- 1Department of Pediatrics, Faculty of Medicine Siriraj Hospital, Mahidol University, Bangkok, Thailand
- 2Division of Pulmonology, Department of Pediatrics, Faculty of Medicine Siriraj Hospital, Mahidol University, Bangkok, Thailand
- 3Department of Otorhinolaryngology, Faculty of Medicine Siriraj Hospital, Mahidol University, Bangkok, Thailand
- 4Department of Medicine, Faculty of Medicine Siriraj Hospital, Mahidol University, Bangkok, Thailand
- 5Siriraj Sleep Center, Faculty of Medicine Siriraj Hospital, Mahidol University, Bangkok, Thailand
Objectives: Different pathophysiological mechanisms and the distribution of respiratory events among rapid eye movement (REM) and non-rapid eye movement (NREM) sleep modulate the effect of obstructive sleep apnea (OSA). We aimed to study the prevalence and risk factors for REM-related OSA in children.
Study Design: Retrospective, cross-sectional study.
Methods: We recruited 366 children with OSA confirmed by polysomnography (PSG) over a 5-year period. REM-related OSA is defined by an obstructive apnea-hypopnea index (OAHI) in the REM sleep ≥2× than during NREM sleep.
Results: The prevalence of REM-related OSA in children was 50.3%. Children with REM-related OSA were more likely to be female (P = 0.042), and had lower prevalence of adenotonsillar hypertrophy (P = 0.043) compared with children with other OSA subtypes. Children with REM-related OSA slept longer in the supine position (P = 0.003), had shorter duration of NREM1 sleep (P = 0.018), lower nadir SpO2 (P = 0.005), and a higher oxygen desaturation index 3% (ODI3%) (P = 0.014), and lower arousal index (P = 0.034) compared with other OSA subtypes. Female gender and supine sleep was the independent risk factors for REM-related OSA.
Conclusion: The prevalence of REM-related OSA was 50.3%. OAHIREM should be considered as an important parameter in future clinical research studies done in children with OSA.
Introduction
Snoring is common in children. Obstructive sleep apnea (OSA) in children usually presents with habitual snoring, defined by snoring more than three nights/week. OSA arises from recurrent episodes of airway collapse during sleep that disrupts sleep architecture, ventilation, and cardiovascular homeostasis which leads to many consequences (1). Adverse consequences of pediatric OSA include neurobehavioral, cardiovascular, and endocrine consequences, which adversely affect the quality of life (2–5). The prevalence of OSA in children may be increasing due to the increasing prevalence of obesity and improved survival of children with chronic diseases such as neuromuscular disorders (6). Furthermore, obese children also have reduced REM sleep; this affects energy balance regulation and predisposes them to additional weight gain (7).
In America, the prevalence of habitual snoring has been reported to be 2.4–17.2%, and the prevalence of obstructive sleep apnea (OSA) was 1.2–5.7% (8). In Thailand in 2005, the prevalence of habitual snoring was reported to be 6.9–8.5%, and the prevalence of OSA was 0.7–1.3% (9). In 2016, the prevalence of OSA in children who underwent diagnostic polysomnography (PSG) at Chulalongkorn Memorial Hospital in Thailand was 92.7% and 40.4% of these children had severe OSA (10).
Human sleep is divided into four stages defined by electroencephalographic criteria. The main sleep stages include rapid eye movement (REM) sleep and non-REM (NREM) sleep (11). In children, OSA occurs predominantly in REM sleep (12, 13) possibly due to the reduced muscle tone, blunted arousal and ventilatory responses during this sleep stage (14, 15). Previous studies reported the prevalence of REM-predominant OSA ranging from 69.6 to 74.7%, while NREM-predominant OSA accounts for 16.7–25.3% of childhood and adolescent OSA (12, 13, 16, 17).
According to polysomnographic phenotypes, REM-related OSA is defined as a ratio of >2 OAHI in REM sleep (OAHIREM) compared with OAHI in non-REM (NREM) sleep (OAHINREM) (18–20). The difference of pathophysiologic factors would predispose to airway obstruction in different sleep stages. These factors include upper airway collapsibility and muscle responsiveness, ventilatory control stability, and arousal threshold. All these factors change with age and disease status and may influence the pattern of polysomnographic phenotypes across age groups (21, 22). Sleep stage affects OSA severity and is influenced by many factors such as comorbidities including allergic rhinitis, asthma, and hypertension (23–27) but the natural history and clinical significance of sleep stage-dependent OSA have not been fully assessed. Because REM sleep plays a role in memory formation, processing of emotional information, neuronal plasticity and excitability (28). Therefore, respiratory disturbances in this sleep stages will have varying consequences, which are not rely only on an overall OAHI. Hence, we aimed to study the prevalence and risk factors for REM-related OSA in children.
Materials and Methods
This retrospective cross-sectional study included children less than 18 years of age who underwent diagnostic polysomnography (PSG) for suspected OSA at the Siriraj Sleep Center between 2016 and 2020. OSA was diagnosed using clinical symptoms such as snoring, labored, paradoxical or obstructed breathing during sleep, sleepiness, hyperactivity, behavioral or learning problems, and an apnea-hypopnea index (AHI) from diagnostic polysomnography equal or more than 1 episode/h (29). We excluded children who had REM sleep less than 30 min during the diagnostic PSG. Before commencement of this study, the research protocol was approved by the Siriraj Institutional Review Board (approval no. Si 246/2563).
