- 1Department of Pediatrics, University of Tennessee Health Science Center, Memphis, TN, United States
- 2Department of Obstetrics and Gynecology, University of Tennessee Health Science Center, Memphis, TN, United States
Bronchopulmonary Dysplasia (BPD) is a multifactorial disease affecting over 35% of extremely preterm infants born each year. Despite the advances made in understanding the pathogenesis of this disease over the last five decades, BPD remains one of the major causes of morbidity and mortality in this population, and the incidence of the disease increases with decreasing gestational age. As inflammation is one of the key drivers in the pathogenesis, it has been targeted by majority of pharmacological and non-pharmacological methods to prevent BPD. Most extremely premature infants receive a myriad of medications during their stay in the neonatal intensive care unit in an effort to prevent or manage BPD, with corticosteroids, caffeine, and diuretics being the most commonly used medications. However, there is no consensus regarding their use and benefits in this population. This review summarizes the available literature regarding these medications and aims to provide neonatologists and neonatal providers with evidence-based recommendations.
Introduction
Northway et al. (1) were the first to describe bronchopulmonary dysplasia (BPD). They coined the term “BPD” to define oxygen and mechanical ventilation (MV) induced injury and the superimposed healing occurring in the lungs of infants with respiratory distress syndrome (RDS) (1). The successful introduction of exogenous surfactant therapy in 1980s resulted in a change from “old BPD” consisting predominantly of airway injury, inflammation and parenchymal fibrosis to “new BPD” characterized by decreased fibrosis, lesser airway injury and more uniform inflation with alveolar simplification. Pulmonary microvascular development is also reduced in some patients with “new BPD” (2, 3). Therefore, BPD was redefined as lung injury in preterm infants requiring ≥28 days of supplemental oxygen (National Institute of Child Health and Human Development (NICHD)/National Heart, Lung and Blood Institute (NHLBI) workshop, 2000). Depending on the amount of supplemental oxygen required at 36 weeks' postmenstrual age (PMA) [for infants < 32 weeks' gestational age (GA)] or at >28 days (for infants ≥ 32 weeks' GA), BPD was classified as mild, moderate, or severe (2).
The advances in treatment methods and the more frequent use of low-flow nasal cannulas with 100% oxygen warranted a newer definition of BPD not dependent solely on supplemental oxygen requirement. To address this, a newer definition of BPD was formulated at the workshop held by NICHD in 2016. This workshop defined BPD as ‘persistent parenchymal lung disease in infants <32 weeks' GA with confirmation of parenchymal disease on chest radiograph and respiratory support requirement for >2 consecutive days at 36 weeks' PMA to maintain transcutaneous oxygen saturation between 90-95%. Furthermore, BPD severity was classified into grades I to III based on the amount of respiratory support, and a class III(A) was defined to include the infants < 36 weeks' PMA, who died because of respiratory failure associated with parenchymal lung disease (4).
In 2019, Jensen et al. concluded that the severity of BPD defined based on the mode of oxygen supplementation at 36 weeks' PMA was the best predictor of morbidity and mortality. Based on a study including 2677 infants (<32 weeks' GA) from 18 centers, the optimal BPD classification at 36 weeks' PMA was defined as: no BPD = no oxygen support, grade 1 = low-flow nasal cannula (≤ 2 L/min), grade 2 = high-flow nasal cannula (>2L/min) or non-invasive positive pressure ventilation (NIPPV), and grade 3 = invasive mechanical ventilation (5).
BPD is a multifactorial disease. Each year, over 10,000–18,000 infants <28 weeks' GA (~ 35%) born in the United States (US) are diagnosed with BPD (6, 7). The risk of BPD increases with decreasing GA and birth weight (5). With more premature infants surviving, the rate of BPD has increased (8). Numerous non-pharmacological and pharmacological interventions have been investigated to prevent and manage BPD and several reviews have been published reviewing the drugs used in BPD. However, there remains no clear consensus on the use of various medications for BPD (9–19). In this review, we summarize the available evidence for the different pharmacological agents currently in use to prevent and manage BPD and aim to provide neonatologists and neonatal practitioners with evidence-based recommendations. Table 1 provides a summary of the drugs commonly used in BPD.
Medications Used in BPD
Antenatal Corticosteroids
In 1972, Liggins et al. first showed that antenatal corticosteroids (ACS) promoted lung maturation and prevented RDS in preterm infants (20). About two decades later, the National Institute of Health (NIH) consensus panel recommended ACS use for all impending preterm births 24–34 weeks of gestation (21). ACS promote alveolar epithelial cell differentiation into type II pneumocytes and increase surfactant protein (SP)-A and SP-B expression and the overall surfactant production. They also improve pulmonary blood flow via endothelial nitric oxide synthase activation and increase epithelial Na channels, thus improving respiratory function (22–25).
The most common regimens for ACS administration used throughout the world include betamethasone and dexamethasone. The American College of Obstetrics and Gynecology (ACOG) recommends two doses of intramuscular (IM) betamethasone or four doses of IM dexamethasone for all pregnant women between 24 0/7 to 33 6/7 weeks of gestation and at risk of delivery within seven days (26). For pregnant women at risk of delivery between 23 0/7 to 23 6/7 weeks of gestation, ACS should be administered if resuscitation is desired. ACOG also recommends ACS for women between 34 0/7 to 36 6/7 weeks of gestation with a risk of preterm delivery within seven days who have not previously received ACS (26).
A Cochrane review comparing various ACS regimens found that dexamethasone was associated with lower rates of intraventricular hemorrhage (IVH) compared to betamethasone. Neonatal death, RDS, or neurodevelopmental outcome at 18 months were similar with the two drugs (27). Despite a clear improvement in mortality and RDS, the 2020 Cochrane review comparing ACS with placebo noted no significant difference in the incidence of BPD between the two groups (28). Another meta-analysis including infants <25 weeks' GA from nine observational studies showed that the incidence of BPD was higher with ACS exposure (Odds Ratio (OR):1.32; 95% confidence interval (CI): 1.04–1.67); however, this is more likely due to the significant decline in mortality (OR: 0.48; 95% CI: 0.42–0.55) as well as the combined outcome of BPD and death (OR: 0.58; 95% CI: 0.42–0.79) with ACS use. (29).
Given the improvement in the incidence of RDS and mortality, in the absence of contraindications, we recommend routine administration of ACS for all pregnant women based on ACOG criteria.
Surfactant
Fujiwara et al. were the first to describe successful exogenous surfactant replacement therapy in preterm infants with RDS in 1980 (30). Several multicenter studies on surfactant use followed, leading to the FDA approval of surfactant in premature infants in 1990. Since then, surfactant is routinely used in neonates with RDS. Surfactants available for clinical use include synthetic and animal-derived surfactants, with animal-derived surfactants being the most commonly used. Surfactant improves lung compliance and increases the functional capacity by decreasing alveolar surface tension and preventing alveolar collapse (31). In addition to reducing the severity of RDS, a Cochrane review in 1998 showed that intratracheal administration of surfactant improved mortality, air-leak syndromes, and chronic lung disease (CLD) (32). However, there have been marked improvements in ventilation strategies since this review, including the introduction of non-invasive positive pressure ventilation (NIPPV), and the benefit of surfactant in BPD prevention was not shown in later studies.
The SUPPORT trial published in 2010 was a multicenter randomized controlled trial (RCT). It consisted of 1316 infants of 24 0/7 to 27 6/7 weeks' GA, randomized to receive continuous positive airway pressure (CPAP) or intubation and surfactant administration within the 1st hour of life. The incidence of BPD at 36 weeks' PMA was similar between the two groups (33).
The role of timing and method of surfactant administration in preventing BPD has also been studied. In a Cochrane meta-analysis, there was a decreased incidence of BPD and air leak syndromes in infants receiving early surfactant followed by early extubation to CPAP compared to those receiving selective surfactant and continued MV (34). Another Cochrane review consisting of six RCTs showed that in intubated infants receiving selective surfactant, the risk of CLD, neonatal mortality, and that of CLD or death at 36 weeks' PMA was lower when surfactant was administered early (within two hours of life) compared to late administration (35).
MV itself is associated with lung injury, and it is known that avoiding MV decreases the incidence of BPD (36). In 1994, Verder et al. introduced the INSURE method to reduce the duration of MV in neonates stable on non-invasive ventilation (37). Over a decade later, to avoid MV, Kribs et al. introduced the “less invasive surfactant administration” (LISA) or the “minimally invasive surfactant therapy” (MIST), where surfactant is delivered intratracheally to an infant on CPAP via a thin catheter (38).
In a meta-analysis by Isayama et al., no statistically significant differences were found in the incidence of CLD, death, or the combined outcome of CLD or death between infants receiving nasal CPAP and those receiving early surfactant using the INSURE (Intubate-SURfactant-Extubate) method (39).
Individual RCTs studying the incidence of BPD have found LISA to be either superior or equivalent to conventional surfactant administration (40–43). A meta-analysis found LISA to be associated with lower BPD incidence at 36 weeks' PMA and the incidence of the composite outcome of death or BPD at 36 weeks' PMA compared to conventional surfactant administration via intubation (44). Similar results were noted in the recent Cochrane review by Abdel-Latif et al. (BPD-relative risk (RR): 0.57; 95% CI: 0.45–0.74; death-RR: 0.63; 95% CI: 0.47–0.84; combined BPD or death-RR: 0.59, 95% CI: 0.48–0.73) (45).
When compared to the various non-invasive ventilation strategies, the meta-analysis by Isayama et al. found LISA to be associated with the lowest likelihood of the composite outcome of death or BPD at 36 weeks' PMA (46). However, the recently published OPTIMIST-A trial, a multicenter masked RCT that compared surfactant treatment using a thin catheter vs. sham treatment in preterm infants 25 to 28 6/7 weeks of gestation on CPAP, found no difference in the incidence of death or the composite outcome of death or BPD at 36 weeks' PMA between the two groups. The incidence of BPD in survivors was lower in the MIST group (47).
Another method of surfactant administration is via laryngeal mask airway (LMA). However, an RCT consisting of 103 infants 28 0/7 to 35 6/7 weeks of gestation requiring 0.3–0.4 fractional inspired oxygen (fiO2) showed that BPD incidence at 36 weeks' PMA was similar in the groups receiving either CPAP or surfactant via LMA (48). Aerosolized surfactant has been used to treat RDS; however, no RCTs have studied its utility in preventing BPD.
Some researchers have studied intra-tracheal administration of combined corticosteroid and surfactant for BPD prevention. In a meta-analysis, a lower risk of BPD was noted when corticosteroids (inhaled or intratracheal) and surfactant were administered concurrently (RR 0.58, 95% CI 0.41 to 0.82) compared to surfactant alone (49). The “Budesonide in Babies” (BiB) trial (NCT04545866) by the NICHD study group is a large RCT that will study the efficacy of intratracheal surfactant administered in combination with budesonide compared to surfactant alone for the prevention of BPD and death and is currently enrolling patients (50). Table 2 lists the ongoing RCTs evaluating various drugs for the management of BPD.
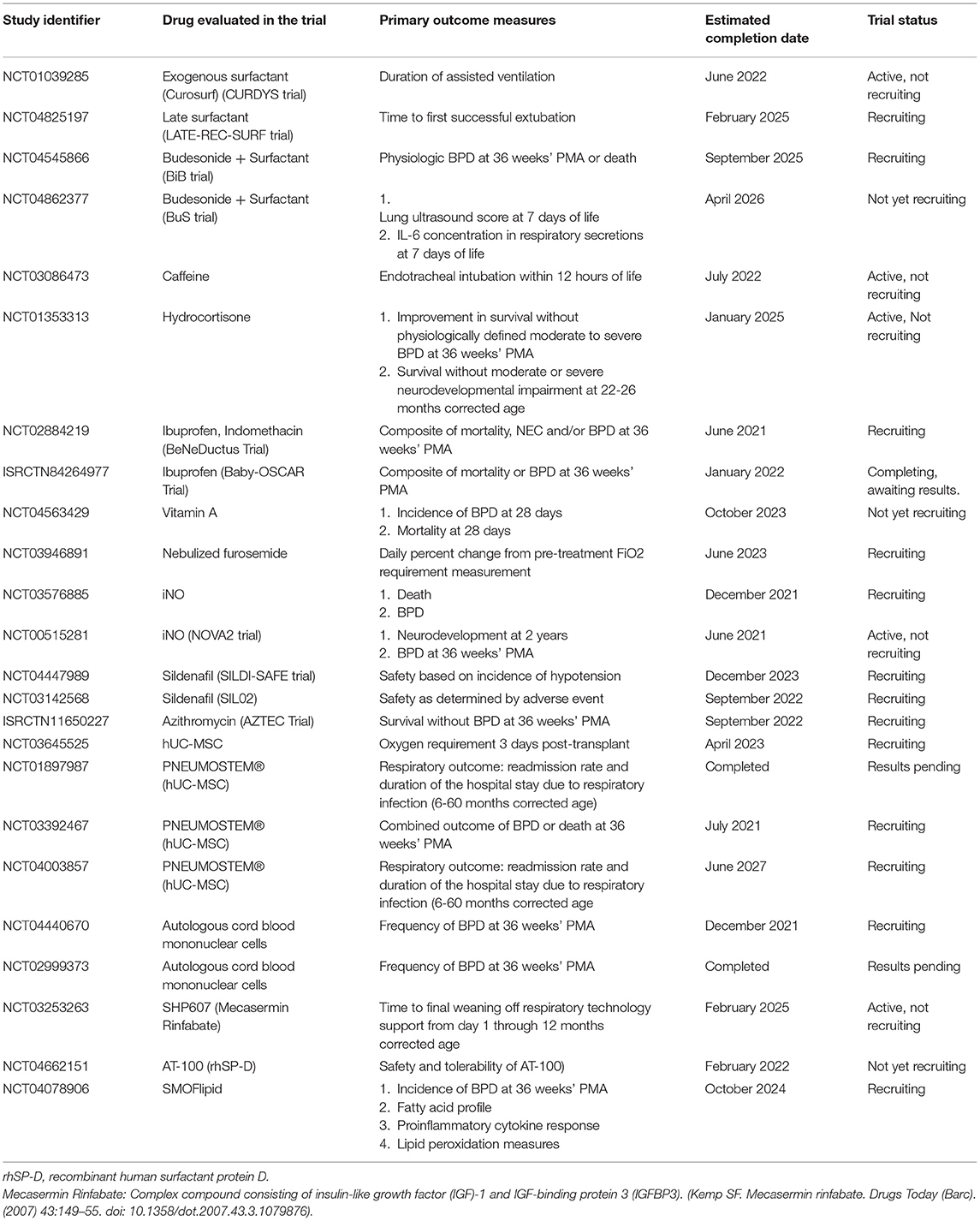
Table 2. Ongoing randomized controlled clinical trials evaluating pharmacotherapy for BPD prevention/treatment listed on ClinicalTrials.gov/ISRCTN registry.
A double-blind RCT consisting of 118 infants <33 weeks' GA requiring MV on day 14 and a fiO2 > 0.3 failed to demonstrate any benefit of late surfactant over placebo in lowering the incidence of severe BPD/death at 36 weeks PMA. However, infants who received surfactant had lower respiratory morbidity before one year of age (51). The TOLSURF study, which included infants ≤ 28 weeks' GA on MV at 7-14 days and inhaled nitric oxide (iNO), yielded similar results (52, 53).
BPD incidence was similar in infants treated with protein-free synthetic surfactant and animal-derived surfactant in a Cochrane review (RR 1.0, 95% CI 0.93–1.07) (54).
For neonates with RDS, surfactant administration is the standard of care and should not be withheld. In our unit, we administer prophylactic surfactant in the delivery room for all infants <27 weeks' GA. However, this practice cannot be routinely recommended, and individual unit practices should be followed. Efforts should be made to wean the infant off MV as soon as possible after surfactant use. In spontaneously breathing infants, LISA or INSURE methods for surfactant administration are preferred to avoid MV-related lung injury. While LISA appears to be a promising new technique, larger RCTs are required to determine its role in preventing BPD. We do not recommend routine aerosolized surfactant use or combination with intratracheal steroids outside the scope of research at this time.
Caffeine
Caffeine is widely used in the neonatal intensive care unit (NICU). In 1977, in a prospective study consisting of eighteen patients, Aranda et al. first showed that caffeine was effective for treating apnea of prematurity (55). Caffeine is a methylxanthine class-central nervous system (CNS) stimulant that primarily acts by inhibiting the adenosine receptors A1 and A2A in the brain (56–58). In addition, caffeine improves diaphragmatic contractility and prevents diaphragmatic fatigue by increasing intracellular Ca2+ and responsiveness of the central and peripheral chemoreceptors to CO2, resulting in increased minute ventilation (58). The bronchodilator effect of caffeine was shown to improve lung mechanics and minute ventilation in sixteen preterm infants with BPD (59). Additionally, caffeine also induces diuresis and natriuresis via antagonism of the renal tubular adenosine A1 receptors (60). However, neither the bronchodilator effect nor the diuretic effect appears to be the primary mechanism of action of caffeine.
The caffeine for apnea of prematurity (CAP) trial was a landmark trial in neonatology. It was a multicenter RCT that studied the long-term effects of caffeine administration starting before ten days of age (median: 3 days) in infants <1,500 g on the combined outcome of death and neurodevelopmental outcomes at 18–21 months of corrected age. This study not only established the safety of long-term caffeine use in premature infants but also showed a decreased incidence of BPD with early caffeine administration (p < 0.001) (61). By preventing apnea of prematurity, caffeine reduces the need for intubation and facilitates early successful extubation of ventilated infants, thus decreasing the MV-associated lung injury, which plays a key role in BPD pathogenesis (62). The CAP trial also showed that only 33.8% of infants exposed to caffeine underwent medical or surgical patent ductus arteriosus (PDA) closure compared to 50.7% of infants exposed to placebo (p < 0.001) (61). The low rate of PDA requiring treatment in the caffeine group may be related to the prostaglandin antagonist effect of methylxanthines (63). Long-term exposure to moderate-severe PDA has been implicated in the pathogenesis of BPD (64).
Animal studies have also shown that caffeine prevents inflammation, improves alveolarization, and upregulates vascular endothelial growth factor (VEGF) and hypoxia-inducible factor 1α (HIF-1α) expression, thus improving pulmonary vascularization following hyperoxia-induced lung injury (65–68). Although one study showed increased alveolar apoptosis with caffeine administration in mice lung exposed to hyperoxia; this effect could be the result of a higher dose used than in other studies (69).
