- 1Women and Children’s Hospital, School of Medicine, Xiamen University, Xiamen, China
- 2The First Affiliated Hospital of Xiamen University, Xiamen, China
Background: Increasing evidence indicated that ozone (O3) exposure could trigger asthma attacks in children. However, the effect of O3 at low concentrations is uncertain.
Purpose: This study aimed to explore the effects of O3 exposure at low concentrations on asthma attacks in children.
Methods: A total of 3,475 children with asthma attacks from the First Affiliated Hospital of Xiamen University were available for the analyses. Air pollution data and meteorological data in Xiamen during 2016–2019 were also collected. A case-crossover design and conditional logistic regression models were conducted to evaluate the association between asthma attacks and outdoor air pollution with lag structures (from lag 0 to lag 6) in both single and multi-pollutant models. Furthermore, we estimated the influence of various levels of O3 exposure on an asthma attack in three groups categorized by maximum daily 8-h sliding average ozone (O3-8 h) (O3-8 h ≥ 100 μg/m3, O3-8 h: 80–99 μg/m3, O3-8 h < 80 μg/m3).
Results: For both single-pollutant models and multi-pollutant models, when O3-8 h was higher than 80 μg/m3, O3 exposure was increased the risk of acute asthma attacks on each day of lag. The effect of O3 on children with asthma was significant when O3 concentration was higher than 100 μg/m3.
Conclusion: O3 concentration above 80 μg/m3 contributed to an increased risk of asthma attacks in children.
Introduction
Bronchial asthma is a common chronic disease in childhood with shortness of breath, wheezing, and coughing from constriction and mucous-membrane swelling in the bronchi (1). It is estimated that the prevalence of asthma among children aged 0–14 years in China in 2010 was 3.02% (95% confidence interval (CI): 2.97–3.06%) (2). Although most children with asthma can be well-controlled through standardized treatment, many children still need rescue medication or hospitalization for acute asthma attacks. Avoiding the risk factors and predisposing factors is essential for the effective management of asthma. The risk factors for acute asthma attacks are complex, including dust mites, fungi, pollen, and infectious factors. In recent years, air pollutants have gradually attracted attention. Ozone (O3), one of the air pollutants, has been repeatedly reported to be associated with respiratory diseases such as asthma (3) when the ozone concentration is too high.
O3 exists in the stratosphere and troposphere. When in the stratosphere, it prevents ultraviolet rays from harming the human body. However, when in the troposphere, it adversely affects the human respiratory and cardiovascular system when it reaches high concentrations. Ozone is a strong oxidizing gas, easily soluble in water, and the inhaled ozone can penetrate deeply into the lungs (4). Some studies found that ozone might cause airway inflammation, worse lung function, airway hyperresponsiveness, and increased sensitivity to allergens (5–7). According to National Ambient Air Quality Standard for Ozone, the daily maximum 8-h average ozone concentration should be less than 150 μg/m3, and the World Health Organization believes that the daily maximum 8-h average ozone concentration should be less than 100 μg/m3.
Previous studies have suggested that O3 exposure only affect children with asthma (including increasing respiratory symptoms, asthma medication use, and impaired lung function) in the most polluted areas (8). Some recent studies have shown that even if it is lower than the standard set by the World Health Organization, O3 exposure may also cause an acute attack of asthma in children. In a Quebec birth cohort study, the average ozone concentration during the study period was 32.07 ppb (68.76 μg/m3). This study found that ozone exposure was associated with the risk of asthma, with an odds ratio (OR) value of 1.11 (95% CI: 1.10, 1.12) (9). Short-term increases in low levels (<70 ppb) of ozone are associated with decreased lung function (10). Children with asthma who use maintenance medications are particularly susceptible to the effects of ozone below the US Environmental Protection Agency (EPA) standard (11).
Xiamen is a city on the southeast coast of China. During 2016–2019, the average concentration of maximum daily 8-h sliding average ozone (O3-8 h) was 81.21 μg/m3, the proportion of ozone concentration above ozone level 2 standard (160 μg/m3)was 0.62%. In the present study, the effects of increasing ozone levels at low concentrations on acute asthma attacks were investigated.
Methods
Study Participants
There were 29 065 cases with the diagnosis code J45 in the emergency department of the First Affiliated Hospital of Xiamen University from 1 January 2016 to 31 December 2019. According to the “Guidelines for the Diagnosis and Treatment of Children’s Bronchial Asthma (2016 Edition),” a total of 3,959 cases were identified as acute asthma attacks. After excluding 221 (5.58%) cases with fever, 15 (0.38%) with acute asthma attacks caused by self-discontinuation within 14 days, 7 cases with allergen exposure, and 2 cases (0.05%) outside of the region, a total of 3,714 cases (93.81%) of acute asthma attacks were eligible for the analyses.
Medical Data
Data were obtained from the Electronic Medical Record for asthma (code J45, International Classification of Diseases, Tenth Revision—ICD-10) among children aged 0-14 years in the First Affiliated Hospital of Xiamen University from 1 January 2016 to 31 December 2019. Combined with the main complaint, current medical history, and time of visit, the date of the first acute onset of asthma in the child was determined, and the recurrent cases were counted as one. The data of non-local residents and cases with fever and a clear diagnosis of asthmatic bronchitis were excluded.
Air Pollution
Ambient monitoring data, including O3-8 h concentrations and 24-h average concentrations of carbon monoxide (CO), nitrogen dioxide (NO2), sulfur dioxide (SO2), and particulate matter (PM10 and PM2.5), were measured at four monitoring stations in Xiamen metropolitan from 1 January 2016 to 31 December 2019. The 8-h average concentration refers to the average concentrations of continuous 8 h, and the daily maximum 8-h average concentration of zone refers to the maximum value of the continuous 8-h average. For example, N1 = average {c1, c2, c3, c8}, N2 = average {c2, c2, c3, c9}, N3 = average {c3, c2, c3, c10}, and so on, N17 = average {c17, c2, c3, c24}. The daily maximum 8-h average concentration = max {N1, N2, N3, N17}. The pollutants concentration was measured by the same method at each monitoring station. O3, NO2, and SO2 concentrations were measured by using an open-path deferential optical absorption spectroscopy (DOAS) instrument.
Meteorological Data
Meteorological data, including daily average temperature (in degrees centigrade), relative humidity (as a percentage), wind velocity (in meters per second), and rainfall (in millimeter) were collected from the Xiamen Meteorological Bureau.
Statistical Methods
In this study, the case-crossover method was used to explore the relationship between ozone and asthma exacerbations. Case-crossover design has been widely used in environmental epidemiology to study the impact of short-term environmental exposure on the risk of individuals with rapid onset events. Since each case served as their control and matches all time-varying, unmeasured or unmeasured thematic characteristics, a case-crossover design eliminates time-constant and slowly varying confounders (such as gender, race, age, genetics, personal lifestyle, or behavior). This study was a retrospective analysis and the approaches was “symmetric two-way.” The date of the asthma attack was the case date, and two control dates were the date 1 week before the case date and the other after, avoiding the day of the week effect.
Considering the lag effect of the concentrations of each pollutant, lag0 to lag6 was selected. To control the potential confounding effect of weather, we set the daily relative humidity, average temperature, average wind speed, and rainfall in the same period lag 0–6 days as covariates. Considering the possible interaction between ozone and other pollutants, we also discussed the relationship between acute asthma attacks and other gaseous pollutants (NO2, CO, and SO2) and particulate pollutants (PM10 and PM2.5).
The analysis strategy of each part of the correlation model is as follows:
(1) Single-pollutant model: After adjusting for the meteorological factors, single-pollutant models of O3 from lag 0 to lag 6 were established.
(2) Multi-pollutant model: After controlling for confounding factors, a multi-pollutant model of O3 + NO2 + CO + SO2 + PM2.5 + PM10 was established and the OR with a 95% CI was calculated.
