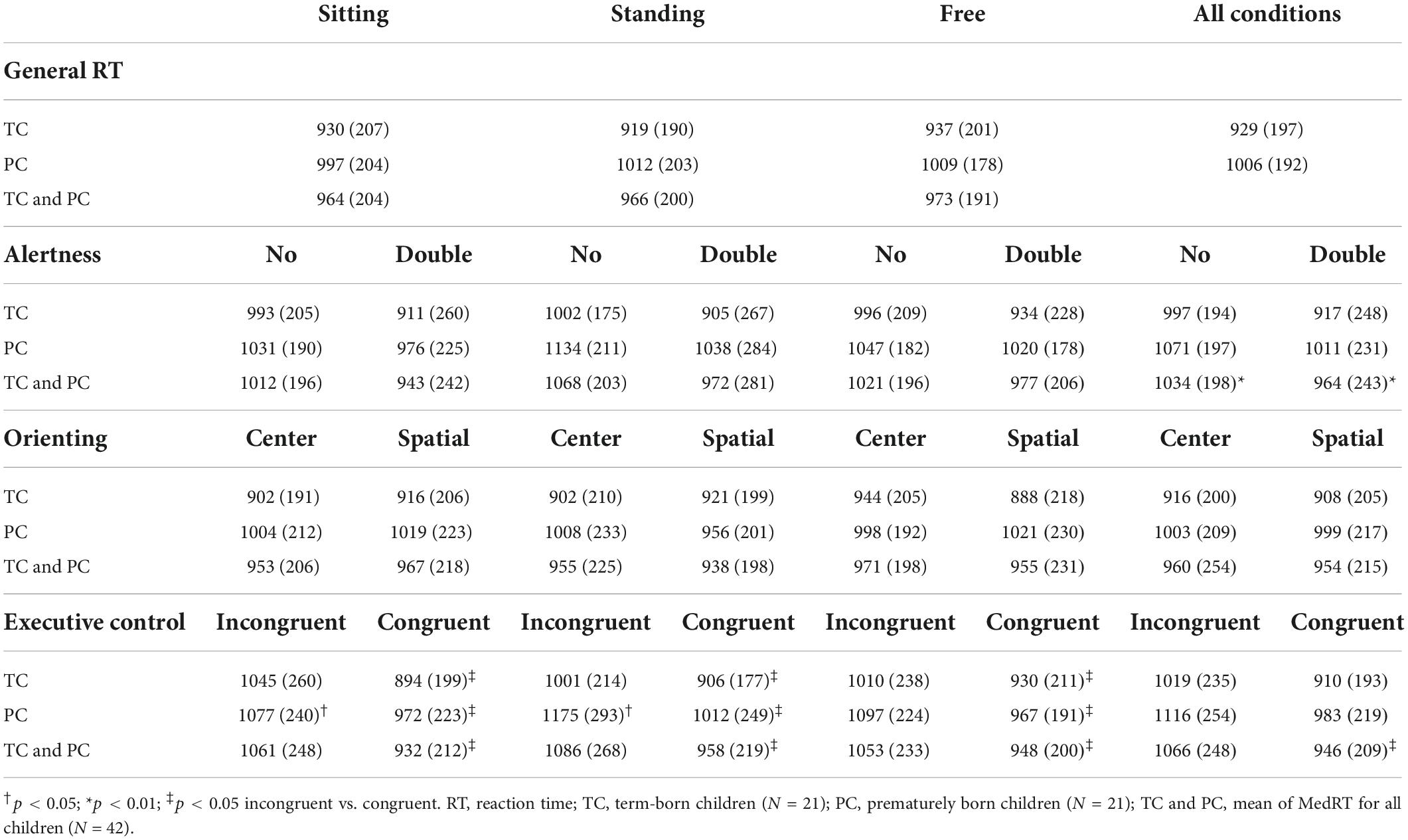
Table 2. Mean (SD) of MedRT (in ms) for PC and TC for the different cues and targets, in the three body mobility conditions.
Separate attentional functions
Table 2 presents MedRT for PC and TC for the different conditions. The attention effects are presented in the supplemental material (Supplementary Figure 1) and can be calculated from the data in Table 2.
Executive control
Our analyses revealed a significant body mobility*prematurity*target interaction effect for MedRT (p = 0.04, η2 = 0.08, 95%CI [0–0.21]). Post hoc analyses showed that PC and TC responded significantly faster in congruent trials compared with incongruent trials under the three body mobility conditions (p < 0.05, Table 2). However, during incongruent trials, PC responded significantly slower in the standing-still condition than in the sitting-still condition (p < 0.05) and tended to respond slower in the standing-still condition than in the free-to-move condition (p = 0.06), with no difference between the sitting-still vs. free-to-move condition (p > 0.05). Among TC, we found no significant difference in MedRT between the three body mobility conditions during incongruent trials. Moreover, among TC, we previously showed that the difference between incongruent and congruent trials was more important in the sitting-still posture than in the standing-still posture (32), suggesting that the sitting-still and standing-still postures have opposite effects between PC and TC. These results are summarized in Figure 1.