The benefits of caffeine in BPD prevention also depend on the timing of initiation of therapy. The definition of early administration has changed since the CAP trial and is now defined as caffeine administration before three days. In a retrospective study, early administration (<3 days) of caffeine was associated with a significantly lower rate of BPD and the composite outcome of death or BPD compared to late caffeine administration (≥ 3 days) (70). Several RCTs and meta-analyses have confirmed this finding (71, 72). The National Institute for Health and Care Excellence (NICE) recommends routine caffeine use for all neonates ≤ 30 weeks' GA as early as possible (73). An RCT from the Netherlands consisting of infants between 24–30 weeks' GA showed that the infants who received caffeine immediately after birth in the delivery room had significantly higher tidal volumes, minute ventilation, and rate of rise to maximum tidal volume compared to those who received caffeine later in the NICU (74). However, the sample size in this study was small (n = 30) and it did not study the long-term effects, including MV duration, BPD, IVH, sepsis, or NEC (74). Another prospective study (the CAFROOM trial, NCT04044976) that will consist of 40 infants 25 0/7 to 29 6/7 weeks GA receiving an initial dose of caffeine (20 mg/kg) in the delivery room within 10 minutes of life is currently underway (75).
A higher maintenance dose of caffeine was associated with lower rates of BPD than the standard dose in many recent meta-analyses; however, firm recommendations could not be provided due to a low level of evidence (76–78). The follow-up data from 142 children enrolled in the CAP trial at the Royal Women's Hospital, Melbourne (52% treated with caffeine and 48% treated with placebo) showed that the caffeine group had significantly improved expiratory flow rates at 11 years compared to the placebo group (79).
The use of caffeine may be associated with side effects, including hyponatremia, hypertension, hyperglycemia, feeding intolerance, and tachycardia. The risk of necrotizing enterocolitis (NEC) was not increased with caffeine use (80).
In line with the NICE guidelines, we recommend routine caffeine use for all premature infants ≤ 30 weeks' GA soon after birth.
Postnatal Corticosteroids
Lung inflammation is often seen in BPD and plays a significant role in its pathogenesis. Corticosteroids, both systemic and inhaled, with their anti-inflammatory action, are often used for BPD prevention and management (81). However, despite over 80 RCTs studying their efficacy and safety, the timing and indications for postnatal corticosteroids (PNS) use for BPD remain a topic of debate among neonatologists (82).
Systemic Corticosteroids
The first RCT assessing the efficacy of systemic PNS in preterm infants (2–6 weeks age) was conducted in 1985 by Avery et al. The study group infants were weaned off MV within 72 hours of dexamethasone treatment and showed a significant improvement in pulmonary compliance compared to the control group (83).
Dexamethasone and hydrocortisone are the most frequently used systemic PNS. Both early (≤7 days) and late (>8 days) administration has been evaluated in various RCTs. There is a wide variation in published studies regarding the dosing of both dexamethasone and hydrocortisone used for BPD prevention (84, 85).
Early Systemic PNS
The Cochrane review by Doyle et al. (85) included 32 RCTs with early PNS use; 21 studies used dexamethasone and 11 studies used hydrocortisone. In this review, the risk of BPD and the combined outcome of BPD or death was lower with early PNS. Although there was no increase in mortality, the risk of gastrointestinal (GI) bleeding increased approximately two times and that of cerebral palsy (CP) increased almost 1.5 times (85). Interestingly, in the subgroup analysis, both the decreased BPD and the increased risks of CP and GI bleeding were seen in the dexamethasone group and not in the hydrocortisone group. In addition, the incidence of hyperglycemia, hypertension, and major neurosensory disability was higher, and that of retinopathy of prematurity (ROP) and PDA was lower with the dexamethasone group (85).
The PREMILOC trial deserves a special mention. Infants <28 weeks' GA were randomized to receive early low-dose intravenous (IV) hydrocortisone (0.5 mg/kg/dose; twice daily for 7 days followed by once daily for 3 days) or a placebo. Hydrocortisone significantly improved BPD-free survival at 36 weeks' PMA (60% vs 51%; p = 0.04) (86).
Late Systemic PNS
The DART (Dexamethasone A Randomized Trial) study by Doyle et al. is a randomized placebo-controlled trial that enrolled 70 infants (<28 weeks' GA or <1,000 g birth weight) dependent on MV at >1 week of life. The treatment (DART) group received 0.89 mg/kg dexamethasone over 10 days (87). This low-dose dexamethasone facilitated successful extubation in more infants by 10 days of treatment (odds ratio [OR]: 11.2; 95% CI: 3.2–39). However, BPD at 36 weeks' PMA, CP at two years of age, and mortality between the two groups remained unchanged with DART (87, 88).
In a retrospective study including 951 infants <27 weeks' GA, the lowest rate of BPD was noted when infants were treated with PNS between weeks two to seven but higher with treatment from week eight onwards (89).
In the Cochrane review assessing late systemic PNS for BPD prevention (2017), preterm infants were found to have a lower incidence of BPD and that of combined death or BPD at 36' weeks PMA with late PNS use, likely due to the facilitation of extubation. Unlike early PNS, late PNS use did not increase the risk of GI bleeding or CP but was associated with an increased rate of hyperglycemia, ROP, and hypertension. Out of the 21 RCTs in this review, only one used late hydrocortisone, while others used late dexamethasone (84).
The STOP-BPD trial was a double-blinded RCT recently published (2019) in which 372 infants <30 weeks and/or 1,250 g birthweight were randomly assigned to receive systemic hydrocortisone (72.5 mg/kg over 22 days) or a placebo. Infants were enrolled if they were MV-dependent in the 2nd week of life. There was no significant difference in the BPD incidence at 36 weeks' PMA or the composite outcome of death or BPD at 36 weeks' PMA with hydrocortisone use. However, death at 36 weeks' PMA was higher in the placebo group. Despite an initial decrease in extubation failure with hydrocortisone, this difference was not seen 21 days after starting the treatment (90).
Prednisolone and betamethasone are less frequently used for the management of BPD (91–94). Two RCTs compared betamethasone to hydrocortisone and dexamethasone, respectively, and found them comparable (91, 92). In a prospective study, Bhandari et al. demonstrated that prednisolone administration facilitated weaning off supplemental oxygen in infants >36 weeks' PMA. However, multiple courses of prednisolone were not practical (93). In a retrospective study, improvement in respiratory status was observed after one week of prednisolone therapy in mechanically ventilated infants; however, no further improvement was noted with continued therapy after one week (94).
Various studies have demonstrated an increase in the risk of neurodevelopmental impairment (NDI) with both early and late dexamethasone use (95–97). In a meta-analysis by Doyle et al., infants with a low baseline risk of moderate to severe BPD (< 33%) had a higher incidence of both CP and death with PNS use. On the contrary, the composite incidence of death or CP in those with a higher baseline risk (>60%) of BPD is lower with PNS administration (98). A retrospective study by Powell et al. also demonstrated a significant positive correlation between the cumulative dexamethasone dose and CP (99).
Another adverse effect of systemic PNS use is the potential to cause adrenal suppression, which occurs when supraphysiologic doses of corticosteroids (equivalent to > 10 mg/m2/day) are administered for >14 days (82).
The policy statement by the American Academy of Pediatrics (AAP) Committee on fetus and newborn issued in 2010 and reaffirmed in 2014 did not recommend the use of high-dose dexamethasone (~0.5 mg/kg per day) and noted that there was insufficient evidence for the use of low-dose dexamethasone (<0.2 mg/kg per day) and hydrocortisone for prevention/management of BPD (100). AAP has not revised this statement since then. A recent article by Stark and Eichenwald recommended use of low-dose dexamethasone in infants who continue require mechanical ventilation and oxygen support. However, this was a weak (grade 2C) recommendation and has not been officially endorsed by the AAP (101).
Inhaled Corticosteroids
To overcome the systemic side-effects of corticosteroids, inhaled corticosteroids (ICS) such as budesonide, fluticasone, and beclomethasone have been used to prevent/manage BPD. Both late and early use of ICS in preterm infants has been studied. Despite being commonly used in extremely preterm infants, both before and after discharge from the NICU, the evidence for ICS use remains unclear.
In the NEuroSIS trial, a multinational RCT, high dose (200-400 μg) inhaled budesonide, or placebo was administered to preterm infants <28 weeks' GA within 24 hours of life and continued until the infant was weaned off respiratory support or until 32 weeks' PMA (whichever was earlier). The budesonide group had a significantly lower incidence of BPD compared to the control group (27.8% vs. 38%; p = 0.004); however, the mortality rate was higher, albeit not significant (16.9% vs. 13.6%, p = 0.17). Inhaled budesonide did not increase the risk of NDI (102).
In a small RCT consisting of 18 preterm infants with established BPD, Yuskel et al. demonstrated decreased coughing and wheezing during infancy and improved functional residual capacity six weeks after initiating beclomethasone therapy (103). However, no reduction in invasive MV duration, supplemental oxygen use, or respiratory symptoms was noted with fluticasone use in preterm infants with moderate-severe BPD in two RCTs (104, 105). The Cochrane review studying the effect of late (>7 days) ICS use in preterm infants included eight studies and did not find a decline in BPD rate at 36 weeks' PMA or mortality. No increase in adverse events was seen with late ICS use (106).
A Cochrane review by Shah et al. found ICS and systemic PNS to be similar in terms of incidence of BPD at 36 weeks' PMA, mortality, GI bleeding, NEC, ROP, or neurodevelopment (107). Intratracheal administration of corticosteroids with surfactant is discussed in the surfactant section of this review.
Early inhaled budesonide or early systemic dexamethasone use cannot be recommended due to the increased risk of GI perforation, NDI, and mortality. Late (>7 days) PNS may be considered in selective infants dependent on invasive MV and/or high concentrations of supplemental oxygen if the benefits of PNS use are likely to be higher than the risk of adverse effects according to the clinical judgment of the provider; these infants are at high risk of mortality or adverse neurodevelopmental outcomes. The risk of BPD for infants <31 weeks' GA can be calculated using the neonatal BPD outcome estimator available at https://neonatal.rti.org/index.cfm and may be used to assess the risks and benefits of PNS in these infants (108). The neonatal provider should explain these risks and benefits to the patients' parents and the decision regarding PNS use should be made jointly with them. When systemic PNS use is considered, the highest benefit is seen when PNS are administered between 8-49 days of life (89).
Prophylactic Non-steroidal Anti-inflammatory Drugs (NSAIDs)
Gay et al. first suggested that in mechanically ventilated preterm infants, a large PDA with a left to right shunt for more than four days was associated with the development of severe BPD (109). A hemodynamically significant PDA results in increased pulmonary fluid filtration rate, reactive inflammatory changes, and alteration in the maturation of the pulmonary vasculature, all of which contribute to the pathogenesis of BPD (110).
A multicenter RCT consisting of 421 patients with hemodynamically significant PDA showed a 79% closure rate in infants treated with indomethacin at <5 days of age, compared to a 28% closure rate in infants receiving placebo (p < 0.05). However, this increase in the PDA closure rate did not correlate with a decrease in BPD or mortality in the indomethacin group (111). Although prophylactic NSAIDs have been shown to increase the rate of PDA closure in preterm infants, the utility of prophylactic indomethacin or ibuprofen in reducing morbidity and mortality has remained controversial. A Cochrane review published in 2010 did not find a difference in the rate of BPD or duration of oxygen use with prophylactic indomethacin administration (112). In a large retrospective study by the NICHD research network consisting of 7,831 infants, prophylactic indomethacin use was associated with a significantly lower requirement for PDA treatment after the first day of life, without a decrease in the incidence of BPD, death, or their combined outcome (113).
In a prospective double cohort-controlled study consisting of 397 infants, Liebowitz and Clyman showed a lower risk of BPD and that of BPD or death when indomethacin treatment was started <15 h of age compared to conservative treatment (114). On the contrary, Pan et al. showed a higher incidence of BPD in infants receiving prophylactic indomethacin (55 vs. 41%, p = 0.014) (115). The recent Cochrane review by Ohlsson et al. included only one RCT comparing the incidence of BPD at 36 weeks' PMA and that of mortality with prophylactic (<72 h) treatment of asymptomatic PDA with IV ibuprofen to placebo (116). Prophylactic ibuprofen did not decrease the incidence of death or BPD (116, 117). The Baby-OSCAR trial (ISRCTN84264977) and the BeNeDuctus trial (NCT02884219) are multicenter RCTs designed to study the difference between prophylactic (<72 hours) treatment with ibuprofen vs. placebo. These studies will likely provide more information and help build a consensus about prophylactic NSAIDs use (118, 119).
Indomethacin treatment in neonates can cause oliguria and acute kidney injury. An increased risk of gastrointestinal perforation has been observed with simultaneous use of corticosteroids and NSAIDs (112, 116, 120).
In the absence of evidence of improved BPD outcomes, we do not recommend routine prophylactic NSAID therapy for BPD prevention in preterm infants.
Vitamin A
Retinol or Vitamin A is required for the growth and differentiation of respiratory epithelial cells. Plasma vitamin A and retinol-binding protein levels were low in preterm infants who developed BPD (121). Vitamin A for BPD prevention was initially studied in 1987 in an RCT by Shenai et al. 40 infants at high risk of developing BPD were enrolled. Infants in the Vitamin A group received 2000 IU of intramuscular Vitamin A every other day starting postnatal day 4 for a total of 14 doses. The incidence of BPD declined remarkably with Vitamin A treatment (45 vs. 85%) (122). This trial, however, was conducted before the widespread use of surfactant therapy and antenatal corticosteroids.
An RCT by Pearson et al. conducted in 1992, consisting of 49 infants using the same dose as Shenai et al., did not reveal a benefit in decreasing BPD with Vitamin A use (46 vs 44%) (123). Due to inconsistent results obtained with various studies regarding the benefit of retinol in the prevention of BPD, a study was conducted by the NICHD neonatal research network to determine the appropriate dosage of Vitamin A that could be administered without significant side effects. Vitamin A administered at 5,000 IU/dose 3x/week for 12 doses was found to be the most effective in increasing serum retinol levels in preterm infants without signs of clinical toxicity (124). A follow-up RCT published by the NICHD neonatal research network in 1999 used this dose and demonstrated a decrease in CLD rate with vitamin A use (number needed to treat (NNT) = 14–15) (125). Subsequently, most studies used a dose of 5,000 IU or higher.
In animals subjected to oxidative lung injury, retinoic acid supplementation has been shown to increase mean alveolar area, improve alveolar regeneration, decrease pulmonary fibrosis, and prevent oxidative damage to the diaphragm (126–128).
The Cochrane review demonstrated a minimal risk reduction in BPD at 36 weeks' PMA and the composite outcome of death or oxygen requirement at four weeks of life with Vitamin A administration. The combined risk of death or BPD at 36 weeks' PMA was not altered with Vitamin A use (129). A meta-analysis by Ding et al. (130) included nine RCTs and 1409 premature infants. This study showed a lower incidence of BPD after Vitamin A administration (OR: 0.67; 95% CI: 0.52–0.88). Vitamin A did not increase the incidence of ROP, NEC, IVH, sepsis, or mortality (130). Although Vitamin A has shown to have some effect in preventing BPD, the increased cost of therapy, pain caused due to intramuscular injections and the modest benefit of the treatment made the neonatologists question “is Vitamin A worth the shot?” (131).
Interestingly, a recent reanalysis of the NICHD RCT conducted in 1997 showed that the risk reduction of BPD or death was greater in infants with lower risk compared to those with higher risk after intramuscular vitamin A administration (125, 131).
Alternate routes for vitamin A administration are being explored. Two RCTs showed higher plasma retinol levels in preterm infants receiving 5,000 IU/day oral vitamin A for 28 days, albeit with no effect on reduction in BPD in either study (132, 133). Another RCT by Basu et al. demonstrated a reduction in the combined effect of death or BPD at 36 weeks' PMA (RR 0.444; 95% CI: 0.229–0.844) after oral administration of alternate day 10,000 IU/dose for 28 days (134). Further studies are necessary to determine the appropriate oral dose required for BPD prevention. A large phase-III RCT, the NeoVitA trial, is currently enrolling patients. This study will test the effect of high dose oral vitamin A administration (5,000 IU/kg/day × 28 days) on BPD and/or mortality at 36 weeks' PMA (135).
Inhalational or endotracheal vitamin A has also been shown to be effective in animal studies but has not yet been evaluated in human trials (136, 137).
Based on current evidence, in centers with low rates of BPD, IM vitamin A cannot be recommended due to the minimal (if any) benefit and the associated discomfort.
Diuretics
Although regularly used in the NICU for BPD, diuretics remain a topic of controversy amongst neonatologists. Brief use of furosemide in BPD management has been reported as early as 1978. In a prospective study involving 28 preterm infants requiring MV, Sniderman et al. showed that the respiratory status improved in 20 of the 28 infants during the first trial and 14 of the 28 infants during the second trial of one week of furosemide therapy (138). Studies have shown improved short-term pulmonary compliance and decreased pulmonary resistance one to two hours after furosemide administration (139, 140). The decrease in alveolar-arterial oxygen gradient observed in the treatment group by Najak et al. was not observed by Patel et al. (141). However, the treatment group in the study by Patel et al. differed from that of Najak et al. in that none of the subjects in the former study were mechanically ventilated at the time of the study, and not all of them were diuretics-naïve (140, 141).
Only two RCTs consisting of a total of 39 infants have studied the long-term use (>1 week) of furosemide (142, 143). Improvement in oxygenation and compliance was noted in preterm infants > 3 weeks after 7–8 days of systemic furosemide use (142–144). Due to the potential complications of long-term furosemide, including nephrocalcinosis, hypokalemia, and hypochloremic metabolic alkalosis, neonatologists sought other diuretics for long-term use in infants with BPD.
In a randomized, double-blind crossover study by Kao et al., the use of chlorothiazide and spironolactone for one week was associated with a decreased mean airway resistance and increased mean dynamic lung compliance (145). However, in another parallel non-crossover RCT consisting of twenty-one infants with BPD, the combination of spironolactone-hydrochlorothiazide for one week did not affect the lung mechanics despite an increase in diuresis in the treatment group (146). In a subsequent placebo-controlled randomized trial by Kao et al., the chlorothiazide-spironolactone combination therapy was associated with a significant improvement in lung compliance (46%, p < 0.001) and resistance (36%, p < 0.05), and less supplemental oxygen use (p<0.01), when measured four weeks after the initiation of therapy. These favorable effects, however, did not persist after discontinuation of the diuretic therapy, and the use of diuretics did not decrease the number of days infants required supplemental oxygen (147).
A Cochrane review showed that airway resistance decreased significantly after one week of thiazide-spironolactone use but not after four weeks. The improvement of compliance was seen after one week and after four weeks of treatment. However, the authors advised exercising caution while interpreting the results due to the small number of patients included in this review (148).
The improvement of pulmonary function after diuretic use is independent of its effect on diuresis (140, 143, 146). As the adverse effects of furosemide use were associated with its action on the renal tubules, the idea that inhaled furosemide may be used to manage BPD was explored. Rastogi et al. first used nebulized furosemide in increasing doses in eight preterm infants with BPD dependent on MV (149). Lung compliance, pulmonary resistance, and tidal volume improved significantly 30 min to 4 h after a 1 mg/kg dose of nebulized furosemide without associated diuresis and renal adverse effects (149). Prabhu et al. showed no difference in the improvement of lung compliance and tidal volume in 13 preterm infants 24–28 weeks' GA dependent of MV and treated with 2 mg/kg compared to 1 mg/kg nebulized furosemide at >14 days of age (150). A Cochrane review including eight studies concluded that at a dose of 1 mg/kg, transient pulmonary function improvement was noted with aerosolized furosemide when used in preterm infants > 3 weeks of age with CLD (151).
