- 1Henan Provincial Clinical Research Center for Pediatric Diseases, Children’s Hospital Affiliated to Zhengzhou University, Henan Children’s Hospital, Zhengzhou Children’s Hospital, Zhengzhou, China
- 2Department of Cardiothoracic Surgery, Children’s Hospital Affiliated to Zhengzhou University, Henan Children’s Hospital, Zhengzhou Children’s Hospital, Zhengzhou, China
Objective: This study aimed to examine the prevalence and the related risk factors of congenital heart disease (CHD) in children with different birth weights in China and the relationship between the subtypes of CHD and birth weight (BW).
Methods: This study conducted a cross-sectional survey on the data collected in the children’s congenital heart disease database (CHDD) established in China. This database contained data from one Grade A, Level III Children’s Public Hospital in Zhengzhou, Henan. The study included all the children and their parents in the database from 2014 to 2020 as the study subjects, and the missing data were processed by means of imputation. Diagnoses of CHD were coded using the International Classification of Diseases version 10 (ICD-10), and subtypes were classified by the codes Q20 to Q26. We reported the prevalence of CHD based on birth weight and gestational age and analyzed the related risk factors for children with CHD in different birth weight groups and factors for children of the same birth weight groups between the CHD groups and the non-CHD groups. The generalized linear model was used to assess the association between the subtypes of CHD and BW by establishing three adjusting models, and the data were stratified for further analysis by urban-rural and infant gender.
Results: A total of 42,814 children were identified as having CHD among 5,071,799 live children; the overall prevalence of CHD was 8.44 per 1,000 live births during 2014–2020; and the three subtypes with the highest prevalence of CHD were atrial septal defect (ASD) (2.75‰), ventricular septal defect (VSD) (2.57‰), and patent foramen ovale (PFO) (1.12‰). The prevalence of CHD was 18.87‰ in the group with BW <1,500 g, 12.84‰ in the group with BW 1,500–2,500 g, 8.24‰ in the group with BW 2,500–4,000 g, and 4.80‰ in the group with BW ≥4,000 g. The prevalence of CHD was 16.62‰ in the small for gestational age (SGA) group, 6.99‰ in the appropriate for gestational age (AGA) group, and 6.40‰ in the larger for gestational age (LGA) group. Parental factors such as drinking, smoking, viral infections, peri-pregnancy exposure to radioactive substances, low family monthly expenditure, and low Apgar scores at 1 and 5 min were related to the increased risk of CHD in the offspring. Parental supplementation of folic acid and exercise during the peri-pregnancy period could reduce the risk of CHD in the offspring. The results of Model 3 adjusting for confounding variables showed that infants with ASD had a birth weight 461 g lower (95% CI: −1,085, –128), infants with VSD had a birth weight 426 g lower (95% CI: –932, –120), infants with tetralogy of Fallot (TOF) had a birth weight 532 g lower (95% CI: –987, –168), and without classification, infants with CHD had a birth weight 973 g lower (95% CI: –1,502, –204).
Conclusion: In very low birth weight (VLBW) and low birth weight (LBW) infants, CHDs are more prevalent than in the general live-born population. Moreover, some peri-pregnancy factors of parents are closely related to the occurrence of CHD in offspring; different types of heart defects can lead to LBW. Therefore, if the fetus is found to have a heart defect during the prenatal examination, the mother should pay more attention to maintaining weight and ensuring that the fetus is within the normal weight range, thereby increasing the postpartum survival rate, reducing complications, and promoting children’s health.
Introduction
Congenital heart disease (CHD) is one of the most common congenital malformations. It refers to the abnormal anatomy caused by the formation of the heart and large blood vessels during the development of the embryo, or abnormal development, or channel that should be closed but failed to shut down after birth (1). It was reported that the global average prevalence of CHD at birth was 5–9 per 1,000 births; however, CHD frequencies were as high as 20–40/1,000 in very low birth weight (VLBW) babies weighing less than 1,500 g (2). The etiology of most CHD is not fully understood, but it is likely to involve complex interactions between environmental and genetic factors (3, 4). Similarly, the etiology for low birth weight (LBW) is also exceedingly broad and wide-ranging.
Some studies have shown that there is an association between CHD and LBW, which is a known comorbidity of CHD. A study based on the population of Sudan shows that infants with CHD are 2.6 times more likely to develop LBW than the general Sudanese population (5). CHDs that caused a decrement in birth weight in a descending order of severity are atrial septal defect (721 g/23%), patent ductus arteriosus (669 g/21%), ventricular septal defect (610 g/19%), pulmonary stenosis (548 g/13%), and tetralogy of Fallot (248 g/8%) (5). In addition, the prevalence of CHD in very premature/VLBW infants is higher than that of general live births, and it is independent of other risk factors and causes death and serious complications (6–8).
In China, the prevalence of CHD varies from 7 to 22.9 per 1,000 live births or perinatal infants (9–13), causing serious disease and economic burden to society and families. However, there are few reports on the prevalence of CHD in infants with birth weights less than 2,500 g and more than 4,000 g, and there is a lack of relevant research on the relationship between CHD and LBW. Therefore, it is necessary to conduct a detailed epidemiological investigation of CHD in children with different birth weights in China. This study aimed to determine the prevalence of CHD in very low birth weight infants, low birth weight infants, and giant infants by using the congenital heart disease database (CHDD) established in China containing detailed clinical data. Related risk factors and possible confounding factors were adjusted to further explore the relationship between CHD and birth weight.
Materials and Methods
Sources and Subjects
This study conducted a cross-sectional survey on the data collected from the children’s CHDD established in China. The database center was established by a university-affiliated children’s hospital in Henan Province, China (Grade A, Level III Children’s Public Hospital in Zhengzhou, Henan). The CHDD contained childbirth information, such as date of birth, birth weight, feeding method, congenital abnormalities, apgar score of newborn, current health status, and medical history data, and parental sociodemographic information, such as maternal perinatal information (parity, gestational week, and mode of delivery), parents’ socioeconomic factors (age, household registration, occupation, education level, and family monthly expenditure), parents’ lifestyle factors (folic acid supplementation, smoking, drinking, and exercise habits during pregnancy), parents’ exposure to virus, toxic, and harmful substances, and radioactive substances during the perinatal period. The CHDD was connected to the doctor’s medical record system, which could simultaneously obtain the child’s birth information, medical treatment, and record data. The sociodemographic information of the parents was obtained by the investigator through questionnaires and imported into the database in real-time. Data had been collected since 2014; all data were collected prospectively by the hospital data management center, which was equipped with 10 data management specialists for real-time data quality inspections; and then the supervisor completed the review before the data were locked every year.
The study included all the children and their parents in the database from 2014 to 2020 as the study subjects, excluding those with insufficient medical records, transfers after birth, and those who did not want to participate in the study. The study was reviewed and approved by the Ethics Committee of Zhengzhou University, and all respondents signed informed consent. The whole process and all methods of the study were performed in accordance with the relevant guidelines and regulations for the construction of clinical diagnosis and treatment and clinical research ethics review committees.
Newborn Birth Weight (g) and Diagnosis of Congenital Heart Disease
The birth weight of the newborn was collected by the birth certificate, or the weight was directly measured: after birth, the body was dried, the package was removed, the naked body was placed on the electronic scale, and the stable data were read. The accuracy of the scale was 1 g. When the newborn weighed <1,500 g, it was defined as very low birth weight (VLBW) infant; when the newborn weighed 1,500–2,500 g, it was defined as low birth weight (LBW) infant; when the newborn birth weighed 2,500–4,000 g, it was defined as normal weight infant (NBW); and when the newborn weighed ≥4,000 g, it was defined as macrosomia (14). Referring to the revised report on the birth weight of newborns of different gestational ages in China, the newborns were divided into three categories according to the relationship between birth weight and gestational age: infants were defined as small for gestational age (SGA) when birth weight was below the 10th percentile (P10) of the average gestational age; infants with birth weight above the 90th percentile (P90) of the same gestational age were defined as larger for gestational age (LGA); and between P10 and P90 of the same gestational age was appropriate for gestational age (AGA) (15).