(3) Ozone concentration grouping model: lag structures was categorized into three groups according to the O3-8 h concentrations (O3-8 h ≥ 100 μg/m3, O3-8 h: 80–99 μg/m3, O3-8 h < 80 μg/m3). After adjusting for the lagging meteorological factors, the single-pollutant and multi-pollutant model were established and the effects of pollutants on acute asthma attacks were calculated.
Conditional logistic regression models were used to identify associations between air pollutants and asthma attacks in children, and all results were expressed as OR and 95% CI. All statistical analyses were performed in the R (version 3.6.0).
Results
Characteristics of Acute Asthma Attacks and Participants
The characteristics of acute asthma attacks are presented in Table 1. Male children accounted for 69.15% of cases, and cases younger than 6 years old accounted for 60.07%. The diagnosis included eight types of J45. Among them, a total of 18 children were hospitalized, and the rest were treated in outpatient clinics and followed up. There were no deaths.
Among the 3,714 cases, there were 3,475 patients, including 2,383 males (68.58%) and 1,092 females (31.42%). Children younger than 6 years old accounted for 61.41%. There were 779 (22.42%) children with a history of eczema, 360 (10.36%) children with rhinitis. There were 784 (22.56%) patients with rhinitis in the family, and 110 (3.17%) patients with a history of asthma, as shown in Table 2.
Characteristics of Air Pollutant Concentrations and Meteorological Variables
As shown in Table 3, the average concentration of O3-8 h in Xiamen during 2016–2019 was 81.21 μg/m3, the proportion of ozone concentration above ozone second-level standard (160 μg/m3) was 0.62% and the number of days exceeding ozone first-level standard (100 μg/m3) was 361 days (24.7%). The average concentrations of NO2, PM2.5, and PM10, were 29.02, 12.057, and 44.85 μg/m3, respectively, and the proportion of days exceeding the first-level standard was 0.34, 7.94, and 33.26%, respectively. The daily average concentrations of CO and SO2 did not exceed the first-level standard.
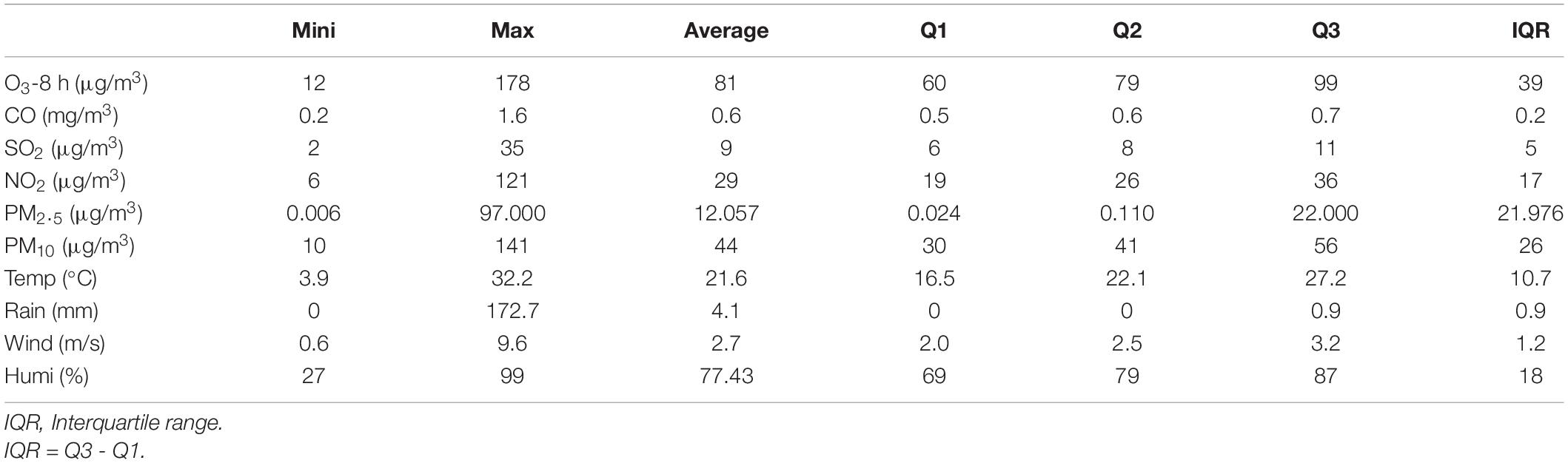
Table 3. Characteristics of daily air pollutants and meteorological variables in Xiamen from 2016 to 2019.
Correlation Analysis of Air Pollutant Concentrations and Meteorological Variables
As shown in Table 4, there was a positive correlation between ozone and SO2, PM2.5, PM10, air temperature, and wind speed (P < 0.05), and the correlation coefficients were 0.125, 0.418, 0.418, 0.124, and 0.118, respectively. There was a negative correlation between relative humidity, rainfall, and ozone (P < 0.05).
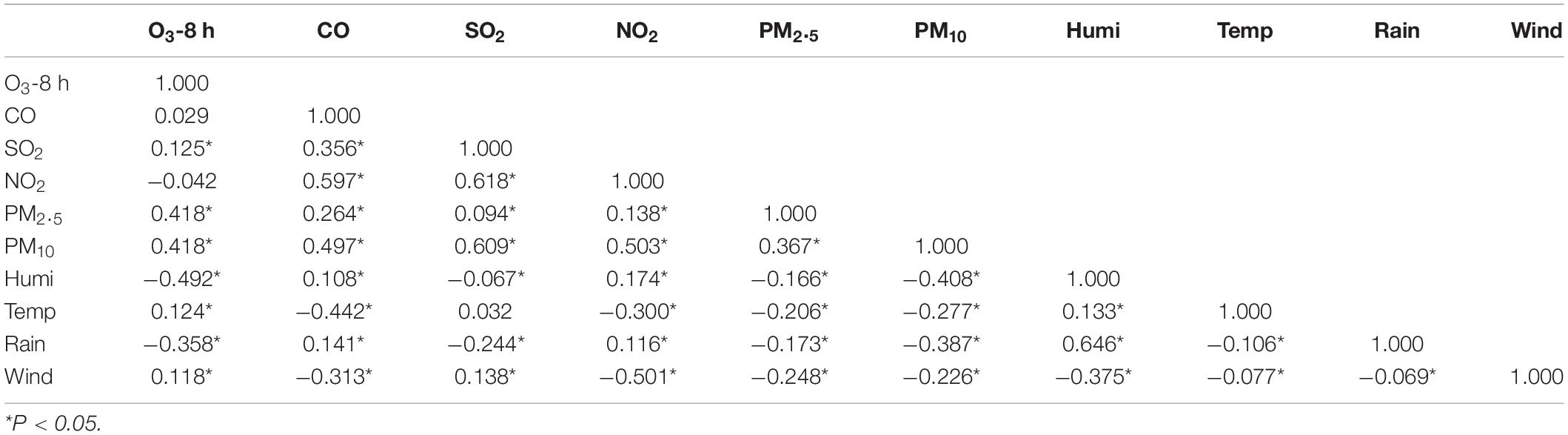
Table 4. Correlation analysis between air pollutants and meteorological factors in Xiamen from 2016 to 2019.
Single Pollutant Model
After controlling the confounding factors of daily relative humidity, average temperature, average wind speed, and rainfall, the single pollutant models were established, as shown in Figure 1.