We collected demographic data from electronic medical records and PSG parameters from the official report. Weight was defined according to percentile weight for height-based and body mass index (BMI) z-score by age and gender. Overweight was defined as percentile weight for height greater than 120, or BMI z-score greater than or equal to 1. Obesity was defined as percentile weight for height greater than 140 or BMI z-score greater than or equal to two. Comorbidities and consequences of OSA were collected including adenotonsillar hypertrophy, allergic rhinitis, asthma, Down syndrome, neuromuscular disease, systemic hypertension, pulmonary hypertension, learning disorder, and attention deficit hyperactivity disorder (ADHD).
All PSG were scored by sleep specialists who were blinded to the condition of the child. Based on the PSG results, children were divided into three groups according to the polysomnographic phenotypes (23). REM-related and NREM-related OSA were defined as a ratio of OAHI in REM sleep (OAHIREM) to OAHI in NREM sleep (OAHINREM) of >2 and <0.5, respectively. Children who did not meet these criteria were classified as stage-independent OSA. The OSA severity was classified based on OAHI: (1) mild OSA (AHI 1 to ≤5 events per hour), (2) moderate OSA (AHI > 5 to ≤10 events per hour), and (3) severe OSA (AHI > 10 events per hour). In addition, positional OSA was defined by OAHI in the supine position ≥2 × OAHI in the non-supine position (30).
Normally distributed data were expressed as mean and standard deviation, non-normally distributed data were expressed as median and interquartile range (25th, 75th percentile). Categorical data were expressed as frequency and percentage. Groups were compared using the unpaired t-test or Mann–Whitney U test, while categorical data were compared using the chi-squared test. Data were analyzed using IBM SPSS Statistics for Windows (version 22.0; IBM Corp., Armonk, NY, United States). A P value of <0.05 was considered statistically significant.
Binary logistic regression was performed to identify independent risk factors associated with REM-related OSA. The possible confounders with P < 0.2 in crude odd ratio (gender, adenotonsillar hypertrophy, asthma, supine total sleep time, NREM 1 sleep), allergic rhinitis, and OSA severity (OAHI) were analyzed to estimate an adjusted odd ratio.
Results
Three hundred and sixty-six children were included in the analysis. One hundred and eighty-four (50.3%) children had REM-related OSA, 150 (41.0%) children had sleep stage independent OSA, and 32 (8.7%) children had NREM-related OSA. The median (interquartile range: IQR) age of the children was 8.0 (5.0, 12.0) years, 245 (66.9%) were male,%weight for height 121.00% (100.0%, 161.5%), and the BMI z-score was 1.4 (±2.5). Fifty-six children (15.3%) were overweight and 131 children (35.8%) were obese. Most children also had comorbidities. Common comorbidities included adenotonsillar hypertrophy 210 (57.4%), allergic rhinitis 130 (35.5%), asthma 39 (10.7%), Down syndrome 25 (6.8%), and neuromuscular disease 41 (11.2%). There was significant difference only in the prevalence of adenotonsillar hypertrophy by OSA subtype. Many children also had OSA consequences. Common consequences included attention deficit hyperactivity disorder (ADHD) 41 (11.2%), pulmonary hypertension 23 (6.3%), learning disorder 23 (6.3%), and systemic hypertension 18 (4.9%). There was a lower trend of children with REM-related OSA had systemic hypertension, but more of these children had pulmonary hypertension and learning disorders. Hence, there was no significant difference in the prevalence of consequences among each OSA subtypes (P = 0.864, 0.257 and 0.545, respectively) (Table 1).
Ninety-two (25.1%) children had mild OSA, 121 (33.1%) had moderate OSA, and 153 (41.8%) had severe OSA. The OSA severity and proportion of children with positional OSA was not significantly different between REM-related OSA and other OSA subtypes (Table 2).
Children with REM-related OSA were more likely to be female (P = 0.042) and had lower prevalence of adenotonsillar hypertrophy (P = 0.043) compared with children with other subtypes. The other comorbidities and consequences were not significantly different in children with REM-related OSA compared with other subtypes (Table 3).
Children with REM-related OSA slept longer in the supine position (P = 0.003), had shorter duration of NREM1 sleep (P = 0.018), lower nadir SpO2 (P = 0.005) and higher oxygen desaturation index 3% (ODI3%) (P = 0.014), and lower arousal index (P = 0.034) compared with children with other subtypes (Table 4).
After adjusting for covariables, we found female gender was a significant risk factor for REM-related OSA with an adjusted odds ratio of 1.874 (95% CI, 1.171–2.999) (P = 0.009). Duration of supine sleep was also another significant risk factor for REM-related OSA with an adjusted odds ratio of 1.003 (95% CI, 1.001–1.005) (P = 0.005) (Table 5).