In this study, those with positive records of prenatal echocardiography or with abnormal findings in CHD screening were offered neonatal ultrasound screening for CHD. The final diagnosis was based on neonatal echocardiographic findings from a scan and confirmed by the pediatrician. Diagnoses of CHD were coded using the International Classification of Diseases version 10 (ICD-10), and subtypes were classified by the codes Q20–Q26 (16). We defined 13 types of CHD, including atrial septal defect (ASD, Q21.102); ventricular septal defect (VSD, Q21.001); patent foramen ovale (PFO, Q21.103); patent ductus arteriosus (PDA, Q25.001); tetralogy of Fallot (TOF, Q21.300); atrioventricular septal defect (AVSD, Q21.200); pulmonary valve stenosis (PS, Q22.101); tricuspid regurgitation (TR, Q22.801); pulmonary hypertension (PHT, Q25.751); coarctation of the aorta (COA, Q25.300); mitral regurgitation (MR, Q23.300); aortic valve stenosis (AOS, Q23.001); transposition of great vessels (TGA, Q20.302), and other classification was not clear. In the case of multiple diagnoses in one patient, a prespecified hierarchical scheme founded on a consensus-based classification of defect severity was used, by means of which the diagnosis with the worst prognosis was established as the main diagnosis.
Statistical Analysis
Data were electronically registered. The prevalence of CHD in children with different birth weights was shown as the number of cases per 1,000 births, and any type of CHD was calculated as the total prevalence of CHD per 1,000 births. The continuous variables were statistically described by mean () and standard deviation (SD) and median (M) and quartile (Q1~Q3); categorical variables were described by composition ratio and rate. For missing values, we used the mean padding method for processing. The X2 test or, when appropriate, Fisher’s exact test was used to compare the prevalence of various types of congenital heart disease in different birth weight groups and characteristics of children with CHD at different birth weight groups and characteristics of children of the same birth weight groups between CHD and non-CHD groups. Chi-square trend analysis was used to track changes in the prevalence of CHD over time. The means were compared by one-way ANOVA; medians were compared with the Wilcoxon Mann–Whitney rank-sum test. The generalized linear model was used to analyze the relationship between BW and each subtype of CHD, taking BW as the dependent variable, whether each subtype of CHD was diseased as an independent variable, and adjusting possible confounding factors. The research model was as follows: g(μi) = β0 + β1x (whether each subtype of CHD was diseased) + β2x (adjusted variable) + εi. Three models were mainly established as follows: Model 1: no variable was adjusted; Model 2: adjusting fetal sex, gestational age, gravidity, parity, and apgar score at 1 min and 5 min; Model 3: on the basis of Model 2, continuing to adjust the parents’ age, residence (urban and rural), education level, occupation, folic acid supplementation, whether drink, smoke, viral infection, radioactive material exposure, doing physical exercise or not, and family monthly expenditure. And the data were stratified for further analysis by urban-rural and infant gender in the gender-specific model; the baby’s gender was no longer adjusted. These analyses were performed using SAS.9.4 and SPSS25.0; the drawing process was carried out using Microsoft Office 2010 Excel and GraphPad Prism software. P values < 0.05 (two-sided) were considered statistically significant, unless indicated otherwise.
Results
Patients’ Characteristics and Time Trends of Congenital Heart Disease With Different Birth Weights
From January 2014 to December 2020, a total of 42,814 children were identified as having CHD among 5,071,799 live children. The mean age of children was 6.4 ± 2.2 months. The overall prevalence of CHD was 8.44 per 1,000 live births. The total number of live births with VLBW was 112,560, the number of patients with CHD was 2,124, and the prevalence of CHD was 18.87 per 1,000 live births. The number of LBW newborns was 160,047, the number of patients with CHD was 2,055, and the prevalence of CHD was 12.84 per 1,000 live births. The number of NBW newborns was 4,541,238, the total number of patients with CHD was 37,405, and the prevalence of CHD was 8.24 per 1,000 live births. A total of 256,250 newborns with birth weight ≥4,000 g, the number of children with CHD was 1,230, and the prevalence of CHD was 4.80 per 1,000 live births. The total number of SGA was 786,129, the number of children with CHD was 13,062, and the prevalence of CHD was 16.62 per 1,000 live births. The total number of AGA was 3,950,931, the number of children with CHD was 27,608, and the prevalence of CHD was 6.99 per 1,000 live births. The total number of LGA was 331,875, the number of children with CHD was 2,142, and the prevalence of CHD was 6.40 per 1,000 live births (Table 1).
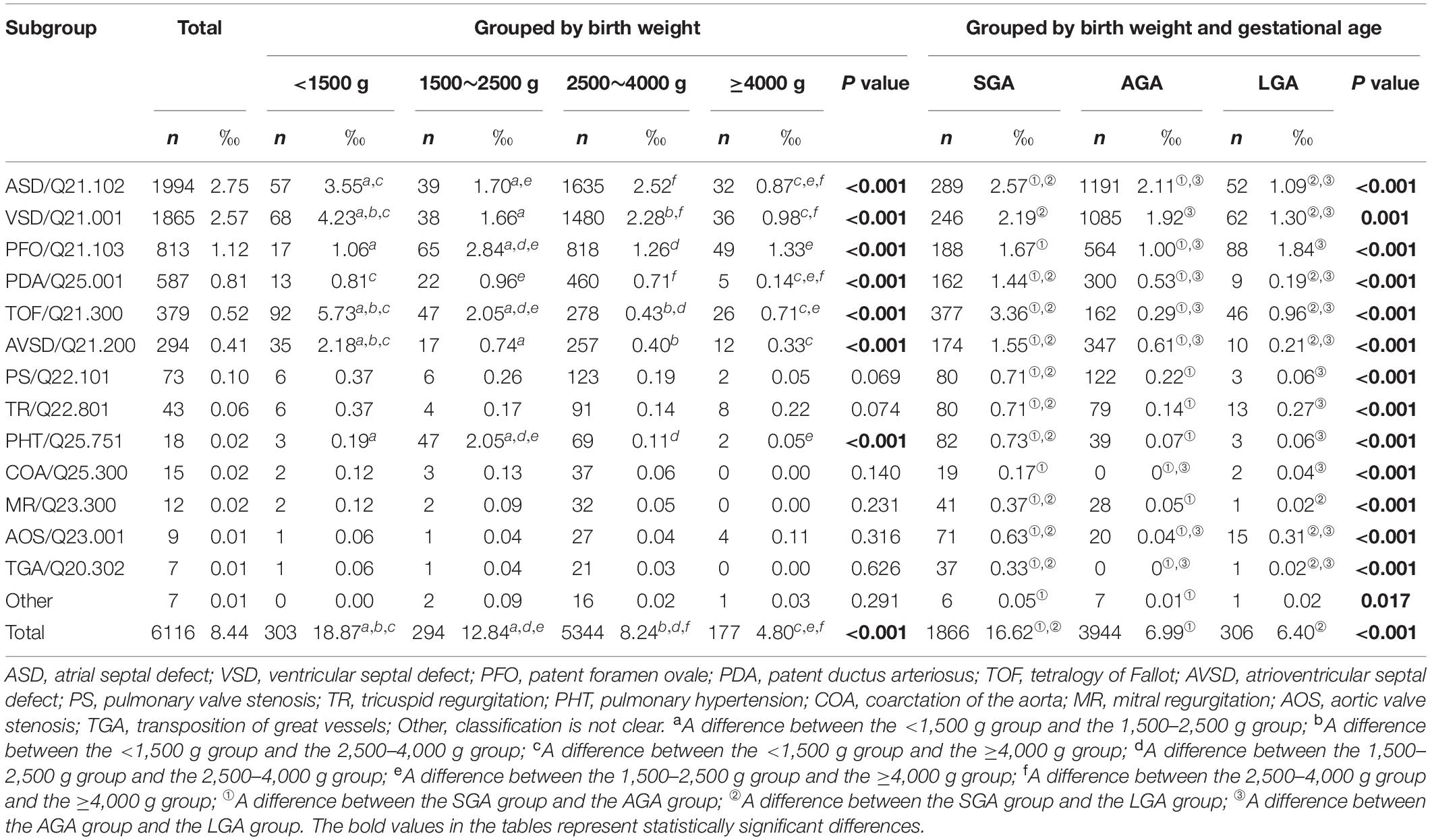
Table 1. Prevalence of different subtypes of congenital heart disease by birth weight (average prevalence from 2014 to 2020).