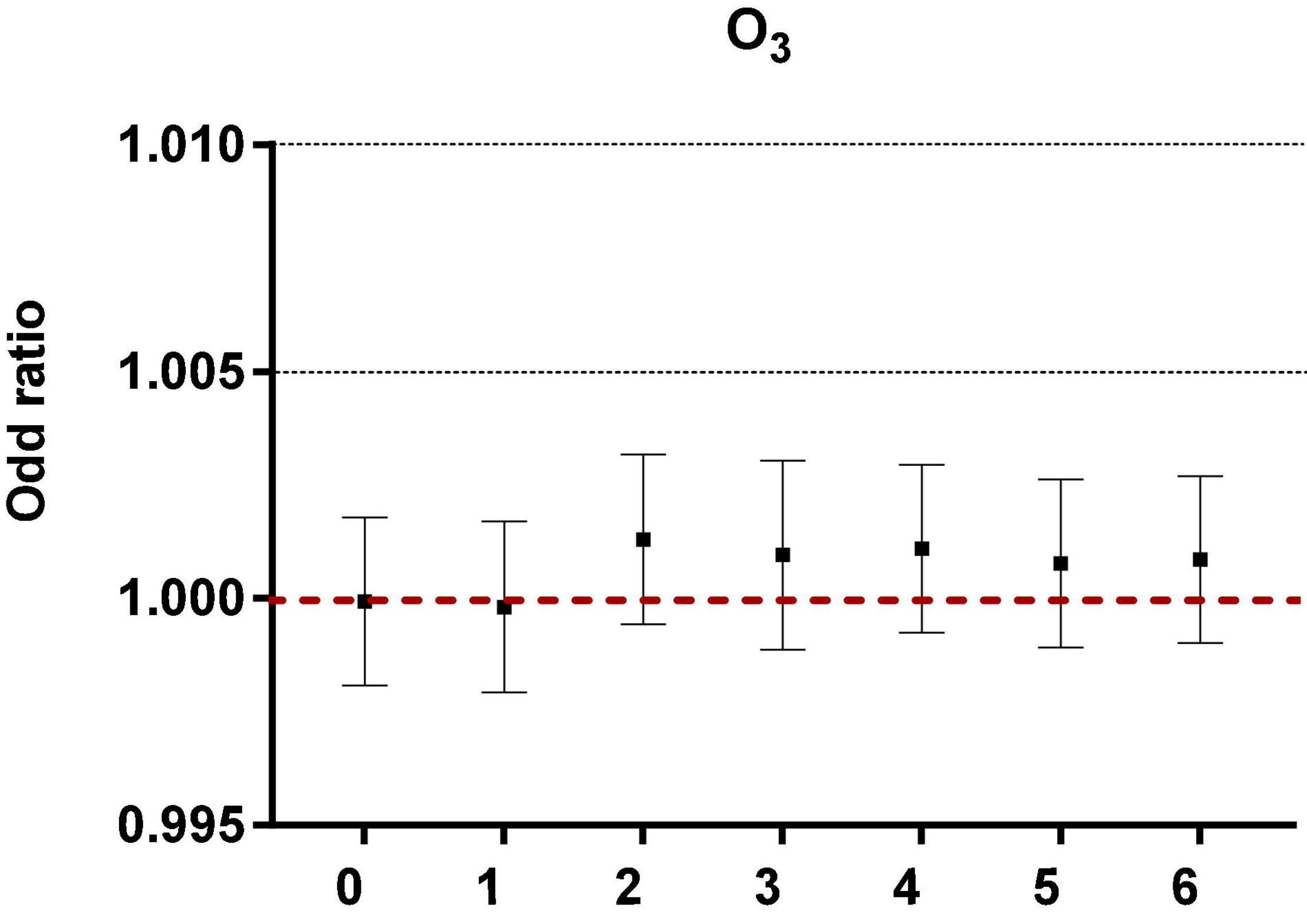
Figure 1. The correlation of ozone in different lag periods to acute asthma attacks in the single pollutant model.
Multi-Pollutant Model
Under the adjustment of meteorological factors and weekly effects, a multi-pollutant model was constructed to evaluate the effects of pollutants on acute asthma attacks, as shown in Figure 2.
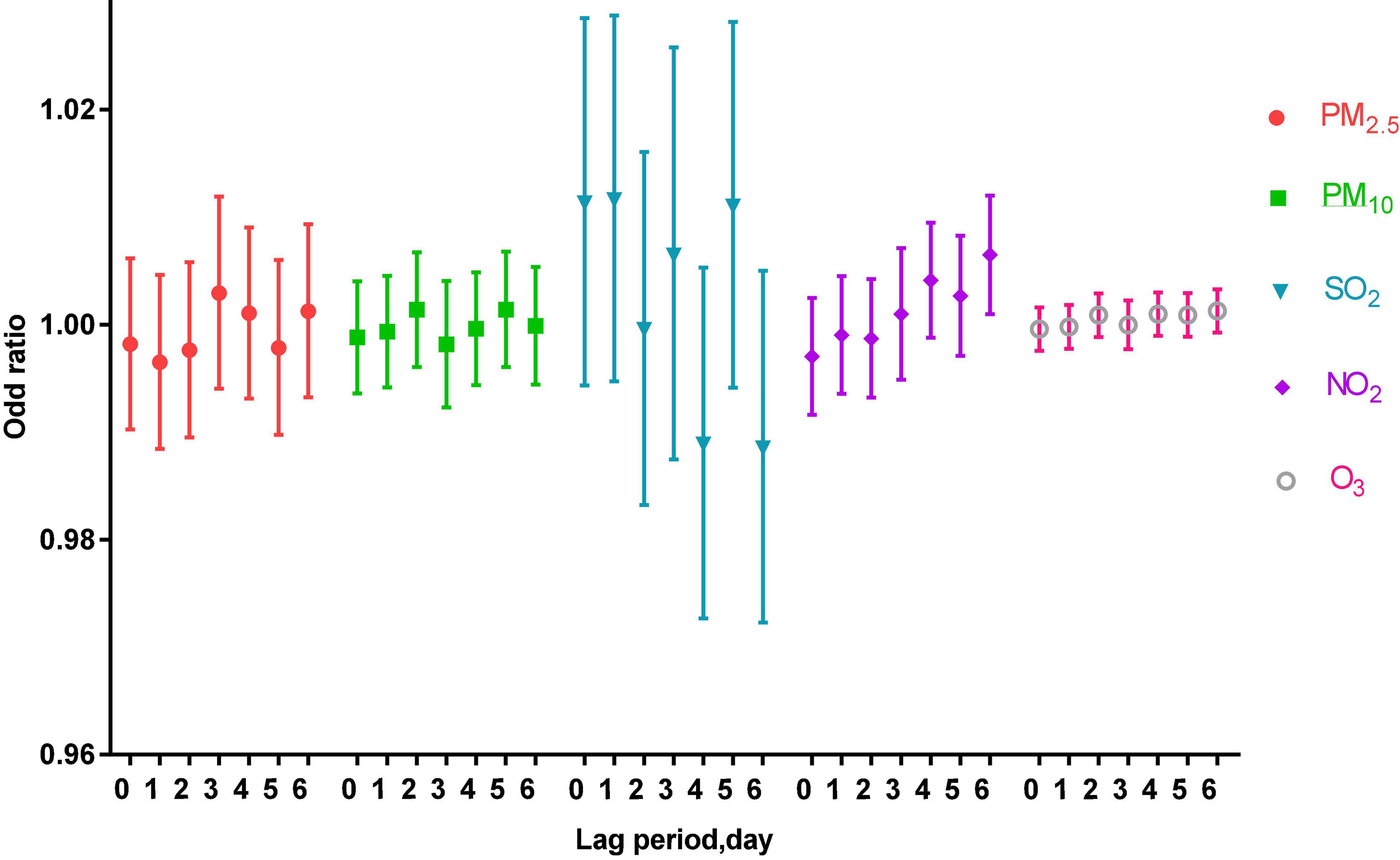
Figure 2. The correlation of air pollutants in different lag periods to acute asthma attacks in the multi-pollutant model.
Analysis of Ozone Concentration in Different Lag Periods
Single Pollutant Model
Figure 3 shows that in the single-pollutant model that adjusts for the lagging meteorological factors, acute asthma attacks were associated with different lag periods and different levels of ozone exposure (P < 0.001). When O3-8 h is greater than or equal to 100 μg/m3, every 10 μg/m3 increase of O3-8 h increased 6.33, 7.08, 6.89, 7.11, 7.06, 6.14, and 6.28% of acute asthma attacks from 0 to 6 days lag.