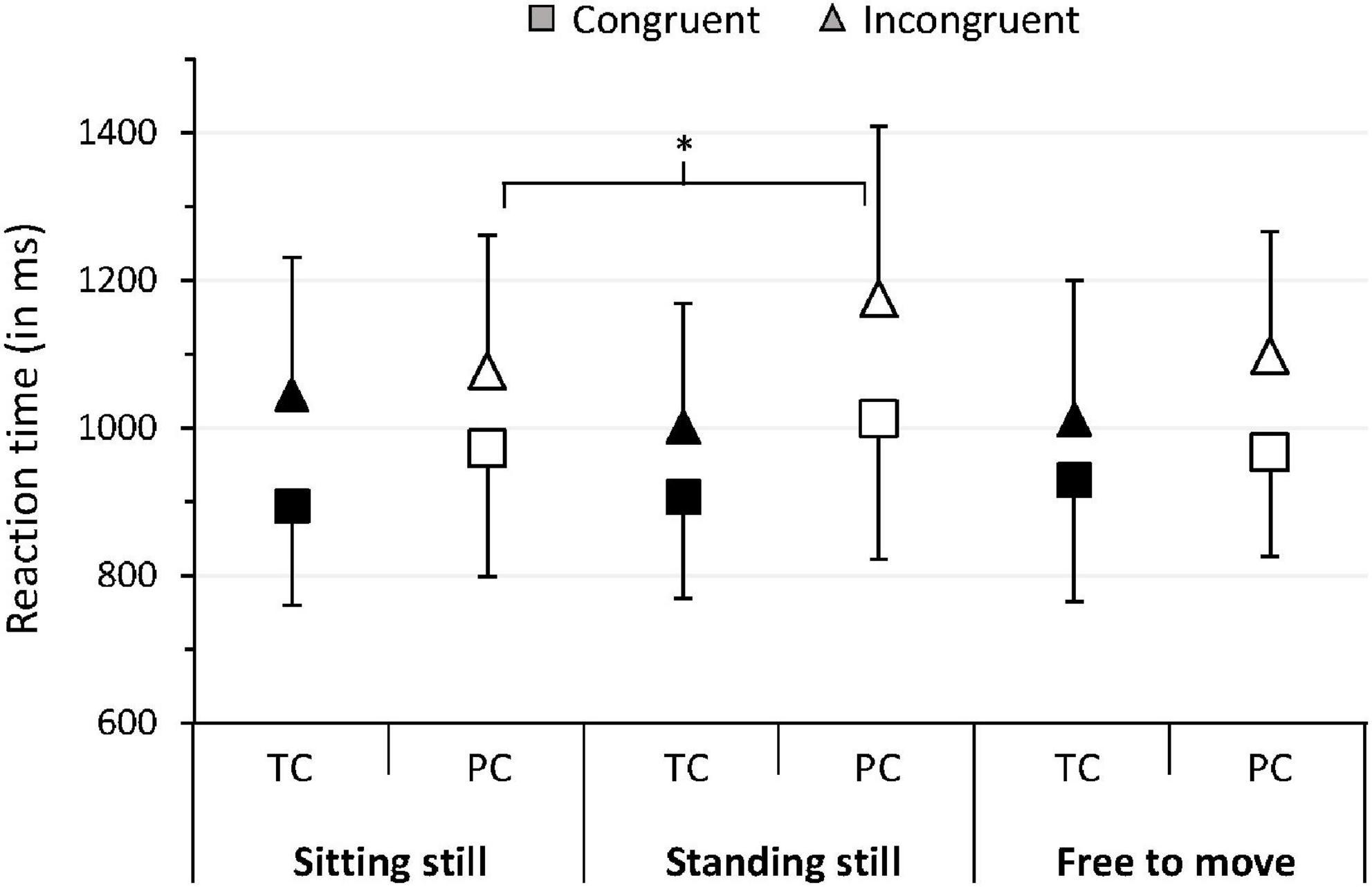
Figure 1. Mean of median RT for PC and TC in trials with congruent and incongruent targets in the three body mobility conditions. Error bars represent the mean absolute difference. *p < 0.05. TC, term-born children; PC, prematurely born children.
Alertness
All children (PC and TC) responded significantly faster with a double cue than without a cue (964 ± 243 vs. 1,034 ± 198 ms, p < 0.001, 95%CI [0.19–0.60]). This effect did not interact neither with body mobility (p < 0.11) with prematurity (p = 0.51) nor with body mobility by prematurity group (p = 0.51).
Orienting
The MedRT of all children (PC and TC) did not differ significantly between the two types of the orienting cue (954 ± 215 ms for the center cue vs. 960 ± 208 ms for the spatial cue, p = 0.61). This result neither interacts with body mobility (p = 0.89) nor with prematurity (p = 0.61). There was a significant body mobility*prematurity*cue interaction for MedRT (p = 0.03), but no difference was found on post hoc analyses.