The prevalence of CHD in the group with birth weight <1,500 g increased from 12.81 per 1,000 births in 2014 to 20.65 per 1,000 births in 2020 (X2 trend = 125.62, P < 0.001). The prevalence of CHD in the group with birth weight 1,500–2,500 g increased from 5.22 per 1,000 births in 2014 to 18.33 per 1,000 births in 2020 (X2 trend = 98.37, P < 0.001). The prevalence of CHD in the group with birth weight 2,500–4,000 g increased from 3.92 per 1,000 births in 2014 to 10.91 per 1,000 births in 2020 (X2 trend = 85.23, P < 0.001). The prevalence of CHD in the group with birth weight ≥4,000 g increased from 1.82 per 1,000 births in 2014 to 6.41 per 1,000 births in 2020 (X2 trend = 80.19, P < 0.001). It was worth noting that the prevalence of CHD in each birth weight group had a peak in 2017 (Figure 1).
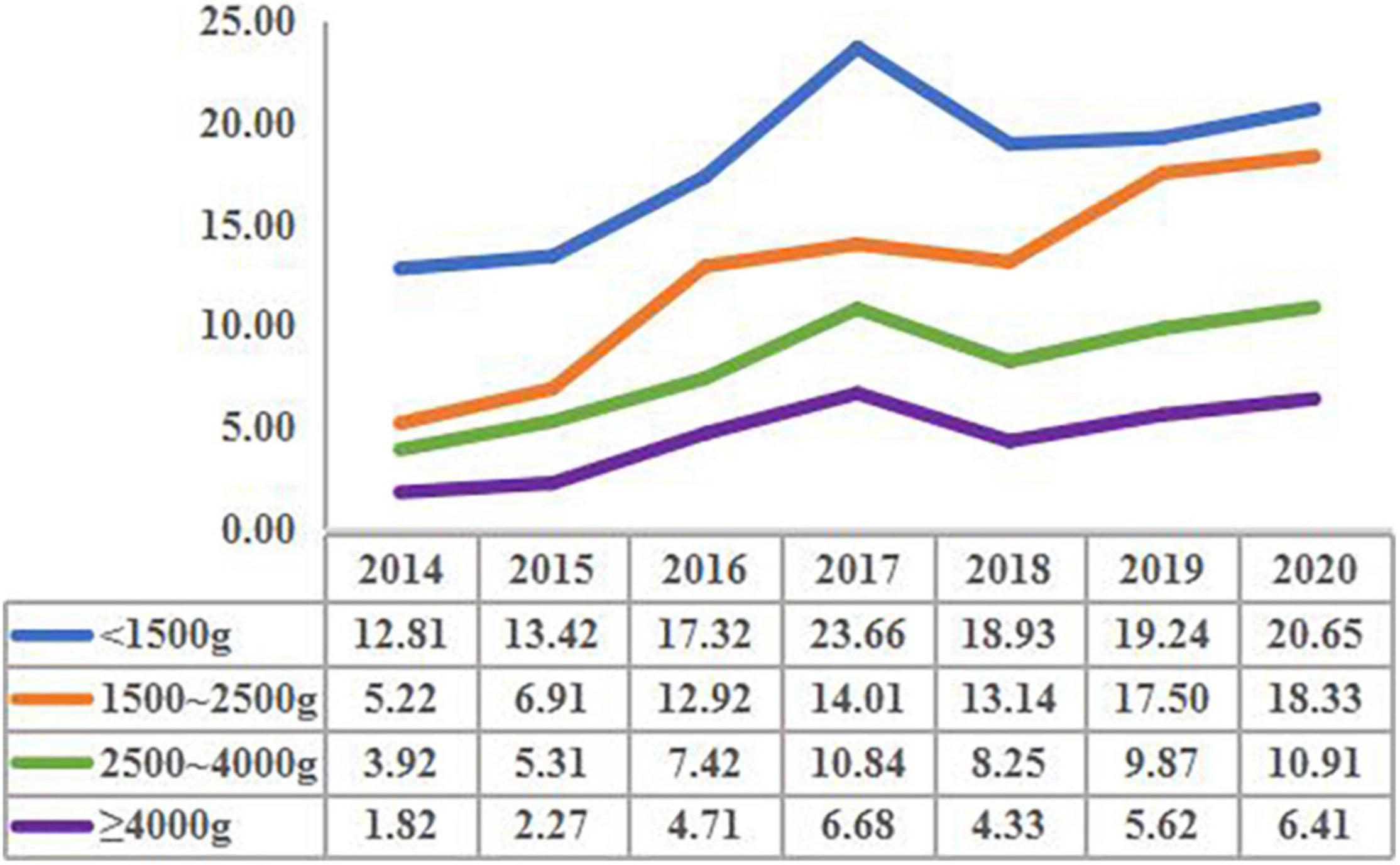
Figure 1. The prevalence of congenital heart disease in children with different birth weights in different years (per 1,000 births).
Prevalence of Different Subtypes of Congenital Heart Disease by Birth Weight
Overall, the three subtypes with the highest prevalence of CHD were ASD (2.75‰), VSD (2.57‰), and PFO (1.12‰). In the VLBW group, the three subtypes with the highest prevalence of CHD were TOF (5.73‰), VSD (4.23‰), and ASD (3.55‰). In the LBW group, the three subtypes with the highest prevalence of CHD were PFO (2.84‰), TOF (2.05‰), and PHT (2.05‰). In the NBW group, the three subtypes with the highest prevalence of CHD were ASD (2.52‰), VSD (2.28‰), and PFO (1.26‰). In the macrosomia group, the three subtypes with the highest prevalence of CHD were PFO (1.33‰), VSD (0.98‰), and ASD (0.87‰). Comparing the prevalence of various types of congenital heart disease within different birth weight groups, there were differences in the prevalence of ASD, VSD, PFO, PDA, TOF, AVSD, and PHT among different weight groups. In the group of SGA, the three subtypes with the highest prevalence of CHD were TOF (3.36‰), ASD (2.57‰), and VSD (2.19‰). In the group AGA, the three subtypes with the highest prevalence of CHD were ASD (2.11‰), VSD (1.92‰), and PFO (1.00‰). In the group of LGA, the three subtypes with the highest prevalence of CHD were PFO (1.84‰), VSD (1.30‰), and ASD (1.09‰). Comparing the prevalence of different types of congenital heart disease in different gestational age groups of birth weights, it was found that there were differences in the prevalence among SGA, AGA, and LGA groups (Table 1).