When O3-8 h was between 80 and 99 μg/m3, every 10 μg/m3 increase of O3-8 h increased 2.36, 3.08, 2.46, 2.52, 2.15, 2.25, and 2.13% of acute asthma attacks from 0 to 6 days lag.
From lag 0 to lag 6, when O3-8 h is less than 80 μg/m3, every 10 μg/m3 increase in O3-8 h, the odds ratios is 0.9700, 0.9714, 0.9717, 0.9741, 0.9719, 0.9734, and 0.9743.
Multi-Pollutant Model
As shown in Figures 4–6, the multi-pollutant model with different ozone levels in different lag periods showed that the health effects of ozone on asthma attacks in children was similar to that in the single-pollutant model. Ozone concentration above 80 μg/m3 contributed to an increased risk of asthma attacks in children. The effect of ozone on children with asthma was significant when ozone concentration was higher than 100 μg/m3. When ozone concentration was less than 80 μg/m3, the ozone concentration was negatively correlated with asthma attacks in children.
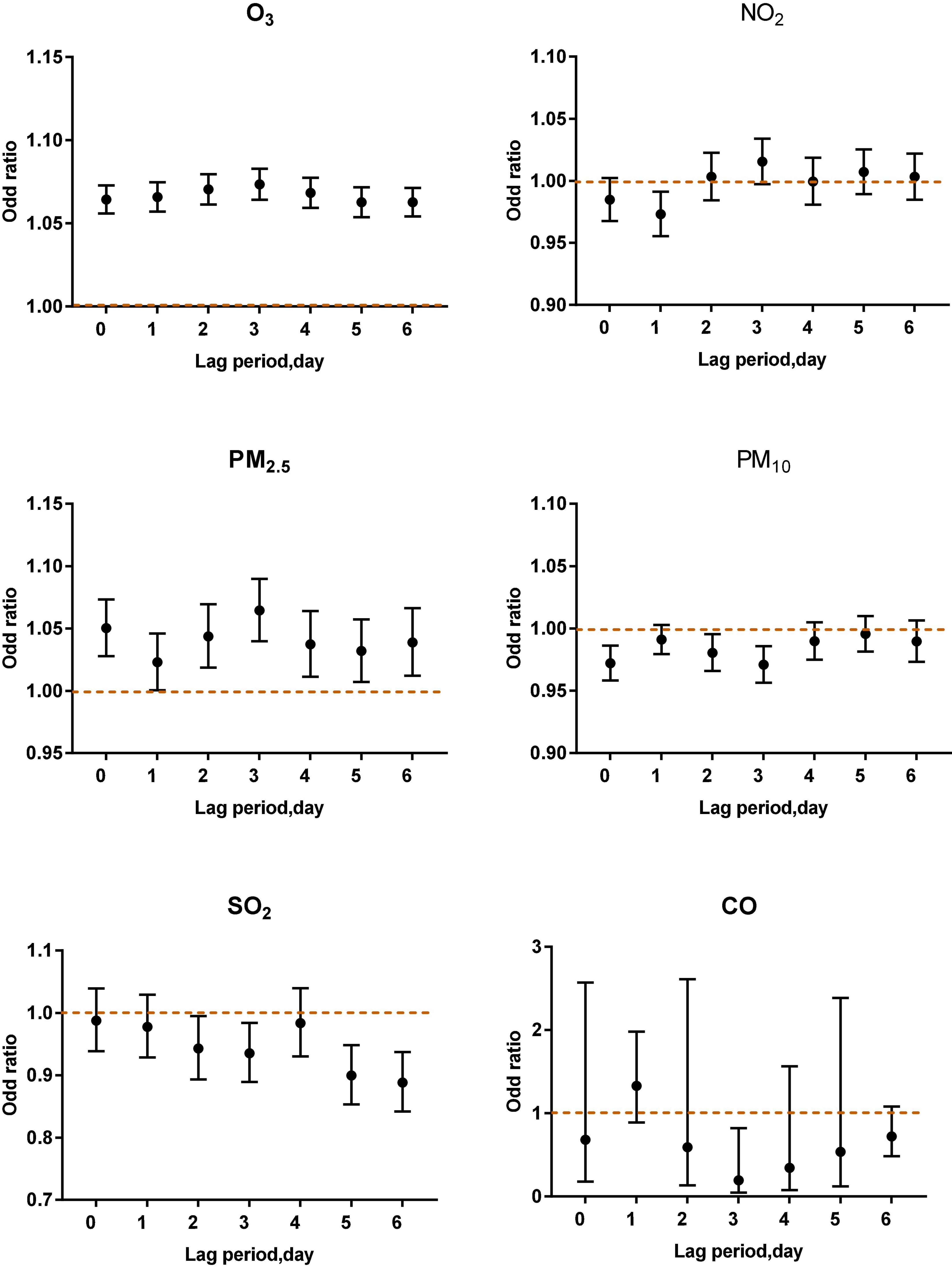
Figure 4. Air pollutants in different lag periods in the multi-pollutant model when O3-8 h was higher than 100 μg/m3.
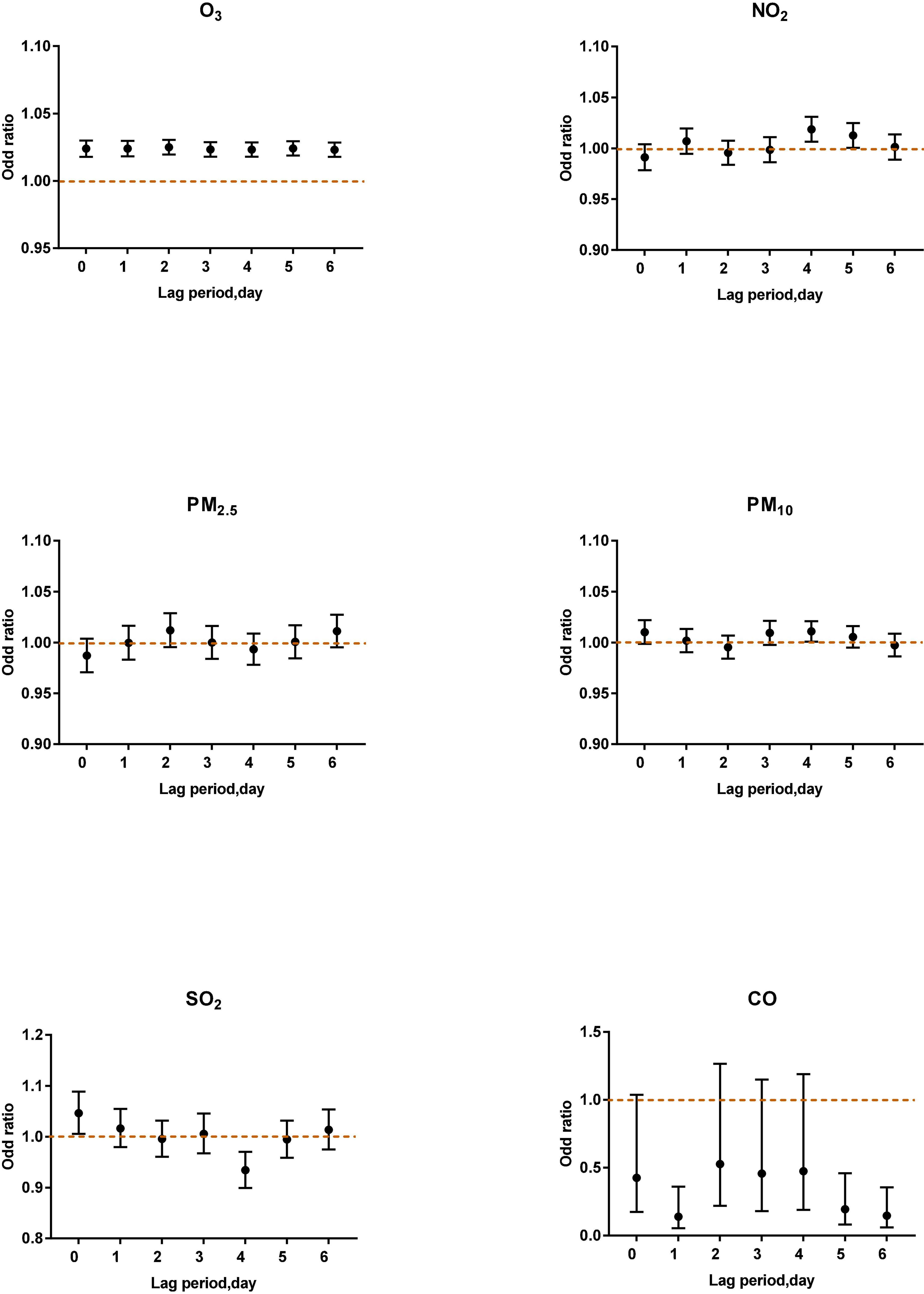
Figure 5. Air pollutants in different lag periods in the multi-pollutant model when O3-8 h was between 80 and 99 μg/m3.
