- 1Translational and Clinical Research Institute, Newcastle University, Newcastle upon Tyne, United Kingdom
- 2Great North Children's Hospital, Paediatric Immunology, Infectious Diseases and Allergy, Newcastle upon Tyne Hospitals NHS Foundation Trust, Newcastle upon Tyne, United Kingdom
Objective: This study aims to assess the performance of biomarkers used for the prediction of bacterial, viral, and fungal infection in immunocompromised children upon presentation with fever.
Methods: We performed a literature search using PubMed and MEDLINE and In-Process & Other Non-indexed Citations databases. Cohort and case–control studies assessing biomarkers for the prediction of bacterial, viral, or fungal infection in immunocompromised children vs. conventional microbiological investigations were eligible. Studies including adult patients were eligible if pediatric data were separately assessable. Data on definitions used for infections, fever, and neutropenia and predictive values were collected. Risk of bias was assessed with the Quality Assessment of Diagnostic Accuracy Studies-2 tool.
Results: Fifty-two studies involving 13,939 febrile episodes in 7,059 children were included. In total, 92.2% were in cancer patients (n = 48), and 15.7% also included hematopoietic stem cell transplantation patients (n = 8). Forty-three biomarkers were investigated, of which 6 (CRP, PCT, IL-8, IL-6, IL-10, and TNFα) were significantly associated with bacterial infection at admission, studied in multiple studies, and provided predictive data. Literature on the prediction of viral and fungal infection was too limited. Eight studies compared C-reactive protein (CRP) and procalcitonin (PCT), with PCT demonstrating superiority in 5. IL-6, IL-8, and IL-10 were compared with CRP in six, four, and one study, respectively, with mixed results on diagnostic superiority. No clear superior biomarker comparing PCT vs. IL-6, IL-8, or IL-10 was identified.
Discussion: There is great heterogeneity in the biomarkers studied and cutoff values and definitions used, thus complicating the analysis. Literature for immunocompromised children with non-malignant disease and for non-bacterial infection is sparse. Literature on novel diagnostics was not available. We illustrated the challenges of diagnosing fever adequately in this study population and the need for improved biomarkers and clinical decision-making tools.
Introduction
Febrile illness is common in children, accounting for 20% of emergency department (ED) attendances (1). Often a self-limiting (viral) illness, 10–15% of febrile children in ED will have serious bacterial infection (SBI) or sepsis (1–4).
Diagnosing fever is challenging in immunocompromised children; often fever is the only symptom. They are at a high risk (HR) for SBI as their immune system cannot combat pathogens adequately. Up to half of solid tumor and ≥80% of hematological malignancy patients have ≥1 episode of febrile neutropenia (5). Moreover, 11.4–31.3% of febrile neutropenic children have positive blood cultures, and 41.3–62.3% have no cause identified (6–9).
Common biomarkers, such as C-reactive protein (CRP) and procalcitonin (PCT), aid us but are often not sensitive enough to rule out a bacterial infection or differentiate other causes of fever (10–12), resulting in admissions for virtually all febrile immunocompromised children for intravenous antibiotics pending microbiological results. The introduction of this approach led to a significant reduction in mortality and morbidity (13).
It is suspected that a significant proportion of febrile illness is deemed viral in nature, caused by medication or the underlying disease itself (14). Unnecessary admissions and antibiotic use could be avoided and the quality of life for patients and families could be improved (15) if diagnostic tests could rule out bacterial infection in ED.
There is a need to investigate new diagnostic markers and clinical decision-making tools to improve the diagnosis of febrile illness in this group. Externally validated tools often exclude the immunocompromised (16). Transcriptomic techniques are increasingly investigated for distinguishing bacterial and viral causes of fever in ED but are not clinically validated (17–19). In immunocompromised patients, two promising studies (20, 21) used transcriptomics to identify biomarkers for bacteremia in patients with febrile neutropenia, but both included adults.
This literature review assessed the biomarkers for diagnosing the etiology of febrile illness in immunocompromised children and their performance.
Methods
The research question for this review was as follows: “Which biomarkers have been studied for diagnosing the etiology of febrile illness in immunocompromised children, and how well do they perform?”
We searched PubMed and MEDLINE and In-Process and Other Non-indexed Citations databases and used the PRISMA 2020 reporting guidelines (22) for this review.
The PubMed search was carried out using the following terms: [“Infant, Newborn”(MeSH) OR “Infant”(MeSH) OR “Child”(MeSH) OR “Child, Preschool”(MeSH) OR “Adolescent”(MeSH)]
AND [“Neoplasms”(MeSH) OR “Immunologic Deficiency Syndromes”(MeSH) OR “Neutropenia”(MeSH) OR “Immunocompromised Host”(MeSH) OR “Hematopoietic Stem Cell Transplantation”(MeSH) OR “Transplant Recipient”(MeSH) OR “Fever”(MeSH) OR “Febrile Neutropenia”(MeSH) OR “neutropenic fever”(TIAB)]
AND [“Biomarkers”(MeSH) OR “Cytokines”(MeSH)] AND [“Bacterial Infections”(MeSH) OR “Virus Diseases”(MeSH) OR “Mycoses”(MeSH) OR “Bacteremia”(MeSH) OR “Sepsis”(MeSH)]. The MEDLINE search is described in Supplementary Data 1.
No language restrictions nor any filters were applied, and relevant Spanish or German publications were translated.
Based on the Population, Intervention, Comparison, Outcome, and Settings (22) criteria, the inclusion criteria were HR children ≤ 18 years of age presenting with fever or suspected infection. HR children were immunocompromised due to primary (PID) or secondary immunodeficiency, with biomarkers assessed for the predictive ability of bacterial, viral, or other infection. The outcomes considered were as follows: SBI, bacteremia, viremia, fungemia, clinically documented infection, microbiologically documented infection, sepsis, septic shock, clinical deterioration, and pediatric intensive care unit (PICU) or high dependency unit admission. These had to be cohort or case–control studies. Studies combining children and adults were considered if pediatric data was assessable separately.
Data Extraction and Bias Assessment
Study data were collected on demographics, the study's inclusion and exclusion criteria, definitions used, clinical characteristics, biomarkers, and outcome.
For every biomarker, data were extracted on cutoff values, specificity, sensitivity, and positive and negative predictive values. If unavailable, outcome assessment was performed by using reported measures of central tendency plus spread.