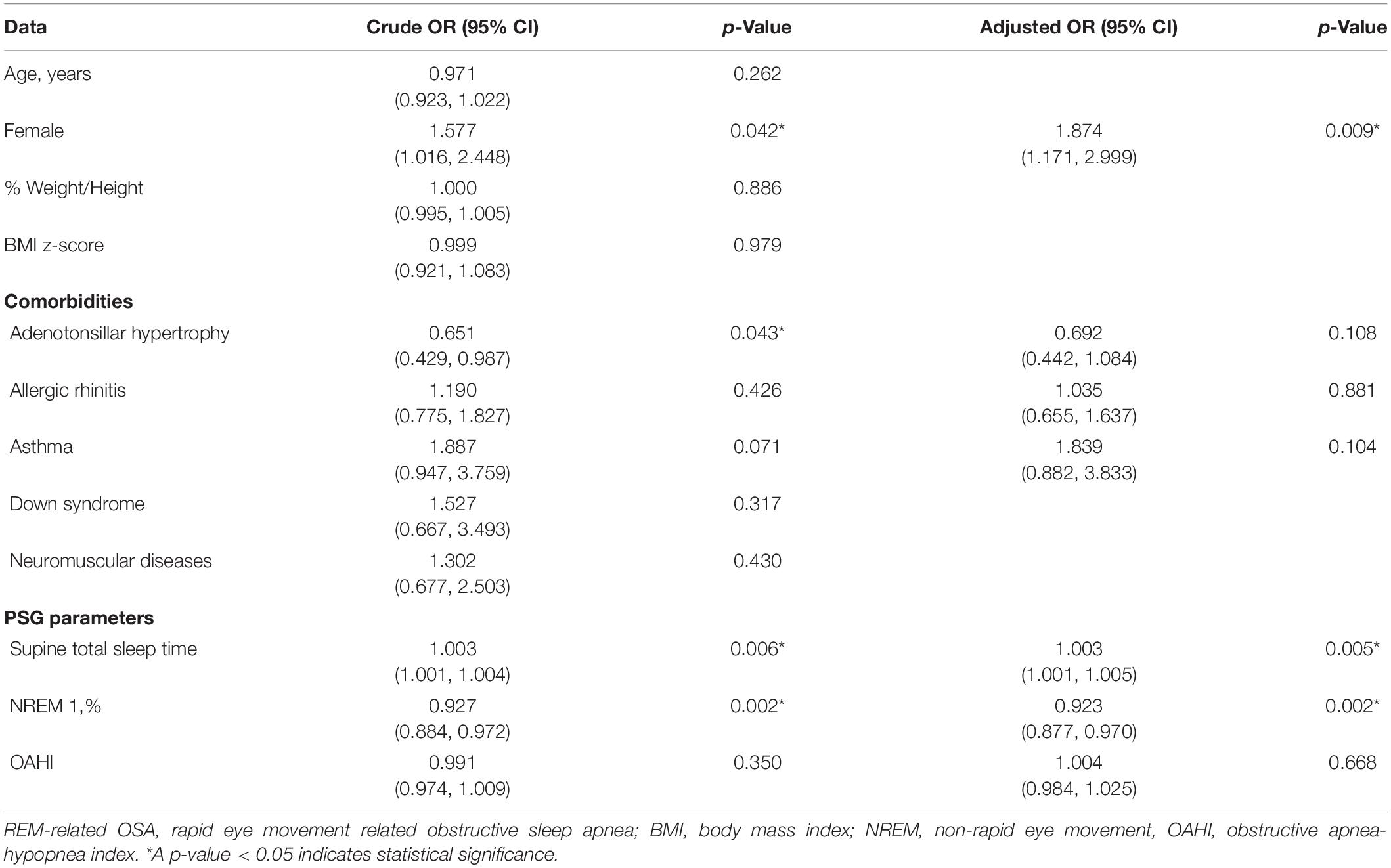
Table 5. Odd ratio, 95% confidence interval and p-value of REM-related OSA and other subtypes for demographic data, comorbidity, consequence, and polysomnographic parameters.
Discussion
The prevalence of REM-related OSA in our study was 50.3%, lower than previous study which reported at 65% (17). In the past, there was significant heterogeneity in defining REM-predominant OSA. Previous studies have reported 69% of OSA children had higher OAHIREM than OAHINREM (12, 13), and 55% of obstructive apneas in children occurred during REM sleep (31). Goh et al. reported that the apnea index, apnea duration and degree of desaturation in REM sleep were greater than during NREM sleep (31). Withdrawal of excitatory noradrenergic and serotonergic inputs to pharyngeal motor neurons during REM sleep predisposes to upper airway collapse (32). OSA is particularly problematic in REM sleep in children due to muscle atonia, reduced ventilatory response to hypoxia and hypercapnia, and reduced arousal threshold (33).