Age
For all attentional functions, age did not significantly interact with a cue or with the interaction of interest (all p > 0.05). When the children were divided according to median postnatal age of < or ≥ 80 months, we observed a significant main effect of age group on MedRT (p = 0.03, η2 = 0.11, 95%CI [0–0.31]), with younger children (74.7 ± 2.5 months) responding significantly slower than older children (85.0 ± 3.5 months, 1,081 ± 241 vs. 947 ± 217ms, respectively). However, age*target interaction (p = 0.89), or age*target*body mobility interaction (p = 0.37) did not reach significance.
Movements of children during the ANT-c
The rates of head and limb movement for PC and TC in the three body mobility conditions are available in the supplemental material (Supplementary Figure 2). For all body mobility conditions and body parts, in both TC and PC, we found an over 80% rate of < 5 s of movement per minute. There was a significant effect of body mobility condition on Mov (W = 0.36; p < 0.001.). Mov was greater in the free-to-move condition than in the sitting-still condition (0.26 [0.84] vs. 0.09 [0.17], r = 0.85, p < 0.001) and the standing-still condition (0.07 [0.18], r = 0.81, p < 0.001), with no difference between the sitting-still and the standing-still conditions (p = 0.40). We found no difference of Mov between PC and TC in the sitting-still (0.12 [0.26] vs. 0.07 [0.15], p = 0.11), standing-still (0.08 [0.21] vs. 0.07 [0.13], p = 0.65), or free-to-move condition (0.53 [0.83] vs. 0.12 [0.50], p = 0.06).
Discussion
Overall performance
This study aimed to determine the influence of three body mobility conditions on the attention functions of prematurely and term-born children aged 6–7 years. It showed that, while no difference between PC and TC in terms of overall performance was found in any mobility condition, differences appeared when attentional functions were studied separately. Contrary to our a priori hypothesis, free mobility did not improve the attentional functions of PC or TC, as compared with fixed imposed postures. Nevertheless, compared with the sitting-still posture, adopting a standing-still posture resulted in decreased executive control for PC but not for TC.
Separate attentional functions
Executive control
Prematurely born children exhibited reduced executive performance when standing still. On the opposite, we previously showed an improvement of executive control in TC when standing still, which we explained by an increase in arousal and optimal mental state in this posture (32). Olivier et al. suggested that interdependency between postural and executive activities may vary during childhood and depend on the level of difficulty of both cognitive and postural tasks (50). In line with this idea, we speculate that the standing-still posture was a more challenging postural task for PC. In fact, PC presents an increased risk of developmental coordination disorder (DCD). Studying 22,989 children from the Danish National Birth Cohort Questionnaire, Zhu et al. observed a significant inverse association between gestational age at birth and the risk of DCD at 7 years of age (51). DCD can be defined as minor gross and/or fine motor impairments, which cannot be explained by cerebral palsy or intellectual impairments but can influence the daily lives of children. In a meta-analysis of 36 studies, Van Horn et al. did not find any specific risk factor of DCD but preterm birth and male sex (52). In our study, despite no major motor coordination impairment was observed, one cannot exclude that some minor coordination disorder had contributed in part to the difficulties observed in PC when standing. In a study comparing 105 prematurely born infants to controls matched for age and sex with the Movement Assessment Battery for Children (M-ABC), De Rose et al. showed a higher risk of perceptual-motor difficulties at 4 years and concluded that a delayed maturation was possibly explaining the observed results (53). In line with our assumption that standing still is difficult for PC, other authors reported lower performance for these children during the balance tasks of the M-ABC, with greater difficulties standing on one leg or walking heel-to-toe (54–58). Also, some studies using a force plate (59–61), but not all (58, 62), revealed less efficient postural control with more body sway in PC. Lorefice et al., especially, reported more postural sway in 4 years PC than in TC when performing a picture naming task (59), supporting the functional link between postural and cognitive activities postulated by Olivier et al. (50). Thus, we proposed that the standing-still posture was difficult to maintain for PC, requiring executive resources, which decreased their performance in the trials demanding executive control. One may speculate that it was the cognitive and motor constraints associated with the instruction to stay still that made standing up very difficult for PC, as they may have difficulties suppressing automatic or inappropriate motor responses due to motor inhibition difficulties (19, 63).
This last assumption implies that the instruction “stay still without moving” may be difficult for PC and alter their performances. Nevertheless, the lack of executive control difference between the free-to-move condition and the sitting posture with the instruction to stay still might support that the posture adopted (standing vs. sitting) may more influence executive performance than the instruction to stay still. Supporting this view was that in the free-to-move condition, children often adopted a sitting posture [21 TC and 18 PC seated at least one time, among them 11 TC and 4 PC exclusively sat (Supplementary Figure 2 in Supplementary material)]. However, this ecological free-to-move condition where children (1) did not have the instruction to stay still and (2) could adopt a free motor behavior did not let us precisely disentangle the influence of the instruction from the influence of the posture and body mobility adopted on the executive performance of children. Further analysis may be interesting in order to respond to this answer.