None of the RCTs have demonstrated an effect on the duration of hospital stay, oxygen use or MV, or the incidence of BPD with the use of diuretics. However, a recent retrospective study from the Pediatrix medical group showed that more days of furosemide exposure (between postnatal day seven and corrected GA 36 weeks) was associated with a lower incidence of BPD and that of BPD/death (152).
The use of diuretics in neonatal ICUs varies widely throughout the country (153–155). In a retrospective study of over a hundred thousand infants < 32 weeks' GA and <1,500 g birthweight admitted to the NICUs managed by the Pediatrix group, 37% of infants received at least one diuretic, with furosemide being the most used (154). In another retrospective study including 41 hospitals with 1429 infants <29 weeks' GA diagnosed with BPD at 28 days of age, 86% infants received diuretics, and 4–86% infants received a diuretic course of > 5 days in the study hospitals (155). In 58% of all infants with established BPD and discharged home on diuretics, the diuretics were tapered or discontinued at the first outpatient visit. Also, the duration of outpatient therapy and taper were longer in patients discharged home on oxygen (156).
Due to the side effects of diuretics use and the absence of benefits, we cannot recommend routine chronic use of diuretics. However, sporadic doses of furosemide may be considered in preterm infants with worsening respiratory status with chest radiograph showing evidence of pulmonary edema, based on clinical judgment.
Bronchodilators
Bronchodilators include beta-agonists [isoproterenol, albuterol (also known as salbutamol), levalbuterol and terbutaline], anticholinergics (atropine and ipratropium), and methylxanthines (theophylline, aminophylline, and caffeine). Lungs of infants with BPD often have increased resistance and decreased compliance (157). The use of beta-agonists has been shown to reduce airway resistance in both intubated and non-intubated BPD patients and improve dynamic compliance and specific airway conductance in intubated BPD patients (158–161). The use of salbutamol was shown to be effective in improving compliance and resistance in very low birth weight (VLBW) infants as early as the second week of life (162). Similarly, inhaled atropine has been shown to improve dynamic compliance in non-intubated patients with BPD (159).
Studies have shown variable results with the combined use of anticholinergic and beta-agonist agents. In a double-blind, placebo-controlled randomized trial performed by Kao et al., although metaproterenol and atropine improved airway resistance in infants with BPD, no synergy was noted with their combined use in these patients (161). However, another RCT by Brundage et al. noted a significantly decreased airway resistance and improved lung compliance one to four hours after treatment with salbutamol + ipratropium compared to ipratropium alone (163).
Inhaled beta-adrenergic agents cause bronchodilatation through their action on β-2 receptors leading to smooth muscle relaxation. Ipratropium and atropine antagonize the action of acetylcholine, thus preventing parasympathetic bronchoconstriction and decreasing secretions. However, despite the short-term improvement in pulmonary mechanics, the use of bronchodilators has not been shown to prevent, treat or reduce the severity of BPD (164–168).
To date, only one RCT has studied the efficacy of beta-adrenergic medications for the prevention of BPD. This study, including 173 infants <31 weeks' GA and randomized to four groups (placebo, salbutamol, beclomethasone or salbutamol and beclomethasone), did not show any improvement in either survival, BPD and severity of BPD or the duration of MV and oxygen therapy in any of the treatment groups (164). Post-hoc analysis from the NEuroSIS trial showed no benefit of early bronchodilators (<48 h of life) in decreasing the incidence of BPD and/or death (169). Nevertheless, bronchodilators, particularly albuterol, continue to be frequently used in the NICU for patients with BPD (170). This is due to the belief that a subset of patients demonstrates airway reactivity that does respond to bronchodilator treatment (171, 172).
In a retrospective study consisting of 40 patients <1500 g birthweight, Morrow et al. found that 21patients (52.5%) had a decrease in resistance of ≥10% on pulmonary function tests (PFTs) (performed at mean PMA 34.9 weeks) after bronchodilator use (172). Another prospective study consisting of 44 children with BPD who received PFTs at 6, 12, and 24 months after initial discharge from the NICU showed that only 30% of the patients responded to bronchodilator treatment (171). The use of bronchodilators in the NICUs is variable and ranged from 0-81% in one study that included infants <29 weeks' GA and <1500 g birth weight (164). Among infants with BPD at 28 days of life, the use of inhaled bronchodilators ranged from 0-59%. Tracheostomy, duration of respiratory support, and exposure to steroids and diuretics positively correlated with the use of inhaled bronchodilators (173).
Although various delivery methods have been used for the administration of inhaled bronchodilators, the use of a metered-dose inhaler (MDI) and a spacer is preferred over nebulizer due to the shorter time required to deliver the medication without causing cooling of gases and lower risk of paradoxical increase in airway resistance (174–176).
Adverse effects of beta-agonists reported to date include hypokalemia, tachycardia, arrhythmias, tremors, hypertension, and hyperglycemia while those of anticholinergics include tachycardia, decreased gastrointestinal motility, tremors, and dry airway (167). It is important to note that the beta-agonists when used in the presence of tracheomalacia, which is often present in infants with BPD, can cause worsening of wheezing due to smooth muscle relaxation of an already floppy airway (177).
Theophylline and aminophylline have been shown to decrease bronchospasm and improve compliance by their action on the adenosine receptors. However, they have a narrow therapeutic window, and the adverse effects include gastrointestinal symptoms, tachycardia, agitation, and hypertension (167). Consequently, their use in the NICU has declined markedly over the years (170).
Thus, based on the current evidence, generalized use of bronchodilators cannot be recommended for either prevention or treatment of BPD. Larger RCTs studying the efficacy of bronchodilators in BPD are needed. Inhaled bronchodilators may be trialed for patients that demonstrate signs of increased airway resistance. Pulmonary function tests in infants with BPD may aid in selecting the appropriate candidates for the use of inhaled bronchodilators as well as their discontinuation when there is a lack of improvement.
Pulmonary Vasodilators
In preterm infants, pulmonary hypertension (PH) often complicates BPD and is known to increase mortality. PH in BPD has a fixed/structural and a reactive component. The fixed component is associated with abnormal development of the pulmonary vasculature following lung injury (178). The reactive component, associated with increased vascular resistance, is the target of various treatments used to treat BPD-associated PH (BPD-PH) (178). Endothelium-derived nitric oxide (NO) causes smooth muscle relaxation through increased levels of cyclic guanosine monophosphate (cGMP) (179). Impaired NO-cGMP signaling pathways have been implicated in BPD pathogenesis and BPD-PH development in experimental models (180). Pulmonary vasodilators such as inhaled NO, sildenafil and bosentan are often used in the NICU for management of BPD-PH.
Inhaled Nitric Oxide (iNO)
The use of iNO in preterm infants remains controversial. Endogenous NO promotes pulmonary vasodilatation by increasing cGMP and decreasing intracellular calcium (181). Through covalent attachment to cysteine, nitric oxide forms S-nitrosothiols, which improve peripheral vasodilatation (182). CLD models of preterm baboons were found to have lower nitric oxide synthase expression and enzyme activity and iNO treatment improved the ability of surfactant protein B to decrease surface tension, thus improving lung volume and dynamic compliance, and lowering expiratory resistance (183, 184). iNO has also been shown to reduce pulmonary inflammation and fibrin deposition, decrease endothelial cell apoptosis and enhance survival in rodent models of CLD (185, 186). It is known that early injury to the pulmonary vasculature results in smooth muscle proliferation, impaired vasodilatation, increased hypoxic vasoconstriction, and decreased arteriolar number, and pulmonary hypertension. Strategies that prevent pulmonary vascular injury may attenuate the development of PH associated with BPD (187).
Despite evidence supporting iNO use from pre-clinical studies, the benefits of iNO have not been proven in clinical studies involving preterm infants. iNO was first used in preterm infants with BPD who had acute hypoxemic respiratory failure due to pneumonia. At 3–10 ppm, iNO improved oxygenation in six infants with BPD complicated by pneumonia (188). Subsequent studies showed that iNO treatment also improved PaO2 in infants with severe BPD (189, 190). However, no evidence of PH was documented in either of these studies.
Unfortunately, there are no RCTs evaluating the efficacy of iNO in BPD-PH. iNO for the prevention of BPD has been studied in various RCTs. A Cochrane review studying the efficacy of iNO in preterm infants included seventeen RCTs (191). Compared to the control group, preterm infants who received routine iNO showed no difference in the overall incidence of death and/or BPD at 36 weeks' PMA (191–195). Similarly, no difference was noted in the above outcomes when the infants were treated with iNO based on supplemental oxygen requirement or BPD risk (191).
Another large RCT, the NEWNO trial published recently, consisting of 451 preterm infants <30 weeks GA and <1,250 g birthweight, receiving MV between postnatal age 5–14 days, showed no difference in BPD at 36 weeks' PMA or neurodevelopmental and respiratory outcomes at 18–24 months between the treatment and placebo groups (196).
In the NOCLD trial, the BPD-free survival was significantly higher with iNO treatment in African American infants compared to placebo (Relative benefit 1.66, 95% CI 1.16–2.37) (197). In the EUNO trial, although not significant, BPD-free survival was higher in black infants compared to non-black infants (194). However, in the study by Kinsella et al., no significant difference was reported in the composite outcome of death, intracranial hemorrhage, or periventricular leukomalacia between white and non-white infants ≤ 34 weeks gestation, treated with prophylactic iNO. This study did not report on the difference in BPD incidence between the two race groups (193). A recently published meta-analysis including three RCTs and a total of 1240 infants <34 weeks' GA found that black infants treated with iNO had a lower incidence of BPD at 36 weeks PMA and that of death or BPD at 36 weeks' PMA compared to those who received placebo (198).
iNO therapy appears to be mostly safe with no increase in the incidence of IVH, NDI, CP, or ROP when used in preterm infants (191). However, long-term data regarding iNO use in preterm infants are limited. Interestingly, follow-up at age one year of 456 infants enrolled in the NOCLD study showed a significantly lower use of bronchodilators, ICS, systemic PNS, diuretics, and supplemental oxygen post-NICU discharge in infants who received iNO (199). Follow-up at seven years of infants enrolled in the EUNO trial did not demonstrate a significant difference in the hospitalization rates or the use of respiratory medications between the two groups (200).
Per the NIH consensus statement from 2010, the available evidence does not support the use of iNO either as routine or as rescue therapy in premature infants <34 weeks' GA requiring respiratory support. Additionally, the group has stated that iNO has been inadequately studied in rare conditions like pulmonary hypertension or hypoplasia in infants <34 weeks' GA, and in such cases, its use should be after discussing the risks, benefits, and uncertainties with the families (201). The data from studies published since this statement are insufficient to recommend routine iNO use for BPD prevention. Further studies are needed to confirm and evaluate the basis for the racial disparity in the responsiveness to iNO and the outcomes. (191, 195, 196, 198).
Based on the current evidence, we do not recommend routine use of iNO for either BPD prophylaxis or the treatment of BPD-associated PH. However, iNO use may be considered in individual cases of BPD during acute PH crises.
Sildenafil
Sildenafil is a selective phosphodiesterase-5 (PDE-5) inhibitor that inhibits the degradation of cGMP, thereby prolonging the actions of cGMP, resulting in smooth muscle relaxation. The use of sildenafil in the NICUs has increased over the years.
In a retrospective study by Thompson et al., sildenafil was administered to 0.11% of infants discharged from the NICUs managed by the Pediatrix medical group over 16 years, with an increase from 0% in 2001 to 0.17% in 2016 (202). Sildenafil is FDA-approved for the treatment of PH in adults, but its use in children remains off-label. There is a paucity of evidence on the safety and efficacy of sildenafil in patients with BPD-PH. Hon et al. first reported using sildenafil for the treatment of BPD-PH in 2005 (203). Most of the current literature on sildenafil in established BPD includes individual case reports, case series, or retrospective studies.
A recent meta-analysis published in 2019, which consisted of five retrospective studies including 101 patients, showed that sildenafil use was associated with an improvement in the respiratory scores in 15% infants (95% CI: 0.0–30.4) within 2–7 days and >20% improvement in the estimated pulmonary arterial pressure within 1–6 months in 69.3% (95% CI: 56.8–81.8) preterm infants with BPD-PH, without any serious adverse events (204). It is, however, difficult to ascertain from this study, whether the improvement in pulmonary arterial pressures was the result of treatment with sildenafil or simply that of tincture of time.
Side effects of sildenafil reported in children include hypotension, tachycardia, priapism, and facial flushing (205). A recent phase 1 trial by Jackson et al. found more adverse effects with faster intravenous use compared to enteral use. Adverse effects listed in this study included hypotension, NEC, and cardiogenic shock, of which only hypotension was determined to be drug-related (206).
Larger prospective studies and RCTs are required to study the utility and safety of this drug in the neonatal population. The SILDI-SAFE trial is a multicenter, sequential dose-escalating, placebo-controlled, double-blinded randomized study expected to complete in December 2023 and will provide valuable information on the safety of sildenafil in preterm infants with severe BPD (207). However, since this study will include only patients with BPD without PH, the efficacy of sildenafil to treat already established BPD-PH cannot be evaluated in this study.
Prophylactic sildenafil in neonatal rats exposed to hyperoxia has been shown to improve alveolarization, promote angiogenesis, decrease lung inflammation and fibrin deposition, and thus decrease BPD via activation of hypoxia-inducible factor signaling pathway (208–210). However, RCTs studying the effect of prophylactic sildenafil in preterm infants did not demonstrate any significant impact on BPD or death (211, 212).
Sildenafil may be used in select premature infants with BPD-associated PH after consultation with a pediatric cardiologist and a pediatric pulmonologist; however, the quality of evidence for its use remains low. There is no role for sildenafil in BPD prevention outside of research setting.
Bosentan
An increase in endothelin-1 (ET-1) expression occurs in the vascular endothelial cells in PH (213). Bosentan, a non-selective competitive antagonist of ET-1 receptor, has been shown to reverse endothelin-mediated smooth muscle constriction, hypertrophy, and hyperplasia (178, 214, 215).
Although not approved by the FDA for children under three years of age, off-label bosentan has been reported to improve PH in infants with BPD, either alone or in combination with sildenafil, in various retrospective studies (216–218). In common practice, it is often used in severe BPD as second-line therapy after sildenafil. A dose of 1–2 mg/kg/dose twice daily is used.
Larger prospective studies are needed to study the safety and efficacy of bosentan in the treatment of BPD-associated PH. In the rodent model of hyperoxia-induced lung injury, bosentan treatment was found to have a protective effect with lower fibrosis and inflammation (219). There are no studies evaluating bosentan use in preterm infants at risk of developing BPD.
Potential adverse effects of bosentan include hepatotoxicity, fluid retention, and dosage-related decline in hematocrit and hemoglobin. Liver function tests should be measured before starting bosentan and monthly thereafter (220).
With no documentation of efficacy and the risk of serious adverse effects, we cannot recommend routine bosentan use in infants with BPD-associated PH at this time. However, where the use of bosentan as a second-line treatment for BPD-PH is considered, it should be done so after discussion with the pediatric cardiologist and after discussing the potential risks with the parents/family.
Macrolides
Ureaplasma colonization has been associated with the development of BPD in various studies (221–223). In a meta-analysis by Wang et al., the risk of development of CLD was 1.72 (95% CI 1.5–1.96) times higher in colonized infants than uncolonized infants (221). The anti-microbial activity of macrolides against Ureaplasma and their anti-inflammatory properties led to research into the use of macrolides to prevent BPD, where inflammation is a key driver. Macrolides exert their anti-inflammatory action by inhibiting neutrophil chemotaxis, tumor necrosis factor (TNF)-α, interleukin (IL)-1, and IL-6 (224, 225).
Earlier studies in the 20th century and the early 21st century did not demonstrate a decrease in either the incidence or the severity of BPD in preterm infants with erythromycin use at various dosages (226–228). This was thought to be due to the failure of erythromycin to eliminate Ureaplasma colonization (228).
Ballard et al. first studied the role of azithromycin in preventing BPD in 43 extremely low birth weight (ELBW) premature infants. In this pilot, double blinded, RCT, infants <72 h of life were randomized to receive a placebo or azithromycin within 12 h of starting MV. The study group received azithromycin 10 mg/kg/day x7 days followed by 5 mg/kg/day until the infant was off MV or supplemental oxygen for a maximum of 6 weeks. Although the rates of mortality and BPD were similar in the two groups, a significantly lower proportion of infants who received azithromycin received PNS (31 vs. 62%; p = 0.05). The treatment group also had a significantly lower MV duration (median 13 days vs. 35 days, p = 0.02) (229). This was thought to be due to increased anti-inflammatory and anti-microbial activity of azithromycin against Ureaplasma compared to erythromycin (229, 230). However, in addition to the lack of beneficial effect on mortality or BPD, a larger study consisting of 220 infants <1,250 g by the same group did not demonstrate any benefit in decreasing either PNS use or duration of MV (231).
Subsequently, two RCTs have demonstrated a significant reduction in BPD incidence at 28 days with azithromycin use (232, 233). IV azithromycin (10 mg/kg/day for five days) administered within 12 h of initiation of MV and within 72 h of birth significantly decreased the levels of plasma IL-2 and IL-8 in VLBW infants (233). A meta-analysis by Nair et al. found that azithromycin significantly reduced the incidence of BPD at 36 weeks' PMA (RR 0.83, 95% CI 0.71–0.98) (234). However, a recent RCT published in 2020 that included infants between 24–28 weeks' GA showed no significant improvement in BPD at 36 weeks' PMA or death with azithromycin treatment regardless of colonization status (235).
Azithromycin has been well-tolerated in neonates in various observational and randomized studies (236, 237). Azithromycin causes less diarrhea and abdominal discomfort compared to erythromycin. However, similar to erythromycin, azithromycin is associated with an increase in the risk of infantile hypertrophic pyloric stenosis, especially when administered within 14 days of life. This is likely due to their agonistic effect on the gastric motilin receptors (236, 238). Arrhythmias were not reported with the use of azithromycin in neonates despite the reports of prolonged QTc and torsades de pointes in adults (236).
A large RCT, the AZTEC trial (ISRCTN11650227), aims to study the effect of a 10-day course of early IV azithromycin (20 mg/kg (days 1–3) followed by 10 mg/kg (days 4–10) administered within 72 h of birth to neonates <29 weeks' GA) at 36 weeks' PMA and is currently enrolling patients (239).
Only one study using clarithromycin to prevent BPD has been published to date. This RCT showed a significantly lower rate of BPD in infants with Ureaplasma urealyticum-positive cultures treated with clarithromycin compared to placebo (2.9 vs. 36.4%; p < 0.001) (240). However, this was an underpowered study and enrolled only 30% of the intended subjects.
While azithromycin may help decrease the incidence of BPD, there is insufficient evidence regarding the dosage, duration, and timing of therapy. Due to the risk of side effects, we do not currently recommend routine prophylactic use of azithromycin for BPD prevention in premature infants.