In adults, the overall AHI is more influenced by the NREM AHI because of longer duration of NREM sleep. Moreover, as the severity of OSA increases, the duration of REM sleep reduces (34). In adults with OSA, the prevalence of REM-related OSA is 10–36% (18–20) and respiratory events with more severe clinical phenotypes occur predominantly during NREM sleep (35–37). Severe sleep fragmentation is characteristic of this subtype (38) in contrast to REM-predominant type in children where sleep architecture is reported to be preserved (31). This pattern was also reported in up to a third of children with moderate to severe OSA, especially in older children with high arousal indices and less severe desaturations with events (13). Thus, different pathophysiological mechanisms will be operational in these two OSA subtypes. Hormonal changes during puberty or thereafter might lead to the reversal of REMS-NREMS OSA predominance. However, recent study revealed that the majority (72%) of children with REM-related OSA at baseline and persistent OSA at 10-year follow-up remained to have REM-related OSA (17). Further study of the longitudinal changes during the transition to adulthood may shed light on this issue, particularly considering the higher risk of OSA recurrence after adolescence in children who were previously diagnosed and treated for OSA during childhood (39).
We observed that OSA severity was not significantly different by OSA subtype nor was the proportion of children with positional OSA. Positional (supine dependent) OSA is less common in children compared to adults. It is defined by an OAHI in the supine position ≥2 × OAHI in the non-supine position. Previous study reported the prevalence of positional OSA in children at 18.7%. They also found children with positional OSA were significantly older, had a high prevalence of obesity, and a lower tonsil score (30). We found the prevalence of positional OSA at 42.3%, much higher than previous study. It may be affected by the high prevalence of obese children in our study. Although OSA worsens during REM sleep, this was not observed for positional OSA. Furthermore, our study also found no difference in the proportion of positional OSA between children with REM-related OSA and others.
Individual variations in endotypes that predispose to airway obstruction in different sleep stages may affect polysomnographic phenotypes. A recent study revealed that individuals with REM-related OSA showed a significantly more collapsible airway in REM compared with NREM sleep, while individuals with NREM-related OSA had a higher loop gain and lower ventilatory drive during obstructed breathing at arousal threshold during NREM sleep (21).
The effect of sleep stage on OSA severity might be influenced by many factors such as body weight and by comorbidities including obesity, allergic rhinitis and asthma (24–26). Obese children have reduced REM sleep; this affects energy balance regulation and predisposes them to additional weight gain (7). REM sleep also influences upper and lower airway disorders in children. Children with rhinitis and OSA tend to have more REM-related upper airway obstruction (OAHIREM), and those with asthma and OSA seem to have more REM-related hypoxemia (25).
Our study showed that the prevalence of asthma in children with REM related-OSA tended to be higher than other subtypes, but did not reach statistical significance. Gutierrez et al. reported that asthma is associated with REM-related breathing abnormalities in children with moderate to severe OSA, and the link between asthma and REM-related OSA is independent of asthma control and obesity (24). OSA is also associated with nasal inflammatory changes (40). Although REM sleep is characterized by significant nasal congestion (41, 42) and children with rhinitis have more REM-related upper airway obstruction independent of confounding factors (25), we did not observe a meaningful difference in the proportion of children with allergic rhinitis between REM-related OSA and other subtypes. In contrast, Huseni et al. found that children with rhinitis have more REM-related OSA independent of age, BMI, gender, ethnicity and atopic status (25). Furthermore, we found that the prevalence of children with adenotonsillar hypertrophy in REM-related OSA was significantly lower than with other subtypes.
The different functions of REM and NREM sleep might result in different clinical outcomes. The distribution of respiratory events among REM and NREM sleep may modulate the effect of OSA in children. As apnea tends to be longer and desaturation more severe in REM sleep, we hypothesize that the consequences of OSA may be different if the events occur predominantly in REM sleep. At birth, a term infant has equal proportions of REM and NREM sleep. REM sleep duration decreases with increasing age. The proportion of REM sleep at 3–5 years of age is 25% that of adults (43). Therefore, children with obstructive events predominantly in REM sleep are more vulnerable to adverse neurocognitive outcomes for a given OAHI.
The clinical significance of REM-related OSA is controversial. Most adult studies demonstrate that REM-predominant OSA is associated with excessive daytime sleepiness, non-adherence to continuous positive airway pressure, depression, hypertension, non-dipping of nocturnal blood pressure, increased insulin resistance, and impaired spatial navigational memory (44–51). However, results are not consistent across all studies. Some studies also suggest that OAHINREM is more often associated with adverse consequences than OAHIREM (37, 52–54). REM sleep is important for memory consolidation and learning (55, 56). Therefore, a higher proportion of respiratory events in REM sleep may be linked to adverse neurocognitive outcomes of OSA.
Pediatric OSA is associated with elevated blood pressure (57–60); thus, the association between blood pressure and REM-related OSA in children is controversial (23, 27). Moderate to severe OSA has a significant effect on pulse transit time during REM sleep, suggesting that these children may have a higher baseline blood pressure during this state (27). In contrast, Au et al. found children with REM-related OSA had a significantly lower daytime and nighttime systolic blood pressure (SBP) than children with stage independent OSA (23). Moreover, OAHINREM, but not OAHIREM, was significantly associated with both daytime and nighttime SBP after controlling for age, gender, and body size. They postulated children with obstructive events mainly in REM sleep may have less cardiovascular complications than those with stage-independent OSA (23). However, recent study revealed that REM-related OSA was associated with higher nocturnal BP and a lesser degree of nocturnal SBP dipping in children (17). In our study, we did not find significant differences in the proportions of children with systemic hypertension between OSA subgroups. REM sleep is associated with greater sympathetic activity and cardiovascular instability than NREM sleep in both healthy subjects and patients with OSA (61). Therefore, respiratory events during REM sleep in REM-related OSA could play an important role in conferring a higher cardiovascular risk (44).