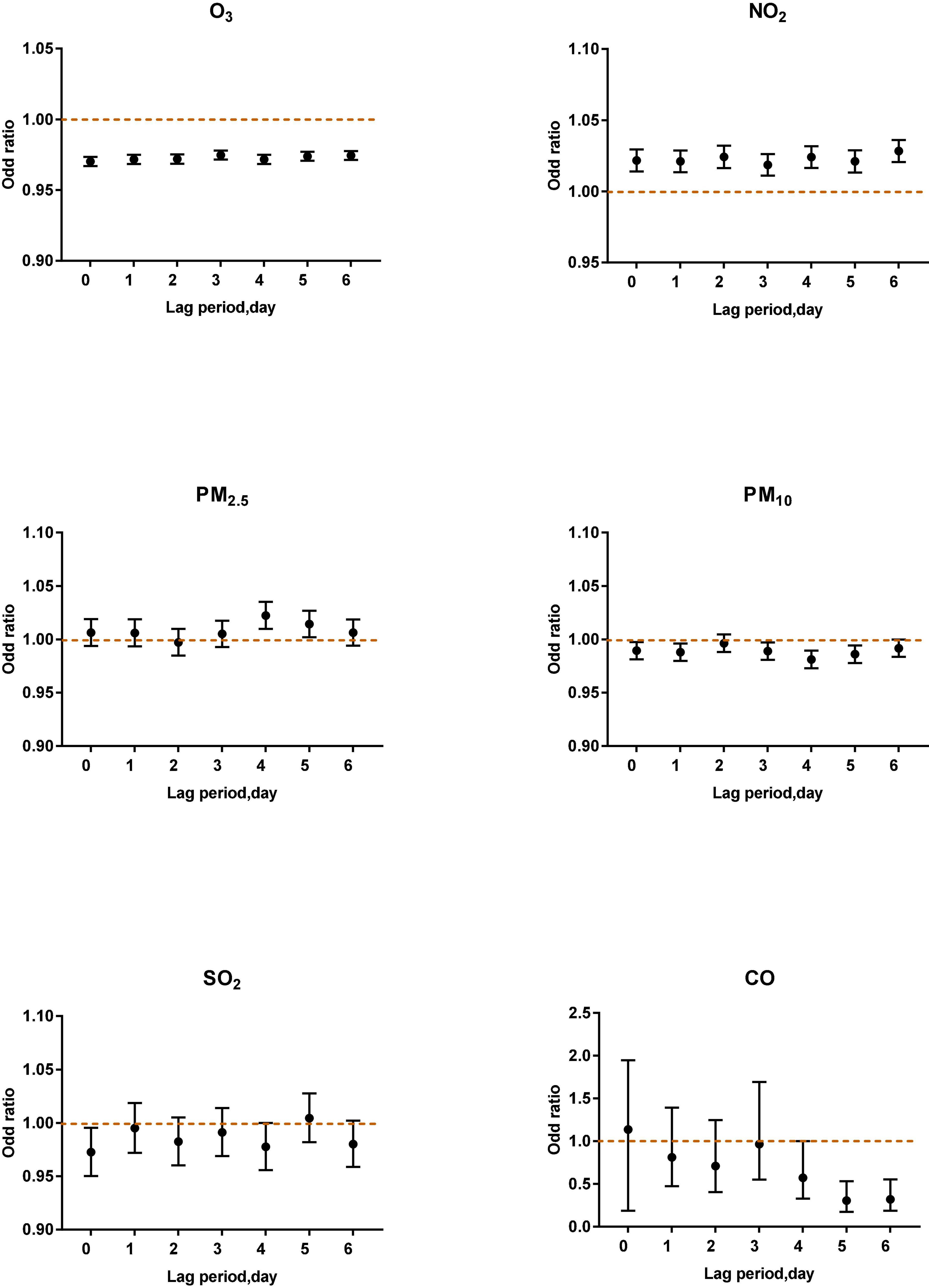
Figure 6. Air pollutants in different lag periods in the multi-pollutant model when O3-8 h was less than 80 μg/m3.
When O3-8 h was higher than 100 μg/m3, PM2.5 (lag0, OR: 1.0503, 95% CI: 1.0277–1.0733; lag2, OR: 1.0437, 95% CI:1.0186–1.0694; lag3 OR: 1.0644, 95% CI: 1.0398–1.0897; lag4, OR: 1.0372, 95% CI: 1.0112–1.0639; lag5, OR: 1.0319, 95% CI: 1.0071–1.0573; lag6, OR: 1.0388, 95% CI: 1.0121–1.0663) was positively correlated with asthma attacks. PM10 (lag0, lag2, lag3), SO2 (lag3, lag5, lag6), NO2 (lag1) were negatively associated with acute asthma attacks (Figure 4).
When O3-8 h was between 80 and 99 μg/m3, NO2 (lag4, OR: 1.0186, 95% CI: 1.0065–1.0309) was positively correlated with asthma attacks. SO2 (lag4, OR: 0.9341, 95% CI: 0.8993–0.9703) was negatively associated with acute asthma attacks (Figure 5).
When O3–8 h was less than 80 μg/m3, PM2.5 (lag4, OR: 1.0223, 95% CI: 1.0097–1.0351) and NO2 (lag0, OR: 1.0216, 95% CI: 1.0140–1.0294; lag1, OR: 1.0210, 95% CI: 1.0134–1.0287; lag2, OR: 1.0242, 95% CI: 1.0164–1.0321; lag3 OR: 1.0186, 95% CI: 1.0111–1.0261; lag4, OR: 1.0241, 95% CI: 1.0165–1.0317; lag5, OR: 1.0210, 95% CI: 1.0132–1.0289; lag6, OR: 1.0283, 95% CI: 1.0206–1.0361) were positively correlated with asthma attacks. PM10 (lag0, lag1, lag2, lag3), SO2 (lag0) were negatively associated with acute asthma attacks (Figure 6).
The above correlations were all statistically significant.
Discussion
Ozone and Asthma
High levels of ozone are a risk factor for childhood asthma attacks. Pulmonary function tests were used to evaluate the prevalence of asthma in children in areas with high and low ozone concentrations, and it was found that ozone pollution would increase the prevalence of asthma (12). Moreover, high levels of ozone may increase the number of hospitalizations in children with asthma (13). A study from South Texas that included 902 children who were hospitalized for asthma at least twice found that a higher level of ozone was significantly associated with an increased number of children hospitalized for asthma. A foreign birth cohort study found that long-term exposure to high levels of ozone (>70 ppb) was also associated with hospitalization in children with asthma (OR 1.16–1.68) (14). In addition, previous epidemiological studies have also reported that children with asthma who take maintenance drugs are particularly susceptible to high concentrations of ozone. The background levels of ozone in this study are O3-1 h average 59 ppb (SD: 19 ppb) and O3-8 h average 51 ppb (SD: 19 ppb). The study found that in the model of PM2.5 + O3, every 50 ppb increase in O3-1 h might increase the risk of wheezing and chest tightness by 35 and 47%, respectively (11).