Future Directions
Table 3 outlines the various experimental drugs currently being studied for the management of BPD.
Stem Cell Therapy
Mesenchymal stromal cell (MSC) therapy is a relatively new treatment that may prove to be an essential weapon in the neonatal provider's arsenal against BPD. However, it currently remains to be in the experimental phase. MSCs can be derived from many sources, including embryonic tissue, umbilical cord/umbilical cord blood, placental tissue or adult bone marrow, liver, or fat (241, 242). MSCs facilitate healing by preferentially entering sites of injury, secreting factors that promote cell growth, cell proliferation, and angiogenesis. They also secrete chemokines that prevent oxidation and fibrosis. Additionally, MSCs inhibit inflammation by acting as immunomodulators and inhibiting the proliferation and the function of lymphocytes, monocytes, and macrophages (241). Several pre-clinical studies have demonstrated the benefit of MSCs in animal models of BPD, and earlier administration is associated with better outcomes (243, 244). A meta-analysis of these studies showed that MSCs improved alveolarization, decreased inflammation, decreased fibrosis, prevented pulmonary remodeling, improved pulmonary angiogenesis, and resulted in lower rates of pulmonary hypertension compared to controls (245). Co-administration of MSC + surfactant is associated with higher lung injury compared to MSC alone, likely due to surfactant-induced decrease in the viability of MSC (246).
A very limited number of clinical trials have studied the use of MSC in preterm infants with risk of or diagnosis of BPD. Chang et al. performed the first phase I study using human umbilical cord blood-derived MSC (hUC-MSC; PNEUMOSTEM®) in nine preterm infants (24-26 6/7 weeks' GA). An intratracheal dose of 1 x 107 cells/kg and 2 x 107 cells/kg was delivered to the first three and the last six patients, respectively (247). hUC-MSC administration was demonstrated to be safe and inflammatory cytokines were lower in infants after hUC-MSC administration. Also, BPD severity was lower in the HUC-MSC group than in the matched controls (247). The 2-year follow-up showed decreased rates of home oxygen use and neurodevelopmental disability, including CP in the transplant group compared to the infants who did not receive MSC (248). This group recently completed a double-blind RCT recruited 66 infants (23–28 weeks' GA) receiving MV at the time of enrollment, randomly allocated to receive intratracheal 1 x 107 cells/kg hUC-MCS or saline between days 5–14. Although infants who received hUC-MSC had decreased intratracheal inflammatory markers, the rate of death or severe/moderate BPD was similar between the two groups. Of note, severe BPD was lower with hUC-MSC use in the 23–24-week group (249). Due to the small sample size in each of these studies, the results must be interpreted with caution. Another phase I study from the United States enrolled 12 infants (23–27 6/7 weeks' GA and 500–1,000 g birth weight). 10/12 patients in the treatment group had severe BPD at 36 weeks (250).
There are ongoing phase I and phase II studies in Spain, Korea, Vietnam, and China (241).
The MSC-BPD trial (NCT03601416) is a randomized dose-escalating open-label phase II trial from China which will include 72 infants <1 year of age with the diagnosis of BPD, randomly allocated to control group, low-dose IV hUC-MSC (2.5 × 106 cells/kg,) and high dose IV hUC-MSC (5 x 106 cells/kg) groups in the 1:1:1 ratio (251). This will be a vital trial to study the efficacy of MSC in already established BPD.
MSC-extracellular vesicles/exosomes have also shown promise in various animal studies by immunomodulating macrophages, decreasing lung inflammation and fibrosis, as well as restoring lung architecture in hyperoxia-induced BPD (252–255). The UNEX-42 trial (NCT03857841) was a phase 1 RCT studying the safety of MSC exosomes in infants <27 weeks. It enrolled three subjects but was discontinued due to a business decision (256). Other forms of stem cells that have been studied include endothelial progenitor cells (EPCs) and human amnion epithelial cells (hAECs) (257–259).
Erythropoetin (EPO)
Recombinant human EPO (rhEPO) treatment in neonatal rats exposed to hyperoxia upregulated epidermal growth factor-line domain 7 and was associated with decreased alveolar simplification, improved angiogenesis and decreased fibrosis (260, 261). However, EPO administration in preterm lambs increased alveolar inflammation and exacerbated ventilator associated lung injury (262). In a multi-center prospective cohort study including 867 neonates <28 weeks' GA, neonates with higher blood EPO concentrations (concentrations in the 4th quartile) on day 14 of life had a higher incidence of moderate but not severe BPD (263).
While retrospective studies have demonstrated a potential benefit of erythropoietin in reducing BPD in preterm infants, a meta-analysis consisting of 17 RCTs showed no difference in the incidence of BPD between infants receiving EPO and placebo (264–266). The PENUT trial (Preterm Erythropoetin Neuroprotection trial) also showed no reduction in the incidence of BPD after high-dose EPO supplementation (267). EPO use did not increase the incidence of ROP, sepsis or NEC (266, 267).
Administration of EPO in combination with MSCs has also been shown to attenuate lung injury by promoting angiogenesis and decreasing fibrosis in murine hyperoxia induced lung injury models (268, 269). EPO in combination with MSCs has not yet been studied in humans.
Docosahexaenoic Acid (DHA)
DHA is a long-chain polyunsaturated fatty acid with anti-inflammatory and antioxidant properties (270). In the DINO trial, a large RCT consisting of over 600 infants < 33 weeks' GA, Manley et al. documented decreased BPD incidence in male infants and infants <1.25 kg consuming maternal breastmilk when mothers consumed tuna oil capsules (3000 mg/day) that contained a high amount of DHA. No difference was seen in female infants or infants >1.25 kg (271). However, a recent study, the N3RO trial including over a thousand infants <29 weeks' GA showed a significantly higher rate of BPD at 36 weeks' PMA and of BPD or death before 36 weeks' PMA in infants receiving 60 mg/kg/day of enteral DHA (272). Similarly, the MOBYDIck trial included mothers who delivered infants at <29 weeks' GA randomized to receive 1.2 g/day DHA capsules vs placebo. The investigators noted no significant improvement in BPD-free survival rates in infants whose mothers received DHA capsules (273). Due to lack of sufficient data, DHA must only be used in research setting. Larger clinical studies are required to determine the appropriate dose as well as to assess the efficacy of DHA in preventing BPD in extremely preterm infants.
Clara Cell Protein
Clara cell kDa 10-16 protein (also known as CC10/CC16/uteroglobin) is an anti-inflammatory immunomodulator present in abundance in the bronchoalveolar lavage (BAL) fluid of neonates (274, 275). CC10 has been identified as a potential biomarker for BPD (276). Studies have shown contradicting results on whether CC10 is elevated or decreased in the cord blood of infants that develop BPD (277–279). However, the CC10 levels in BAL fluid were found to be lower in infants that developed BPD (280, 281). In an RCT consisting of 22 infants randomized to receive either placebo, 1.5 mg/kg of recombinant human CC10 (rhCC10) or 5 mg/kg rhCC10 intratracheally, rhCC10 was found to be safe and decreased IL-6, neutrophil count, and total protein concentration in the tracheal aspirate during the first 3 days of life, but no difference was noted between the three groups in terms of BPD at 36 weeks' PMA (282). In another multicenter RCT consisting of 88 infants 24 0/7 to 29 0/7 weeks' GA, a single dose of intratracheal rhCC10 (1.5 mg/kg or 5 mg/kg) was found to be ineffective in changing long term respiratory outcomes at 12 months of age (283).
Superoxide Dismutase (SOD)
SODs are antioxidant enzymes shown to be decreased in various animal models of BPD (284, 285). Additionally, pulmonary angiogenesis was preserved after exposure to hyperoxia in transgenic mice that had increased expression of extracellular SOD (286). Rosenfeld et al. first showed a decrease in both radiologic and clinical features of BPD after subcutaneous administration of multiple doses of bovine SOD to infants with severe RDS (287). However, the RCT by Davis et al. found no difference in rate of death or that of BPD at 28 days or at 36 weeks' PMA in infants receiving recombinant human CuZn-SOD (rh-CuZn-SOD) or placebo. Of note, infants treated with rhCuZn-SOD had fewer episodes of wheezing, required asthma medications less often and had lower hospitalization rates before one-year corrected age (288). A recent study found no correlation between EC-SOD in serum and the risk of BPD in infants < 32 weeks' GA (289). SODs may potentially improve the long-term pulmonary outcomes of infants with BPD; however, further research is needed regarding their efficacy, safety and dosing before they can be used in the clinical setting.
Pentoxifylline
Pentoxifylline, a synthetic methylxanthine derivative, is a non-selective phosphodiesterase inhibitor with anti-inflammatory properties (290). Additionally, pentoxifylline has also been shown to prevent fibrin deposition and improve lung vascularization in rat pups exposed to hyperoxia (291, 292). Lauterbach et al. demonstrated an improvement in lung resistance and compliance and a decreased oxygen requirement after treatment with nebulized pentoxifylline in five preterm infants with BPD (293). Subsequently, in a non-blinded RCT, Lauterbach et al. demonstrated a significant decrease in the risk of BPD after treatment with pentoxifylline compared to placebo (OR: 0.32; CI: 0.11–0.94) (294). However, a recent double-blind RCT consisting of 81 infants between 23 0/7 to 27 6/8 weeks' GA did not show a difference in oxygen supplementation at 40 weeks' PMA after treatment with 10 days of nebulized pentoxifylline vs placebo (290). While promising, there currently exist limited data on both the efficacy as well as safety and dosing of pentoxifylline for prevention and treatment of BPD.
Citrulline
L-citrulline is byproduct of conversion of L-arginine and oxygen to NO (295). In the kidney, L-citrulline is converted back to L-arginine. As L-arginine has a low oral bioavailability, L-citrulline supplementation has been studied for its effect on the arginine/NO metabolism pathway, which has been shown to contribute to the development of BPD (295, 296). Hyperoxia exposure in rat pups was associated with lower L-citrulline and L-arginine concentrations in the plasma. L-citrulline administration in these pups exposed to hyperoxia resulted in preservation of lung angiogenesis and prevention of alveolar simplification and PH (297). Montgomery et al. showed that infants with BPD-associated PH had lower concentrations of L-citrulline (5–28 μmol/L) compared to those with BPD without PH (21–57 μmol/L) (p = 0.005) (298). On the contrary, infants with BPD had a higher concentration of citrulline in the tracheal aspirate compared to those without BPD. The authors theorized that this was likely due to an effort by the infants with BPD to prevent PH onset by producing higher NO levels (299). Lauterbach et al. described a case of successful treatment of BPD-associated PH with oral citrulline therapy (150 mg/kg/day x 70 days) in an ex-25 weeker male infant (300). L-citrulline may serve as a potential therapy for BPD-PH. However, in the absence of data from clinical studies regarding the efficacy and safety, the use of L-citrulline for BPD-associated PH should be reserved for research settings only.
Inositol
Inositol is a nutrient that promotes the production of phosphatidylcholine and phosphatidylinositol, and preterm infants with a premature decline in myoinositol have a more severe course of RDS (301). Many RCTs have evaluated the role of inositol in prevention of RDS and BPD. While no acute adverse effects were noted with inositol use, a recent Cochrane review found that inositol supplementation did not decrease the rates of BPD, death and the composite outcome of BPD or death in preterm infants and recommended against further clinical trials in neonates (302, 303).
Other Potential Treatments
Various potential targets including growth factors, anti-inflammatory agents, and antioxidants are currently being evaluated for the prevention of BPD in extremely premature infants. In a phase II RCT that included infants 23 0/7 to 27 6/7 weeks' GA, Ley et al. showed a significant decrease in severe BPD after treatment with recombinant human insulin-like growth factor (rhIGF-1) complexed with rhIGF-binding protein 3 (rhIGFBP3) (304). Other factors showing promise in the pre-clinical phase include growth factors such as HIF-1α and VEGF, anti-inflammatory agents like interleukin-1 receptor antagonists (ILR1A), and long non-coding and micro RNAs (lncRNA and miRNA) (305–310).
Conclusion
BPD continues to be one of the leading causes of mortality and morbidity in extremely preterm infants. Most of the pharmacologic agents currently in use for either the prevention or management of BPD provide minimal benefit, if any. While surfactant therapy and antenatal corticosteroids have failed to prevent BPD, one cannot underestimate their role in preventing RDS and overall mortality in preterm infants. Of the drugs currently available, early caffeine therapy provides the most promise in preventing BPD without increased risk of adverse effects. At our institution, we regularly prescribe caffeine to all infants <30 weeks' GA at birth, and it is continued until 34 weeks' PMA.
Due to the lack of evidence regarding the use of the other medications in BPD, we currently do not have any guidelines/protocols for their use. When using these medications, the neonatal provider should carefully weigh their possible benefits against the myriad of side effects most of them cause and individualize the therapy based on the infant's clinical picture. At this time, routine use of these medications cannot be recommended. Newer experimental therapies appear to be promising for BPD treatment/prevention in extremely preterm infants; however, further research is necessary before their safety and efficacy in clinical practice can be established.
Author Contributions
RS conceptualized the study and drafted the manuscript. RD conceptualized the study and revised the manuscript for critically important content. RS and RD reviewed the final draft of the manuscript and approved the final version. Both authors contributed to the article and approved the submitted version.
Conflict of Interest
The authors declare that the research was conducted in the absence of any commercial or financial relationships that could be construed as a potential conflict of interest.
Publisher's Note
All claims expressed in this article are solely those of the authors and do not necessarily represent those of their affiliated organizations, or those of the publisher, the editors and the reviewers. Any product that may be evaluated in this article, or claim that may be made by its manufacturer, is not guaranteed or endorsed by the publisher.
References
1. Northway WH, Rosan RC, Porter DY. Pulmonary disease following respirator therapy of hyaline-membrane disease. N Engl J Med. (1967) 276:357–68. doi: 10.1056/NEJM196702162760701
2. Jobe AH, Bancalari E. Bronchopulmonary dysplasia. Am J Respir Crit Care Med. (2001) 163:1723–9. doi: 10.1164/ajrccm.163.7.2011060
3. Husain AN, Siddiqui NH, Stocker JT. Pathology of arrested acinar development in postsurfactant bronchopulmonary dysplasia. Hum Pathol. (1998) 29:710–7. doi: 10.1016/s0046-8177(98)90280-5
4. Higgins RD, Jobe AH, Koso-Thomas M, Bancalari E, Viscardi RM, Hartert Tv, et al. Bronchopulmonary dysplasia: executive summary of a workshop. J Pediatr. (2018) 197:300–8. doi: 10.1016/j.jpeds.2018.01.043
5. Jensen EA, Dysart K, Gantz MG, et al. The diagnosis of bronchopulmonary dysplasia in very preterm infants. An evidence-based approach. Am J Respir Crit Care Med. (2019) 200:751–9. doi: 10.1164/rccm.201812-2348OC
6. Jensen EA, Schmidt B. Epidemiology of bronchopulmonary dysplasia. Birth Defects Res A Clin Mol Teratol. (2014) 100:145–57. doi: 10.1002/bdra.23235
7. Thébaud B, Goss KN, Laughon M, Whitsett JA, Abman SH, Steinhorn RH, et al. Bronchopulmonary dysplasia. Nat Rev Dis Primers. (2019) 5:78. doi: 10.1038/s41572-019-0127-7
8. Stoll BJ, Hansen NI, Bell EF, Walsh MC, Carlo WA, Shankaran S, et al. Trends in care practices, morbidity, and mortality of extremely preterm neonates, 1993-2012. JAMA. (2015) 314:1039–51. doi: 10.1001/jama.2015.10244
9. Principi N, Di Pietro GM, Esposito S. Bronchopulmonary dysplasia: clinical aspects and preventive and therapeutic strategies. J Transl Med. (2018) 16:36. doi: 10.1186/s12967-018-1417-7
10. Michael Z, Spyropoulos F, Ghanta S, Christou H. Bronchopulmonary dysplasia: an update of current pharmacologic therapies and new approaches. Clin Med Insights Pediatr. (2018) 12:1179556518817322. doi: 10.1177/1179556518817322
11. Jensen EA. Prevention of bronchopulmonary dysplasia: a summary of evidence-based strategies. Neoreviews. (2019) 20:e189–201. doi: 10.1542/neo.20-4-e189
12. Mandell EW, Kratimenos P, Abman SH, Steinhorn RH. Drugs for the prevention and treatment of bronchopulmonary dysplasia. Clin Perinatol. (2019) 46:291–310. doi: 10.1016/j.clp.2019.02.011
13. Williams E, Greenough A. Advances in treating bronchopulmonary dysplasia. Expert Rev Respir Med. (2019)13:727–35. doi: 10.1080/17476348.2019.1637738
14. Truog WE, Lewis TR, Bamat NA. Pharmacologic management of severe bronchopulmonary dysplasia. Neoreviews. (2020) 21:e454–68. doi: 10.1542/neo.21-7-e454
15. Roberts K, Stepanovich G, Bhatt-Mehta V, Donn SM. New pharmacologic approaches to bronchopulmonary dysplasia. J Exp Pharmacol. (2021) 13:377–96. doi: 10.2147/JEP.S262350
16. Muehlbacher T, Bassler D, Bryant MB. Evidence for the management of bronchopulmonary dysplasia in very preterm infants. Children (Basel). (2021) 8:298. doi: 10.3390/children8040298
17. Iyengar A, Davis JM. Drug therapy for the prevention and treatment of bronchopulmonary dysplasia. Front Pharmacol. (2015) 6:12. doi: 10.3389/fphar.2015.00012
18. Hennelly M, Greenberg RG, Aleem S. An update on the prevention and management of bronchopulmonary dysplasia. Pediatric Health Med Ther. (2021) 12:405–19. doi: 10.2147/PHMT.S287693
19. Gilfillan M, Bhandari A, Bhandari V. Diagnosis and management of bronchopulmonary dysplasia. BMJ. (2021) 375:n1974. doi: 10.1136/bmj.n1974
20. Liggins GC, Howie RN A. controlled trial of antepartum glucocorticoid treatment for prevention of the respiratory distress syndrome in premature infants. Pediatrics. (1972) 50:515–25.