Risk Factors Related to Congenital Heart Disease
Table 2 shows that the influencing factors of children with CHD in different birth weight groups included maternal characteristics (gestational age, mother’s place of residence, educational levels, folic acid supplementation status, and doing physical exercise during pregnancy and 3 months before pregnancy), paternal characteristics (father’s place of residence, drinking status, smoking status, folic acid supplementation status before pregnancy, and doing physical exercise 3 months before pregnancy), family monthly expenditure, and child factors (apgar score at 1 and 5 min). Mothers tended to be full-term when they gave birth, and the higher the proportion of urban residents and taking folic acid supplements and doing exercise, the higher the education level; the higher the proportion of fathers living in cities and taking folic acid supplements and doing exercise, the lower the proportion of alcohol drinkers and smokers were lower; the higher the monthly family expenditure, the higher the apgar scores of 1 min and 5 min; and the more children with CHD tended to have a normal birth weight.
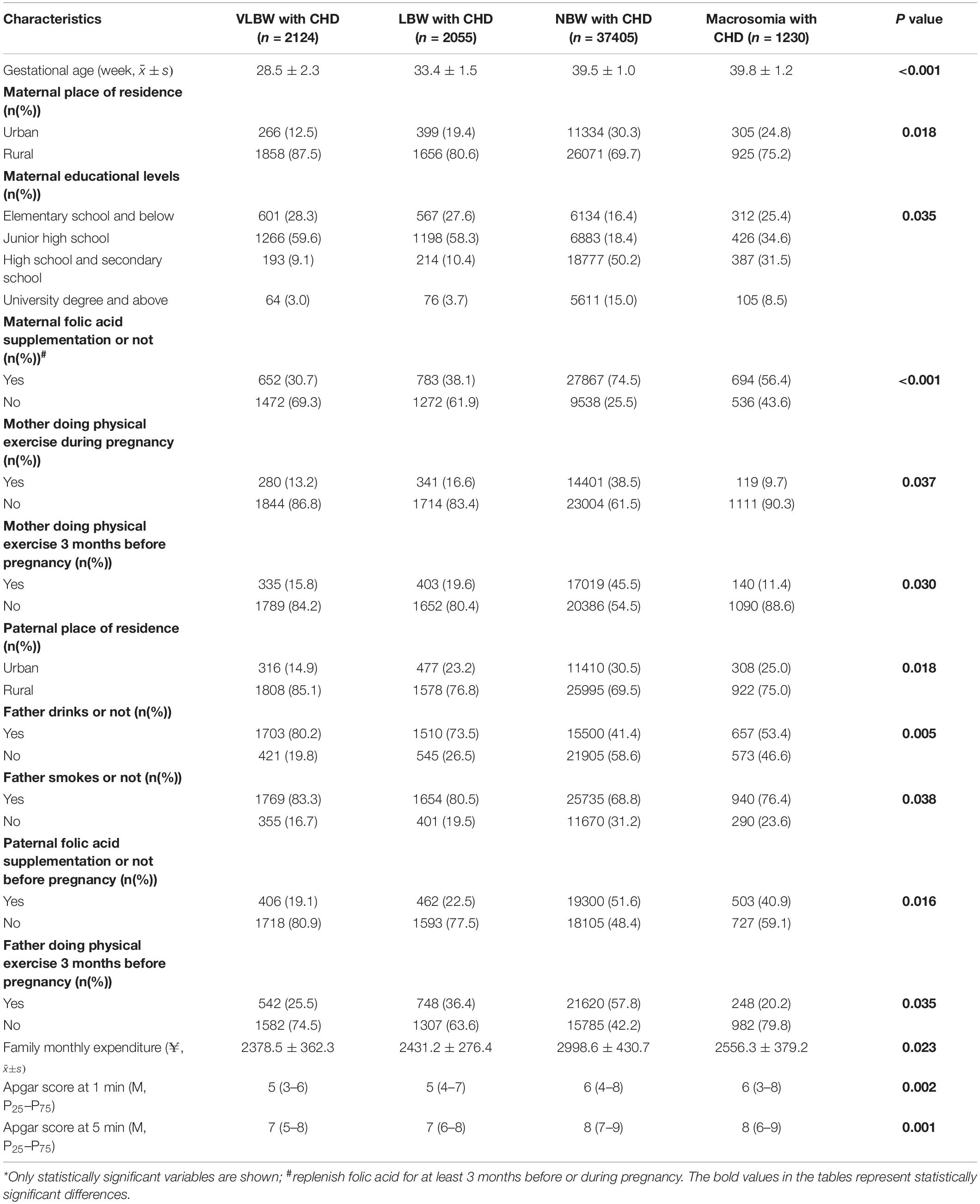
Table 2. Characteristics of children with congenital heart disease at different birth weight groups (N = 42,814).*
Further analysis showed that factors that differed between those with and without CHD in the VLBW group included parents’ characteristics (maternal and paternal viral infection, drinking status, radioactive material exposure, folic acid supplementation status, and maternal smokes status), family monthly expenditure, and child factors (apgar score at 1 min). The factors that differed between those with and without CHD in the LBW group included parents’ characteristics (maternal and paternal drinking status, smoking status, radioactive material exposure, folic acid supplementation status, and maternal viral infection), family monthly expenditure, and child factors (apgar score at 1 min and 5 min). The factors that differed between those with and without CHD in the NBW and macrosomia group were identical, including parents’ characteristics (maternal and paternal viral infection, drinking status, smoking status, radioactive material exposure, folic acid supplementation status, and maternal educational levels) and child factors (apgar score at 5 min) (Table 3).
The Relationship Between Different Subtypes of Congenital Heart Disease and Birth Weight
In the absence of stratified analysis, the ASD, VSD, TOF, and total CHD status had a statistically significant detrimental effect on birth weight in Model 1; after adjusting for some confounding factors (Model 2), similar results were still obtained; and after further adjusting for other confounding factors (Model 3), the results had not changed. On average, infants with ASD had a birth weight 461 g lower (95% CI: –1,085, –128), infants with VSD had a birth weight 426 g lower (95% CI: –932, –120), infants with TOF had a birth weight 532 g lower (95% CI: –987, –168), and without classification, infants with CHD had a birth weight 973 g lower (95% CI: –1,502, –204). After stratifying by gender of children, among boys, multiple models showed that VSD and TOF had significant adverse effects on birth weight. Infants with VSD were associated with a mean loss of birth weight of 467 g (95% CI: –1,052, –117), and infants with TOF were associated with a mean loss of birth weight of 1,056 g (95% CI: –1,424, –537) in Model 3. Among girls, multiple models showed that ASD, TOF, and total CHD had significant adverse effects on birth weight. Infants with ASD were associated with a mean loss of birth weight of 738 g (95% CI: –1,519, –295), infants with TOF were associated with a mean loss of birth weight of 544 g (95% CI: –906, –194), and infants with CHD were associated with a mean loss of birth weight of 812 g (95% CI: –1,359, –285) in Model 3. In addition, infants with PDA were associated with a mean loss of birth weight of 283 g (95% CI: –511, –55) only in Model 3 (Table 4).