Risk of bias was assessed using the Quality Assessment of Diagnostic Accuracy Studies (QUADAS-2) tool (23), which was slightly modified: the quality item “time between index test and reference test were appropriate” was not deemed discriminative because the index (biomarker) and the reference test (conventional microbiology) were obtained simultaneously.
Results
Database searches were performed on November 23, 2021 and yielded 884 potential articles. Eighty-eight studies were analyzed in-depth after title and abstract screening and removal of duplicates. Thirty-six studies were excluded: pediatric data inseparable from adult data (n = 26), inclusion of non-HR patients (n = 2), not relevant study design (n = 4), not in English or unable to translate (n = 2), inappropriate interventions or outcomes (n = 1), and full text unavailable (n = 1). In total, 52 articles were included (Figure 1).
Study and Population Characteristics
Across the included studies, 13,939 episodes of 7,059 children from 20 countries were analyzed. The median age ranged between 3.0 and 9.9 years. Eight studies did not provide age data for their whole cohort, only subgroups (24–31).
The majority of studies used pediatric cancer patients as their immunocompromised group (92.2%), and 8 (15.7%) explicitly stated the inclusion of hematopoietic stem cell transplant (HSCT) patients. Three studies (5.9%) included solid organ transplant recipients (Table 1).
The definitions of fever were comparable between the studies, with most using a single temperature ≥38.5°C (40.4%) or ≥38.3°C (21.2%) combined with a sustained temperature ≥38.0°C within 1-, 4-, 6-, 12-, or 24-h interval (59.6%). Eight studies did not formally state their definition of fever.
The definitions of neutropenia were mostly consistent, defined as absolute neutrophil count (ANC) <0.5 × 109/L (n = 37, 71.2%), but with 7 studies defining neutropenia as ANC <1.0 × 109/L. Seven studies expanded their definition to include initial ANC <1.0 × 109/L if it is expected to drop to <0.5 × 109/L within 24–72 h. Four studies additionally defined neutropenia as white cell count <1.0 × 109/L if no ANC was available. Eight studies did not state their definitions.
The outcome measures were reported as bacteremia, clinically documented infection, microbiologically documented infection, clinical sepsis, fever of unknown origin (FUO), fungemia, localized infection, sepsis, septic shock, severe infection, and viremia. Other outcomes such as graft-vs.-host disease, length of stay, PICU admission, and prolonged fever were usually not reported in the context of biomarker performance in the included studies.
Overall, 52 studies analyzed 43 biomarkers, of which 24 only were in one study each. Most of the studied biomarkers were CRP (n = 37, 71.2%), PCT (n = 26, 50.0%), interleukin (IL)-6 (n = 23, 44.2%), and IL-8 (n = 22, 42.3%) (Figure 2). In total, 47 studies assessed ≥1 biomarker in their respective study population.
Quality and Bias Assessment
All studies underwent risk of bias assessment using QUADAS-2 (Figure 3, detailed in Supplementary Data 2).
Twenty-four studies were of fair quality (46.1%), 22 studies were good (42.3%), and six studies were poor (11.5%). The selection process was inadequately described in six studies, and six studies did not state if they were retrospective or prospective. Seven studies did not clearly state how inappropriate exclusions were avoided.
The majority of studies did not state if the index test results had blinded interpretation independent of the reference standard test (86.3%). The reference standards were clearly described.
Regarding “flow and timing,” in two studies (9, 33), it was unclear if all patients received the same reference standard. Fifteen studies did not state if all patients were accounted for. Two studies (9, 42) may have introduced patient flow bias, as one did not state the index test timepoints, and in one >50% of patients did not receive the index test at the prespecified timepoint.
Biomarker Assessment for Predicting Bacterial Infection at Admission
Eighteen biomarkers were not discriminatory at admission for the prediction of bacterial infection. For Tregs (27), Neoptrin (40), and IL-12 (26), only one study each found a significant association between the increased levels of the respective biomarker and bacterial infection. Four biomarkers were assessed in a combined model in one study (45) and were excluded from further analysis as the available literature is too limited for effective comparison.
For 22 biomarkers, significant associations were described between biomarker and predicting bacterial infection at admission (Table 2). CRP, PCT, IL-6, IL-8, tumor necrosis factor alpha (TNFα), and IL-10 were the most studied biomarkers and had ≥2 studies statistically analyzing their predictive performance.
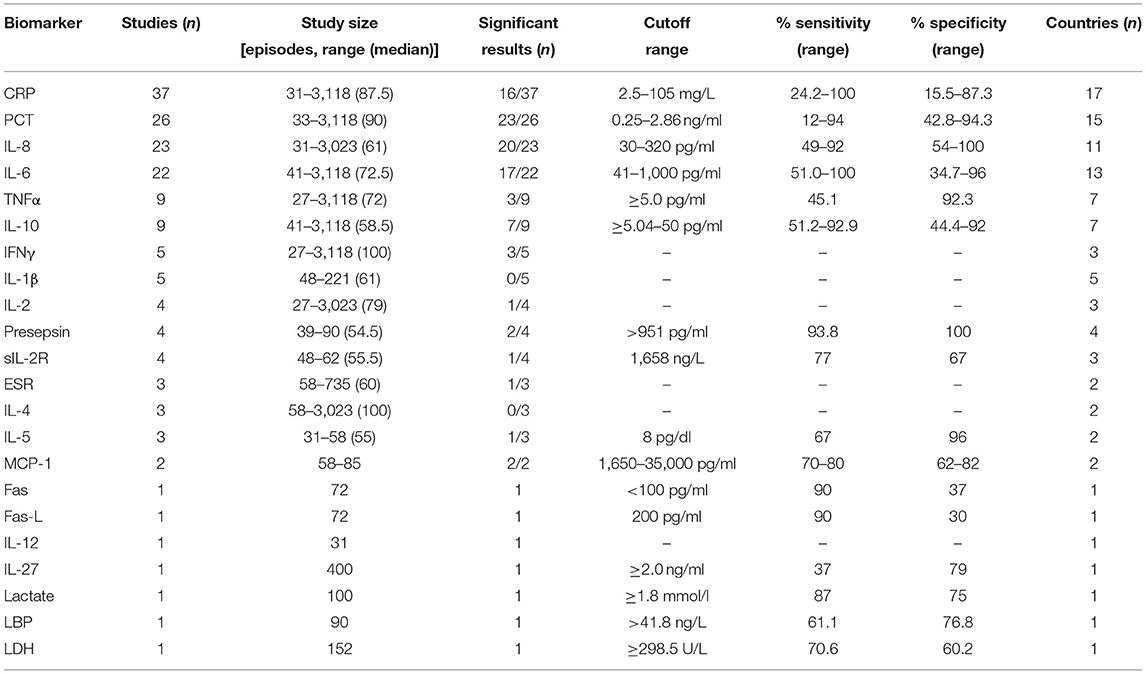
Table 2. Biomarker performance for biomarkers significantly associated with bacterial infection at admission.