21. Effect of Corticosteroids for Fetal Maturation on Perinatal Outcomes. NIH consensus development panel on the effect of corticosteroids for fetal maturation on perinatal outcomes. JAMA. (1995) 273:413–8. doi: 10.1001/jama.1995.03520290065031
22. Venkatesh VC, Katzberg HD. Glucocorticoid regulation of epithelial sodium channel genes in human fetal lung. Am J Physiol. (1997) 273:L227–33. doi: 10.1152/ajplung.1997.273.1.L227
23. Ballard PL. Scientific rationale for the use of antenatal glucocorticoids to promote fetal development. Neoreviews. (2000) 1:e83–90. doi: 10.1542/neo.1-5-e83
24. Millage AR, Latuga MS, Aschner JL. Effect of perinatal glucocorticoids on vascular health and disease. Pediatr Res. (2017) 81:4–10. doi: 10.1038/pr.2016.188
25. Haviv HR, Said J, Mol BW. The place of antenatal corticosteroids in late preterm and early term births. Semin Fetal Neonatal Med. (2019) 24:37–42. doi: 10.1016/j.siny.2018.10.001
26. Committee on Obstetric Practice. Committee opinion No. 713: antenatal corticosteroid therapy for fetal maturation. Obstet Gynecol. (2017) 130:e102–9. doi: 10.1097/AOG.0000000000002237
27. Brownfoot FC, Gagliardi DI, Bain E, Middleton P, Crowther CA. Different corticosteroids and regimens for accelerating fetal lung maturation for women at risk of preterm birth. Cochrane Database Syst Rev. (2013) CD006764. doi: 10.1002/14651858.CD006764.pub3
28. McGoldrick E, Stewart F, Parker R, Dalziel SR. Antenatal corticosteroids for accelerating fetal lung maturation for women at risk of preterm birth. Cochrane Database Syst Rev. (2020) CD004454. doi: 10.1002/14651858.CD004454.pub4
29. Deshmukh M, Patole S. Antenatal corticosteroids in impending preterm deliveries before 25 weeks' gestation. Arch Dis Child Fetal Neonatal Ed. (2018) 103:F173–6. doi: 10.1136/archdischild-2017-313840
30. Fujiwara T, Chida S, Watabe Y, Maeta H, Morita T, Abe T. Artificial surfactant therapy in hyaline-membrane disease. Lancet. (1980) 1:55–9. doi: 10.1016/s0140-6736(80)90489-4
31. Jobe AH. Pulmonary surfactant therapy. N Engl J Med. (1993) 328:861–8. doi: 10.1056/NEJM199303253281208
32. Soll RF. Synthetic surfactant for respiratory distress syndrome in preterm infants. Cochrane Database Syst Rev. (2000) 1998:CD001149. doi: 10.1002/14651858.CD001149
33. SUPPORT SUPPORT Study Group of the Eunice Kennedy Shriver NICHD Neonatal Research Network, Finer NN, Carlo WA, Walsh MC, Rich W, Gantz MG, et al. Early CPAP versus Surfactant in Extremely Preterm Infants. N Engl J Med. (2010) 362:1970–9. doi: 10.1056/NEJMoa0911783
34. Stevens TP, Blennow M, Myers EH, Soll RF. Early surfactant administration with brief ventilation vs. selective surfactant and continued mechanical ventilation for preterm infants with or at risk for respiratory distress syndrome. Cochrane Database Syst Rev. (2007) CD003063. doi: 10.1002/14651858.CD003063.pub3
35. Bahadue FL, Soll R. Early versus delayed selective surfactant treatment for neonatal respiratory distress syndrome. Cochrane Database Syst Rev. (2012) 11:CD001456. doi: 10.1002/14651858.CD001456.pub2
36. Fischer HS, Bührer C. Avoiding endotracheal ventilation to prevent bronchopulmonary dysplasia: a meta-analysis. Pediatrics. (2013) 132:e1351–60. doi: 10.1542/peds.2013-1880
37. Verder H, Robertson B, Greisen G, Ebbesen F, Albertsen P, Lundstrom K, et al. Surfactant therapy and nasal continuous positive airway pressure for newborns with respiratory distress syndrome. N Engl J Med. (1994) 331:1051–5. doi: 10.1056/NEJM199410203311603
38. Kribs A, Pillekamp F, Hünseler C, Vierzig A, Roth B. Early administration of surfactant in spontaneous breathing with nCPAP: feasibility and outcome in extremely premature infants (postmenstrual age ≤ 27 weeks). Paediatr Anaesth. (2007) 17:364–9. doi: 10.1111/j.1460-9592.2006.02126.x
39. Isayama T, Chai-Adisaksopha C, McDonald SD. Noninvasive ventilation with vs without early surfactant to prevent chronic lung disease in preterm infants. JAMA Pediatr. (2015) 169:731–9. doi: 10.1001/jamapediatrics.2015.0510
40. Kribs A, Roll C, Göpel W, Wieg C, Groneck P, Laux R, et al. Nonintubated surfactant application vs conventional therapy in extremely preterm infants. JAMA Pediatr. (2015) 169:723–30. doi: 10.1001/jamapediatrics.2015.0504
41. Jena SR, Bains HS, Pandita A, Verma A, Gupta V, Kallem VR, et al. Surfactant therapy in premature babies: SurE or InSurE. Pediatr Pulmonol. (2019) 54:1747–52. doi: 10.1002/ppul.24479
42. Gupta BK, Saha AK, Mukherjee S, Saha B. Minimally invasive surfactant therapy versus InSurE in preterm neonates of 28 to 34 weeks with respiratory distress syndrome on non-invasive positive pressure ventilation—a randomized controlled trial. Eur J Pediatr. (2020) 179:1287–93. doi: 10.1007/s00431-020-03682-9
43. Kanmaz HG, Erdeve O, Canpolat FE, Mutlu B, Dilmen U. Surfactant administration via thin catheter during spontaneous breathing: randomized controlled trial. Pediatrics. (2013) 131:e502–9. doi: 10.1542/peds.2012-0603
44. Aldana-Aguirre JC, Pinto M, Featherstone RM, Kumar M. Less invasive surfactant administration versus intubation for surfactant delivery in preterm infants with respiratory distress syndrome: a systematic review and meta-analysis. Arch Dis Child Fetal Neonatal Ed. (2017) 102:F17–23. doi: 10.1136/archdischild-2015-310299
45. Abdel-Latif ME, Davis PG, Wheeler KI, de Paoli AG, Dargaville PA. Surfactant therapy via thin catheter in preterm infants with or at risk of respiratory distress syndrome. Cochrane Database Syst Rev. (2021) 5:CD011672. doi: 10.1002/14651858.CD011672.pub2
46. Isayama T, Iwami H, McDonald S, Beyene J. Association of noninvasive ventilation strategies with mortality and bronchopulmonary dysplasia among preterm infants: a systematic review and meta-analysis. JAMA (2016) 316:611–24. doi: 10.1001/jama.2016.10708
47. Dargaville PA, Kamlin COF, Orsini F, Wang X, De Paoli AG, Kanmaz Kutman HG, et al. Effect of minimally invasive surfactant therapy vs sham treatment on death or bronchopulmonary dysplasia in preterm infants with respiratory distress syndrome: the optimist-a randomized clinical trial. JAMA. (2021) 326:2478–87. doi: 10.1001/jama.2021.21892
48. Roberts KD, Brown R, Lampland AL, Leone TA, Rudser KD, Finer NN, et al. Laryngeal mask airway for surfactant administration in neonates: a randomized, controlled trial. J Pediatr. (2018) 193:40-6.e1 doi: 10.1016/j.jpeds.2017.09.068
49. Zhong YY Li JC, Liu YL, Zhao XB, Male M, Song DK, et al. Early intratracheal administration of corticosteroid and pulmonary surfactant for preventing bronchopulmonary dysplasia in preterm infants with neonatal respiratory distress syndrome: a meta-analysis. Curr Med Sci. (2019) 39:493–9. doi: 10.1007/s11596-019-2064-9
50. NICHD. The Budesonide in Babies (BiB) Trial (BiB) Bethesda: Clinicaltrials.gov. (2020). Available online at: https://clinicaltrials.gov/ct2/show/NCT04545866 (accessed on November 3, 2021).
51. Hascoët J-M, Picaud J-C, Ligi I, Blanc T, Moreau F, Pinturier M-F, et al. Late surfactant administration in very preterm neonates with prolonged respiratory distress and pulmonary outcome at 1 year of age. JAMA Pediatr. (2016) 170:365–72. doi: 10.1001/jamapediatrics.2015.4617
52. Ballard RA, Keller RL, Black DM, Ballard PL, Merrill JD, Eichenwald EC, et al. Randomized trial of late surfactant treatment in ventilated preterm infants receiving inhaled nitric oxide. J Pediatr. (2016) 168:23–29.e4. doi: 10.1016/j.jpeds.2015.09.031
53. Keller RL, Eichenwald EC, Hibbs AM, Rogers EE, Wai KC, Black DM, et al. The randomized, controlled trial of late surfactant: effects on respiratory outcomes at 1-year corrected age. J Pediatr. (2017) 183:19–25.e2. doi: 10.1016/j.jpeds.2016.12.059
54. Ardell S, Pfister RH, Soll R. Animal derived surfactant extract versus protein free synthetic surfactant for the prevention and treatment of respiratory distress syndrome. Cochrane Database Syst Rev. (2015) 8:CD000144. doi: 10.1002/14651858.CD000144.pub3
55. Aranda Jv, Gorman W, Bergsteinsson H, Gunn T. Efficacy of caffeine in treatment of apnea in the low-birth-weight infant. J Pediatr. (1977) 90:467–72. doi: 10.1016/s0022-3476(77)80718-x
56. Ribeiro JA, Sebastião AM. Caffeine and adenosine. J Alzheimers Dis. (2010) 20:S3–15. doi: 10.3233/JAD-2010-1379
57. Ferré S. An update on the mechanisms of the psychostimulant effects of caffeine. J Neurochem. (2008) 105:1067–79. doi: 10.1111/j.1471-4159.2007.05196.x
58. Abu-Shaweesh JM, Martin RJ. Caffeine use in the neonatal intensive care unit. Semin Fetal Neonatal Med. (2017) 22:342–7. doi: 10.1016/j.siny.2017.07.011
59. Davis JM, Bhutani VK, Stefano JL, Fox WW, Spitzer AR. Changes in pulmonary mechanics following caffeine administration in infants with bronchopulmonary dysplasia. Pediatr Pulmonol. (1989) 6:49–52. doi: 10.1002/ppul.1950060112
60. Rieg T, Steigele H, Schnermann J, Richter K, Osswald H, Vallon V. Requirement of intact adenosine A1 receptors for the diuretic and natriuretic action of the methylxanthines theophylline and caffeine. J Pharmacol Exp Ther. (2005) 313:403–9. doi: 10.1124/jpet.104.080432
61. Schmidt B, Roberts RS, Davis P, Doyle LW, Barrington KJ, Ohlsson A, et al. Caffeine therapy for apnea of prematurity. N Engl J Med. (2006) 354:2112–21. doi: 10.1056/NEJMoa054065
62. Ferguson KN, Roberts CT, Manley BJ, Davis PG. Interventions to improve rates of successful extubation in preterm infants. JAMA Pediatr. (2017) 171:165–74. doi: 10.1001/jamapediatrics.2016.3015
63. Manku MS, Horrobin DF. Chloroquine, quinine, procaine, quinidine, tricyclic antidepressants, and methylxanthines as prostaglandin agonists and antagonists. Lancet. (1976) 2:1115–7. doi: 10.1016/s0140-6736(76)91090-4
64. Clyman RI, Hills NK, Liebowitz M, Johng S. Relationship between duration of infant exposure to a moderate-to-large patent ductus arteriosus shunt and the risk of developing bronchopulmonary dysplasia or death before 36 weeks. Am J Perinatol. (2020) 37:216–23. doi: 10.1055/s-0039-1697672
65. Weichelt U, Cay R, Schmitz T, Strauss E, Sifringer M, Bührer C, et al. Prevention of hyperoxia-mediated pulmonary inflammation in neonatal rats by caffeine. Eur Respir J. 2(013) 41:966–73. doi: 10.1183/09031936.00012412
66. Nagatomo T, Jiménez J, Richter J, de Baere S, Vanoirbeek J, Naulaers G, et al. Caffeine prevents hyperoxia-induced functional and structural lung damage in preterm rabbits. Neonatology. (2016) 109:274–81. doi: 10.1159/000442937
67. Dumpa V, Nielsen L, Wang H, Kumar VHS. Caffeine is associated with improved alveolarization and angiogenesis in male mice following hyperoxia induced lung injury. BMC Pulm Med. (2019) 19:138. doi: 10.1186/s12890-019-0903-x
68. Endesfelder S, Strauß E, Bendix I, Schmitz T, Bührer C. Prevention of oxygen-induced inflammatory lung injury by caffeine in neonatal rats. Oxid Med Cell Longev. (2020) 2020:3840124. doi: 10.1155/2020/3840124
69. Dayanim S, Lopez B, Maisonet TM, Grewal S, Londhe VA. Caffeine induces alveolar apoptosis in the hyperoxia-exposed developing mouse lung. Pediatr Res. (2014) 75:395–402. doi: 10.1038/pr.2013.233
70. Patel RM, Leong T, Carlton DP, Vyas-Read S. Early caffeine therapy and clinical outcomes in extremely preterm infants. J Perinatol. (2013) 33:134–40. doi: 10.1038/jp.2012.52
71. Kua KP, Lee SWH. Systematic review and meta-analysis of clinical outcomes of early caffeine therapy in preterm neonates. Br J Clin Pharmacol. (2017) 83:180–91. doi: 10.1111/bcp.13089
72. Park HW, Lim G, Chung S-H, Chung S, Kim KS, Kim SN. Early caffeine use in very low birth weight infants and neonatal outcomes: a systematic review and meta-analysis. J Korean Med Sci. (2015) 30:1828–35. doi: 10.3346/jkms.2015.30.12.1828
73. National Guideline Alliance (UK). Specialist neonatal respiratory care for babies born preterm. London: National Institute for Health and Care Excellence (UK). (2019). Available online at: https://www.nice.org.uk/guidance/ng124 [accessed on November 1, 2021]
74. Dekker J, Hooper SB, van Vonderen JJ, Witlox RSGM, Lopriore E, Te Pas AB. Caffeine to improve breathing effort of preterm infants at birth: a randomized controlled trial. Pediatr Res. (2017) 82:290–6. doi: 10.1038/pr.2017.45
75. Dani C, Cecchi A, Remaschi G, Mercadante D. la Marca G, Boni L, et al. Study protocol: treatment with caffeine of the very preterm infant in the delivery room: a feasibility study. BMJ Open. (2020) 10:e040105. doi: 10.1136/bmjopen-2020-040105
76. Chen J, Jin L, Chen X. Efficacy and safety of different maintenance doses of caffeine citrate for treatment of apnea in premature infants: a systematic review and meta-analysis. Biomed Res Int. (2018) 2018:9061234. doi: 10.1155/2018/9061234
77. Vliegenthart R, Miedema M, Hutten GJ, van Kaam AH, Onland W. High versus standard dose caffeine for apnoea: a systematic review. Arch Dis Child Fetal Neonatal Ed. (2018) 103:F523–9. doi: 10.1136/archdischild-2017-313556
78. Brattström P, Russo C, Ley D, Bruschettini M. High-versus low-dose caffeine in preterm infants: a systematic review and meta-analysis. Acta Paediatr. (2019 108:401-10. doi: 10.1111/apa.14586
79. Doyle LW, Ranganathan S, Cheong JLY. Neonatal caffeine treatment and respiratory function at 11 years in children under 1,251 g at birth. Am J Respir Crit Care Med. (2017) 196:1318–24. doi: 10.1164/rccm.201704-0767OC
80. Puia-Dumitrescu M, Smith PB, Zhao J, Soriano A, Payne EH, Harper B, et al. Dosing and safety of off-label use of caffeine citrate in premature infants. J Pediatr. (2019) 211:27–32.e1. doi: 10.1016/j.jpeds.2019.04.028
81. Doyle LW. Postnatal corticosteroids to prevent or treat bronchopulmonary dysplasia. Neonatology. (2021) 118:244–51. doi: 10.1159/000515950
82. Htun ZT, Schulz E, Desai RK, Marasch JL, McPherson CC, Mastrandrea LD, et al. Postnatal steroid management in preterm infants with evolving bronchopulmonary dysplasia. J Perinatol. (2021) 41:1783–96. doi: 10.1038/s41372-021-01083-w
83. Avery GB, Fletcher AB, Kaplan M, Brudno DS. Controlled trial of dexamethasone in respirator-dependent infants with bronchopulmonary dysplasia. Pediatrics. (1985) 75:106–11.
84. Doyle LW, Cheong JL, Ehrenkranz RA, Halliday HL. Late (>7 days) systemic postnatal corticosteroids for prevention of bronchopulmonary dysplasia in preterm infants. Cochrane Database Syst Rev. (2017) 10:CD001145. doi: 10.1002/14651858.CD001145.pub4
85. Doyle LW, Cheong JL, Ehrenkranz RA, Halliday HL. Early (<8 days) systemic postnatal corticosteroids for prevention of bronchopulmonary dysplasia in preterm infants. Cochrane Database Syst Rev. (2017) 10:CD001146. doi: 10.1002/14651858.CD001146.pub5
86. Baud O, Maury L, Lebail F, Ramful D. el Moussawi F, Nicaise C, et al. Effect of early low-dose hydrocortisone on survival without bronchopulmonary dysplasia in extremely preterm infants (PREMILOC): a double-blind, placebo-controlled, multicentre, randomised trial. Lancet. (2016) 387:1827–36. doi: 10.1016/S0140-6736(16)00202-6
87. Doyle LW, Davis PG, Morley CJ, McPhee A, Carlin JB. DART study investigators. Low-dose dexamethasone facilitates extubation among chronically ventilator-dependent infants: a multicenter, international, randomized, controlled trial. Pediatrics. (2006) 117:75–83. doi: 10.1542/peds.2004-2843
88. Doyle LW, Davis PG, Morley CJ, McPhee A, Carlin JB. Outcome at 2 years of age of infants from the dart study: a multicenter, international, randomized, controlled trial of low-dose dexamethasone. Pediatrics. (2007) 119:716–21. doi: 10.1542/peds.2006-2806
89. Harmon HM, Jensen EA, Tan S, Chaudhary AS, Slaughter JL, Bell EF, et al. Timing of postnatal steroids for bronchopulmonary dysplasia: association with pulmonary and neurodevelopmental outcomes. J Perinatol. (2020) 40:616–27. doi: 10.1038/s41372-020-0594-4
90. Onland W, Cools F, Kroon A, Rademaker K, Merkus MP, Dijk PH, et al. Effect of hydrocortisone therapy initiated 7 to 14 days after birth on mortality or bronchopulmonary dysplasia among very preterm infants receiving mechanical ventilation: a randomized clinical trial. JAMA. (2019) 321:354–63. doi: 10.1001/jama.2018.21443
91. Ben Said M, Hays S, Loys C-M, Coletto L, Godbert I, Picaud J-C. Postnatal steroids in extremely low birth weight infants: betamethasone or hydrocortisone? Acta Paediatr. (2013) 102:689–94. doi: 10.1111/apa.12255
92. DeCastro M, El-Khoury N, Parton L, Ballabh P, LaGamma EF. Postnatal betamethasone vs dexamethasone in premature infants with bronchopulmonary dysplasia: a pilot study. J Perinatol. (2009) 29:297–304. doi: 10.1038/jp.2008.194
93. Bhandari A, Schramm CM, Kimble C, Pappagallo M, Hussain N. Effect of a short course of prednisolone in infants with oxygen-dependent bronchopulmonary dysplasia. Pediatrics. (2008) 121:e344–9. doi: 10.1542/peds.2006-3668
94. Linafelter A, Cuna A, Liu C, Quigley A, Truog WE, Sampath V, et al. Extended course of prednisolone in infants with severe bronchopulmonary dysplasia. Early Hum Dev. (2019)136:1-6. doi: 10.1016/j.earlhumdev.2019.06.007
95. Stark AR, Carlo WA, Tyson JE, Papile L-A, Wright LL, Shankaran S, et al. Adverse effects of early dexamethasone treatment in extremely-low-birth-weight infants. N Engl J Med. (2001)344:95-101. doi: 10.1056/NEJM200101113440203
96. Wilson-Costello D, Walsh MC, Langer JC, Guillet R, Laptook AR, Stoll BJ, et al. Impact of postnatal corticosteroid use on neurodevelopment at 18 to 22 months' adjusted age: effects of dose, timing, and risk of bronchopulmonary dysplasia in extremely low birth weight infants. Pediatrics. (2009)123:e430–7. doi: 10.1542/peds.2008-1928
97. Qin G, Lo JW, Marlow N, Calvert SA, Greenough A, Peacock JL. Postnatal dexamethasone, respiratory and neurodevelopmental outcomes at two years in babies born extremely preterm. PLoS ONE. (2017) 12:e0181176. doi: 10.1371/journal.pone.0181176
98. Doyle LW, Halliday HL, Ehrenkranz RA, Davis PG, Sinclair JC. An Update on the impact of postnatal systemic corticosteroids on mortality and cerebral palsy in preterm infants: effect modification by risk of bronchopulmonary dysplasia. J Pediatr. (2014) 165:1258–60. doi: 10.1016/j.jpeds.2014.07.049
99. Powell K, Kerkering KW, Barker G, Rozycki HJ. Dexamethasone dosing, mechanical ventilation and the risk of cerebral palsy. J Matern Fetal Neonatal Med. (2006) 19:43–8. doi: 10.1080/14767050500363519
100. Watterberg KL KL & American Academy of Pediatrics. Committee on Fetus and Newborn (2010). Policy statement–postnatal corticosteroids to prevent or treat bronchopulmonary dysplasia. Pediatrics. 126:800–8. doi: 10.1542/peds.2010-1534
101. Stark AR, Eichenwald EC. Prevention of bronchopulmonary dysplasia: Postnatal use of corticosteroids. In UptoDate (2021). Available online at: https://www.uptodate.com/contents/prevention-of-bronchopulmonary-dysplasia-postnatal-use-of-corticosteroids (accessed January 4, 2022).