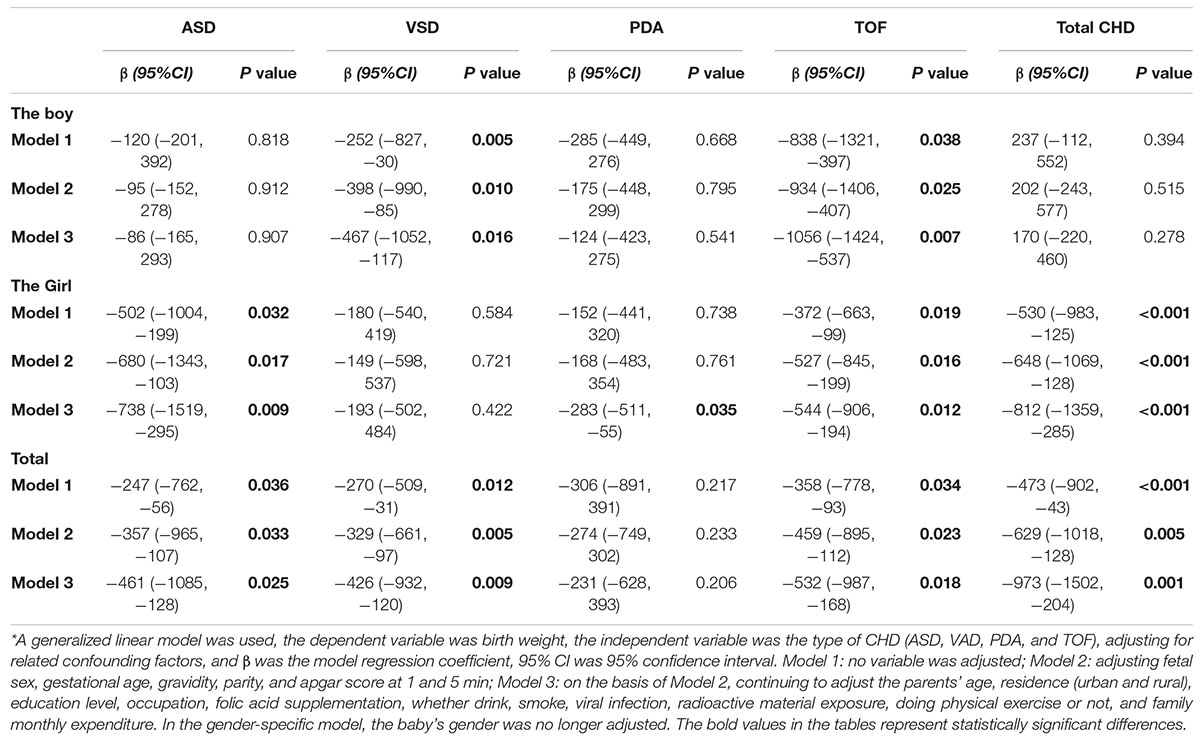
Table 4. Generalized linear model results of the relationship between different subtypes of congenital heart disease and birth weight in children.*
After stratified by residence, children with urban households showed that TOF and total CHD had a significant adverse effect on birth weight under multiple models. On average, infants with TOF had a birth weight 875 g lower (95% CI: –1,406, –293), and infants with CHD had a birth weight 649 g lower (95% CI: –1,125, –160) in Model 3. In addition, infants with ASD had a birth weight 507 g lower (95% CI: 1,033, –147), and infants with VSD had a birth weight 345 g lower (95% CI: –764, –96) only in Model 3. For rural children, the ASD, VSD, TOF, and total CHD status had a statistically significant detrimental effect on birth weight in multiple models. Infants with ASD would cause an average reduction in birth weight of 688 g (95% CI: –1,241, –133), infants with VSD would cause an average weight loss of 392 g (95% CI: –677, –123), infants with TOF would cause an average weight loss of 631 g (95% CI: –1,181, –193), and infants with CHD would cause an average weight loss of 879 g (95% CI: –1,367, –234) in Model 3 (Figure 2).
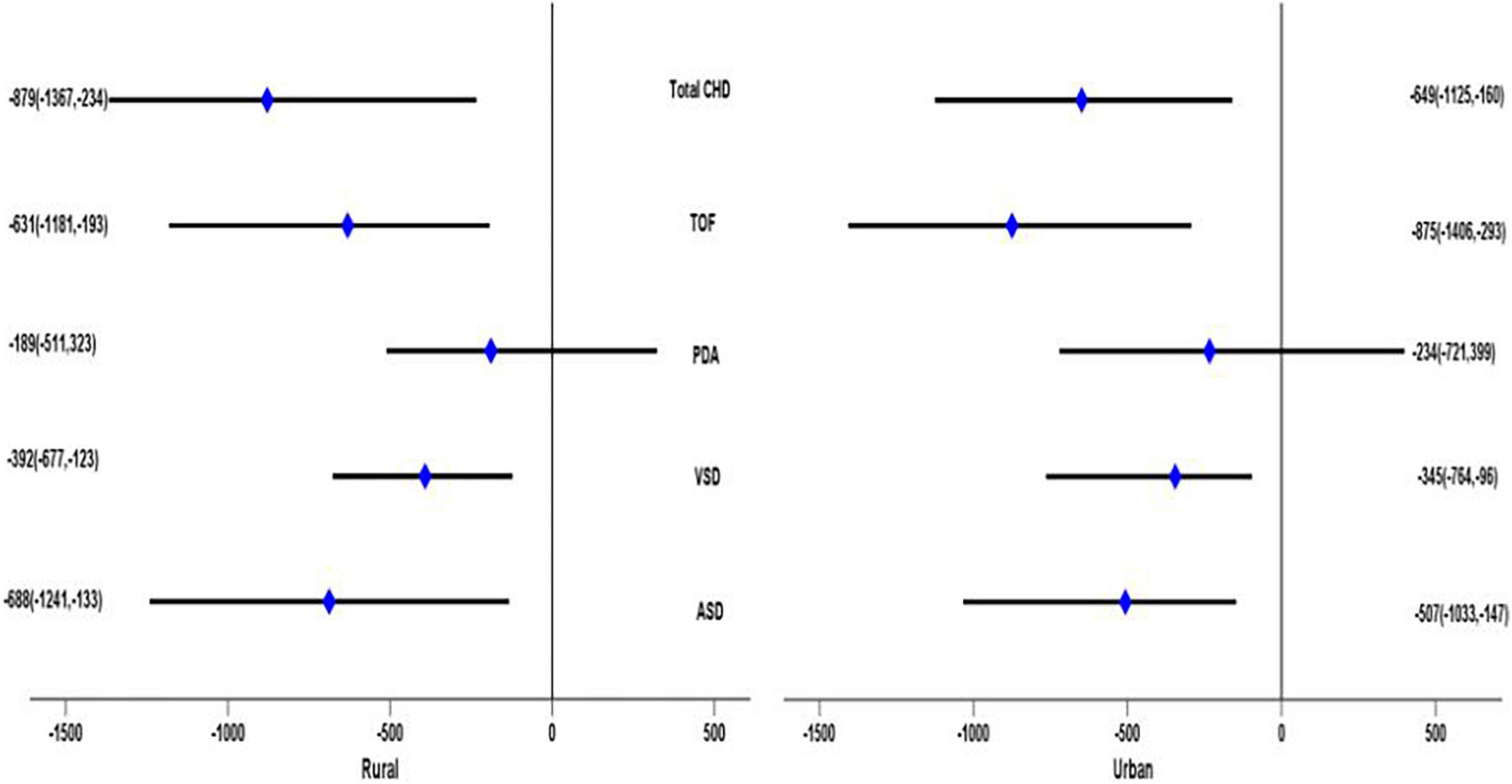
Figure 2. Adjusted β value and 95% CI of the relationship between different subtypes of congenital heart disease and birth weight in children of different places of residence (results of Model 3).
Discussion
At present, there have been some reports on the prevalence of CHD in LBW infants and premature infants, and some studies have revealed that there is a certain correlation between CHD and birth weight in the world. However, in China, there are few reports on the prevalence of CHD in VLBW and macrosomia, and there is also a lack of relevant research on the relationship between CHD and birth weight. This study with a large sample size described the epidemiology of CHD in Central Plains of China and could accurately reflect the occurrence of CHD, including the prevalence of some rare subtypes. And we explored the relationship between the subtypes of CHD and birth weight and provided theoretical and practical references for Chinese children to reduce the occurrence of CHD and abnormal birth weight.