Erythrocyte sedimentation rate, interferon gamma, IL-1β, IL-2, and, IL-4 were studied in multiple studies and found to have elevated levels associated with bacterial infection compared to controls or non-bacterial infection, but no performance analysis was described (27, 30–32, 38, 41, 60, 66, 67, 72–74). For presepsin, soluble IL-2 receptor, IL-5, and monocyte chemotactic protein-1, multiple studies did assess the potential for predicting bacterial infection, but only one study per biomarker stated the sensitivity and specificity of data (Table 2).
CRP
CRP is an acute phase inflammatory cytokine induced by IL-6. The concentrations during an infection depend on the extent of tissue destruction, presence of malignant disease, and fever duration. It increases in the first 24–48 h after the onset of inflammation. It is known for its poor specificity (10, 12).
Thirty-seven studies assessed CRP as a biomarker for bacterial infection for HR children. Sixteen of these (41.6%) found significant associations between elevated CRP and bacterial infection, either microbiologically or clinically confirmed. Moreover, 14/16 significant studies provided predictive data with various cutoff values (range: 5–120 mg/L) (6, 9, 31, 33, 35, 37, 39, 40, 48, 53, 55, 62, 63, 74).
The low cutoff values provided good sensitivity (77.7–92.3%) but poor specificity (15.5–72.2%). The higher cutoff values saw a decrease of sensitivity (24.2–77.8%) and a slight increase of specificity (63–87.3%), yet the values were subpar to be an effective marker.
One study (9) reported 100% sensitivity for a CRP cutoff of 41.2 mg/L, but with 64.3% specificity. As a biomarker for bacterial infection in high-risk children, CRP remains a poor predictor.
PCT
PCT is a 116-amino-acid peptide with the same sequence as the prohormone of calcitonin. Considered an acute-phase protein, the PCT levels are low in healthy individuals. PCT is a stable molecule, and the levels rapidly increase in severe (bacterial) infections, without changing the calcitonin levels. In infections in the immunocompetent, PCT is known to increase earlier than CRP, usually within 2–4 h after onset and with peak levels after 24–48 h in septic patients.
Twenty-six studies assessed PCT for the prediction of bacterial infection, of which 23 found significant associations between increased PCT levels at admission in bacterial infection. Furthermore, 17/23 studies (73.9%) provided predictive data with cutoff values ranging 0.25–2.86 ng/ml. Across the studies, seven different measurement techniques were used. Eighteen used immunoassays, of which most were enzyme-linked immunosorbent assays (ELISA) and LUMItest, at seven and six times, respectively. Two studies used flow cytometry, and one used time-resolved amplified cryptate emission. The cutoff values among PCT studies were variable. The sensitivity ranged between 12 and 94%, with the highest sensitivities reported with a cutoff of 0.25 (72) or 2.0 ng/ml (41). Although the range is smaller (42.8–96.5%), the specificity of PCT turned out to have mixed performance across the cutoff values. A specificity of 94.2% was reported for a cutoff value of 0.3 ng/ml (66), and a specificity of 96.5% was reported for a cutoff of 2.0 ng/ml (41). Lowest specificities of 42.8 and 50% were reported on cutoff values of 0.25 and 0.38 ng/ml (71, 72).
IL-8
IL-8 is a pro-inflammatory cytokine released from monocytes, endothelial cells, and fibroblasts. Production is induced by IL-1, TNFα, and lipopolysaccharide-binding protein. The IL-8 levels increase more quickly than CRP and are reported to rise before fever onset (75, 76).
Twenty-two studies assessed IL-8, of which 19 found a significant association between increased IL-8 levels and infection. Five techniques for IL-8 measurement were used across the studies. The most common were ELISA in 12/22 studies, followed by five studies utilizing flow cytometry. In total, 9/22 studies analyzed IL-8's predictive properties, with cutoff values ranging 30–320 pg/ml. The sensitivities reported were similar across the cutoff values (56–92%), and the specificity tended to be higher if a higher cutoff value was used, with a cutoff of 30 pg/ml showing specificity of 59% (36) and a cutoff value of 320 pg/ml yielding 79–100% specificity.
IL-6
IL-6 is a pleiotropic cytokine produced by T-lymphocytes, macrophages, and endothelial cells. Synthesis and secretion are stimulated by IL-1. It influences multiple processes: upregulating neutrophil production, B-lymphocyte proliferation, and acute phase protein synthesis induction. It plays a vital role in trans-signaling in sepsis pathogenesis, and the levels peak early in the illness (26).
Twenty-two studies analyzed IL-6 use. In total, 17/22 studies (77.3%) reported significant associations between elevated IL-6 and bacterial infection. Six measurement techniques were utilized, the most common being ELISA in 11/22 studies. Of 17 studies with significant associations, 12 reported predictive data with cutoff values ranging 41.8–1,000 pg/ml. Higher cutoffs provided higher specificity, and lower cutoffs provided higher sensitivity. IL-6 sensitivity ranged 50–100%, and the specificity was 34.7–96%. Moreover, 100% sensitivity was reported with 60-pg/ml cutoff; however, the specificity was 34.7% (72). A cutoff of 235 pg/ml provided the best specificity at 91%, with a sensitivity of 89% (50).
TNFα
TNFα, a small cytokine secreted by macrophages and other antigen-presenting cells, induces immune response by stimulating T-lymphocyte activation. It subsequently promotes humoral and cellular immune responses via cell signaling (77).
Nine studies assessed TNFα, and three found significant associations between the elevated levels of TNFα and bacterial infection. Only one study calculated the predictive data, reporting a sensitivity of 45.1% and specificity of 92.3% using a cutoff value of 5.0 pg/ml (73).
IL-10
IL-10, an anti-inflammatory cytokine produced by macrophages and T-helper lymphocytes, plays an essential role in limiting the inflammatory process and preventing excessive host damaging (78).
Nine studies analyzed IL-10 as a predictive biomarker, with six studies reporting significant associations between the elevated IL-10 levels and bacterial or severe infection. Immunoassays were used in four and flow cytometry in five. In total, five different reported diagnostic measurement techniques were used.