Alertness
In all body mobility conditions, PC and TC were both faster after a warning signal, with PC achieving a level of readiness comparable with TC. This is consistent with the literature evaluating the phasic alertness of PC (14, 15, 17). Notably, the short duration of each block of trials did not allow the evaluation of tonic alertness in our study. Body mobility condition did not affect alertness in PC or TC, in contrast to studies of adults showing improved alertness when standing (38) or during physical activity (64). Children may not exhibit improved alertness when standing because it is more challenging for them to stand still than adults since their postural control is not fully developed (65). Regarding the free-to-move condition, one may speculate that the amount of children’s motor activities was not high enough to increase arousal to a sufficient level for improving alertness (45).
Orienting
Prematurity was not found to influence orienting. This is consistent with the litterature (14, 15, 17) and suggests that automatic gaze shifting is equally developed in PC and TC. Notably, neither PC nor TC responded faster when a cue oriented their attention to the location of the upcoming target, suggesting that orienting may not be yet fully developed in PC or TC aged 6–7 years (26). In addition, no influence of the body mobility condition was found on the function of orienting, neither in PC nor in TC. A decrease in body mobility may be observed when participants have to precisely displace and orient their gaze (66). If a decrease in body mobility and gaze stabilization is beneficial for the function of orienting, it can explain why a more challenging posture than the standing posture and a free-to-move condition did not help children to orient their attention better.
Age
The small sample size and the limited age range of our study might have prevented the observation of a significant improvement in attentional function with age. In fact, Mezzacappa (26) showed that age was associated with improved use of alerting cues and orienting cues and improved dealing with interference, among 249 children between 5 and 7 years. Notably, we observed an overall faster reaction time for children older than 80 months compared with those who were younger, consistent with improvement of processing with age. A clinical implication is that infants born at the end of a calendar year might show slightly lower performances than their counterparts born at the beginning of the same calendar year.
Strengths and limitations
The use of the ANT-c enabled testing of the attentional functions both together and separately. We showed different influences on body mobility and prematurity depending on the attention function studied.
This study has limitations. First, the relatively small sample size and the exclusion criteria may limit the generalization of the results to the entire PC population. However, these results may apply to PC attending normal schools in their appropriate grade level. Second, mothers of PCs had a generally lower social level, but this reflects the higher rate of PC within a lower socioeconomic background (67). Third, we did not evaluate the IQ of the children at the time of the study. This was mainly due to the need for limitation of the experiment duration. An excessive children’s tiredness would have been an important bias for evaluating attentional performance. However, it was confirmed during the interview with parents and the clinical exam by the trained pediatrician that none of the children had cognitive, developmental, or motor disorders. Finally, regarding the free-to-move condition, approximately 80% of the children moved their body parts for less than 5 s per minute (Supplementary Figure 2 in Supplementary material). As stated previously, one may speculate that the level of motor activity in our children was not sufficient to improve alertness or executive performance. One explanation may be that all children needed to stabilize the position of their head and their gaze in order to correctly perform the requested visual task, reducing their motor activities. Furthermore, despite the interest in using a head-mounted display in order to keep the eyes and the target constant, it is possible that this device reduced the spontaneous motricity of the children due to their infrequent use in ecological situations and the lack of visual information about the environment. Evaluating the influence of body mobility on other cognitive tasks, using other devices and modalities (e.g., auditive tasks), may be interesting to confirm this assumption. In addition, it would be interesting in future studies to use devices, such as accelerometers or force plates, to more precisely evaluate the levels of motor activity and postural control among PC and TC.
Conclusion
This study supports the importance of including body activity analysis when evaluating cognitive performance. It showed that it is important (1) to study more precisely and separately the three attentional functions that may have different development timings in children born at term or prematurely and (2) to include observation of their motor activities in the evaluation.
Our results showed that executive control decreased in the standing-still posture compared with the sitting-still posture among PC, contrasting with TC and adult behavior. The clinical implication may be that PC would alter their cognitive performance and learning ability when asked to stand up at school contrary to term-born children. Taking into account body posture and mobility during classes might prevent those vulnerable children from altering their cognitive function. It would improve their learning ability and academic achievement. To confirm this assumption, it would be interesting to perform a more precise analysis of PC postural activity while performing cognitive tasks.
Data availability statement
The raw data supporting the conclusions of this article will be made available by the authors, without undue reservation.
Ethics statement
The studies involving human participants were reviewed and approved by Comité de Protection des Personnes Sud-Est III Ethics Committee (2017–010 B). Written informed consent to participate in this study was provided by the participants’ legal guardian/next of kin.
Author contributions
JR, J-MH, HC, SC, and HD contributed to the conception and design of this study. IH performed a clinical examination for each child. HD followed the yearly evaluation of the PC. JR, AMJH, and HC conducted the experiments and collected the data. JR and HC analyzed children’s movements. JR, SC, HC, and J-MH processed the data and performed the statistical analysis. JR, HC, SC, IH, and J-MH discussed the results and wrote the manuscript. All authors contributed to this study and approved the submitted version.