We observed that the overall prevalence of CHD was 8.44 per 1,000 live births, and the three subtypes with the highest prevalence of CHD were ASD, VSD, and PFO, which was similar to most of the previous studies (8–10 per 1,000 births at global and local levels) (17–21). The prevalence of CHD in VLBW, LBW, and SGA infants was higher than in other birth weight groups, which was consistent with previous studies (2, 6–8), but the prevalence varied slightly in different regions; the reasons why heterogeneity of CHD prevalence might be differences in study populations, prenatal detection capability, and ascertainment of criteria. The reasons for the increased frequency of CHD in VLBW and SGA infants are still a matter of speculation (22), small septal defects may close spontaneously in utero, and therefore fewer may be apparent in term infants (7). CHD may impair intrauterine growth (23), which justifies the higher frequency of CHD in the VLBW and SGA groups documented by our study. Our research results had observed that, in each birth body reorganization, the prevalence of CHD also increased as the years grew; the increase in the prevalence of CHD in our study might reflect a true increase. The global prevalence of CHD increased from 4.6 per 1,000 live births in 1970–1974 to 9.4 per 1,000 live births in 2010–2017 (17). Increasing trends had also been observed for some specific forms of CHD, such as ASD in the United States and SV, ASD, and TOF in Europe (24, 25). Our results presented that the prevalence of CHD in each birth weight group had a peak in 2017. It might be related to China’s change in the birth policy that opened up the second child in 2016. The parents who gave birth to the second child were generally older, which might lead to an increase in the prevalence of CHD in their offspring.
Our results showed the influencing factors of children with CHD in different birth weight groups, among them, the factors that could be changed included folic acid supplementation before and during pregnancy, more exercise, drinking, and smoking. Folic acid is a water-soluble vitamin, which is indispensable in human metabolism as a coenzyme. Folic acid promotes the development of the fetal nervous system, and the lack of folic acid in the first trimester can lead to neural tube defects in infants (26). Due to the rapid growth and development of the fetus in the second and third trimesters, the demand for folic acid increases sharply; it will be extremely unfavorable to the growth and development of the fetus if the supplement of folic acid is ignored (27). Some studies had suggested that the nutritional status of folic acid during pregnancy was closely related to the growth and development of the fetal (27, 28). One meta-analysis also found that folic acid supplementation during pregnancy was associated with a reduced risk of low birth weight, although only at high doses (29). European and American scholars believed that if pregnant women received adequate nutrition during the development of the fetal nervous system, the heavier the baby’s weight, and the higher the baby’s IQ (28). In addition, good living habits such as physical exercise before and during pregnancy and not drinking or smoking can keep the parents in good health and cultivate healthy fertilized eggs, thereby avoiding the adverse outcome of low birth weight in the offspring (30).
Previous researches had proved that CHD was a multifactorial disease. Our study found that several maternal and paternal factors during pregnancy were associated with an increased risk of their children’s CHD in each birth weight group. These factors included pregnancy viral infection, smoking and drinking, exposure to radioactive substances, folic acid supplementation, exercise, family monthly expenditure, and apgar score. It has been suggested that the key period of heart embryonic development is the second to eighth weeks of pregnancy. Some studies indicated that maternal factors, including viral infection, smoking and drinking, exposure to radioactive substances, or treatment of such infections during the first trimester of pregnancy might precipitate CHD in the children (30, 31). There have been some studies on the relationship between folic acid supplementation during pregnancy and CHD in offspring (32, 33). Our study found that folic acid supplementation could reduce the risk of CHD. Folic acid participates in one-carbon unit metabolism and plays an important role in the process of nucleotide synthesis, amino acid conversion, and methylation (34). Cell proliferation and differentiation during early embryonic development are active, so folic acid metabolism disorders are closely related to early embryonic development abnormalities and birth defects (35). Epidemiological evidence shows that peri-pregnancy supplementation of folic acid or folic acid-containing multivitamins can effectively reduce the risk of CHD, and the key enzyme gene polymorphisms of its metabolic pathways are closely related to CHD (34–37). Folic acid metabolism disorders can lead to the occurrence of hyperhomocysteinemia, which is an independent risk factor for the onset of CHD (38); however, the mechanism of folate metabolism disorders in CHD is still unclear. Some studies have shown that it might cause CHD by affecting the formation and migration of cardiac neural crest cells, hindering DNA synthesis, and interfering with cell proliferation and apoptosis (37–39). Previous studies focused on the influence of maternal perinatal factors on CHD, and our research found that some of the father’s prepregnancy factors also had an impact on the occurrence of CHD. Deng K et al. had showed that fathers who smoked during peri-pregnancy increased the risk of CHD in their offspring, which might be related to the increased probability of sperm chromosome abnormalities and DNA mutations caused by toxic and harmful substances in tobacco, or it might also be related to the increased passive smoking of mothers caused by their fathers’ smoking (40). However, the association between paternal smoking and CHD in offspring needs to be further explored in a large population study. Besides, a father’s alcohol usage and radioactive material intake can increase the risk of CHD in the offspring, which may be largely due to the influence of alcohol and radioactive substances on sperm quality (41, 42).
Our findings clearly demonstrated that infants with CHDs were more likely to be of low birth weight than the general China children. And infants with ASD had a birth weight 461 g lower (95% CI: –1,085, –128), infants with VSD had a birth weight 426 g lower (95% CI: –932, –120), infants with TOF had a birth weight 532 g lower (95% CI: –987, –168), and without classification, infants with CHD had a birth weight 973 g lower (95% CI: –1,502, –204). It closely mirrored previous research done on this topic. Kramer et al. pointed out that the CHD group had significantly lower birth weights, and the decrease in birth weight was distinct only in children with TOF and ASD (43). Other studies showed that the mean birth weight of pure VSD and ASD case subjects was 2.52 and 2.41 kg, which was correspondingly 23% (deficit of 612 g) and 28.6% (deficit of 722 g) less than the control, and the comorbidity of congenital cardiovascular malformations was low birth weight, but the reasons for this association remained obscure (44). It is well established that ventricular and atrial septum are associated with a significant decrease in birth weight (5, 45). Different types of CHD may lead to LBW due to hemodynamics changes, and the specific mechanisms need to be further explored. The types of CHD that causes LBW are not the same in boys and girls, and the specific mechanisms are still unclear.
The advantage of this research is that the survey has been strictly scientifically designed, the data management personnel are of high quality, the data collection work is organized and planned, and the data collected is relatively accurate. This study is with large sample size, and the possible confounding factors are controlled as much as possible during the analysis to increase the reliability of the conclusion. However, there are still some limitations to our study. First of all, we mainly adopt the cross-sectional research method, which cannot verify the causal relationship. Second, we cannot fully monitor all factors that affect birth weight. Peri-pregnancy factors of parents are acquired through recall, which has a certain recall bias. Eventually, some potential confounding factors may not be controlled during the analysis process. This study is an ongoing project, and we will obtain more data on children with continuous improvement of our database system. In this study, we used a database with a large sample size to describe the prevalence of CHD in different birth weights of children in central China from 2014 to 2020, analyzed the parental peri-pregnancy risk factors related to CHD, and explored the relationship between different types of CHD and birth weights. It fills up the gaps in related research in China, and the research results have important reference significance for parents’ peri-pregnancy healthcare, the reduction of CHD, and the improvement of birth weight.
Conclusion
This study confirms that the prevalence of CHD in VLBW and LBW infants is higher than that of NBW infants in China. Moreover, some peri-pregnancy factors of the parents are closely related to the occurrence of CHD in the offspring; ASD, VSD, and TOF are the main types of CHD that cause LBW in infants. Therefore, prenatal care and other public health efforts such as folic acid supplementation and exercise by parents are expected to reduce the occurrence of CHD and improve the birth weight of the children. And if the fetus is found to have a heart defect during the prenatal examination, the mother should pay more attention to maintaining weight and ensuring that the fetus is within the normal weight range, so as to improve the survival rate after birth, reduce complications, and promote the children’s health.
Data Availability Statement
The original contributions presented in the study are included in the article/supplementary material, further inquiries can be directed to the corresponding author/s.
Ethics Statement
The studies involving human participants were reviewed and approved by Ethics Committee of Zhengzhou University. Written informed consent to participate in this study was provided by the participants’ legal guardian/next of kin. Written informed consent was obtained from the individual(s), and minor(s)’ legal guardian/next of kin, for the publication of any potentially identifiable images or data included in this article.