Two studies report the predictive data. Sahbudak et al. (62) reported a sensitivity of 92.9% and specificity of 44.4% for a cutoff of 5.04 pg/ml in predicting a bacterial infection.
Xia et al. (31) calculated the predictive performance for IL-10 and gram-negative bacteremia and for IL-10 and severe infection. At a cutoff of 32.3 pg/ml, IL-10 had optimal sensitivity for predicting gram-negative bacteremia at 69.2% and optimal specificity for a cutoff of 100 pg/ml at 84.8%.
For severe infection, highest sensitivity and specificity were calculated at a cutoff of 49.5 pg/ml at 77.6 and 94.9% respectively.
Biomarker Assessment for Viral and Fungal Infection at Admission
Twelve studies reported on biomarkers for viral infection prediction, and 10 studies reported on fungal infection (Table 1). For viral infection, two studies reported significantly elevated levels for IL-6 (38), and one study reported significantly elevated CRP (31, 34), but the numbers were too low to draw conclusions.
For fungal infections, one study (34) found increased PCT and CRP levels in children with fungal infection. There was no further statistical analysis performed regarding performance.
Comparison of Biomarkers for Predicting Bacterial Infection
Mostly compared were CRP and PCT, with eight studies comparing their performance. Five of these found PCT to be performing better (34, 40, 41, 65, 72), one found CRP (63) to be performing better, and two studies found neither to be outperforming (6, 46).
CRP was also compared to IL-6 (6, 36, 46, 68, 72, 74), IL-8 (36, 46, 63, 64), and IL-10 (74). CRP did not outperform these ILs in any of these studies. IL-6, IL-8, and IL-10 outperformed CRP in 3/6 studies. In the remaining studies, both biomarkers performed equally.
The comparative performance of PCT and ILs was explored in six studies for IL-6 (6, 13, 31, 41, 72, 74), 5 studies for IL-8 (13, 41, 46, 63, 72), and two studies for IL-10 (31, 74). Two studies found IL-6 and two found PCT to be better compared to each other, respectively, and in two they performed equally. For IL-8 and PCT, it showed a similar mixed picture. The two studies comparing PCT and IL-10 were in favor of IL-10. Comparisons IL-6 and IL-8 were reported in five studies (36, 46, 62, 67, 72), with two favoring IL-6 and one favoring IL-8, and two could not state a better biomarker.
Discussion
This review analyzed 51 studies, including 43 biomarkers for infection prediction in immunocompromised children. We illustrate the ongoing quest for better diagnostics for fever in this vulnerable group and did not limit to patients presenting with febrile neutropenia, aiming to reflect clinical practice more truthfully.
In total, 39.5% of the studied biomarkers were not discriminatory between bacterial and non-bacterial causes of febrile illness. Literature assessing biomarker performance in predicting viral or fungal disease was sparse, although potentially equally important causes of a severe illness in this group (79).
Most studies were performed in children with malignancy. HSCT, PID, and solid organ transplant patients are underrepresented.
Across studies, the definitions of fever and neutropenia were quite consistent, with recent studies providing more consistent definitions, thus allowing a better comparison.
The differences between the groups and definitions used lead to heterogeneity—for example, bacteremia, sepsis, severe infection, microbiologically or clinically documented infection, localized infection, sepsis, and bacteremia were used, which are not equally interchangeable. In biomarker predictive performance assessment, we observed that, not unexpectedly, by increasing the cutoff levels, the sensitivities decreased and the specificities increased. The literature shows that many biomarkers have no normal range of cutoff value identified. The techniques used for biomarker measurement varied across the studies, complicating the interpretation of cutoff values for biomarker performance, especially for PCT, as 7 different methods of measurement were used.
Of all biomarkers assessed, CRP, PCT, and IL-6/8/10 were the most thoroughly studied. In studies comparing PCT and CRP, PCT was felt superior to CRP for predicting bacterial infection (2, 42, 50, 64). Kitanovski et al. (6) found that PCT in this group performed well in predicting systemic bacterial infections, but not for focal infections.
In HSCT patients, an elevated PCT level is also associated with other transplant-related adverse events (34, 38).
The literature shows a tendency to favor IL-6 and IL-8 over CRP, having better specificities.
There is insufficient evidence for these markers to replace CRP in current practice. Most studies also assessed the use of biomarkers for discriminating between non-bacterial and bacterial infection. The role of inflammatory illness has not yet been well-explored in the HR population. IL-6 might have a role in the prediction of gram-negative bacterial infection in HR patients, as both studies in cancer patients and HSCT patients describe significantly higher IL-6 levels compared to CRP and IL-8 (31, 43, 50, 67, 73, 74). Similar statements are made for IL-8 and performance in the prediction of gram-negative infection (49, 62, 67).
Although many studies have been conducted on this topic, generalizability remains a challenge due to methodological inconsistency. Definitions changed over time for important features of febrile illness in this group, e.g., fever and neutropenia, although more methodological consistency is observed in recent literature. Furthermore, the outcome measures would benefit from more uniformity. We observed a variety of outcomes in the studies, which are similar but not interchangeable, such as bacteremia and sepsis, or which are less commonly used, such as clinically documented infection. We acknowledge that this will be challenging, as clinical definitions in the field of febrile illness are still a topic of debate.
Novel diagnostics, e.g., host RNA biosignatures, have proven able to accurately differentiate between viral and bacterial infections in immunocompetent children, with sensitivities of 94–98% and specificities of 91–96% (11, 12). It is unknown if a similar approach is feasible for immunocompromised HR children, particularly those with low cell counts.
In conclusion, this review shows that adequately diagnosing febrile illness in immunocompromised children at ED remains challenging. Better diagnostics are needed for this population.
Data Availability Statement
The raw data supporting the conclusions of this article will be made available by the authors, without undue reservation.
Author Contributions
FV, AG, and ME wrote the manuscript. FV was responsible for the literature search, search strategies, study selection, data extraction, bias assessment, and analysis. ME and AG reviewed and supervised FV during these processes. All co-authors reviewed the manuscript and agreed to its submission for publication.
Funding
FV and ME were funded by the European Union's Horizon 2020 research and innovation program, under grant agreement numbers 668303 and 848196.
Conflict of Interest
The authors declare that the research was conducted in the absence of any commercial or financial relationships that could be construed as a potential conflict of interest.