Funding
This study was supported by the Université de Lorraine “Soutien à des Actions de Recherches – Crédits SC-UL 2017”.
Conflict of interest
The authors declare that the research was conducted in the absence of any commercial or financial relationships that could be construed as a potential conflict of interest.
Publisher’s note
All claims expressed in this article are solely those of the authors and do not necessarily represent those of their affiliated organizations, or those of the publisher, the editors and the reviewers. Any product that may be evaluated in this article, or claim that may be made by its manufacturer, is not guaranteed or endorsed by the publisher.
Supplementary material
The Supplementary Material for this article can be found online at: https://www.frontiersin.org/articles/10.3389/fped.2022.928541/full#supplementary-material
References
1. Larroque B, Ancel PY, Marret S, Marchand L, André M, Arnaud C, et al. Neurodevelopmental disabilities and special care of 5-year-old children born before 33 weeks of gestation (the EPIPAGE study): a longitudinal cohort study. Lancet. (2008) 371:813–20. doi: 10.1016/S0140-6736(08)60380-3
2. Larroque B, Ancel PY, Marchand-Martin L, Cambonie G, Fresson J, Pierrat V, et al. Special care and school difficulties in 8-year-old very preterm children: the epipage cohort study. PLoS One. (2011) 6:e21361. doi: 10.1371/journal.pone.0021361
3. Delobel-Ayoub M, Arnaud C, White-Koning M, Casper C, Pierrat V, Garel M, et al. Behavioral problems and cognitive performance at 5 years of age after very preterm birth: the epipage study. Pediatrics. (2009) 123:1485–92. doi: 10.1542/peds.2008-1216s
4. Erdei C, Austin NC, Cherkerzian S, Morris AR, Woodward LJ. Predicting School-Aged Cognitive Impairment in Children Born Very Preterm. Pediatrics. (2020) 145:e20191982. doi: 10.1542/peds.2019-1982
5. Twilhaar ES, de Kieviet JF, van Elburg RM, Oosterlaan J. Academic trajectories of very preterm born children at school age. Arch Dis Child Fetal Neonatal Ed. (2019) 104:F419–23. doi: 10.1136/archdischild-2018-315028
6. Twilhaar ES, de Kieviet JF, Aarnoudse-Moens CS, van Elburg RM, Oosterlaan J. Academic performance of children born preterm: a meta-analysis and meta-regression. Arch Dis Child Fetal Neonatal Ed. (2018) 103:F322–30. doi: 10.1136/archdischild-2017-312916
7. McBryde M, Fitzallen GC, Liley HG, Taylor HG, Bora S. Academic outcomes of school-aged children born preterm: a systematic review and meta-analysis. JAMA Netw Open. (2020) 3:e202027. doi: 10.1001/jamanetworkopen.2020.2027
8. Taylor R, Pascoe L, Scratch S, Doyle LW, Anderson P, Roberts G. A simple screen performed at school entry can predict academic under-achievement at age seven in children born very preterm: academic impairment and prematurity. J Paediat Child Health. (2016) 52:759–64. doi: 10.1111/jpc.13186
9. Akshoomoff N, Joseph RM, Taylor HG, Allred EN, Heeren T, O’Shea TM, et al. Academic achievement deficits and their neuropsychological correlates in children born extremely preterm. J Dev Behav Pediatr. (2017) 38:627–37. doi: 10.1097/DBP.0000000000000479
10. Jaekel J, Wolke D, Bartmann P. Poor attention rather than hyperactivity/impulsivity predicts academic achievement in very preterm and full-term adolescents. Psychol Med. (2013) 43:183–96. doi: 10.1017/S0033291712001031
11. Leijon I, Ingemansson F, Nelson N, Wadsby M, Samuelsson S. Reading deficits in very low birthweight children are associated with vocabulary and attention issues at the age of seven. Acta Paediat. (2016) 105:60–8. doi: 10.1111/apa.13094
12. Rose SA, Feldman JF, Jankowski JJ. Modeling a cascade of effects: the role of speed and executive functioning in preterm/full-term differences in academic achievement: executive function in preterm adolescents. Develop Sci. (2011) 14:1161–75. doi: 10.1111/j.1467-7687.2011.01068.x
13. Loe IM, Lee ES, Luna B, Feldman HM. Executive function skills are associated with reading and parent-rated child function in children born prematurely. Early Hum Develop. (2012) 88:111–8. doi: 10.1016/j.earlhumdev.2011.07.018
14. de Kieviet JF, van Elburg RM, Lafeber HN, Oosterlaan J. Attention Problems of Very Preterm Children Compared with Age-Matched Term Controls at School-Age. J Pediat. (2012) 161:824–9. doi: 10.1016/j.jpeds.2012.05.010