Exposure to the high levels of ozone might lead to innate lymphoid cells (ILC2)-mediated type 2 immunity in children with asthma (15). T and B cells play a role in the adaptive immune. However, an animal study found that the upper respiratory tract changes induced by repeated exposure to ozone were mediated by the second group of ILC2, not T cells and B cells. In addition, surfactant protein D (SP-D) is a product of airway epithelial cells and plays an important role in immune defense mechanisms. SP-D can resist inflammatory changes and has a multimeric structure that is easily oxidized. Inhaled corticosteroids, which could induce the synthesis of SP-D have anti-inflammatory effects on the lungs and can effectively treat asthma (16). However, an animal study showed that ozone exposure weakened the anti-inflammatory effect of budesonide. Ozone exposure inhibited the release of SP-D in the airway.
As shown in Figures 3, 5, this study found that relatively low-level ozone (O3-8 h: 80–99 μg/m3) was harmful to acute asthma attacks. Studies have explored the effects of ozone exposure close to or lower than the US EPA standard on infant respiratory system. Previously study found that ozone exposure increased the risk of wheezing and dyspnea in infants whose mothers were diagnosed with asthma. The risk of wheezing and dyspnea increased by 59% (95% CI, 1–154%) and 83% (95% CI, 42–136%) for each additional Inter-quartile range (IQR) respectively (17). In recent years, some studies have also found the effects of a relatively low ozone environment on children with asthma. A case-crossover study collected the number of emergency department visits (n = 91 386) for asthma or wheezing in 41 hospitals for children aged 5–17 years. The Poisson generalized linear model found that even at relatively low ozone concentrations (47.3 ppb), ozone was still associated with childhood asthma or wheezing (18). In a cohort study focused on asthma drug treatment, an electronic drug monitor was installed on a short-acting Beta2 agonist (SABA) metered-dose inhaler to monitor the use of SABA. This study showed that ozone exposure below the US EPA standard was positively correlated with SABA use in both children and adults. Each interquartile range increase in ozone (16.8 ppb) increased the use of SABA in children (11.3%; 95% CI: 7.0–18.2%) than that in adults (8.4%; 95% CI: 6.4–11.0%) (19). Besides, the low ozone concentrations in the urban area might increase the responsiveness to allergens in patients with atopic asthma (20).
For both the single-pollutant model and multi-pollutant model (Figures 3, 6), when ozone concentration was less than 80 μg/m3, the ozone concentration was negatively correlated with asthma attacks in children. The potential mechanism of the protective effect of ozone at lower concentrations is still unclear. It might be related to the reactive oxygen species (ROS), produced by ozone, which has a dual influence on cell integrity. ROS are beneficial to cells at low concentrations (21). After ozone exposure, damage to the bronchiolar cell and airway inflammatory reaction is reversible. The study explored the influence of the interaction between genes and environmental factors on children with asthma observed that children with asthma who carry the TNF-308 GG gene have a significantly lower risk of bronchial symptoms under low ozone exposure (OR: 0.53, 95% CI: 0.31–0.91) (22). In addition, studies have found that oxidant genes have a protective effect on children living in low-ozone communities. A cohort study found that the effect of HMOX-1 gene variants on the risk of new-onset asthma varies with environmental ozone levels. The shorter HMOX-1 alleles (less than 23 repetitions) have the protective effect (HR, 0.44; 95% CI, 0.23–0.83) in children living in a low-level ozone environment (23).
In addition, indoor pollution has gradually entered the field of vision, and no association has been found between asthma and indoor ozone. A recent study from Beijing, China evaluated children’s cardiopulmonary response to indoor ozone exposure. During the study period, the average indoor ozone concentration (SD) was 8.7 (6.6) ppb, which was lower than the current guidelines and standards. The results showed low levels of indoor ozone were associated with decreased cardiac autonomic nerve function and increased heart rate in children. For every increase in IQR, cardiac autonomic nerve function decreases by −7.8% (95% CI: −9.9%, −5.6%), and heart rate increases by 2.6% (95% CI: 1.6%, 3.6%), but no significant correlation was found between the effects of airway inflammation and lung function (24).
Other Pollutants and Asthma
Reports show that air pollution is a risk factor leading to global morbidity and mortality. Pollutants can induce a variety of respiratory symptoms and are closely related to respiratory diseases. Many studies have shown that it was significantly associated with asthma outcomes, such as morbidity, prevalence, hospitalization rate, emergency room visits, mortality, and asthma attacks (25). This study explores the effects of both gaseous pollutants and particulate pollutants on acute asthma attacks. The results showed the association between acute asthma attacks and PM2.5, PM10, NO2, and SO2 in the single-pollutant and multi-pollutant models. The results of a meta-analysis which included 22 case-crossover studies showed that all pollutants except SO2 and PM10 were significantly related to asthma attacks. The OR of ozone was 1.032 (95% CI: 1.005, 1.060) (26). A domestic study investigated 4,454 deaths from asthma in China from 2013 to 2018 by using case cross-analysis and conditional logistic regression model. The results showed that PM2.5 (lag 3; IQR, 47.1 μg/m3), NO2 (lag 3; IQR, 26.3 μg/m3) and O3 (lag 3; IQR, 52.9 μg/m3) were positively correlated with asthma mortality, with OR of 1.07 (95% CI): 1.01–1.22), 1.11 (95% CI: 1.01–1.22), and 1.09 (95% CI: 1.01–1.18), indicating that short-term exposure to pollutants may increase asthma mortality (27). Even exposure early in life may be related to the development of childhood asthma. A foreign prospective birth cohort study, which included 14 126 participants and followed up for 14–16 years found that the increase in PM2.5 and NO2 was associated with an increase in the risk of asthma. For every 10 μg/m3 increase, the OR values were 1.13 (95% CI 1.02–1.25) and 1.29 (1.00–1.66), respectively (28).
The effects of particulate pollutants on acute asthma attacks are consistent with findings from many previous studies (29, 30). We are facing the challenge of the combined effects of the environment and health. In this study, the daily average concentration of PM2.5 between 2016 and 2019 was 12.057 μg/m3, of which 116 days exceeded the first-level standard 35 μg/m3 (accounting for 7.94%). The average PM10 was 44.85 μg/m3, and the number of days exceeding the standard accounted for 33.26%. The average value of O3-8 h was 81.21 μg/m3, and the number of days exceeding the standard accounted for 24.7%. Controlling the balance between PM2.5 and ozone, and reducing the impact of particulate pollutants and ozone on health was still needed to be illustrated in the future. Merely reducing PM will also increase ozone, especially in winter. Merely reducing PM will also increase ozone, especially in winter. By reducing particulate pollutants, reducing the aerosol optical depth by 50% can increase ozone by 25% and also enhance the effect of volatile organic compounds (VOC) (31).
A study in North China using the Goddard Earth Observation System chemical transport model showed that PM2.5 might stimulate the production of ozone by slowing down the aerosol deposition of hydroperoxide radicals (8). The impact of short-term exposure to particulate pollutants and ozone on acute asthma attacks might vary depending on the asthma phenotype, such as whether it was associated with allergic diseases. A time-stratified case-crossover study found that in the two pollutant models with and without allergic diseases, when the 3-day moving average of ozone (lag 0–2) increased by 20 ppb, the OR was 1.08 (95%CI: 1.02, 1.14) and 1.00 (95% CI: 0.95, 1.05), for every 10 μg/m3 increase in PM2.5 3-day moving average (lag 0–2), the odds ratio was 1.10 (95% CI: 1.07, 1.13) and 1.05 (95% CI: 1.02, 1.09), suggesting that asthma patients with allergic diseases were more susceptible to PM2.5 and ozone (32). In addition, exposure to particulate pollutants may be associated with inflammation of the lower respiratory tract. An animal experimental study found that PM2.5 (lag by 2 and 3 weeks) per IQR increased the risk of granulocytosis in bronchoalveolar lavage fluid by 11% (p = 0.04, 95% CI = 1.01–1.22) (33).