Publisher's Note
All claims expressed in this article are solely those of the authors and do not necessarily represent those of their affiliated organizations, or those of the publisher, the editors and the reviewers. Any product that may be evaluated in this article, or claim that may be made by its manufacturer, is not guaranteed or endorsed by the publisher.
Supplementary Material
The Supplementary Material for this article can be found online at: https://www.frontiersin.org/articles/10.3389/fped.2022.828569/full#supplementary-material
Supplementary Data 1. Search strategy for Medline.
Supplementary Data 2. In-depth QUADAS-2 assessment for each study.
References
1. Van den Bruel A, Thompson M. Research into practice: acutely ill children. Br J Gen Pract. (2014) 64:311–3. doi: 10.3399/bjgp14X680317
2. Martinon-Torres F, Salas A, Rivero-Calle I, Cebey-Lopez M, Pardo-Seco J, Herberg JA, et al. Life-threatening infections in children in Europe (the EUCLIDS Project): a prospective cohort study. Lancet Child Adolesc Health. (2018) 2:404–14. doi: 10.1016/S2352-4642(18)30113-5
3. Ladhani S, Pebody RG, Ramsay ME, Lamagni TL, Johnson AP, Sharland M. Continuing impact of infectious diseases on childhood deaths in England and Wales, 2003-2005. Pediatr Infect Dis J. (2010) 29:310–3. doi: 10.1097/INF.0b013e3181d73322
4. Roukema J, Steyerberg EW, van der Lei J, Moll HA. Randomized trial of a clinical decision support system: impact on the management of children with fever without apparent source. J Am Med Inform Assoc. (2008) 15:107–13. doi: 10.1197/jamia.M2164
5. Klastersky J. Management of fever in neutropenic patients with different risks of complications. Clin Infect Dis. (2004) 39(Suppl. 1):S32–7. doi: 10.1086/383050
6. Kitanovski L, Jazbec J, Hojker S, Derganc M. Diagnostic accuracy of lipopolysaccharide-binding protein for predicting bacteremia/clinical sepsis in children with febrile neutropenia: comparison with interleukin-6, procalcitonin, and C-reactive protein. Support Care Cancer. (2014) 22:269–77. doi: 10.1007/s00520-013-1978-1
7. Aimoto M, Koh H, Katayama T, Okamura H, Yoshimura T, Koh S, et al. Diagnostic performance of serum high-sensitivity procalcitonin and serum C-reactive protein tests for detecting bacterial infection in febrile neutropenia. Infection. (2014) 42:971–9. doi: 10.1007/s15010-014-0657-6
8. Urbonas V, Eidukaite A, Tamuliene I. The diagnostic value of interleukin-6 and interleukin-8 for early prediction of bacteremia and sepsis in children with febrile neutropenia and cancer. J Pediatr Hematol Oncol. (2012) 34:122–7. doi: 10.1097/MPH.0b013e3182446a60
9. Baraka A, Zakaria M. Presepsin as a diagnostic marker of bacterial infections in febrile neutropenic pediatric patients with hematological malignancies. Int J Hematol. (2018) 108:184–91. doi: 10.1007/s12185-018-2447-x
10. Randolph AG, McCulloh RJ. Pediatric sepsis: important considerations for diagnosing and managing severe infections in infants, children, and adolescents. Virulence. (2014) 5:179–89. doi: 10.4161/viru.27045
11. Herberg JA, Kaforou M, Wright VJ, Shailes H, Eleftherohorinou H, Hoggart CJ, et al. Diagnostic test accuracy of a 2-transcript host RNA signature for discriminating bacterial vs viral infection in febrile children. JAMA. (2016) 316:835–45. doi: 10.1001/jama.2016.11236
12. Srugo I, Klein A, Stein M, Golan-Shany O, Kerem N, Chistyakov I, et al. Validation of a novel assay to distinguish bacterial and viral infections. Pediatrics. (2017) 140:e20163453. doi: 10.1542/peds.2016-3453
13. Stryjewski GR, Nylen ES, Bell MJ, Snider RH, Becker KL, Wu A, et al. Interleukin-6, interleukin-8, and a rapid and sensitive assay for calcitonin precursors for the determination of bacterial sepsis in febrile neutropenic children. Pediatr Crit Care Med. (2005) 6:129–35. doi: 10.1097/01.PCC.0000149317.15274.48
14. Hakim H, Flynn PM, Knapp KM, Srivastava DK, Gaur AH. Etiology and clinical course of febrile neutropenia in children with cancer. J Pediatr Hematol Oncol. (2009) 31:623–9. doi: 10.1097/MPH.0b013e3181b1edc6
15. Crothers A, Haeusler GM, Slavin MA, Babl FE, Mechinaud F, Phillips R, et al. Examining health-related quality of life in pediatric cancer patients with febrile neutropenia: factors predicting poor recovery in children and their parents. EClinicalMedicine. (2021) 40:101095. doi: 10.1016/j.eclinm.2021.101095
16. Nijman RG, Vergouwe Y, Moll HA, Smit FJ, Weerkamp F, Steyerberg EW, et al. Validation of the Feverkidstool and procalcitonin for detecting serious bacterial infections in febrile children. Pediatr Res. (2018) 83:466–76. doi: 10.1038/pr.2017.216
17. Ramilo O, Allman W, Chung W, Mejias A, Ardura M, Glaser C, et al. Gene expression patterns in blood leukocytes discriminate patients with acute infections. Blood. (2007) 109:2066–77. doi: 10.1182/blood-2006-02-002477
18. Hu X, Yu J, Crosby SD, Storch GA. Gene expression profiles in febrile children with defined viral and bacterial infection. Proc Natl Acad Sci USA. (2013) 110:12792–7. doi: 10.1073/pnas.1302968110
19. Warhurst G, Dunn G, Chadwick P, Blackwood B, McAuley D, Perkins GD, et al. Rapid detection of health-care-associated bloodstream infection in critical care using multipathogen real-time polymerase chain reaction technology: a diagnostic accuracy study and systematic review. Health Technol Assess. (2015) 19:1–142. doi: 10.3310/hta19350
20. Kelly RS, Lasky-Su J, Yeung SJ, Stone RM, Caterino JM, Hagan SC, et al. Integrative omics to detect bacteremia in patients with febrile neutropenia. PLoS ONE. (2018) 13:e0197049. doi: 10.1371/journal.pone.0197049
21. Weycker D, Barron R, Kartashov A, Legg J, Lyman GH. Incidence, treatment, and consequences of chemotherapy-induced febrile neutropenia in the inpatient and outpatient settings. J Oncol Pharm Pract. (2014) 20:190–8. doi: 10.1177/1078155213492450
22. Page MJ, McKenzie JE, Bossuyt PM, Boutron I, Hoffmann TC, Mulrow CD, et al. The PRISMA 2020 statement: an updated guideline for reporting systematic reviews. BMJ. (2021) 372:n71. doi: 10.1136/bmj.n71
23. Whiting PF, Rutjes AW, Westwood ME, Mallett S, Deeks JJ, Reitsma JB, et al. QUADAS-2: a revised tool for the quality assessment of diagnostic accuracy studies. Ann Intern Med. (2011) 155:529–36. doi: 10.7326/0003-4819-155-8-201110180-00009
24. Badurdeen S, Hodge G, Osborn M, Scott J, St John-Green C, Tapp H, et al. Elevated serum cytokine levels using cytometric bead arrays predict culture-positive infections in childhood oncology patients with febrile neutropenia. J Pediatr Hematol Oncol. (2012) 34:e36–8. doi: 10.1097/MPH.0b013e3182193009
25. Gunasekaran V, Radhakrishnan N, Dinand V, Sachdeva A. Serum procalcitonin for predicting significant infections and mortality in pediatric oncology. Indian Pediatr. (2016) 53:1075–8.