15. Pizzo R, Urben S, Van Der Linden M, Borradori-Tolsa C, Freschi M, Forcada-Guex M, et al. Attentional networks efficiency in preterm children. J Int Neuropsychol Soc. (2010) 16:130–7. doi: 10.1017/S1355617709991032
16. Giordano V, Fuiko R, Leiss U, Brandstetter S, Hayde M, Bartha-Doering E, et al. Differences in attentional functioning between preterm and full-term children underline the importance of new neuropsychological detection techniques. Acta Paediat. (2017) 106:601–11. doi: 10.1111/apa.13723
17. Geldof CJ, de Kieviet JF, Dik M, Kok JH, van Wassenaer-Leemhuis AG, Oosterlaan J. Visual search and attention in five-year-old very preterm/very low birth weight children. Early Hum Develop. (2013) 89:983–8. doi: 10.1016/j.earlhumdev.2013.08.021
18. Mulder H, Pitchford NJ, Hagger MS, Marlow N. Development of executive function and attention in preterm children: a systematic review. Develop Neuropsychol. (2009) 34:393–421. doi: 10.1080/87565640902964524
19. Réveillon M, Hüppi PS, Barisnikov K. Inhibition difficulties in preterm children: developmental delay or persistent deficit? Child Neuropsychol. (2018) 24:734–62. doi: 10.1080/09297049.2017.1294665
20. Breeman LD, Jaekel J, Baumann N, Bartmann P, Wolke D. Attention problems in very preterm children from childhood to adulthood: the bavarian longitudinal study. J Child Psychol Psychiat. (2016) 57:132–40. doi: 10.1111/jcpp.12456
21. Murray AL, Thompson DK, Pascoe L, Leemans A, Inder TE, Doyle LW, et al. White matter abnormalities and impaired attention abilities in children born very preterm. NeuroImage. (2016) 124:75–84. doi: 10.1016/j.neuroimage.2015.08.044
22. Posner MI, Petersen SE. The attention system of the human brain. Ann Rev Neurosci. (1990) 13:25–42. doi: 10.1146/annurev.ne.13.030190.000325
23. Raz A, Buhle J. Typologies of attentional networks. Nat Rev Neurosci. (2006) 7:367–79. doi: 10.1038/nrn1903
24. Rueda MR, Posner MI. Development of Attention Networks. In: The Oxford Handbook of Developmental Psychology (vol 1): Body and Mind. Oxford, UK: Oxford University Press (2013). p. 683–705.
25. Rueda MR, Fan J, McCandliss BD, Halparin JD, Gruber DB, Lercari LP, et al. Development of attentional networks in childhood. Neuropsychologia. (2004) 42:1029–40. doi: 10.1016/j.neuropsychologia.2003.12.012
26. Mezzacappa E. Alerting, orienting, and executive attention: developmental properties and sociodemographic correlates in an epidemiological sample of young, urban children. Child Develop. (2004) 75:1373–86. doi: 10.1111/j.1467-8624.2004.00746.x
27. Ishigami Y, Klein RM. Repeated measurement of the components of attention with young children using the attention network test: stability, isolability, robustness, and reliability. J Cogn Develop. (2015) 16:144–59. doi: 10.1080/15248372.2013.803971
28. Lewis FC, Reeve RA, Johnson KA. A longitudinal analysis of the attention networks in 6-to 11-year-old children. Child Neuropsychol. (2018) 24:145–65. doi: 10.1080/09297049.2016.1235145
29. Mullane JC, Lawrence MA, Corkum PV, Klein RM, McLaughlin EN. The development of and interaction among alerting, orienting, and executive attention in children. Child Neuropsychol. (2016) 22:155–76. doi: 10.1080/09297049.2014.981252
30. Pozuelos JP, Paz-Alonso PM, Castillo A, Fuentes LJ, Rueda MR. Development of attention networks and their interactions in childhood. Develop Psychol. (2014) 50:2405–15. doi: 10.1037/a0037469
31. Fan J, McCandliss BD, Sommer T, Raz A, Posner MI. Testing the efficiency and independence of attentional networks. J Cogn Neurosci. (2002) 14:340–7. doi: 10.1162/089892902317361886
32. Rosenbaum J, Hascoët JM, Hamon I, Petel A, Caudron S, Ceyte H. Body mobility and attention networks in 6-to 7-year-old children. Front Psychol. (2021) 12:743504. doi: 10.3389/fpsyg.2021.743504
33. Eaton WO, McKeen NA, Campbell DW. The waxing and waning of movement: implications for psychological development. Develop Rev. (2001) 21:205–23. doi: 10.1006/drev.2000.0519
34. Krasner AJ, Turner JB, Feldman JF, Silberman AE, Fisher PW, Workman CC, et al. ADHD symptoms in a non-referred low birthweight/preterm cohort: longitudinal profiles, outcomes, and associated features. J Attent Disord. (2018) 22:827–38. doi: 10.1177/1087054715617532