102. Bassler D, Plavka R, Shinwell ES, Hallman M, Jarreau P-H, Carnielli V, et al. Early inhaled budesonide for the prevention of bronchopulmonary dysplasia. N Engl J Med. (2015) 373:1497–506. doi: 10.1056/NEJMoa1501917
103. Yuksel B, Greenough A. Randomised trial of inhaled steroids in preterm infants with respiratory symptoms at follow up. Thorax. (1992) 47:910–3. doi: 10.1136/thx.47.11.910
104. Dugas M-A, Nguyen D, Frenette L, Lachance C, St-Onge O, Fougères A, et al. Fluticasone inhalation in moderate cases of bronchopulmonary dysplasia. Pediatrics. (2005) 115:e566–72. doi: 10.1542/peds.2004-0951
105. Beresford MW. Randomised double blind placebo controlled trial of inhaled fluticasone propionate in infants with chronic lung disease. Arch Dis Child Fetal Neonatal Ed. (2002) 87:F62–3. doi: 10.1136/fn.87.1.f62
106. Onland W, Offringa M, van Kaam A. Late (≥ 7 days) inhalation corticosteroids to reduce bronchopulmonary dysplasia in preterm infants. Cochrane Database Syst Rev. (2017) 8:CD002311. doi: 10.1002/14651858.CD002311.pub4
107. Shah SS, Ohlsson A, Halliday HL, Shah VS. Inhaled versus systemic corticosteroids for the treatment of bronchopulmonary dysplasia in ventilated very low birth weight preterm infants. Cochrane Database Syst Rev. (2017) 10:CD002057. doi: 10.1002/14651858.CD002057.pub4
108. Laughon MM, Langer JC, Bose CL, et al. Prediction of bronchopulmonary dysplasia by postnatal age in extremely premature infants. Am J Respir Crit Care Med. (2011) 183:1715–22. doi: 10.1164/rccm.201101-0055OC
109. Gay JH, Daily WJR, Meyer BHP, Trump DS, Cloud DT, Molthan ME. Ligation of the patent ductus arteriosus in premature infants: Report of 45 cases. J Pediatr Surg. (1973) 8:677–83. doi: 10.1016/0022-3468(73)90406-5
110. Willis KA, Weems MF. Hemodynamically significant patent ductus arteriosus and the development of bronchopulmonary dysplasia. Congenit Heart Dis. (2019) 14:27–32. doi: 10.1111/chd.12691
111. Gersony WM, Peckham GJ, Ellison RC, Miettinen OS, Nadas AS. Effects of indomethacin in premature infants with patent ductus arteriosus: Results of a national collaborative study. J Pediatr. (1983) 102:895–906. doi: 10.1016/s0022-3476(83)80022-5
112. Fowlie PW, Davis PG, McGuire W. Prophylactic intravenous indomethacin for preventing mortality and morbidity in preterm infants. Cochrane Database Syst Rev. (2010) 2010:CD000174. doi: 10.1002/14651858.CD000174.pub2
113. Jensen EA, Dysart KC, Gantz MG, Carper B, Higgins RD, Keszler M, et al. Association between use of prophylactic indomethacin and the risk for bronchopulmonary dysplasia in extremely preterm infants. J Pediatr. (2017) 186:34–40.e2. doi: 10.1016/j.jpeds.2017.02.003
114. Liebowitz M, Clyman RI. Prophylactic indomethacin compared with delayed conservative management of the patent ductus arteriosus in extremely preterm infants: effects on neonatal outcomes. J Pediatr. (2017) 187:119–26.e1. doi: 10.1016/j.jpeds.2017.03.021
115. Pan I, Shah PA, Singh J, Kelly KN, Bondi DS. Comparison of neonatal outcomes with and without prophylaxis with indomethacin in premature neonates. J Pediatr Pharmacol Ther. (2021) 26:478–83. doi: 10.5863/1551-6776-26.5.478
116. Ohlsson A, Walia R, Shah SS. Ibuprofen for the treatment of patent ductus arteriosus in preterm or low birth weight (or both) infants. Cochrane Database Syst Rev. (2020) 2:CD003481. doi: 10.1002/14651858.CD003481.pub8
117. Aranda J, Clyman R, Cox B, van Overmeire B, Wozniak P, Sosenko I, et al. A randomized, double-blind, placebo-controlled trial on intravenous ibuprofen l-lysine for the early closure of nonsymptomatic patent ductus arteriosus within 72 hours of birth in extremely low-birth-weight infants. Am J Perinatol. (2009) 26:235–45. doi: 10.1055/s-0028-1103515
118. Bell JL, Gupta S, Juszczak E, Hardy P, Linsell L. Baby-OSCAR: Outcome after selective early treatment for closure of patent ductus arteriosus in preterm babies—a statistical analysis plan for short-term outcomes. Trials. (2021) 22:368. doi: 10.1186/s13063-021-05324-3102
119. Hundscheid T, Onland W, van Overmeire B, Dijk P, van Kaam AHLC, Dijkman KP, et al. Early treatment versus expectative management of patent ductus arteriosus in preterm infants: a multicentre, randomised, non-inferiority trial in Europe (BeNeDuctus trial). BMC Pediatr. (2018) 18:262. doi: 10.1186/s12887-018-1215-7
120. Paquette L, Friedlich P, Ramanathan R, Seri I. Concurrent use of indomethacin and dexamethasone increases the risk of spontaneous intestinal perforation in very low birth weight neonates. J Perinatol. (2006) 26:486–92. doi: 10.1038/sj.jp.7211548
121. Hustead VA, Gutcher GR, Anderson SA, Zachman RD. Relationship of vitamin A (retinol) status to lung disease in the preterm infant. J Pediatr. (1984) 105:610–5. doi: 10.1016/s0022-3476(84)80432-1
122. Shenai JP, Kennedy KA, Chytil F, Stahlman MT. Clinical trial of vitamin A supplementation in infants susceptible to bronchopulmonary dysplasia. J Pediatr. (1987) 111:269–77. doi: 10.1016/s0022-3476(87)80086-0
123. Pearson E, Bose C, Snidow T, Ransom L, Young T, Bose G, et al. Trial of vitamin A supplementation in very low birth weight infants at risk for bronchopulmonary dysplasia. J Pediatr. (1992) 121:420–7. doi: 10.1016/s0022-3476(05)81800-1
124. Kennedy KA, Stoll BJ, Ehrenkranz RA, Oh W, Wright LL, Stevenson DK, et al. Vitamin A to prevent bronchopulmonary dysplasia in very-low-birth-weight infants: has the dose been too low? Early Hum Dev. (1997) 49:19–31. doi: 10.1016/s0378-3782(97)01869-0
125. Tyson JE, Wright LL, Oh W, Kennedy KA, Mele L, Ehrenkranz RA, et al. Vitamin A supplementation for extremely-low-birth-weight infants. N Engl J Med. (1999) 340:1962–8. doi: 10.1056/NEJM199906243402505
126. Hind M, Maden M. Retinoic acid induces alveolar regeneration in the adult mouse lung. Eur Respir J. (2004) 23:20–7. doi: 10.1183/09031936.03.00119103
127. Ozer EA, Kumral A, Ozer E, Duman N, Yilmaz O, Ozkal S, et al. Effect of retinoic acid on oxygen-induced lung injury in the newborn rat. Pediatr Pulmonol. (2005) 39:35–40. doi: 10.1002/ppul.20131
128. Song Y, Dahl M, Leavitt W, Alvord J, Bradford CY, Albertine KH, et al. Vitamin A protects the preterm lamb diaphragm against adverse effects of mechanical ventilation. Front Physiol. (2018) 9:1119. doi: 10.3389/fphys.2018.01119
129. Darlow BA, Graham PJ, Rojas-Reyes MX. Vitamin A supplementation to prevent mortality and short- and long-term morbidity in very low birth weight infants. Cochrane Database Syst Rev. (2016) 2016:CD000501. doi: 10.1002/14651858.CD000501.pub4
130. Ding Y, Chen Z, Lu Y. Vitamin A supplementation prevents the bronchopulmonary dysplasia in premature infants. Medicine (Baltimore). (2021) 100:e23101. doi: 10.1097/MD.0000000000023101
131. Rysavy MA, Li L, Tyson JE, Jensen EA, Das A, Ambalavanan N, et al. Should vitamin A injections to prevent bronchopulmonary dysplasia or death be reserved for high-risk infants? reanalysis of the national institute of child health and human development neonatal research network randomized trial. J Pediatr. (2021) S0022-3476(21)00445-5. doi: 10.1016/j.jpeds.2021.05.022
132. Wardle SP, Hughes A, Chen S, Shaw NJ. Randomised controlled trial of oral vitamin A supplementation in preterm infants to prevent chronic lung disease. Arch Dis Child Fetal Neonatal Ed. (2001) 84:F9–13. doi: 10.1136/fn.84.1.f9
133. Rakshasbhuvankar A, Patole S, Simmer K, Pillow JJ. Enteral vitamin A for reducing severity of bronchopulmonary dysplasia in extremely preterm infants: a randomised controlled trial. BMC Pediatr. (2017) 17:204. doi: 10.1186/s12887-017-0958-x
134. Basu S, Khanna P, Srivastava R, Kumar A. Oral vitamin A supplementation in very low birth weight neonates: a randomized controlled trial. Eur J Pediatr. (2019) 178:1255–65. doi: 10.1007/s00431-019-03412-w
135. Meyer S, Gortner L. NeoVitaA trial investigators. Early postnatal additional high-dose oral vitamin A supplementation versus placebo for 28 days for preventing bronchopulmonary dysplasia or death in extremely low birth weight infants. Neonatology. (2014) 105:182–8. doi: 10.1159/000357212
136. Gelfand CA, Sakurai R, Wang Y, Liu Y, Segal R, Rehan VK. Inhaled vitamin A is more effective than intramuscular dosing in mitigating hyperoxia-induced lung injury in a neonatal rat model of bronchopulmonary dysplasia. Am J Physiol Lung Cell Mol Physiol. (2020) 319:L576–84. doi: 10.1152/ajplung.00266.2020
137. Wahl HB, Hütten MC, Monz D, Tutdibi E, Ophelders D, Nikiforou M, et al. Vitamin A supplementation by endotracheal application of a nano-encapsulated preparation is feasible in ventilated preterm lambs. J Aerosol Med Pulm Drug Deliv. (2018) 31:323–30. doi: 10.1089/jamp.2017.1438
138. Sniderman S, Clyman R, Chung M, Roth R, Ballard R. 1432 Treatment of neonatal chronic lung disease with furosemide. Pediatr Res. (1981) 15:682. doi: 10.1203/00006450-198104001-01461
139. Kao LC, Warburton D, Sargent CW, Platzker ACG, Keens TG. Furosemide acutely decreases airways resistance in chronic bronchopulmonary dysplasia. J Pediatr. (1983) 103:624–9. doi: 10.1016/s0022-3476(83)80602-7
140. Najak ZD, Harris EM, Lazzara A, Pruitt AW. Pulmonary effects of furosemide in preterm infants with lung disease. J Pediatr. (1983) 102:758–63. doi: 10.1016/s0022-3476(83)80253-4
141. Patel H, Yeh TF, Jain R, Pildes R. Pulmonary and renal responses to furosemide in infants with stage III-IV bronchopulmonary dysplasia. Am J Dis Child. (1985) 139:917–9. doi: 10.1001/archpedi.1985.02140110071032
142. McCann EM, Lewis K, Deming DD, Donovan MJ, Brady JP. Controlled trial of furosemide therapy in infants with chronic lung disease. J Pediatr. (1985) 106:957–62. doi: 10.1016/s0022-3476(85)80252-3
143. Rush MG, Engelhardt B, Parker RA, Hazinski TA. Double-blind, placebo-controlled trial of alternate-day furosemide therapy in infants with chronic bronchopulmonary dysplasia. J Pediatr. (1990) 117:112–8. doi: 10.1016/s0022-3476(05)82458-8
144. Stewart A, Brion LP. Intravenous or enteral loop diuretics for preterm infants with (or developing) chronic lung disease. Cochrane Database Syst Rev. (2011) 2011:CD001453. doi: 10.1002/14651858.CD001453.pub2
145. Kao LC, Warburton D, Cheng MH, Cedeño C, Platzker AC, Keens TG. Effect of oral diuretics on pulmonary mechanics in infants with chronic bronchopulmonary dysplasia: results of a double-blind crossover sequential trial. Pediatrics. (1984) 74:37–44.
146. Engelhardt B, Blalock WA, DonLevy S, Rush M, Hazinski TA. Effect of spironolactone-hydrochlorothiazide on lung function in infants with chronic bronchopulmonary dysplasia. J Pediatr. (1989) 114:619–24. doi: 10.1016/s0022-3476(89)80708-5
147. Kao LC, Durand DJ, McCrea RC, Birch M, Powers RJ, Nickerson BG. Randomized trial of long-term diuretic therapy for infants with oxygen-dependent bronchopulmonary dysplasia. J Pediatr. (1994) 124:772–81. doi: 10.1016/s0022-3476(05)81373-3
148. Stewart A, Brion LP, Ambrosio-Perez I. Diuretics acting on the distal renal tubule for preterm infants with (or developing) chronic lung disease. Cochrane Database Syst Rev. (2011) 2011:CD001817. doi: 10.1002/14651858.CD001817.pub2
149. Rastogi A, Luayon M, Ajayi OA, Pildes RS. Nebulized furosemide in infants with bronchopulmonary dysplasia. J Pediatr. (1994) 125:976–9. doi: 10.1016/s0022-3476(05)82018-9
150. Prabhu VG, Keszler M, Dhanireddy R. Dose-dependent evaluation of the effects of nebulized furosemide on pulmonary function in ventilated preterm infants. J Perinatol. (1998) 18:357–60.
151. Brion LP, Primhak RA, Yong W. Aerosolized diuretics for preterm infants with (or developing) chronic lung disease. Cochrane Database Syst Rev. (2006) 2006:CD001694. doi: 10.1002/14651858.CD001694.pub2
152. Greenberg RG, Gayam S, Savage D, Tong A, Gorham D, Sholomon A, et al. Furosemide exposure and prevention of bronchopulmonary dysplasia in premature infants. J Pediatr. (2019) 208:134-40.e2. doi: 10.1016/j.jpeds.2018.11.043
153. Bamat NA, Nelin TD, Eichenwald EC, Kirpalani H, Laughon MM, Jackson WM, et al. Loop diuretics in severe bronchopulmonary dysplasia: cumulative use and associations with mortality and age at discharge. J Pediatr. (2021) 231:43-9.e3. doi: 10.1016/j.jpeds.2020.10.073
154. Laughon M, Chantala K, Aliaga S, Herring A, Hornik C, Hughes R, et al. Diuretic Exposure in Premature Infants from 1997 to 2011. Am J Perinatol. (2015) 32:49–56. doi: 10.1055/s-0034-1373845
155. Slaughter JL, Stenger MR, Reagan PB. Variation in the use of diuretic therapy for infants with bronchopulmonary dysplasia. Pediatrics. (2013) 131:716–23. doi: 10.1542/peds.2012-1835
156. Bhandari A, Chow U, Hagadorn J. Variability in duration of outpatient diuretic therapy in bronchopulmonary dysplasia: a clinical experience. Am J Perinatol. (2010) 27:529–35. doi: 10.1055/s-0030-1248939
157. Kalikkot Thekkeveedu R, Guaman MC, Shivanna B. Bronchopulmonary dysplasia: A review of pathogenesis and pathophysiology. Respir Med. (2017) 132:170–7. doi: 10.1016/j.rmed.2017.10.014
158. Kao LC, Warburton D, Platzker AC, Keens TG. Effect of isoproterenol inhalation on airway resistance in chronic bronchopulmonary dysplasia. Pediatrics. (1984) 73:509–14.