We observed that children with REM-related OSA had longer sleep in the supine position, shorter sleep duration in NREM1, and lower SpO2 nadir and higher ODI3% than children with other OSA subtypes. The severity of intermittent hypoxia would increase sympathetic activity, endothelial dysfunction, and systemic inflammation (62).
While our study confirms that OSA is predominantly a REM sleep-related disorder in the majority of children, we identified a significant proportion of other OSA subtypes. This difference of pathophysiology and outcomes has implications for diagnostic monitoring. Logistic regression analysis revealed that female gender and supine sleep was a significant predictor of REM-related OSA after adjustment for other confounders.
The strengths of our study are the relatively large sample size and the use of objective data to evaluate OSA variables. Our findings highlight the importance of sleep stage distribution of respiratory events in studies of the pathophysiology and outcomes of OSA in children. All PSG were scored by sleep specialists who were blinded to the condition of the child. However, the cross-sectional design of our study prevents us from arriving at any type of cause-effect conclusion. Follow-up is needed to know the treatment effect on REM-related OSA and whether it is similar to the NREM-related OSA subgroup or not. Therefore, we recommended further study in specific population at risk of REM-related OSA to confirm our hypothesis. New insights may allow for the discovery of mechanistic pathways that connect with sleep biology, which in turn, may result in novel strategies for the treatment of pediatric OSA.
Conclusion
The prevalence of REM-related OSA in children from our study was 50.3%. Female gender and supine sleep were independent risk factors for REM-related OSA in children. OAHIREM should be considered as an important parameter in future clinical research studies done in children with OSA.
Data Availability Statement
The raw data supporting the conclusions of this article will be made available by the authors, without undue reservation.
Ethics Statement
The studies involving human participants were reviewed and approved by the Siriraj Institutional Review Board. Written informed consent from the participants’ legal guardian/next of kin was not required to participate in this study in accordance with the national legislation and the institutional requirements.
Author Contributions
SC performed data collection, statistical analysis, and manuscript preparation. PT contributed to study conception, study design, statistical analysis, and manuscript preparation. AT and WC critically revised the manuscript for important intellectual content. All authors contributed to the article and approved the submitted version.
Conflict of Interest
The authors declare that the research was conducted in the absence of any commercial or financial relationships that could be construed as a potential conflict of interest.
Publisher’s Note
All claims expressed in this article are solely those of the authors and do not necessarily represent those of their affiliated organizations, or those of the publisher, the editors and the reviewers. Any product that may be evaluated in this article, or claim that may be made by its manufacturer, is not guaranteed or endorsed by the publisher.
Acknowledgments
We thank our colleagues from Siriraj Sleep Center who provided insight and expertise that greatly assisted the research. We gratefully acknowledge Siriraj Institute of Clinical Research (SICRES) for supporting the manuscript development, Mark Simmerman for editing the manuscript and Kanokwan Sommai for statistical analysis.
References
1. Katz ES, D’Ambrosio CM. Pathophysiology of pediatric obstructive sleep apnea. Proc Am Thorac Soc. (2008) 5:253–62.
2. Capdevila OS, Kheirandish-Gozal L, Dayyat E, Gozal D. Pediatric obstructive sleep apnea: complications, management, and long-term outcomes. Proc Am Thorac Soc. (2008) 5:274–82. doi: 10.1513/pats.200708-138MG
3. Gozal D, Brockmann PE, Alonso-Álvarez ML. Morbidity of pediatric obstructive sleep apnea in children: myth, reality, or hidden iceberg? Arch Bronconeumol (Engl Ed). (2018) 54:253–4. doi: 10.1016/j.arbres.2017.11.013
4. Hunter SJ, Gozal D, Smith DL, Philby MF, Kaylegian J, Kheirandish-Gozal L. Effect of sleep-disordered breathing severity on cognitive performance measures in a large community cohort of young school-aged children. Am J Respir Crit Care Med. (2016) 194:739–47. doi: 10.1164/rccm.201510-2099OC
5. Bhattacharjee R, Kheirandish-Gozal L, Pillar G, Gozal D. Cardiovascular complications of obstructive sleep apnea syndrome: evidence from children. Prog Cardiovasc Dis. (2009) 51:416–33. doi: 10.1016/j.pcad.2008.03.002
6. MacLean JE, DeHaan K, Chowdhury T, Nehme J, Bendiak GN, Hoey L, et al. The scope of sleep problems in Canadian children and adolescents with obesity. Sleep Med. (2018) 47:44–50. doi: 10.1016/j.sleep.2018.03.006
7. Jalilolghadr S, Yazdi Z, Mahram M, Babaei F, Esmailzadehha N, Nozari H, et al. Sleep architecture and obstructive sleep apnea in obese children with and without metabolic syndrome: a case control study. Sleep Breath. (2016) 20:845–51. doi: 10.1007/s11325-015-1291-y