35. de Greeff JW, Bosker RJ, Oosterlaan J, Visscher C, Hartman E. Effects of physical activity on executive functions, attention and academic performance in preadolescent children: a meta-analysis. J Sci Med Sport. (2018) 21:501–7. doi: 10.1016/j.jsams.2017.09.595
36. Janssen M, Toussaint HM, van Mechelen W, Verhagen EA. Effects of acute bouts of physical activity on children’s attention: a systematic review of the literature. Springerplus. (2014) 3:410. doi: 10.1016/j.mhpa.2014.07.001
37. Abou Khalil G, Doré-Mazars K, Senot P, Wang DP, Legrand A. Is it better to sit down, stand up or walk when performing memory and arithmetic activities? Exp Brain Res. (2020) 238:2487–96. doi: 10.1007/s00221-020-05858-z
38. Barra J, Auclair L, Charvillat A, Vidal M, Pérennou D. Postural control system influences intrinsic alerting state. Neuropsychology. (2015) 29:226–34. doi: 10.1037/neu0000174
39. Caldwell JA, Prazinko B, Caldwell JL. Body posture affects electroencephalographic activity and psychomotor vigilance task performance in sleep-deprived subjects. Clin Neurophysiol. (2003) 114:23–31. doi: 10.1016/S1388-2457(02)00283-3
40. Smith KC, Davoli CC, Knapp WH, Abrams RA. Standing enhances cognitive control and alters visual search. Attent Percept Psychophys. (2019) 81:2320–9. doi: 10.3758/s13414-019-01723-6
41. Rosenbaum D, Mama Y, Algom D. Stand by your Stroop: standing up enhances selective attention and cognitive control. Psychol Sci. (2017) 28:1864–7. doi: 10.1177/0956797617721270
42. Caron EE, Reynolds MG, Ralph BCW, Carriere JSA, Besner D, Smilek D. Does posture influence the stroop effect? Psychol Sci. (2020) 31:1452–60. doi: 10.1177/0956797620953842
43. Straub ER, Dames H, Kiesel A, Dignath D. Does body posture reduce the Stroop effect? Evidence from two conceptual replications and a meta-analysis. Acta Psychol. (2022) 224:103497. doi: 10.1016/j.actpsy.2022.103497
44. Rapport MD, Bolden J, Kofler MJ, Sarver DE, Raiker JS, Alderson RM. Hyperactivity in boys with attention-deficit/hyperactivity disorder (ADHD): a ubiquitous core symptom or manifestation of working memory deficits? J Abnorm Child Psychol. (2009) 37:521–34. doi: 10.1007/s10802-008-9287-8
45. Patros CHG, Alderson RM, Hudec KL, Tarle SJ, Lea SE. Hyperactivity in boys with attention-deficit/hyperactivity disorder: the influence of underlying visuospatial working memory and self-control processes. J Exp Child Psychol. (2017) 154:1–12. doi: 10.1016/j.jecp.2016.09.008
46. Thibault RT, Raz A. Imaging posture veils neural signals. Front Hum Neurosci. (2016) 10:520. doi: 10.3389/fnhum.2016.00520
47. Schaefer S, Lövdén M, Wieckhorst B, Lindenberger U. Cognitive performance is improved while walking: differences in cognitive–sensorimotor couplings between children and young adults. Eur J Develop Psychol. (2010) 7:371–89. doi: 10.1080/17405620802535666
48. Ceyte H, Rosenbaum J, Hamon I, Wirth M, Caudron S, Hascoët JM. Mobility may impact attention abilities in healthy term or prematurely born children at 7-years of age: protocol for an intervention controlled trial. BMC Pediat. (2018) 18:264. doi: 10.1186/s12887-018-1229-1
49. Schneider BA, Avivi-Reich M, Mozuraitis M. A cautionary note on the use of the analysis of covariance (ANCOVA) in classification designs with and without within-subject factors. Front Psychol. (2015) 6:474. doi: 10.3389/fpsyg.2015.00474
50. Olivier I, Cuisinier R, Vaugoyeau M, Nougier V, Assaiante C. Age-related differences in cognitive and postural dual-task performance. Gait Post. (2010) 32:494–9. doi: 10.1016/j.gaitpost.2010.07.008
51. Zhu JL, Olsen J, Olesen AW. Risk for developmental coordination disorder correlates with gestational age at birth. Paediat Perinat Epidemiol. (2012) 26:572–7. doi: 10.1111/j.1365-3016.2012.01316.x
52. van Hoorn JF, Schoemaker MM, Stuive I, Dijkstra PU, Rodrigues Trigo Pereira F, Van der Sluis CK, et al. Risk factors in early life for developmental coordination disorder: a scoping review. Develop Med Child Neurol. (2021) 63:511–9. doi: 10.1111/dmcn.14781
53. de Rose P, Albamonte E, Laganà V, Sivo S, Pisoni S, Gallini F, et al. Perceptual-motor abilities in pre-school preterm children. Early Hum Develop. (2013) 89:809–14. doi: 10.1016/j.earlhumdev.2013.07.001