26. Hodge G, Osborn M, Hodge S, Nairn J, Tapp H, Kirby M, et al. Rapid simultaneous measurement of multiple cytokines in childhood oncology patients with febrile neutropenia: increased interleukin (IL)-8 or IL-5 correlates with culture-positive infection. Br J Haematol. (2006) 132:247–8. doi: 10.1111/j.1365-2141.2005.05870.x
27. Hodge G, Scott J, Osborn M, To LB, Zola H, Hodge S, et al. Increased T regulatory cells and decreased Th1 pro-inflammatory cytokines correlate with culture-positive infection in febrile neutropenia childhood oncology patients. Cytokine. (2011) 53:286–8. doi: 10.1016/j.cyto.2010.11.009
28. Miedema KG, de Bont ES, Elferink RF, van Vliet MJ, Nijhuis CS, Kamps WA, et al. The diagnostic value of CRP, IL-8, PCT, and sTREM-1 in the detection of bacterial infections in pediatric oncology patients with febrile neutropenia. Support Care Cancer. (2011) 19:1593–600. doi: 10.1007/s00520-010-0987-6
29. Ruggiero A, Pocino K, Catalano M, Maurizi P, D'Ambra M, Rizzo D, et al. Serum biomarkers for sepsis in children with febrile neutropenia and cancer. J Biol Regul Homeost Agents. (2019) 33:999–1003.
30. Vyles D, Gnagi F, Bulloch B, Muenzer J, Hu C. Procalcitonin as a marker of bacteremia in patients with fever and acute lymphoblastic leukemia. Pediatr Emerg Care. (2016) 32:590–3. doi: 10.1097/PEC.0000000000000660
31. Xia T, Xu X, Zhao N, Luo Z, Tang Y. Comparison of the diagnostic power of cytokine patterns and procalcitonin for predicting infection among paediatric haematology/oncology patients. Clin Microbiol Infect. (2016) 22:996–1001. doi: 10.1016/j.cmi.2016.09.013
32. Aquino VM, Cost C, Gomez A, Bowers DC, Ramilo O, Ahmad N, et al. Predictive value of interleukin-5 and monocyte chemotactic protein-1 for bacteremia in children with febrile neutropenia. J Pediatr Hematol Oncol. (2012) 34:e241–5. doi: 10.1097/MPH.0b013e31824e498d
33. Ashkenazi-Hoffnung L, Davidovits M, Bilavsky E, Yassin R, Rom E, Amir J. Children after renal transplantation hospitalized for fever: is empirical antibiotic treatment always justified? Pediatr Transplant. (2017) 21:e12862. doi: 10.1111/petr.12862
34. Cabanillas Stanchi KM, Queudeville M, Malaval C, Feucht J, Schlegel P, Dobratz M, et al. Comparison of procalcitonin and C-reactive protein as early diagnostic marker for the identification of transplant-related adverse events after allogeneic hematopoietic stem cell transplantation in pediatric patients. J Cancer Res Clin Oncol. (2019) 145:2779–91. doi: 10.1007/s00432-019-03008-9
35. Delebarre M, Garnier N, Macher E, Thebaud E, Mazingue F, Leblond P, et al. Which variables are useful for predicting severe infection in children with febrile neutropenia? J Pediatr Hematol Oncol. (2015) 37:e468–74. doi: 10.1097/MPH.0000000000000440
36. Diepold M, Noellke P, Duffner U, Kontny U, Berner R. Performance of interleukin-6 and interleukin-8 serum levels in pediatric oncology patients with neutropenia and fever for the assessment of low-risk. BMC Infect Dis. (2008) 8:28. doi: 10.1186/1471-2334-8-28
37. Doerflinger M, Haeusler GM, Li-Wai-Suen CSN, Clark JE, Slavin M, Babl FE, et al. Procalcitonin and interleukin-10 may assist in early prediction of bacteraemia in children with cancer and febrile neutropenia. Front Immunol. (2021) 12:641879. doi: 10.3389/fimmu.2021.641879
38. Döring M, Cabanillas Stanchi KM, Mezger M, Erbacher A, Feucht J, Pfeiffer M, et al. Cytokine serum levels during post-transplant adverse events in 61 pediatric patients after hematopoietic stem cell transplantation. BMC Cancer. (2015) 15:607. doi: 10.1186/s12885-015-1616-z
39. El-Maghraby SM, Moneer MM, Ismail MM, Shalaby LM, El-Mahallawy HA. The diagnostic value of C-reactive protein, interleukin-8, and monocyte chemotactic protein in risk stratification of febrile neutropenic children with hematologic malignancies. J Pediatr Hematol Oncol. (2007) 29:131–6. doi: 10.1097/MPH.0b013e3180308770
40. Hatzistilianou M, Rekleity A, Athanassiadou F, DeLutiis MA, Conti P, Catriu D. Serial procalcitonin responses in infection of children with secondary immunodeficiency. Clin Invest Med. (2007) 30:E75–85. doi: 10.25011/cim.v30i2.983
41. Hatzistilianou M, Rekliti A, Athanassiadou F, Catriu D. Procalcitonin as an early marker of bacterial infection in neutropenic febrile children with acute lymphoblastic leukemia. Inflamm Res. (2010) 59:339–47. doi: 10.1007/s00011-009-0100-0