NO2 also affects children with asthma, such as asthma symptoms, decreased response to bronchodilators, and lung function damage. Studies have shown that asthma has the strongest association with O3 and NO2 (34). A systematic review of short-term exposure to O3 and NO2 found that daily short-term exposure to O3 and NO2 increased the risk of asthma exacerbation (35). We found that when O3-8 h was between 80 and 99 μg/m3, NO2 (lag 4 days) was positively correlated with the risk of an acute asthma attack. When O3-8 h was less than 80 μg/m3, NO2 had a significant positive correlation with the risk of an acute asthma attack after 0–6 days of lag, while ozone shows a negative correlation. Environmental ozone is mainly formed through complex photochemical reactions, and its precursors include nitrogen oxides (NOX: NO and NO2) and volatile organic compounds. The ozone level has a complex non-linear relationship with the concentration of NO2, and NO2 will weaken the photochemical reaction of O3 to a certain extent. When the NOX concentration is low, the ozone concentration increases with the increase in the NOX concentration and has nothing to do with the VOC concentration. When the NOX concentration is high, reducing NOX will increase ozone (31, 36). A study has shown that NO2 and O3 are closely related, and there is a long-term time sequence in some periods (37).
Sulfur dioxide can cause bronchoconstriction (5). A study in China pointed out that sulfur dioxide is related to the prevalence and symptoms of asthma in children (especially children with atopic allergies) (38) when the concentration of sulfur dioxide exceeded the World Health Organization’s Clean Air Guidelines. The results of this study showed thatSO2 (lag 0) was positively correlated with an acute asthma attack (OR: 1.0461, 95% CI: 1.0055–1.0884) when O3-8 h was between 80 and 99 μg/m3. Ozone is an effective oxidant, while sulfur dioxide is a reducing agent. They may cause asthma symptoms through different mechanisms.
Advantages and Limitations
There are some advantages to this study. First of all, this study analyzed the influence of increasing ozone levels at low concentrations on acute asthma attacks. Secondly, the case-crossover study design is suitable for assessing the transient effects on the risk of the onset of acute events. Moreover, this design allows each patient to serve as his control. Patient-level confounding factors and time-varying variables are readily controlled. Finally, this study used single and multiple pollutant models to examine the relationship between ozone and asthma exacerbations.
The current research has some potential limitations. First, ambient concentration data were from only four monitoring stations of Xiamen. Ambient air monitoring equipment measures air quality at fixed outdoor locations, while asthma children breathe air in several indoor and outdoor environs throughout the day—ultimately do not fully represent children’s exposures. Second, this study did not include pollen exposure in the study. Pollen exposure in the daily environment may also affect lung function. Finally, this study is a single-center study. A multi-center study is needed to verify the reliability of the results.
Conclusion
Our data provided a unique opportunity to examine the influence of ambient ozone on asthma attacks in children. The data indicate that short-term exposure to O3, PM2.5, PM10, NO2, and SO2 may be associated with acute asthma exacerbations. When ozone concentrations are higher than 80 μg/m3, children are at significantly increased risk of asthma attacks.
Data Availability Statement
The original contributions presented in the study are included in the article/supplementary material, further inquiries can be directed to the corresponding author.
Ethics Statement
The studies involving human participants were reviewed and approved by the Ethical Review Board of The First Affiliated Hospital of Xiamen University. Written informed consent from the participants’ legal guardian/next of kin was not required to participate in this study in accordance with the national legislation and the institutional requirements.
Author Contributions
WH and JW coordinated and supervised the data. WH wrote the manuscript. JW and XL revised the manuscript. All authors contributed to the conception, interpretation of the results, and approved the final manuscript.
Conflict of Interest
The authors declare that the research was conducted in the absence of any commercial or financial relationships that could be construed as a potential conflict of interest.
Publisher’s Note
All claims expressed in this article are solely those of the authors and do not necessarily represent those of their affiliated organizations, or those of the publisher, the editors and the reviewers. Any product that may be evaluated in this article, or claim that may be made by its manufacturer, is not guaranteed or endorsed by the publisher.
Acknowledgments
We thank the Xiamen Meteorological Bureau for providing meteorological data, including ozone. We also thank the Xiamen Department of Environmental Protection for providing air pollutant data. We also thank Yu Zhu, Zhifan Hu, and Xiaoshan Dai, for their contributions to data collection.
References
1. Bao Y, Chen A, Fu Z, Li C, Liu C, Xiang L, et al. Chinese medical association guidelines for the diagnosis and prevention of asthma in children. Chin J Pediatr. (2016) 54:167–81.
2. Liu C, Hong J, Shang Y, Sun J, Liu L, Duolikun M, et al. The third nationwide survy of childhood asthma in urban areas of China. Chin J Pediatr. (2013) 51:729–35.
3. Zhang JJ, Wei Y, Fang Z. Ozone pollution: a major health hazard worldwide. Front Immunol. (2019) 10:2518. doi: 10.3389/fimmu.2019.02518
4. Manisalidis I, Stavropoulou E, Stavropoulos A, Bezirtzoglou E. Environmental and health impacts of air pollution: a review. Front Public Health. (2020) 8:14. doi: 10.3389/fpubh.2020.00014
5. Guarnieri M, Balmes JR. Outdoor air pollution and asthma. Lancet. (2014) 383:1581–92. doi: 10.1016/s0140-6736(14)60617-6
6. D’Amato G, Cecchi L, D’Amato M, Liccardi G. Urban air pollution and climate change as environmental risk factors of respiratory allergy: an update. J Investig Allergol Clin Immunol. (2010) 20:95–102; quiz following 102.