159. Logvinoff MM, Lemen RJ, Taussig LM, Lamont BA. Bronchodilators and diuretics in children with bronchopulmonary dysplasia. Pediatr Pulmonol. (1985) 1:198–203. doi: 10.1002/ppul.1950010406
160. Sosulski R, Abbasi S, Bhutani VK, Fox WW. Physiologic effects of terbutaline on pulmonary function of infants with bronchopulmonary dysplasia. Pediatr Pulmonol. (1986) 2:269–73. doi: 10.1002/ppul.1950020504
161. Kao LC, Durand DJ, Nickerson BG. Effects of inhaled metaproterenol and atropine on the pulmonary mechanics of infants with bronchopulmonary dysplasia. Pediatr Pulmonol. (1989) 6:74–80. doi: 10.1002/ppul.1950060204
162. Rotschild A, Solimano A, Puterman M, Smyth J, Sharma A, Albersheim S. Increased compliance in response to salbutamol in premature infants with developing bronchopulmonary dysplasia. J Pediatr. (1989) 115:984–91. doi: 10.1016/s0022-3476(89)80755-3
163. Brundage KL, Mohsini KG, Froese AB, Fisher JT. Bronchodilator response to ipratropium bromide in infants with bronchopulmonary dysplasia. Am Rev Respir Dis. (1990) 142:1137–42. doi: 10.1164/ajrccm/142.5.1137
164. Denjean A, Paris-Llado J, Zupan V, Debillon T, Kieffer F, Magny J-F, et al. Inhaled salbutamol and beclomethasone for preventing broncho-pulmonary dysplasia: a randomised double-blind study. Eur J Pediatr. (1998) 157:926–31. doi: 10.1007/s004310050969
165. Kotecha SJ, Edwards MO, Watkins WJ, Lowe J, Henderson AJ, Kotecha S. Effect of bronchodilators on forced expiratory volume in 1 s in preterm-born participants aged 5 and over: a systematic review. Neonatology. (2015) 107:31–240. doi: 10.1159/000371539
166. Slaughter JL, Stenger MR, Reagan PB, Jadcherla SR. Inhaled bronchodilator use for infants with bronchopulmonary dysplasia. J Perinatol. (2015) 35:61–6. doi: 10.1038/jp.2014.141
167. Ng G, da Silva O, Ohlsson A. Bronchodilators for the prevention and treatment of chronic lung disease in preterm infants. Cochrane Database Syst Rev. (2016) 12:CD003214. doi: 10.1002/14651858.CD003214.pub3
168. Clouse BJ, Jadcherla SR, Slaughter JL. Systematic review of inhaled bronchodilator and corticosteroid therapies in infants with bronchopulmonary dysplasia: implications and future directions. PLoS ONE. (2016) 11:e0148188. doi: 10.1371/journal.pone.0148188
169. Koch A, Kreutzer KB, Poets C, Engel C, Bassler D. The impact of inhaled bronchodilators on bronchopulmonary dysplasia: a nonrandomized comparison from the NEuroSIS trial. J Matern Fetal Neonatal Med. (2019) 1–3. doi: 10.1080/14767058.2019.1590331
170. Clark RH. Reported medication use in the neonatal intensive care unit: data from a large national data set. Pediatrics. (2006) 117:1979–87. doi: 10.1542/peds.2005-1707
171. Fakhoury KF, Sellers C, Smith EO, Rama JA, Fan LL. Serial measurements of lung function in a cohort of young children with bronchopulmonary dysplasia. Pediatrics. (2010) 125:e1441–7. doi: 10.1542/peds.2009-0668
172. Morrow DK, Schilling D, McEvoy C. Response to bronchodilators in very preterm infants with evolving bronchopulmonary dysplasia. Res Rep Neonatol. (2015) 5:113–7. doi: 10.2147/RRN.S96961
173. Euteneuer JC, Kerns E, Leiting C, McCulloh RJ, Peeples ES. Inhaled bronchodilator exposure in the management of bronchopulmonary dysplasia in hospitalized infants. J Perinatol. (2021) 41:53–61. doi: 10.1038/s41372-020-0760-8
174. Fok TF, Monkman S, Dolovich M, Gray S, Coates G, Paes B, et al. Efficiency of aerosol medication delivery from a metered dose inhaler versus jet nebulizer in infants with bronchopulmonary dysplasia. Pediatr Pulmonol. (1996) 21:301–9. doi: 10.1002/(SICI)1099-0496(199605)21:5<301::AID-PPUL5>3.0.CO;2-P
175. Yuksel B, Greenough A. Comparison of the effects on lung function of two methods of bronchodilator administration. Respir Med. (1994) 88:229–33. doi: 10.1016/s0954-6111(05)80351-8
176. Allen J, Zwerdling R, Ehrenkranz R, Gaultier C, Geggel R, Greenough A, et al. Statement on the care of the child with chronic lung disease of infancy and childhood. Am J Respir Crit Care Med. (2003) 168:356–96. doi: 10.1164/rccm.168.3.356
177. Panitch HB, Keklikian EN, Motley RA, Wolfson MR, Schidlow D. Effect of altering smooth muscle tone on maximal expiratory flows in patients with tracheomalacia. Pediatr Pulmonol. (1990) 9:170–6. doi: 10.1002/ppul.1950090309
178. Papoff P, Cerasaro C, Caresta E, Barbàra CS, Midulla F, Moretti C. Current strategies for treating infants with severe bronchopulmonary dysplasia. J Matern Fetal Neonatal Med. (2012) 25:15–20. doi: 10.3109/14767058.2012.712352
179. Barnett CF, Machado RF. Sildenafil in the treatment of pulmonary hypertension. Vasc Health Risk Manag. (2006) 2:411–22. doi: 10.2147/vhrm.2006.2.4.411
180. Berkelhamer SK, Mestan KK, Steinhorn RH. Pulmonary hypertension in bronchopulmonary dysplasia. Semin Perinatol. (2013) 37:124–31. doi: 10.1053/j.semperi.2013.01.009
181. Berger S, Konduri GG. Pulmonary hypertension in children: the twenty-first century. Pediatr Clin North Am. (2006) 53:961-x. doi: 10.1016/j.pcl.2006.08.001
182. Lima B, Forrester MT, Hess DT, Stamler JS. S -Nitrosylation in cardiovascular signaling. Circ Res. (2010) 106:633–46. doi: 10.1161/CIRCRESAHA.109.207381
183. Afshar S, Gibson LL, Yuhanna IS, Sherman TS, Kerecman JD, Grubb PH, et al. Pulmonary NO synthase expression is attenuated in a fetal baboon model of chronic lung disease. Am J Physiol Lung Cell Mol Physiol. (2003) 284:L749–58. doi: 10.1152/ajplung.00334.2002
184. Ballard PL, Gonzales LW, Godinez RI, Godinez MH, Savani RC, McCurnin DC, et al. Surfactant composition and function in a primate model of infant chronic lung disease: effects of inhaled nitric oxide. Pediatr Res. (2006) 59:157–62. doi: 10.1203/01.pdr.0000190664.69081.f1
185. ter Horst SAJ, Walther FJ, Poorthuis BJHM, Hiemstra PS, Wagenaar GTM. Inhaled nitric oxide attenuates pulmonary inflammation and fibrin deposition and prolongs survival in neonatal hyperoxic lung injury. Am J Physiol Lung Cell Mol Physiol. (2007) 293:L35–44. doi: 10.1152/ajplung.00381.2006
186. Tang J-R, Seedorf G, Balasubramaniam V, Maxey A, Markham N, Abman SH. Early inhaled nitric oxide treatment decreases apoptosis of endothelial cells in neonatal rat lungs after vascular endothelial growth factor inhibition. Am J Physiol Lung Cell Mol Physiol. (2007) 293:L1271–80. doi: 10.1152/ajplung.00224.2007
187. Abman SH. Monitoring cardiovascular function in infants with chronic lung disease of prematurity. Arch Dis Child Fetal Neonatal Ed. (2002) 87:F15–8. doi: 10.1136/fn.87.1.f15
188. Abman SH, Griebel JL, Parker DK, Schmidt JM, Swanton D, Kinsella JP. Acute effects of inhaled nitric oxide in children with severe hypoxemic respiratory failure. J Pediatr. (1994) 124:881–8. doi: 10.1016/s0022-3476(05)83175-0
189. Banks BA, Seri I, Ischiropoulos H, Merrill J, Rychik J, Ballard RA. Changes in oxygenation with inhaled nitric oxide in severe bronchopulmonary dysplasia. Pediatrics. (1999) 103:610–8. doi: 10.1542/peds.103.3.610
190. Mupanemunda R, Siveiman M. 169 Low-Dose Inhaled nitric oxide (NO) in infants with bronchopulmonary dysplasia (BPD). Pediatr Res. (1994) 36:1. doi: 10.1203/00006450-199407000-00169
191. Barrington KJ, Finer N, Pennaforte T. Inhaled nitric oxide for respiratory failure in preterm infants. Cochrane Database Syst Rev. (2017) 1:CD000509. doi: 10.1002/14651858.CD000509.pub5
192. Schreiber MD, Gin-Mestan K, Marks JD, Huo D, Lee G, Srisuparp P. Inhaled nitric oxide in premature infants with the respiratory distress syndrome. N Engl J Med. (2003) 349:2099–107. doi: 10.1056/NEJMoa031154
193. Kinsella JP, Cutter GR, Walsh WF, Gerstmann DR, Bose CL, Hart C, et al. Early inhaled nitric oxide therapy in premature newborns with respiratory failure. N Engl J Med. (2006) 355:354–64. doi: 10.1056/NEJMoa060442
194. Mercier J-C, Hummler H, Durrmeyer X, Sanchez-Luna M, Carnielli V, Field D, et al. Inhaled nitric oxide for prevention of bronchopulmonary dysplasia in premature babies (EUNO): a randomised controlled trial. Lancet. (2010) 376:346–54. doi: 10.1016/S0140-6736(10)60664-2
195. Kinsella JP, Cutter GR, Steinhorn RH, Nelin LD, Walsh WF, Finer NN, et al. Noninvasive inhaled nitric oxide does not prevent bronchopulmonary dysplasia in premature newborns. J Pediatr. (2014) 165:1104–8.e1. doi: 10.1016/j.jpeds.2014.06.018
196. Hasan SU, Potenziano J, Konduri GG, Perez JA, van Meurs KP, Walker MW, et al. Effect of inhaled nitric oxide on survival without bronchopulmonary dysplasia in preterm infants. JAMA Pediatr. (2017) 171:1081–9. doi: 10.1001/jamapediatrics.2017.2618
197. Ballard RA, Truog WE, Cnaan A, Martin RJ, Ballard PL, Merrill JD, et al. Inhaled nitric oxide in preterm infants undergoing mechanical ventilation. N Engl J Med. (2006) 355:343–53. doi: 10.1056/NEJMoa061088
198. Askie LM, Davies LC, Schreiber MD, Hibbs AM, Ballard PL, Ballard RA. Race effects of inhaled nitric oxide in preterm infants: an individual participant data meta-analysis. J Pediatr. (2018) 193:34–9.e2. doi: 10.1016/j.jpeds.2017.10.004
199. Hibbs AM, Walsh MC, Martin RJ, Truog WE, Lorch SA, Alessandrini E, et al. One-year respiratory outcomes of preterm infants enrolled in the nitric oxide (to prevent) chronic lung disease trial. J Pediatr. (2008) 153:525–9. doi: 10.1016/j.jpeds.2008.04.033
200. Greenough A, Decobert F, Field D, Hallman M, Hummler HD, Jonsson B, et al. Inhaled nitric oxide (iNO) for preventing prematurity-related bronchopulmonary dysplasia (BPD): 7-year follow-up of the European Union Nitric Oxide (EUNO) trial. J Perinat Med. (2020) 49:104–10. doi: 10.1515/jpm-2020-0164
201. Cole FS, Alleyne C, Barks JD, Boyle RJ, Carroll JL, Dokken D, et al. NIH consensus development conference statement: inhaled nitric-oxide therapy for premature infants. Pediatrics. (2011) 127:363–9. doi: 10.1542/peds.2010-3507
202. Thompson E, Perez K, Hornik C, Smith P, Clark R, Laughon M. Sildenafil exposure in the neonatal intensive care unit. Am J Perinatol. (2019) 36:262–7. doi: 10.1055/s-0038-1667378
203. Hon KE, Cheung K, Siu K, Leung T, Yam M, Fok T, et al. Oral sildenafil for treatment of severe pulmonary hypertension in an infant. Biol Neonate. (2005) 88:109–12. doi: 10.1159/000085646
204. van der Graaf M, Rojer LA, Helbing WA, Reiss IKM, Etnel JRG, Bartelds B. Sildenafil for bronchopulmonary dysplasia and pulmonary hypertension: a meta-analysis. Pulm Circ. (2019) 9:2045894019837875. doi: 10.1177/2045894019837875
205. van Heugten M, van Onzenoort-Bokken L. Sildenafil citrate overdose in a month postterm premature infant with pulmonary artery hypertension. Pediatr Emerg Care. (202) 10.1097/PEC.0000000000002472. doi: 10.1097/PEC.0000000000002472
206. Jackson W, Gonzalez D, Smith PB, Ambalavanan N, Atz AM, Sokol GM et al. Safety of sildenafil in extremely premature infants: a phase I trial. J perinatal. (2022) 42:31–6. doi: 10.1038/s41372-021-01261-w
207. Schneider S, Bailey M, Spears T, Esther CR, Laughon MM, Hornik CP, et al. Safety of sildenafil in premature infants with severe bronchopulmonary dysplasia (SILDI-SAFE): a multicenter, randomized, placebo-controlled, sequential dose-escalating, double-masked, safety study. BMC Pediatr. (2020) 20:559. doi: 10.1186/s12887-020-02453-7
208. Ladha F, Bonnet S, Eaton F, Hashimoto K, Korbutt G, Thébaud B. Sildenafil improves alveolar growth and pulmonary hypertension in hyperoxia-induced lung injury. Am J Respir Crit Care Med. (2005) 172:750–6. doi: 10.1164/rccm.200503-510OC
209. de Visser YP, Walther FJ, Laghmani EH, Boersma H, van der Laarse A, Wagenaar GT. Sildenafil attenuates pulmonary inflammation and fibrin deposition, mortality and right ventricular hypertrophy in neonatal hyperoxic lung injury. Respir Res. (2009) 10:30. doi: 10.1186/1465-9921-10-30
210. Park H-S, Park J-W, Kim H-J, Choi CW, Lee H-J, Kim B, et al. Sildenafil alleviates bronchopulmonary dysplasia in neonatal rats by activating the hypoxia-inducible factor signaling pathway. Am J Respir Cell Mol Biol. (2013) 48:105–13. doi: 10.1165/rcmb.2012-0043OC
211. Abounahia FF, Abu-Jarir R, Abounahia MF, Al-Badriyeh D, Abushanab D, Abu-Ghalwa M, et al. Prophylactic sildenafil in preterm infants at risk of bronchopulmonary dysplasia: a pilot randomized, double-blinded, placebo-controlled trial. Clin Drug Investig. (2019) 39:1093–107. doi: 10.1007/s40261-019-00834-0
212. König K, Barfield CP, Guy KJ, Drew SM, Andersen CC. The effect of sildenafil on evolving bronchopulmonary dysplasia in extremely preterm infants: a randomised controlled pilot study. J Matern Fetal Neonatal Med. (2014) 27:439–44. doi: 10.3109/14767058.2013.818650
213. Giaid A, Yanagisawa M, Langleben D, Michel RP, Levy R, Shennib H, et al. Expression of Endothelin-1 in the Lungs of Patients with Pulmonary Hypertension. N Engl J Med. (1993) 328:1732–9. doi: 10.1056/NEJM199306173282402
214. Rosenzweig EB, Ivy DD, Widlitz A, Doran A, Claussen LR, Yung D, et al. Effects of long-term bosentan in children with pulmonary arterial hypertension. J Am Coll Cardiol. (2005) 46:697–704. doi: 10.1016/j.jacc.2005.01.066
216. Krishnan U, Krishnan S, Gewitz M. Treatment of pulmonary hypertension in children with chronic lung disease with newer oral therapies. Pediatr Cardiol. (2008) 29:1082–6. doi: 10.1007/s00246-008-9260-x
217. Kadmon G, Schiller O, Dagan T, Bruckheimer E, Birk E, Schonfeld T. Pulmonary hypertension specific treatment in infants with bronchopulmonary dysplasia. Pediatr Pulmonol. (2017) 52:77–83. doi: 10.1002/ppul.23508
218. Nees SN, Rosenzweig EB, Cohen JL, Valencia Villeda GA, Krishnan US. Targeted therapy for pulmonary hypertension in premature infants. Children (Basel). (2020) 7:97. doi: 10.3390/children7080097
219. Özdemir ÖM, Taban Ö, Enli Y, Bir F, Sahin B, Ergin H. The effects of bosentan on hyperoxia-induced lung injury in neonatal rats. Pediatr Int. (2019) 61:1120–6. doi: 10.1111/ped.14013
220. Tracleer. (2021). Available online at: https://www.janssenlabels.com/package-insert/product-monograph/prescribing-information/TRACLEER-pi.pdf (accessed October 5, 2021).
221. Wang EEL, Ohlsson A, Kellner JD. Association of Ureaplasma urealyticum colonization with chronic lung disease of prematurity: Results of a metaanalysis. J Pediatr. (1995) 127:640–4. doi: 10.1016/s0022-3476(95)70130-3
222. Schelonka RL, Katz B, Waites KB, Benjamin DK. Critical appraisal of the role of ureaplasma in the development of bronchopulmonary dysplasia with metaanalytic techniques. Pediatr Infect Dis J. (2005) 24:1033–9. doi: 10.1097/01.inf.0000190632.31565.83
223. Viscardi RM, Kallapur SG. Role of ureaplasma respiratory tract colonization in bronchopulmonary dysplasia pathogenesis. Clin Perinatol. (2015) 42:719–38. doi: 10.1016/j.clp.2015.08.003
224. Ianaro A, Ialenti A, Maffia P, Sautebin L, Rombolà L, Carnuccio R, et al. Anti-inflammatory activity of macrolide antibiotics. Anti-inflammatory activity of macrolide antibiotics. J Pharmacol Exp Ther. (2000) 292:156–63.