Author Contributions
HY contributed to conceptualization, methodology, software, statistical analysis of data, writing—original draft preparation, review and editing, investigation, and data curation. BZ contributed to management and coordination responsibility for the research activity planning and execution, data curation, resources, project administration, and validation. RF contributed to formal analysis, data collection and curation, supervision, investigation, and validation. PW contributed to oversight and leadership responsibility for the research activity planning and execution, including mentorship external to the core team, and investigation. YZha contributed to conducting a research and investigation process, specifically performing the data/evidence collection, and investigation and validation. YW contributed to data collection, scrub data, and maintain research data. YH contributed to data collection and data quality control. YZho contributed to visualization, investigation, data curation, supervision, project administration, resources, article revision, and project financial support. All authors contributed to the article and approved the submitted version.
Funding
The Joint Co-construction Project of Henan Provincial Medical Science and Technology Research Project (LHGJ20190958).
Conflict of Interest
The authors declare that the research was conducted in the absence of any commercial or financial relationships that could be construed as a potential conflict of interest.
Publisher’s Note
All claims expressed in this article are solely those of the authors and do not necessarily represent those of their affiliated organizations, or those of the publisher, the editors and the reviewers. Any product that may be evaluated in this article, or claim that may be made by its manufacturer, is not guaranteed or endorsed by the publisher.
Acknowledgments
Thanks to YZho, who helped me a lot through suggestions, comments, and criticism. His encouragement and firm support helped me through setbacks and frustrations. Without his urging, this dissertation would be impossible to complete. In addition, I am very grateful to all colleagues in the Thoracic and Cardiovascular Surgery and Clinical Epidemiology Research Center of the Children’s Hospital Affiliated to Zhengzhou University for their contributions to this study in various ways.
References
1. Kumar P, Clark ML. Kumar and Clark’s Clinical Medicine E-Book. Amsterdam: Elsevier Health Sciences (2012).
2. Song WS, Kim CY, Lee BS, Kim EAR, Kim KS, Jung E. Morbidity and mortality of very low birth weight infants with congenital heart disease. Korean Circulat J. (2020) 50:1113–23. doi: 10.4070/kcj.2020.0135
3. Jenkins KJ, Correa A, Feinstein JA, Botto L, Britt AE, Daniels SR, et al. Noninherited risk factors and congenital cardiovascular defects: current knowledge: a scientific statement from the American Heart Association Council on Cardiovascular Disease in the Young: endorsed by the American Academy of Pediatrics. Circulation. (2007) 115:2995–3014. doi: 10.1161/CIRCULATIONAHA.106.183216
4. Malik S, Cleves MA, Zhao W, Correa A, Hobbs CA. Association between congenital heart defects and small for gestational age. Pediatrics. (2007) 119:e976–82. doi: 10.1542/peds.2006-2742
5. Elshazali HOH, Elshazali OH, Elshazali H. The relationship between birth weight and congenital heart disease at Ahmed Gasim Cardiac Centre, Bahri, Sudan. Sudanese J Paediatr. (2017) 17:49–55. doi: 10.24911/SJP.2017.2.6
6. Archer JM, Yeager SB, Kenny MJ, Soll RF, Horbar JD. Distribution of and mortality from serious congenital heart disease in very low birth weight infants. Pediatrics. (2011) 127:293–9. doi: 10.1542/peds.2010-0418
7. Polito A, Piga S, Cogo PE, Corchia C, Carnielli V, Da Frè M, et al. Increased morbidity and mortality in very preterm/VLBW infants with congenital heart disease. Intens Care Med. (2013) 39:1104–12. doi: 10.1007/s00134-013-2887-y
8. Puia-Dumitrescu M, Sullivan LN, Tanaka D, Fisher K, Pittman R, Kumar KR, et al. Survival, morbidities, and developmental outcomes among low birth weight infants with congenital heart defects. Am J Perinatol. (2021) 38:1366–72. doi: 10.1055/s-0040-1712964
9. Pei L, Kang Y, Zhao Y, Yan H. Prevalence and risk factors of congenital heart defects among live births: a population-based cross-sectional survey in Shaanxi province, Northwestern China. BMC Pediatr. (2017) 17:18. doi: 10.1186/s12887-017-0784-1
10. Qu Y, Liu X, Zhuang J, Chen G, Mai J, Guo X, et al. Incidence of congenital heart disease: the 9-Year experience of the guangdong registry of congenital heart disease, China. PLoS One. (2016) 11:e0159257. doi: 10.1371/journal.pone.0159257
11. Xie D, Fang J, Liu Z, Wang H, Yang T, Sun Z, et al. Epidemiology and major subtypes of congenital heart defects in Hunan Province, China. Medicine. (2018) 97:e11770. doi: 10.1097/MD.0000000000011770
12. Zhao QM, Liu F, Wu L, Ma XJ, Niu C, Huang GY. Prevalence of congenital heart disease at live birth in China. J Pediatr. (2019) 204:53–8. doi: 10.1016/j.jpeds.2018.08.040
13. Sun PF, Ding GC, Zhang MY, He SN, Gao Y, Wang JH. Prevalence of congenital heart disease among infants from 2012 to 2014 in Langfang, China. Chin Med. J. (2017) 130:1069–73. doi: 10.4103/0366-6999.204923
14. Uchinuma H, Tsuchiya K, Sekine T, Horiuchi S, Kushima M, Otawa S, et al. Gestational body weight gain and risk of low birth weight or macrosomia in women of Japan: a nationwide cohort study. Int J Obesity. (2021) 45:2666–74. doi: 10.1038/s41366-021-00947-7
15. Ikem E, Halldorsson TI, Birgisdóttir BE, Rasmussen MA, Olsen SF, Maslova E. Dietary patterns and the risk of pregnancy-associated hypertension in the Danish National Birth Cohort: a prospective longitudinal study. BJOG Int J Obstetrics Gynaecol. (2019) 126:663–73. doi: 10.1111/1471-0528.15593
16. Bakker MK, Bergman JEH, Krikov S, Amar E, Cocchi G, Cragan J, et al. Prenatal diagnosis and prevalence of critical congenital heart defects: an international retrospective cohort study. BMJ Open. (2019) 9:e028139. doi: 10.1136/bmjopen-2018-028139
17. Liu Y, Chen S, Zühlke L, Black GC, Choy MK, Li N, et al. Global birth prevalence of congenital heart defects 1970-2017: updated systematic review and meta-analysis of 260 studies. Int. J Epidemiol. (2019) 48:455–63. doi: 10.1093/ije/dyz009
18. Chun H, Yue Y, Wang Y, Dawa Z, Zhen P, La Q, et al. High prevalence of congenital heart disease at high altitudes in Tibet. Eur J Prevent Cardiol. (2019) 26:756–9. doi: 10.1177/2047487318812502
19. Zhou Y, Mao X, Zhou H, Qin Z, Wang L, Cai Z, et al. Epidemiology of birth defects based on a birth defect surveillance system in Southern Jiangsu, China, 2014–2018. J Maternal-Fetal Neonatal Med. (2022) 35:745–51. doi: 10.1080/14767058.2020.1731459
20. Mat Bah MN, Sapian MH, Jamil MT, Abdullah N, Alias EY, Zahari N. The birth prevalence, severity, and temporal trends of congenital heart disease in the middle-income country: a population-based study. Congenital Heart Dis. (2018) 13:1012–27. doi: 10.1111/chd.12672
21. Lytzen R, Vejlstrup N, Bjerre J, Petersen OB, Leenskjold S, Dodd JK, et al. Live-born major congenital heart disease in Denmark: incidence, detection rate, and termination of pregnancy rate from 1996 to 2013. JAMA Cardiol. (2018) 3:829–37. doi: 10.1001/jamacardio.2018.2009
22. Godfrey M, Schimmel MS, Hammerman C, Farber B, Glaser J, Nir A. The incidence of congenital heart defects in very low birth weight and extremely low birth weight infants. Israel Med Assoc J IMAJ. (2010) 12:36–8.