7. Ierodiakonou D, Zanobetti A, Coull BA, Melly S, Postma DS, Boezen HM, et al. Ambient air pollution, lung function, and airway responsiveness in asthmatic children. J Allergy Clin Immunol. (2016) 137:390–9. doi: 10.1016/j.jaci.2015.05.028
8. Gold DR, Damokosh AI, Pope CA, Dockery DW, McDonnell WF, Serrano P, et al. Particulate and ozone pollutant effects on the respiratory function of children in southwest Mexico city. Epidemiology. (1999) 10:8–16. doi: 10.1097/00001648-199901000-00004
9. Tétreault L-F, Doucet M, Gamache P, Fournier M, Brand A, Kosatsky T, et al. Childhood exposure to ambient air pollutants and the onset of asthma: an administrative cohort study in Québec. Environ Health Perspect. (2016) 124:1276–82. doi: 10.1289/ehp.1509838
10. Hernandez ML, Dhingra R, Burbank AJ, Todorich K, Loughlin CE, Frye M, et al. Low-level ozone has both respiratory and systemic effects in African American adolescents with asthma despite asthma controller therapy. J Allergy Clin Immunol. (2018) 142:1974–7.e3. doi: 10.1016/j.jaci.2018.08.003
11. Gent JF, Triche EW, Holford TR, Belanger K, Bracken MB, Beckett WS, et al. Association of low-level ozone and fine particles with respiratory symptoms in children with asthma. JAMA. (2003) 290:1859–67. doi: 10.1001/jama.290.14.1859
12. Sousa SIV, Ferraz C, Alvim-Ferraz MCM, Martins FG, Vaz LG, Pereira MC. Spirometric tests to assess the prevalence of childhood asthma at Portuguese rural areas: influence of exposure to high ozone levels. Environ Int. (2011) 37:474–8. doi: 10.1016/j.envint.2010.11.014
13. Baek J, Kash BA, Xu X, Benden M, Roberts J, Carrillo G. Pediatric asthma hospitalization: individual and environmental characteristics of high utilizers in South Texas. J Asthma. (2020). 59:11. doi: 10.1080/02770903.2020.1827424
14. Lin S, Liu X, Le LH, Hwang SA. Chronic exposure to ambient ozone and asthma hospital admissions among children. Environ Health Perspect. (2008) 116:1725–30. doi: 10.1289/ehp.11184
15. Harkema JR, Wagner JG. Innate lymphoid cell-dependent airway epithelial and inflammatory responses to inhaled ozone: a new paradigm in pathogenesis. Toxicol Pathol. (2019) 47:993–1003. doi: 10.1177/0192623319873872
16. Flayer CH, Ge MQ, Hwang JW, Kokalari B, Redai IG, Jiang Z, et al. Ozone inhalation attenuated the effects of budesonide on –induced airway inflammation and hyperreactivity in mice. Front Immunol. (2019) 10:2173. doi: 10.3389/fimmu.2019.02173
17. Triche EW, Gent JF, Holford TR, Belanger K, Bracken MB, Beckett WS, et al. Low-level ozone exposure and respiratory symptoms in infants. Environ Health Perspect. (2006) 114:911–6. doi: 10.1289/ehp.8559
18. Strickland MJ, Darrow LA, Klein M, Flanders WD, Sarnat JA, Waller LA. Short-term associations between ambient air pollutants and pediatric asthma emergency department visits. Am J Respir Crit Care Med. (2010) 182:307–16. doi: 10.1164/rccm.200908-1201OC
19. Pepper JR, Barrett MA, Su JG, Merchant R, Henderson K, Van Sickle D, et al. Geospatial-temporal analysis of the impact of ozone on asthma rescue inhaler use. Environ Int. (2020) 136:105331. doi: 10.1016/j.envint.2019.105331
20. Molfino NA, Wright SC, Katz I, Tarlo S, Silverman F, McClean PA, et al. Effect of low concentrations of ozone on inhaled allergen responses in asthmatic subjects. Lancet. (1991) 338:199–203. doi: 10.1016/0140-6736(91)90346-q
21. Sokolowska M, Quesniaux VFJ, Akdis CA, Chung KF, Ryffel B, Togbe D. Acute respiratory barrier disruption by ozone exposure in mice. Front Immunol. (2019) 10:2169. doi: 10.3389/fimmu.2019.02169
22. Lee YL, McConnell R, Berhane K, Gilliland FD. Ambient ozone modifies the effect of tumor necrosis factor G-308A on bronchitic symptoms among children with asthma. Allergy. (2009) 64:1342–8. doi: 10.1111/j.1398-9995.2009.02014.x
23. Islam T, McConnell R, Gauderman WJ, Avol E, Peters JM, Gilliland FD. Ozone, oxidant defense genes, and risk of asthma during adolescence. Am J Respir Crit Care Med. (2008) 177:388–95. doi: 10.1164/rccm.200706-863OC
24. Huang J, Song Y, Chu M, Dong W, Miller MR, Loh M, et al. Cardiorespiratory responses to low-level ozone exposure: the inDoor ozone study in childrEn (DOSE). Environ Int. (2019) 131:105021. doi: 10.1016/j.envint.2019.105021
25. Chatkin J, Correa L, Santos U. External environmental pollution as a risk factor for asthma. Clin Rev Allergy Immunol. (2021). 62:1–18. doi: 10.1007/s12016-020-08830-5
26. Orellano P, Quaranta N, Reynoso J, Balbi B, Vasquez J. Effect of outdoor air pollution on asthma exacerbations in children and adults: systematic review and multilevel meta-analysis. PLoS One. (2017) 12:e0174050. doi: 10.1371/journal.pone.0174050
27. Liu Y, Pan J, Zhang H, Shi C, Li G, Peng Z, et al. Short-term exposure to ambient air pollution and asthma mortality. Am J Respir Crit Care Med. (2019) 200:24–32. doi: 10.1164/rccm.201810-1823OC
28. Gehring U, Wijga AH, Hoek G, Vonk JM, Smit HA, Brunekreef B. Exposure to air pollution and development of asthma and rhinoconjunctivitis throughout childhood and adolescence: a population-based birth cohort study. Lancet Respir Med. (2015) 3:933–42. doi: 10.1016/S2213-2600(15)00426-9
29. Wu J, Zhong T, Zhu Y, Ge D, Lin X, Li Q. Effects of particulate matter (PM) on childhood asthma exacerbation and control in Xiamen, China. BMC Pediatr. (2019) 19:194. doi: 10.1186/s12887-019-1530-7
30. Zhu Y, Zhong T, Ge D, Li Q, Wu J. Multi-factor analysis of single-center asthma control in Xiamen, China. Front Pediatr. (2019) 7:498. doi: 10.3389/fped.2019.00498
31. Chen Y, Beig G, Archer-Nicholls S, Drysdale W, Acton WJF, Lowe D, et al. Avoiding high ozone pollution in Delhi, India. Faraday Discuss. (2020). 226:502–14. doi: 10.1039/d0fd00079e
32. Rosenquist NA, Metcalf WJ, Ryu SY, Rutledge A, Coppes MJ, Grzymski JJ, et al. Acute associations between PM and ozone concentrations and asthma exacerbations among patients with and without allergic comorbidities. J Expo Sci Environ Epidemiol. (2020) 30:795–804. doi: 10.1038/s41370-020-0213-7
33. Brankston G, Greer AL, Marshall Q, Lang B, Moore K, Hodgins D, et al. Increased weekly mean PM, and NO are associated with increased proportions of lower airway granulocytes in Ontario horses. Front Vet Sci. (2020) 7:185. doi: 10.3389/fvets.2020.00185
34. Sarnat SE, Winquist A, Schauer JJ, Turner JR, Sarnat JA. Fine particulate matter components and emergency department visits for cardiovascular and respiratory diseases in the St. Louis, Missouri-Illinois, metropolitan area. Environ Health Perspect. (2015) 123:437–44. doi: 10.1289/ehp.1307776
35. Zheng X-Y, Orellano P, Lin H-L, Jiang M, Guan WJ. Short-term exposure to ozone, nitrogen dioxide, and sulphur dioxide and emergency department visits and hospital admissions due to asthma: a systematic review and meta-analysis. Environ Int. (2021) 150:106435. doi: 10.1016/j.envint.2021.106435
36. Nassikas N, Spangler K, Fann N, Nolte CG, Dolwick P, Spero TL, et al. Ozone-related asthma emergency department visits in the US in a warming climate. Environ Res. (2020) 183:109206. doi: 10.1016/j.envres.2020.109206
37. Xiao JN, Du GM, Shi YQ, Wen YY, Yao J, Gao YT, et al. Spatiotemporal distribution pattern of ambient air pollution and its correlation with meteorological factors in Xiamen City. Acta Sci Circumst. (2016) 36:3363–71.
Keywords: asthma attack, ozone, child, case-crossover design, air pollution
Citation: Huang W, Wu J and Lin X (2022) Ozone Exposure and Asthma Attack in Children. Front. Pediatr. 10:830897. doi: 10.3389/fped.2022.830897
Received: 07 December 2021; Accepted: 21 February 2022;
Published: 05 April 2022.
Edited by:
Kostas N. Priftis, National and Kapodistrian University of Athens, GreeceReviewed by:
Salvatore Fasola, National Research Council (CNR), ItalyOlympia Sardeli, University General Hospital Attikon, Greece
Copyright © 2022 Huang, Wu and Lin. This is an open-access article distributed under the terms of the Creative Commons Attribution License (CC BY). The use, distribution or reproduction in other forums is permitted, provided the original author(s) and the copyright owner(s) are credited and that the original publication in this journal is cited, in accordance with accepted academic practice. No use, distribution or reproduction is permitted which does not comply with these terms.
*Correspondence: Jinzhun Wu, MTkyMzczMTIwMUBxcS5jb20=