42. Hazan G, Ben-Shimol S, Fruchtman Y, Abu-Quider A, Kapelushnik J, Moser A, et al. Clinical and laboratory parameter dynamics as markers of blood stream infections in pediatric oncology patients with fever and neutropenia. J Pediatr Hematol Oncol. (2014) 36:e275–9. doi: 10.1097/MPH.0000000000000057
43. Heney D, Lewis IJ, Evans SW, Banks R, Bailey CC, Whicher JT. Interleukin-6 and its relationship to C-reactive protein and fever in children with febrile neutropenia. J Infect Dis. (1992) 165:886–90. doi: 10.1093/infdis/165.5.886
44. Jacobs L, Berrens Z, Stenson EK, Zackoff M, Danziger-Isakov L, Lahni P, et al. Interleukin-27 as a candidate diagnostic biomarker for bacterial infection in immunocompromised pediatric patients. PLoS ONE. (2018) 13:e0207620. doi: 10.1371/journal.pone.0207620
45. Jacobs L, Berrens Z, Stenson EK, Zackoff MW, Danziger LA, Lahni P, et al. The Pediatric Sepsis Biomarker Risk Model (PERSEVERE) biomarkers predict clinical deterioration and mortality in immunocompromised children evaluated for infection. Sci Rep. (2019) 9:424. doi: 10.1038/s41598-018-36743-z
46. Karakurt DG, Demirsoy U, Corapcioglu F, Oncel S, Karadogan M, Arisoy ES. Do proinflammatory cytokine levels predict serious complication risk of infection in pediatric cancer patients? Pediatr Hematol Oncol. (2014) 31:415–24. doi: 10.3109/08880018.2013.848387
47. Kassam A, Chan AK, Dzolganovski B, Constantin J, Ramphal R, Grant R, et al. No association between protein C levels and bacteremia in children with febrile neutropenia. J Pediatr Hematol Oncol. (2009) 31:647–50. doi: 10.1097/MPH.0b013e3181b1ec89
48. Kitanovski L, Jazbec J, Hojker S, Gubina M, Derganc M. Diagnostic accuracy of procalcitonin and interleukin-6 values for predicting bacteremia and clinical sepsis in febrile neutropenic children with cancer. Eur J Clin Microbiol Infect Dis. (2006) 25:413–5. doi: 10.1007/s10096-006-0143-x
49. Lehrnbecher T, Venzon D, de Haas M, Chanock SJ, Kühl J. Assessment of measuring circulating levels of interleukin-6, interleukin-8, C-reactive protein, soluble Fc gamma receptor type III, and mannose-binding protein in febrile children with cancer and neutropenia. Clin Infect Dis. (1999) 29:414–9. doi: 10.1086/520224
50. Lehrnbecher T, Fleischhack G, Hanisch M, Deinlein F, Simon A, Bernig T, et al. Circulating levels and promoter polymorphisms of interleukins-6 and 8 in pediatric cancer patients with fever and neutropenia. Haematologica. (2004) 89:234–6.
51. Li F, Zhang W, Hu H, Zhang Y, Huang D. Diagnostic value of procalcitonin, C-reactive protein and lactate dehydrogenase in paediatric malignant solid tumour concurrent with infection and tumour progression. Sci Rep. (2019) 9:5903. doi: 10.1038/s41598-019-42264-0
52. Garner JS, Jarvis WR, Emori TG, Horan TC, Hughes JM. CDC definitions for nosocomial infections, 1988. Am J Infect Control. (1988) 16:128–40. doi: 10.1016/0196-6553(88)90053-3
53. Martinez-Albarran M, Perez-Molina Jde J, Gallegos-Castorena S, Sanchez-Zubieta F, Del Toro-Arreola S, Troyo-Sanroman R, et al. Procalcitonin and C-reactive protein serum levels as markers of infection in a pediatric population with febrile neutropenia and cancer. Pediatr Hematol Oncol. (2009) 26:414–25. doi: 10.3109/08880010903044797
54. Miedema KG, Vermont CL, Ball LM, de Bont ES, Kamps WA, van Tol MJ, et al. The diagnostic value of interleukin-8 for the detection of bacteremia in pediatric hematopoietic stem cell recipients with febrile neutropenia. Transplantation. (2014) 98:e80–1. doi: 10.1097/TP.0000000000000434
55. Nath SR, Jayapalan S, Nair H, Kusumakumary P, Prema NS, Priyakumari T, et al. Comparative diagnostic test evaluation of serum procalcitonin and C-reactive protein in suspected bloodstream infections in children with cancer. J Med Microbiol. (2017) 66:622–7. doi: 10.1099/jmm.0.000478
56. Özdemir ZC, Düzenli-Kar Y, Canik A, Küskü-Kiraz Z, Özen H, Bör Ö. The predictive value of procalcitonin, C-reactive protein, presepsin, and soluble-triggering receptor expressed on myeloid cell levels in bloodstream infections in pediatric patients with febrile neutropenia. Turk J Pediatr. (2019) 61:359–67. doi: 10.24953/turkjped.2019.03.007
57. Pacheco-Rosas DO, Huelgas-Plaza AC, Miranda-Novales MG. [Serum lactate as a biomarker of severe sepsis in children with cancer, neutropenia and fever]. Rev Med Inst Mex Seguro Soc. (2014) 52(Suppl. 2):S24–9.