8. Lumeng JC, Chervin RD. Epidemiology of pediatric obstructive sleep apnea. Proc Am Thorac Soc. (2008) 5:242–52.
9. Anuntaseree W, Kuasirikul S, Suntornlohanakul S. Natural history of snoring and obstructive sleep apnea in Thai school-age children. Pediatr Pulmonol. (2005) 39:415–20. doi: 10.1002/ppul.20207
10. Veeravigrom M, Desudchit T. Prevalence of sleep disorders in Thai children. Indian J Pediatr. (2016) 83:1237–41. doi: 10.1007/s12098-016-2148-5
12. Spruyt K, Gozal D. REM and NREM sleep-state distribution of respiratory events in habitually snoring school-aged community children. Sleep Med. (2012) 13:178–84. doi: 10.1016/j.sleep.2011.10.025
13. Verginis N, Jolley D, Horne RS, Davey MJ, Nixon GM. Sleep state distribution of obstructive events in children: is obstructive sleep apnoea really a rapid eye movement sleep-related condition? J Sleep Res. (2009) 18:411–4. doi: 10.1111/j.1365-2869.2009.00760.x
14. Katz ES, Marcus CL, White DP. Influence of airway pressure on genioglossus activity during sleep in normal children. Am J Respir Crit Care Med. (2006) 173:902–9. doi: 10.1164/rccm.200509-1450OC
15. Katz ES, White DP. Genioglossus activity during sleep in normal control subjects and children with obstructive sleep apnea. Am J Respir Crit Care Med. (2004) 170:553–60. doi: 10.1164/rccm.200403-262OC
16. El-Kersh K, Cavallazzi R, Patel PM, Senthilvel E. Effect of sleep state and position on obstructive respiratory events distribution in adolescent children. J Clin Sleep Med. (2016) 12:513–7. doi: 10.5664/jcsm.5678
17. Chan KC, Au CT, Yu MW, Wing YK, Li AM. Natural history of REM-OSA in children and its associations with adverse blood pressure outcomes: a longitudinal follow-up study. Nat Sci Sleep. (2021) 13:1967–84. doi: 10.2147/NSS.S331389
18. Haba-Rubio J, Janssens JP, Rochat T, Sforza E. Rapid eye movement-related disordered breathing: clinical and polysomnographic features. Chest. (2005) 128:3350–7. doi: 10.1378/chest.128.5.3350
19. Koo BB, Dostal J, Ioachimescu O, Budur K. The effects of gender and age on REM-related sleep-disordered breathing. Sleep Breath. (2008) 12:259–64. doi: 10.1007/s11325-007-0161-7
20. Koo BB, Patel SR, Strohl K, Hoffstein V. Rapid eye movement-related sleep-disordered breathing: influence of age and gender. Chest. (2008) 134:1156–61. doi: 10.1378/chest.08-1311
21. Joosten SA, Landry SA, Wong AM, Mann DL, Terrill PI, Sands SA, et al. Assessing the physiologic endotypes responsible for REM- and NREM-Based OSA. Chest. (2021) 159:1998–2007. doi: 10.1016/j.chest.2020.10.080
22. Eckert DJ, White DP, Jordan AS, Malhotra A, Wellman A. Defining phenotypic causes of obstructive sleep apnea. Identification of novel therapeutic targets. Am J Respir Crit Care Med. (2013) 188:996–1004. doi: 10.1164/rccm.201303-0448OC
23. Au CT, Ho CK, Wing YK, Li AM. The effect of childhood obstructive sleep apnea on ambulatory blood pressure is modulated by the distribution of respiratory events during rapid eye movement and nonrapid eye movement sleep. Sleep Med. (2013) 14:1317–22. doi: 10.1016/j.sleep.2013.09.017
24. Gutierrez MJ, Zhu J, Rodriguez-Martinez CE, Nino CL, Nino G. Nocturnal phenotypical features of obstructive sleep apnea (OSA) in asthmatic children. Pediatr Pulmonol. (2013) 48:592–600. doi: 10.1002/ppul.22713
25. Huseni S, Gutierrez MJ, Rodriguez-Martinez CE, Nino CL, Perez GF, Pancham K, et al. The link between rhinitis and rapid-eye-movement sleep breathing disturbances in children with obstructive sleep apnea. Am J Rhinol Allergy. (2014) 28:e56–61. doi: 10.2500/ajra.2014.28.3994
26. Baldassari CM, Alam L, Vigilar M, Benke J, Martin C, Ishman S. Correlation between REM AHI and quality-of-life scores in children with sleep-disordered breathing. Otolaryngol Head Neck Surg. (2014) 151:687–91. doi: 10.1177/0194599814547504
27. Nisbet LC, Yiallourou SR, Biggs SN, Nixon GM, Davey MJ, Trinder JA, et al. Preschool children with obstructive sleep apnea: the beginnings of elevated blood pressure? Sleep. (2013) 36:1219–26. doi: 10.5665/sleep.2890
28. Vyazovskiy VV, Delogu A. NREM and REM sleep: complementary roles in recovery after wakefulness. Neuroscientist. (2014) 20:203–19. doi: 10.1177/1073858413518152
29. Kaditis AG, Alonso Alvarez ML, Boudewyns A, Alexopoulos EI, Ersu R, Joosten K, et al. Obstructive sleep disordered breathing in 2- to 18-year-old children: diagnosis and management. Eur Respir J. (2016) 47:69–94. doi: 10.1183/13993003.00385-2015
30. Verhelst E, Clinck I, Deboutte I, Vanderveken O, Verhulst S, Boudewyns A. Positional obstructive sleep apnea in children: prevalence and risk factors. Sleep Breath. (2019) 23:1323–30. doi: 10.1007/s11325-019-01853-z
31. Goh DY, Galster P, Marcus CL. Sleep architecture and respiratory disturbances in children with obstructive sleep apnea. Am J Respir Crit Care Med. (2000) 162:682–6. doi: 10.1164/ajrccm.162.2.9908058