23. Li Q, Dimopoulos K, Liu T, Xu Z, Liu Q, Li Y, et al. Peripartum outcomes in a large population of women with pulmonary arterial hypertension associated with congenital heart disease. Eur J Prevent Cardiol. (2019) 26:1067–76. doi: 10.1177/2047487318821246
24. Mai CT, Isenburg JL, Canfield MA, Meyer RE, Correa A, Alverson CJ, et al. National population-based estimates for major birth defects, 2010-2014. Birth Defects Res. (2019) 111:1420–35. doi: 10.1002/bdr2.1589
25. Morris JK, Springett AL, Greenlees R, Loane M, Addor MC, Arriola L, et al. Trends in congenital anomalies in Europe from 1980 to 2012. PLoS One. (2018) 13:e0194986. doi: 10.1371/journal.pone.0194986
26. Li B, Zhang X, Peng X, Zhang S, Wang X, Zhu C. Folic acid and risk of preterm birth: a meta-analysis. Front Neurosci. (2019) 13:1284. doi: 10.3389/fnins.2019.01284
27. Souza R, Miranda C, Ferreira LB, Dos Santos LC. The influence of nutrients intake during pregnancy on Baby’s birth weight: a systematic review. J Tropical Pediatr. (2021) 67:fmab034. doi: 10.1093/tropej/fmab034
28. Petry CJ, Ong KK, Hughes IA, Dunger DB. Folic acid supplementation during pregnancy and associations with offspring size at birth and adiposity: a cohort study. BMC Res Notes. (2021) 14:160. doi: 10.1186/s13104-021-05575-y
29. Charles DH, Ness AR, Campbell D, Smith GD, Whitley E, Hall MH. Folic acid supplements in pregnancy and birth outcome: re-analysis of a large randomised controlled trial and update of Cochrane review. Paediatric Perinatal Epidemiol. (2005) 19:112–24. doi: 10.1111/j.1365-3016.2005.00633.x
30. Mohammed S, Bonsing I, Yakubu I, Wondong WP. Maternal obstetric and socio-demographic determinants of low birth weight: a retrospective cross-sectional study in Ghana. Reprod Health. (2019) 16:70. doi: 10.1186/s12978-019-0742-5
31. Liu X, Liu G, Wang P, Huang Y, Liu E, Li D, et al. Prevalence of congenital heart disease and its related risk indicators among 90,796 Chinese infants aged less than 6 months in Tianjin. Int J Epidemiol. (2015) 44:884–93. doi: 10.1093/ije/dyv107
32. Sun R, Liu M, Lu L, Zheng Y, Zhang P. Congenital Heart Disease: causes, diagnosis, symptoms, and treatments. Cell Biochem Biophys. (2015) 72:857–60. doi: 10.1007/s12013-015-0551-6
33. Oster ME, Kim CH, Kusano AS, Cragan JD, Dressler P, Hales AR, et al. A population-based study of the association of prenatal diagnosis with survival rate for infants with congenital heart defects. Am J Cardiol. (2014) 113:1036–40. doi: 10.1016/j.amjcard.2013.11.066
34. Selhub J, Rosenberg IH. Excessive folic acid intake and relation to adverse health outcome. Biochimie. (2016) 126:71–8. doi: 10.1016/j.biochi.2016.04.010
35. Czeizel AE, Dudás I, Vereczkey A, Bánhidy F. Folate deficiency and folic acid supplementation: the prevention of neural-tube defects and congenital heart defects. Nutrients. (2013) 5:4760–75. doi: 10.3390/nu5114760
36. Qu Y, Lin S, Zhuang J, Bloom MS, Smith M, Nie Z, et al. First-trimester maternal folic acid supplementation reduced risks of severe and most congenital heart diseases in offspring: a large case-control study. J Am Heart Assoc. (2020) 9:e015652. doi: 10.1161/JAHA.119.015652
37. Viswanathan M, Treiman KA, Kish-Doto J, Middleton JC, Coker-Schwimmer EJ, Nicholson WK. Folic acid supplementation for the prevention of neural tube defects: an updated evidence report and systematic review for the US preventive services task force. JAMA. (2017) 317:190–203. doi: 10.1001/jama.2016.19193
38. Peng Y, Ou BQ, Li HH, Zhou Z, Mo JL, Huang J, et al. Synergistic effect of atorvastatin and folic acid on cardiac function and ventricular remodeling in chronic heart failure patients with Hyperhomocysteinemia. Med Sci Monit Int Med J Exp Clin Res. (2018) 24:3744–51. doi: 10.12659/MSM.906893
39. Wilson RD, Wilson RD, Audibert F, Brock J-A, Carroll J, Cartier L, et al. Pre-conception folic acid and multivitamin supplementation for the primary and secondary prevention of neural tube defects and other folic acid-sensitive congenital anomalies. J Obstetrics Gynaecol Canada. (2015) 37:534–49. doi: 10.1016/s1701-2163(15)30230-9
40. Yu ZB, Han SP, Guo XR. [A Meta-analysis on the risk factors of perinatal congenital heart disease in Chinese people]. Zhonghua Liu Xing Bing Xue Za Zhi Zhonghua Liuxingbingxue Zazhi. (2008) 29:1137–40.
41. Deng K, Liu Z, Lin Y, Mu D, Chen X, Li J, et al. Periconceptional paternal smoking and the risk of congenital heart defects: a case-control study. Birth Defects Res Part A Clin Mol Teratol. (2013) 97:210–6. doi: 10.1002/bdra.23128
42. Taylor K, Elhakeem A, Thorbjørnsrud Nader JL, Yang TC, Isaevska E, Richiardi L, et al. Effect of maternal prepregnancy/Early-pregnancy body mass index and pregnancy smoking and alcohol on congenital heart diseases: a parental negative control study. J Am Heart Assoc. (2021) 10:e020051. doi: 10.1161/JAHA.120.020051
43. Kramer HH, Trampisch HJ, Rammos S, Giese A. Birth weight of children with congenital heart disease. Eur J Pediatr. (1990) 149:752–7. doi: 10.1007/BF01957272
44. Petrossian RA, Kuehl KS, Loffredo CA. Relationship of birth weight with congenital cardiovascular malformations in a population-based study. Cardiol Young. (2015) 25:1086–92. doi: 10.1017/S1047951114001644
Keywords: congenital heart disease, birth weight, prevalence, special disease database, children
Citation: Yan H, Zhai B, Feng R, Wang P, Zhang Y, Wang Y, Hou Y and Zhou Y (2022) Prevalence of Congenital Heart Disease in Chinese Children With Different Birth Weights and Its Relationship to the Neonatal Birth Weight. Front. Pediatr. 10:828300. doi: 10.3389/fped.2022.828300
Received: 03 December 2021; Accepted: 06 April 2022;
Published: 19 May 2022.
Edited by:
Gerhard-Paul Diller, University Hospital Münster, GermanyReviewed by:
Pengfei Qu, Xi’an Jiaotong University, ChinaAshish Garg, Washington State University Tri-Cities, United States
Copyright © 2022 Yan, Zhai, Feng, Wang, Zhang, Wang, Hou and Zhou. This is an open-access article distributed under the terms of the Creative Commons Attribution License (CC BY). The use, distribution or reproduction in other forums is permitted, provided the original author(s) and the copyright owner(s) are credited and that the original publication in this journal is cited, in accordance with accepted academic practice. No use, distribution or reproduction is permitted which does not comply with these terms.
*Correspondence: Yang Zhou, enlhbmdjcHVAMTYzLmNvbQ==