58. Goldstein B, Giroir B, Randolph A, International International Consensus Conference on Pediatric S. International pediatric sepsis consensus conference: definitions for sepsis and organ dysfunction in pediatrics. Pediatr Crit Care Med. (2005) 6:2–8. doi: 10.1097/01.PCC.0000149131.72248.E6
59. Reyna-Figueroa J, Lagunas-Martínez A, Galindo-Delgado P, Fernández-Bautista MF, Castro-Oteo PG, Martínez-Matsumoto P, et al. Serum concentrations of apoptosis-associated molecules in septic children with leukemia, neutropenia and fever. Int J Hematol. (2017) 105:668–75. doi: 10.1007/s12185-016-2175-z
60. Riikonen P, Saarinen UM, Teppo AM, Metsärinne K, Fyhrquist F, Jalanko H. Cytokine and acute-phase reactant levels in serum of children with cancer admitted for fever and neutropenia. J Infect Dis. (1992) 166:432–6. doi: 10.1093/infdis/166.2.432
61. Riikonen P, Jalanko H, Hovi L, Saarinen UM. Fever and neutropenia in children with cancer: diagnostic parameters at presentation. Acta Paediatr. (1993) 82:271–5. doi: 10.1111/j.1651-2227.1993.tb12658.x
62. Sahbudak Bal Z, Karadaş Özdemir N, Sen S, Yilmaz Karapinar D, Azarsiz E, Aydemir S, et al. Diagnostic accuracy of interleukin-6, interleukin-8, and interleukin-10 for predicting bacteremia in children with febrile neutropenia. Turk J Haematol. (2017) 34:254–7. doi: 10.4274/tjh.2016.0434
63. Santolaya ME, Alvarez AM, Aviles CL, Becker A, King A, Mosso C, et al. Predictors of severe sepsis not clinically apparent during the first twenty-four hours of hospitalization in children with cancer, neutropenia, and fever: a prospective, multicenter trial. Pediatr Infect Dis J. (2008) 27:538–43. doi: 10.1097/INF.0b013e3181673c3c
64. Santolaya ME, Alvarez AM, Avilés CL, Becker A, Venegas M, O'Ryan M, et al. Prospective validation of a risk prediction model for severe sepsis in children with cancer and high-risk febrile neutropenia. Pediatr Infect Dis J. (2013) 32:1318–23. doi: 10.1097/01.inf.0000436128.49972.16
65. Schmidt N, Palma J, King A, Santolaya ME. [C reactive protein and procalcitonin levels for the diagnosis of invasive bacterial infections in allogenic hematopoietic stem cell transplantation recipients]. Rev Med Chil. (2007) 135:982–9. doi: 10.4067/s0034-98872007000800004
66. Secmeer G, Devrim I, Kara A, Ceyhan M, Cengiz B, Kutluk T, et al. Role of procalcitonin and CRP in differentiating a stable from a deteriorating clinical course in pediatric febrile neutropenia. J Pediatr Hematol Oncol. (2007) 29:107–11. doi: 10.1097/MPH.0b013e3180320b5b
67. Soker M, Colpan L, Ece A, Devecioglu C, Haspolat K. Serum levels of IL-1 beta, sIL-2R, IL-6, IL-8, and TNF-alpha in febrile children with cancer and neutropenia. Med Oncol. (2001) 18:51–7. doi: 10.1385/MO:18:1:51
68. Spasova MI, Terzieva DD, Tzvetkova TZ, Stoyanova AA, Mumdzhiev IN, Yanev IB, et al. Interleukin-6, interleukin-8, interleukin-10, and C-reactive protein in febrile neutropenia in children with malignant diseases. Folia Med. (2005) 47:46–52.
69. American College of Chest Physicians/Society of Critical Care Medicine Consensus Conference: definitions for sepsis and organ failure and guidelines for the use of innovative therapies in sepsis. Crit Care Med. (1992) 20:864–74. doi: 10.1097/00003246-199206000-00025
70. Urbonas V, Eidukaite A, Tamuliene I. Increased interleukin-10 levels correlate with bacteremia and sepsis in febrile neutropenia pediatric oncology patients. Cytokine. (2012) 57:313–5. doi: 10.1016/j.cyto.2011.11.012
71. Urbonas V, Eidukaite A, Tamuliene I. The predictive value of soluble biomarkers (CD14 subtype, interleukin-2 receptor, human leucocyte antigen-G) and procalcitonin in the detection of bacteremia and sepsis in pediatric oncology patients with chemotherapy-induced febrile neutropenia. Cytokine. (2013) 62:34–7. doi: 10.1016/j.cyto.2013.02.030
72. van der Galiën HT, Loeffen EAH, Miedema KGE, Tissing WJE. Predictive value of PCT and IL-6 for bacterial infection in children with cancer and febrile neutropenia. Support Care Cancer. (2018) 26:3819–26. doi: 10.1007/s00520-018-4249-3
73. Xu XJ, Tang YM, Liao C, Song H, Yang SL, Xu WQ, et al. Inflammatory cytokine measurement quickly discriminates gram-negative from gram-positive bacteremia in pediatric hematology/oncology patients with septic shock. Intensive Care Med. (2013) 39:319–26. doi: 10.1007/s00134-012-2752-4
74. Xu XJ, Luo ZB, Xia T, Song H, Yang SL, Xu WQ, et al. Comparison of interleukin-6, interleukin-10, procalcitonin and C-reactive protein in identifying high-risk febrile illness in pediatric cancer patients: a prospective observational study. Cytokine. (2019) 116:1–6. doi: 10.1016/j.cyto.2019.01.004
75. Mukaida N. Interleukin-8: an expanding universe beyond neutrophil chemotaxis and activation. Int J Hematol. (2000) 72:391–8.
76. Lindemann A, Tamm I, Tanodi K, Mertelsmann R. Interleukin-8 serum levels for early detection of infectious episodes in neutropenic patients. J Infect Dis. (1995) 172:610. doi: 10.1093/infdis/172.2.610
77. Nijsten MW, Olinga P, The The TH, de Vries EG, Koops HS, Groothuis GM. Procalcitonin behaves as a fast responding acute phase protein in vivo and in vitro. Crit Care Med. (2000) 28:458–61. doi: 10.1097/00003246-200002000-00028
78. Saraiva M, O'Garra A. The regulation of IL-10 production by immune cells. Nat Rev Immunol. (2010) 10:170–81. doi: 10.1038/nri2711
Keywords: pediatric, fever, biomarkers, immunocompromised, diagnostics
Citation: van der Velden FJS, Gennery AR and Emonts M (2022) Biomarkers for Diagnosing Febrile Illness in Immunocompromised Children: A Systematic Review of the Literature. Front. Pediatr. 10:828569. doi: 10.3389/fped.2022.828569
Received: 03 December 2021; Accepted: 25 January 2022;
Published: 10 March 2022.
Edited by:
Hans Van Rostenberghe, Universiti Sains Malaysia (USM), MalaysiaReviewed by:
Bob Phillips, University of York, United KingdomTanıl Kendirli, Ankara University, Turkey
Copyright © 2022 van der Velden, Gennery and Emonts. This is an open-access article distributed under the terms of the Creative Commons Attribution License (CC BY). The use, distribution or reproduction in other forums is permitted, provided the original author(s) and the copyright owner(s) are credited and that the original publication in this journal is cited, in accordance with accepted academic practice. No use, distribution or reproduction is permitted which does not comply with these terms.
*Correspondence: Marieke Emonts, marieke.emonts@ncl.ac.uk