32. Fenik VB, Davies RO, Kubin L. REM sleep-like atonia of hypoglossal (XII) motoneurons is caused by loss of noradrenergic and serotonergic inputs. Am J Respir Crit Care Med. (2005) 172:1322–30. doi: 10.1164/rccm.200412-1750OC
33. Marcus CL, Lutz J, Carroll JL, Bamford O. Arousal and ventilatory responses during sleep in children with obstructive sleep apnea. J Appl Physiol. (1998) 84:1926–36. doi: 10.1152/jappl.1998.84.6.1926
34. Wali SO, Abaalkhail B, AlQassas I, Alhejaili F, Spence DW, Pandi-Perumal SR. The correlation between oxygen saturation indices and the standard obstructive sleep apnea severity. Ann Thorac Med. (2020) 15:70–5. doi: 10.4103/atm.ATM_215_19
35. Conwell W, Patel B, Doeing D, Pamidi S, Knutson KL, Ghods F, et al. Prevalence, clinical features, and CPAP adherence in REM-related sleep-disordered breathing: a cross-sectional analysis of a large clinical population. Sleep Breath. (2012) 16:519–26. doi: 10.1007/s11325-011-0537-6
36. Liu Y, Su C, Liu R, Lei G, Zhang W, Yang T, et al. NREM-AHI greater than REM-AHI versus REM-AHI greater than NREM-AHI in patients with obstructive sleep apnea: clinical and polysomnographic features. Sleep Breath. (2011) 15:463–70. doi: 10.1007/s11325-010-0358-z
37. Chami HA, Baldwin CM, Silverman A, Zhang Y, Rapoport D, Punjabi NM, et al. Sleepiness, quality of life, and sleep maintenance in REM versus non-REM sleep-disordered breathing. Am J Respir Crit Care Med. (2010) 181:997–1002. doi: 10.1164/rccm.200908-1304OC
38. Charbonneau M, Marin JM, Olha A, Kimoff RJ, Levy RD, Cosio MG. Changes in obstructive sleep apnea characteristics through the night. Chest. (1994) 106:1695–701. doi: 10.1378/chest.106.6.1695
39. Contencin P, Guilleminault C, Manach Y. Long-term follow-up and mechanisms of obstructive sleep apnea (OSA) and related syndromes through infancy and childhood. Int J Pediatr Otorhinolaryngol. (2003) 67:S119–23. doi: 10.1016/j.ijporl.2003.08.009
40. Koutsourelakis I, Vagiakis E, Perraki E, Karatza M, Magkou C, Kopaka M, et al. Nasal inflammation in sleep apnoea patients using CPAP and effect of heated humidification. Eur Respir J. (2011) 37:587–94. doi: 10.1183/09031936.00036910
41. Morris LG, Burschtin O, Setlur J, Bommelje CC, Lee KC, Jacobs JB, et al. REM-associated nasal obstruction: a study with acoustic rhinometry during sleep. Otolaryngol Head Neck Surg. (2008) 139:619–23. doi: 10.1016/j.otohns.2008.08.017
42. Kimura A, Chiba S, Capasso R, Yagi T, Ando Y, Watanabe S, et al. Phase of nasal cycle during sleep tends to be associated with sleep stage. Laryngoscope. (2013) 123:2050–5. doi: 10.1002/lary.23986
44. Alzoubaidi M, Mokhlesi B. Obstructive sleep apnea during rapid eye movement sleep: clinical relevance and therapeutic implications. Curr Opin Pulm Med. (2016) 22:545–54. doi: 10.1097/MCP.0000000000000319
45. Kass JE, Akers SM, Bartter TC, Pratter MR. Rapid-eye-movement-specific sleep-disordered breathing: a possible cause of excessive daytime sleepiness. Am J Respir Crit Care Med. (1996) 154:167–9. doi: 10.1164/ajrccm.154.1.8680674
46. Lee SA, Paek JH, Han SH. REM-related sleep-disordered breathing is associated with depressive symptoms in men but not in women. Sleep Breath. (2016) 20:995–1002. doi: 10.1007/s11325-016-1323-2
47. Varga AW, Kishi A, Mantua J, Lim J, Koushyk V, Leibert DP, et al. Apnea-induced rapid eye movement sleep disruption impairs human spatial navigational memory. J Neurosci. (2014) 34:14571–7. doi: 10.1523/JNEUROSCI.3220-14.2014
48. Hoshino T, Sasanabe R, Tanigawa T, Murotani K, Arimoto M, Ueda H, et al. Effect of rapid eye movement-related obstructive sleep apnea on adherence to continuous positive airway pressure. J Int Med Res. (2018) 46:2238–48. doi: 10.1177/0300060518758583
49. Mokhlesi B, Hagen EW, Finn LA, Hla KM, Carter JR, Peppard PE. Obstructive sleep apnoea during REM sleep and incident non-dipping of nocturnal blood pressure: a longitudinal analysis of the Wisconsin sleep cohort. Thorax. (2015) 70:1062–9. doi: 10.1136/thoraxjnl-2015-207231
50. Mokhlesi B, Finn LA, Hagen EW, Young T, Hla KM, Van Cauter E, et al. Obstructive sleep apnea during REM sleep and hypertension. Results of the Wisconsin sleep cohort. Am J Respir Crit Care Med. (2014) 190:1158–67. doi: 10.1164/rccm.201406-1136OC
51. Appleton SL, Vakulin A, Martin SA, Lang CJ, Wittert GA, Taylor AW, et al. Hypertension is associated with undiagnosed OSA during rapid eye movement sleep. Chest. (2016) 150:495–505. doi: 10.1016/j.chest.2016.03.010
52. Punjabi NM, Bandeen-Roche K, Marx JJ, Neubauer DN, Smith PL, Schwartz AR. The association between daytime sleepiness and sleep-disordered breathing in NREM and REM sleep. Sleep. (2002) 25:307–14.