54. de Kieviet JF, Piek JP, Aarnoudse-Moens CS, Oosterlaan J. Motor development in very preterm and very low-birth-weight children from birth to adolescence: a meta-analysis. JAMA. (2009) 302:2235–42. doi: 10.1001/jama.2009.1708
55. de Kieviet JF, Stoof CJJ, Geldof CJA, Smits N, Piek JP, Lafeber HN, et al. The crucial role of the predictability of motor response in visuomotor deficits in very preterm children at school age. Develop Med Child Neurol. (2013) 55:624–30. doi: 10.1111/dmcn.12125
56. Bolk J, Farooqi A, Hafström M, Åden U, Serenius F. Developmental coordination disorder and its association with developmental comorbidities at 6.5 years in apparently healthy children born extremely preterm. JAMA Pediat. (2018) 172:765–74. doi: 10.1001/jamapediatrics.2018.1394
57. Marlow N, Hennessy EM, Bracewell MA, Wolke D, EPICure Study Group. Motor and executive function at 6 years of age after extremely preterm birth. Pediatrics. (2007) 120:793–804. doi: 10.1542/peds.2007-0440
58. Rodríguez Fernández C, Mata Zubillaga D, Rodríguez Fernández LM, Regueras Santos L, Reguera García MM, de Paz Fernández JA, et al. Evaluation of coordination and balance in preterm children. An Pediatr. (2016) 85:86–94. doi: 10.1016/j.anpede.2015.10.023
59. Lorefice LE, Galea MP, Clark RA, Doyle LW, Anderson PJ, Spittle AJ. Postural control at 4 years in very preterm children compared with term-born peers. Develop Med Child Neurol. (2015) 57:175–80. doi: 10.1111/dmcn.12550
60. Bucci MP, Tringali M, Trousson C, Husson I, Baud O, Biran V. Spatial and temporal postural analysis in children born prematurely. Gait Post. (2017) 57:230–5. doi: 10.1016/j.gaitpost.2017.06.023
61. Bucci MP, Wiener-Vacher S, Trousson C, Baud O, Biran V. Subjective visual vertical and postural capability in children born prematurely. PLoS One. (2015) 10:e0121616. doi: 10.1371/journal.pone.0121616
62. Kluenter H, Roedder D, Kribs A, Fricke O, Roth B, Guntinas-Lichius O. Postural control at 7 years of age after preterm birth with very low birth weight. Otol Neurotol. (2008) 29:1171–5. doi: 10.1097/MAO.0b013e31818a0f5c
63. Aarnoudse-Moens CS, Smidts DP, Oosterlaan J, Duivenvoorden HJ, Weisglas-Kuperus N. Executive function in very preterm children at early school age. J Abnorm Child Psychol. (2009) 37:981–93. doi: 10.1007/s10802-009-9327-z
64. Huertas F, Zahonero J, Sanabria D, Lupiáñez J. Functioning of the attentional networks at rest vs. during acute bouts of aerobic exercise. J Sport Exerc Psychol. (2011) 33:649–65. doi: 10.1123/jsep.33.5.649
65. Assaiante C, Amblard B. An ontogenetic model for the sensorimotor organization of balance control in humans. Hum Move Sci. (1995) 14:13–43. doi: 10.1016/0167-9457(94)00048-J
66. Bonnet CT, Baudry S. Active vision task and postural control in healthy, young adults: synergy and probably not duality. Gait Post. (2016) 48:57–63. doi: 10.1016/j.gaitpost.2016.04.016
Keywords: prematurity, posture, body mobility, alertness, orienting, executive control
Citation: Rosenbaum J, Ceyte H, Hamon I, Deforge H, Hascoët AMJ, Caudron S and Hascoët J-M (2022) Influence of body mobility on attention networks in school-aged prematurely born children: A controlled trial. Front. Pediatr. 10:928541. doi: 10.3389/fped.2022.928541
Received: 25 April 2022; Accepted: 17 August 2022;
Published: 08 September 2022.
Edited by:
Francesca Tinelli, IRCCS Stella Maris Foundation, ItalyReviewed by:
Daniela Ricci, Polo Nazionale di Servizi e Ricerca per la Prevenzione della Cecità e la Riabilitazione Visiva degli Ipovedenti, ItalyGiulia Purpura, University of Milano-Bicocca, Italy
Copyright © 2022 Rosenbaum, Ceyte, Hamon, Deforge, Hascoët, Caudron and Hascoët. This is an open-access article distributed under the terms of the Creative Commons Attribution License (CC BY). The use, distribution or reproduction in other forums is permitted, provided the original author(s) and the copyright owner(s) are credited and that the original publication in this journal is cited, in accordance with accepted academic practice. No use, distribution or reproduction is permitted which does not comply with these terms.
*Correspondence: Jean-Michel Hascoët, amVhbi1taWNoZWwuaGFzY29ldEB1bml2LWxvcnJhaW5lLmZy