53. Pamidi S, Knutson KL, Ghods F, Mokhlesi B. Depressive symptoms and obesity as predictors of sleepiness and quality of life in patients with REM-related obstructive sleep apnea: cross-sectional analysis of a large clinical population. Sleep Med. (2011) 12:827–31. doi: 10.1016/j.sleep.2011.08.003
54. Oh SM, Choi SH, Kim HJ, Park KS, Lee YJ. The association between obstructive sleep apnea during REM sleep and autonomic dysfunction as measured by heart rate variability. Sleep Breath. (2019) 23:865–71. doi: 10.1007/s11325-018-01779-y
55. Maquet P, Laureys S, Peigneux P, Fuchs S, Petiau C, Phillips C, et al. Experience-dependent changes in cerebral activation during human REM sleep. Nat Neurosci. (2000) 3:831–6. doi: 10.1038/77744
56. Smith C. Sleep states and memory processes in humans: procedural versus declarative memory systems. Sleep Med Rev. (2001) 5:491–506. doi: 10.1053/smrv.2001.0164
57. Ng DK, Wong JC, Chan CH, Leung LC, Leung SY. Ambulatory blood pressure before and after adenotonsillectomy in children with obstructive sleep apnea. Sleep Med. (2010) 11:721–5. doi: 10.1016/j.sleep.2009.10.007
58. Bixler EO, Vgontzas AN, Lin HM, Liao D, Calhoun S, Fedok F, et al. Blood pressure associated with sleep-disordered breathing in a population sample of children. Hypertension. (2008) 52:841–6. doi: 10.1161/HYPERTENSIONAHA.108.116756
59. Li AM, Au CT, Sung RY, Ho C, Ng PC, Fok TF, et al. Ambulatory blood pressure in children with obstructive sleep apnoea: a community based study. Thorax. (2008) 63:803–9. doi: 10.1136/thx.2007.091132
60. Amin R, Somers VK, McConnell K, Willging P, Myer C, Sherman M, et al. Activity-adjusted 24-hour ambulatory blood pressure and cardiac remodeling in children with sleep disordered breathing. Hypertension. (2008) 51:84–91. doi: 10.1161/HYPERTENSIONAHA.107.099762
61. Kohler M, Stradling JR. CrossTalk proposal: most of the cardiovascular consequences of OSA are due to increased sympathetic activity. J Physiol. (2012) 590:2813–5. doi: 10.1113/jphysiol.2012.229633
Keywords: prevalence, riskfactor, REM sleep disorder, OSA, children
Citation: Chamnanpet S, Tovichien P, Tanphaichitr A and Chotinaiwattarakul W (2022) Prevalence and Risk Factors for Rapid Eye Movement-Related Obstructive Sleep Apnea in Children. Front. Pediatr. 10:869986. doi: 10.3389/fped.2022.869986
Received: 05 February 2022; Accepted: 22 March 2022;
Published: 28 April 2022.
Edited by:
Kostas N. Priftis, National and Kapodistrian University of Athens, GreeceReviewed by:
Angeliki Galani, University General Hospital Attikon, GreeceRefika Ersu, Children’s Hospital of Eastern Ontario (CHEO), Canada
Copyright © 2022 Chamnanpet, Tovichien, Tanphaichitr and Chotinaiwattarakul. This is an open-access article distributed under the terms of the Creative Commons Attribution License (CC BY). The use, distribution or reproduction in other forums is permitted, provided the original author(s) and the copyright owner(s) are credited and that the original publication in this journal is cited, in accordance with accepted academic practice. No use, distribution or reproduction is permitted which does not comply with these terms.
*Correspondence: Prakarn Tovichien, cHJha2Fybi50b3ZAbWFoaWRvbC5lZHU=