225. Culić O, Eraković V, Cepelak I, Barišić K, Brajša K, Ferenčić Ž, et al. Azithromycin modulates neutrophil function and circulating inflammatory mediators in healthy human subjects. Eur J Pharmacol. (2002) 450:277–89. doi: 10.1016/s0014-2999(02)02042-3
226. Jónsson B, Rylander M, Faxelius G. Ureaplasma urealyticum, erythromycin and respiratory morbidity in high-risk preterm neonates. Acta Paediatr. (1998) 87:1079–84. doi: 10.1080/080352598750031428
227. Lyon AJ, McColm J, Middlemist L, Fergusson S, McIntosh N, Ross PW. Randomised trial of erythromycin on the development of chronic lung disease in preterm infants. Arch Dis Child Fetal Neonatal Ed. (1998) 78:F10–4. doi: 10.1136/fn.78.1.f10
228. Baier RJ, Loggins J, Kruger TE. Failure of erythromycin to eliminate airway colonization with ureaplasma urealyticumin very low birth weight infants. BMC Pediatr. (2003) 3:10. doi: 10.1186/1471-2431-3-10
229. Ballard HO, Anstead MI, Shook LA. Azithromycin in the extremely low birth weight infant for the prevention of Bronchopulmonary Dysplasia: a pilot study. Respir Res. (2007) 8:41. doi: 10.1186/1465-9921-8-41
230. Matlow A. Th'ng C, Kovach D, Quinn P, Dunn M, Wang E. Susceptibilities of neonatal respiratory isolates of ureaplasma urealyticum to antimicrobial agents. Antimicrob Agents Chemother. (1998) 42:1290–2. doi: 10.1128/AAC.42.5.1290
231. Ballard HO, Shook LA, Bernard P, Anstead MI, Kuhn R, Whitehead V, et al. Use of azithromycin for the prevention of bronchopulmonary dysplasia in preterm infants: a randomized, double-blind, placebo controlled trial. Pediatr Pulmonol. (2011) 46:111–8. doi: 10.1002/ppul.21352
232. Gharehbaghi MM, Peirovifar A, Ghojazadeh M, Mahallei M. Efficacy of azithromycin for prevention of bronchopulmonary dysplasia (BPD). Turk J Med Sci. (2012) 42:1070–5. doi: 10.3906/sag-1107-18
233. Nunes CR, Procianoy RS, Corso AL, Silveira RC. Use of azithromycin for the prevention of lung injury in mechanically ventilated preterm neonates: a randomized controlled trial. Neonatology. (2020) 117:522–8. doi: 10.1159/000509462
234. Nair V, Loganathan P, Soraisham AS. Azithromycin and other macrolides for prevention of bronchopulmonary dysplasia: a systematic review and meta-analysis. Neonatology. (2014) 106:337–47. doi: 10.1159/000363493
235. Viscardi RM, Terrin ML, Magder LS, Davis NL, Dulkerian SJ, Waites KB, et al. Randomised trial of azithromycin to eradicate Ureaplasma in preterm infants. Arch Dis Child Fetal Neonatal Ed. (2020) 105:615–22. doi: 10.1136/archdischild-2019-318122
236. Smith C, Egunsola O, Choonara I, Kotecha S, Jacqz-Aigrain E, Sammons H. Use and safety of azithromycin in neonates: a systematic review. BMJ Open. (2015) 5:e008194. doi: 10.1136/bmjopen-2015-008194
237. Hassan HE, Othman AA, Eddington ND, Duffy L, Xiao L, Waites KB, et al. Pharmacokinetics, safety, and biologic effects of azithromycin in extremely preterm infants at risk for ureaplasma colonization and bronchopulmonary dysplasia. J Clin Pharmacol. (2011) 51:1264–75. doi: 10.1177/0091270010382021
238. Eberly MD Eide MB, Thompson JL, Nylund CM. Azithromycin in early infancy and pyloric stenosis. Pediatrics. (2015) 135:483–8. doi: 10.1542/peds.2014-2026
239. Lowe J, Gillespie D, Hubbard M, Zhang L, Kirby N, Pickles T, et al. Study protocol: azithromycin therapy for chronic lung disease of prematurity (AZTEC) - a randomised, placebo-controlled trial of azithromycin for the prevention of chronic lung disease of prematurity in preterm infants. BMJ Open. (2020) 10:e041528. doi: 10.1136/bmjopen-2020-041528
240. Ozdemir R, Erdeve O, Dizdar EA, Oguz SS, Uras N, Saygan S, et al. Clarithromycin in preventing bronchopulmonary dysplasia in ureaplasma urealyticum-positive preterm infants. Pediatrics. (2011) 128:e1496–501. doi: 10.1542/peds.2011-1350
241. Tong Y, Zuo J, Yue D. Application prospects of mesenchymal stem cell therapy for bronchopulmonary dysplasia and the challenges encountered. Biomed Res Int. (2021) 2021:9983664. doi: 10.1155/2021/9983664
242. Hass R, Kasper C, Böhm S, Jacobs R. Different populations and sources of human mesenchymal stem cells (MSC): A comparison of adult and neonatal tissue-derived MSC. Cell Commun Signal. (2011) 9:12. doi: 10.1186/1478-811X-9-12
243. Sutsko RP, Young KC, Ribeiro A, Torres E, Rodriguez M, Hehre D, et al. Long-term reparative effects of mesenchymal stem cell therapy following neonatal hyperoxia-induced lung injury. Pediatr Res. (2013) 73:46–53. doi: 10.1038/pr.2012.152
244. van Haaften T, Byrne R, Bonnet S, Rochefort GY, Akabutu J, Bouchentouf M, et al. Airway delivery of mesenchymal stem cells prevents arrested alveolar growth in neonatal lung injury in rats. Am J Respir Crit Care Med. (2009) 180:1131–42. doi: 10.1164/rccm.200902-0179OC
245. Augustine S, Avey MT, Harrison B, Locke T, Ghannad M, Moher D, et al. Mesenchymal Stromal Cell Therapy in Bronchopulmonary Dysplasia: Systematic Review and Meta-Analysis of Preclinical Studies. Stem Cells Transl Med. (2017) 6:2079–93. doi: 10.1002/sctm.17-0126
246. Chen CM, Chou HC, Lin W, Tseng C. Surfactant effects on the viability and function of human mesenchymal stem cells: in vitro and in vivo assessment. Stem Cell Res Ther. (2017) 8:180. doi: 10.1186/s13287-017-0634-y
247. Chang YS, Ahn SY, Yoo HS, Sung SI, Choi SJ, Oh WI, et al. Mesenchymal stem cells for bronchopulmonary dysplasia: phase 1 dose-escalation clinical trial. J Pediatr. (2014) 164:966–72.e6. doi: 10.1016/j.jpeds.2013.12.011
248. Ahn SY, Chang YS, Kim JH, Sung SI, Park WS. Two-year follow-up outcomes of premature infants enrolled in the phase i trial of mesenchymal stem cells transplantation for bronchopulmonary dysplasia. J Pediatr. (2017) 185:49–54.e2. doi: 10.1016/j.jpeds.2017.02.061
249. Ahn SY, Chang YS, Lee MH, Sung SI, Lee BS, Kim KS, et al. Stem cells for bronchopulmonary dysplasia in preterm infants: A randomized controlled phase II trial. Stem Cells Transl Med. (2021)10:1129–37. doi: 10.1002/sctm.20-0330
250. Powell SB, Silvestri JM. Safety of intratracheal administration of human umbilical cord blood derived mesenchymal stromal cells in extremely low birth weight preterm infants. J Pediatr. (2019) 210:209–13.e2. doi: 10.1016/j.jpeds.2019.02.029
251. Wu X, Xia Y, Zhou O, Song Y, Zhang X, Tian D, et al. Allogeneic human umbilical cord-derived mesenchymal stem cells for severe bronchopulmonary dysplasia in children: study protocol for a randomized controlled trial (MSC-BPD trial). Trials. (2020) 21:125. doi: 10.1186/s13063-019-3935-x
252. Willis GR, Fernandez-Gonzalez A, Anastas J, Vitali SH, Liu X, Ericsson M, et al. Mesenchymal stromal cell exosomes ameliorate experimental bronchopulmonary dysplasia and restore lung function through macrophage immunomodulation. Am J Respir Crit Care Med. (2018) 197:104–16. doi: 10.1164/rccm.201705-0925OC
253. Chaubey S, Thueson S, Ponnalagu D, Alam MA, Gheorghe CP, Aghai Z, et al. Early gestational mesenchymal stem cell secretome attenuates experimental bronchopulmonary dysplasia in part via exosome-associated factor TSG-6. Stem Cell Res Ther. (2018) 9:173. doi: 10.1186/s13287-018-0903-4
254. Porzionato A, Zaramella P, Dedja A, Guidolin D, Van Wemmel K, Macchi V, et al. Intratracheal administration of clinical-grade mesenchymal stem cell-derived extracellular vesicles reduces lung injury in a rat model of bronchopulmonary dysplasia. Am J Physiol Lung Cell Mol Physiol. (2019) 316:L6–19. doi: 10.1152/ajplung.00109.2018
255. Willis GR, Fernandez-Gonzalez A, Reis M, Yeung V, Liu X, Ericsson M, et al. Mesenchymal stromal cell-derived small extracellular vesicles restore lung architecture and improve exercise capacity in a model of neonatal hyperoxia-induced lung injury. J Extracell Vesicles. (2020) 9:1790874. doi: 10.1080/20013078.2020.1790874
256. A Safety Study of IV Stem Cell-derived Extracellular Vesicles (UNEX-42) in Preterm Neonates at HIgh Risk for BPD. ClinicalTrials.gov (2019). Available online at: https://clinicaltrials.gov/ct2/show/NCT03857841 (accessed January 10, 2022).
257. Firsova AB, Bird AD, Abebe D, Ng J, Mollard R, Cole TJ. Fresh noncultured endothelial progenitor cells improve neonatal lung hyperoxia-induced alveolar injury. Stem Cells Transl Med. (2017) 6:2094–105. doi: 10.1002/sctm.17-0093
258. Alphonse RS, Vadivel A, Fung M, Shelley WC, Critser PJ, Ionescu L, et al. Existence, functional impairment, and lung repair potential of endothelial colony-forming cells in oxygen-induced arrested alveolar growth. Circulation. (2014) 129:2144–57. doi: 10.1161/CIRCULATIONAHA.114.009124
259. Baker EK, Malhotra A, Lim R, Jacobs SE, Hooper SB, Davis PG, et al. Human amnion cells for the prevention of bronchopulmonary dysplasia: a protocol for a phase I dose escalation study. BMJ Open. (2019) 9:e026265. doi: 10.1136/bmjopen-2018-026265
260. Ozer EA, Kumral A, Ozer E, Yilmaz O, Duman N, Ozkal S, et al. Effects of erythropoietin on hyperoxic lung injury in neonatal rats. Pediatr Res. (2005) 58:38–41. doi: 10.1203/01.PDR.0000163391.75389.52
261. Cui H, He J, Chen H, Chen J, Qian X, Huang W. Erythropoietin attenuates hyperoxia-induced lung injury by upregulating epidermal growth factor-like domain 7 in newborn rats. Biomed Rep. (2017) 6:32–8. doi: 10.3892/br.2016.820
262. Polglase GR, Barton SK, Melville JM, Zahra V, Wallace MJ, Siew ML, et al. Prophylactic erythropoietin exacerbates ventilation-induced lung inflammation and injury in preterm lambs. J Physiol. (2014) 592:1993–2002. doi: 10.1113/jphysiol.2013.270348
263. Holm M, Skranes J, Dammann O, Fichorova RN, Allred EN, Leviton A. Systemic endogenous erythropoietin and associated disorders in extremely preterm newborns. Arch Dis Child Fetal Neonatal Ed. (2016) 101:F458–63. doi: 10.1136/archdischild-2015-309127
264. Bui KCT, Kim R, Abbasi A, Nguyen M, Villosis MF, Chen Q. Erythropoietin treatment is associated with a reduction in moderate to severe bronchopulmonary dysplasia in preterm infants. A regional retrospective study Early Hum Dev. (2019) 137:104831. doi: 10.1016/j.earlhumdev.2019.104831
265. Rayjada N, Barton L, Chan LS, Plasencia S, Biniwale M, Bui KC. Decrease in incidence of bronchopulmonary dysplasia with erythropoietin administration in preterm infants: a retrospective study. Neonatology. (2012) 102:287–92. doi: 10.1159/000341615
266. Ananthan A, Balasubramanian H, Rao S, Patole S. Clinical outcomes related to the gastrointestinal trophic effects of erythropoietin in preterm neonates: a systematic review and meta-analysis. Adv Nutr. (2018) 9:238–46. doi: 10.1093/advances/nmy005
267. Juul SE, Comstock BA, Wadhawan R, Mayock DE, Courtney SE, Robinson T, et al. A Randomized trial of erythropoietin for neuroprotection in preterm infants. N Engl J Med. (2020) 382:233–43. doi: 10.1056/NEJMoa1907423
268. Sun C, Zhang S, Wang J, Jiang W, Xin Q, Chen X, et al. EPO enhances the protective effects of MSCs in experimental hyperoxia-induced neonatal mice by promoting angiogenesis. Aging. (2019) 11:2477–87. doi: 10.18632/aging.101937
269. Luan Y, Zhang L, Chao S, Liu X, Li K, Wang Y, et al. Mesenchymal stem cells in combination with erythropoietin repair hyperoxia-induced alveoli dysplasia injury in neonatal mice via inhibition of TGF-β1 signaling. Oncotarget. (2016) 7:47082–94. doi: 10.18632/oncotarget.9314
270. Valentine CJ. Maternal dietary DHA supplementation to improve inflammatory outcomes in the preterm infant. Adv Nutr. (2012) 3:370–6. doi: 10.3945/an.111.001248
271. Manley BJ, Makrides M, Collins CT, McPhee AJ, Gibson RA, Ryan P, et al. DINO Steering Committee. High-dose docosahexaenoic acid supplementation of preterm infants: respiratory and allergy outcomes. Pediatrics. (2011) 128:e71–7. doi: 10.1542/peds.2010-2405
272. Collins CT, Makrides M, McPhee AJ, Sullivan TR, Davis PG, Thio M, et al. Docosahexaenoic acid and bronchopulmonary dysplasia in preterm infants. N Engl J Med. (2017) 376:1245–55. doi: 10.1056/NEJMoa1611942
273. Marc I, Piedboeuf B, Lacaze-Masmonteil T, Fraser W, Mâsse B, Mohamed I, et al. Effect of maternal docosahexaenoic acid supplementation on bronchopulmonary dysplasia-free survival in breastfed preterm infants: a randomized clinical trial. JAMA. (2020) 324:157–67. doi: 10.1001/jama.2020.8896
274. Dhanireddy R, Kikukawa T, Mukherjee AB. Detection of a rabbit uteroglobin-like protein in human neonatal tracheobronchial washings. Biochem Biophys Res Commun. (1988) 152:1447–54. doi: 10.1016/s0006-291x(88)80448-0
275. Arias-Martínez J, Palacios-Sánchez M, Delgado-Franco D, Guzmán-Bárcenas J, García-Latorre E, Zhang L, et al. Clara cell protein expression in human neonates during respiratory distress syndrome. Cell Physiol Biochem. (2012) 29:753–60. doi: 10.1159/000264417
276. Rivera L, Siddaiah R, Oji-Mmuo C, Silveyra GR, Silveyra P. Biomarkers for bronchopulmonary dysplasia in the preterm infant. Front Pediatr. (2016) 4:33. doi: 10.3389/fped.2016.00033
277. Schrama AJ, Bernard A, Poorthuis BJ, Zwinderman AH, Berger HM, Walther FJ. Cord blood Clara cell protein CC16 predicts the development of bronchopulmonary dysplasia. Eur J Pediatr. (2008) 167:1305–12. doi: 10.1007/s00431-008-0713-2
278. Sarafidis K, Stathopoulou T, Diamanti E, Soubasi V, Agakidis C, Balaska A, et al. Clara cell secretory protein (CC16) as a peripheral blood biomarker of lung injury in ventilated preterm neonates. Eur J Pediatr. (2008) 167:1297–303. doi: 10.1007/s00431-008-0712-3
279. Wang K, Huang X, Lu H, Zhang Z A. comparison of KL-6 and Clara cell protein as markers for predicting bronchopulmonary dysplasia in preterm infants. Dis Markers. (2014) 2014:736536. doi: 10.1155/2014/736536
280. Guzmán-Bárcenas J, Calderón-Moore A, Baptista-González H, Irles C. Clara cell protein expression in mechanically ventilated term and preterm infants with respiratory distress syndrome and at risk of bronchopulmonary dysplasia: a pilot study. Can Respir J. (2017) 2017:8074678. doi: 10.1155/2017/8074678
281. Ramsay PL, DeMayo FJ, Hegemier SE, Wearden ME, Smith CV, Welty SE. Clara cell secretory protein oxidation and expression in premature infants who develop bronchopulmonary dysplasia. Am J Respir Crit Care Med. (2001) 164:155–61. doi: 10.1164/ajrccm.164.1.2008022
282. Levine CR, Gewolb IH, Allen K, Welch RW, Melby JM, Pollack S, et al. The safety, pharmacokinetics, and anti-inflammatory effects of intratracheal recombinant human Clara cell protein in premature infants with respiratory distress syndrome. Pediatr Res. (2005) 58:15–21. doi: 10.1203/01.PDR.0000156371.89952.35
283. Davis JM, Pilon AL, Shenberger J, Breeze JL, Terrin N, Mazela J, et al. The role of recombinant human CC10 in the prevention of chronic pulmonary insufficiency of prematurity. Pediatr Res. (2019) 86:254–60. doi: 10.1038/s41390-019-0419-3
284. Poonyagariyagorn HK, Metzger S, Dikeman D, Mercado AL, Malinina A, Calvi C, et al. Superoxide dismutase 3 dysregulation in a murine model of neonatal lung injury. Am J Respir Cell Mol Biol. (2014) 51:380–90. doi: 10.1165/rcmb.2013-0043OC
285. Delaney C, Wright RH, Tang JR, Woods C, Villegas L, Sherlock L, et al. Lack of EC-SOD worsens alveolar and vascular development in a neonatal mouse model of bleomycin-induced bronchopulmonary dysplasia and pulmonary hypertension. Pediatr Res. (2015) 78:634–40. doi: 10.1038/pr.2015.166
286. Perveen S, Patel H, Arif A, Younis S, Codipilly CN, Ahmed M. Role of EC-SOD overexpression in preserving pulmonary angiogenesis inhibited by oxidative stress. PLoS ONE. (2012) 7:e51945. doi: 10.1371/journal.pone.0051945
287. Rosenfeld W, Evans H, Concepcion L, Jhaveri R, Schaeffer H, Friedman A. Prevention of bronchopulmonary dysplasia by administration of bovine superoxide dismutase in preterm infants with respiratory distress syndrome. J Pediatr. (1984) 105:781–5. doi: 10.1016/s0022-3476(84)80307-8
288. Davis JM, Parad RB, Michele T, Allred E, Price A, Rosenfeld W. North American Recombinant Human CuZnSOD Study Group. Pulmonary outcome at 1 year corrected age in premature infants treated at birth with recombinant human CuZn superoxide dismutase. Pediatrics. (2003) 111:469–76. doi: 10.1542/peds.111.3.469
289. Kicinski P, Malachowska B, Wyka K, Gach A, Jakubowski L, Gulczynska E. The level of extracellular superoxide dismutase in the first week of life in very and extremely low birth weight infants and the risk of developing bronchopulmonary dysplasia. J Perinat Med. (2019) 47:671–6. doi: 10.1515/jpm-2018-0418
290. Schulzke SM, Deshmukh M, Nathan EA, Doherty DA, Patole SK. Nebulized pentoxifylline for reducing the duration of oxygen supplementation in extremely preterm neonates. J Pediatr. (2015) 166:1158–62.e2. doi: 10.1016/j.jpeds.2015.01.040
291. ter Horst SA, Wagenaar GT, de Boer E, van Gastelen MA, Meijers JC, Biemond BJ, et al. Pentoxifylline reduces fibrin deposition and prolongs survival in neonatal hyperoxic lung injury. J Appl Physiol. (1985) (2004) 97:2014–9. doi: 10.1152/japplphysiol.00452.2004
292. Almario B, Wu S, Peng J, Alapati D, Chen S, Sosenko IR. Pentoxifylline and prevention of hyperoxia-induced lung -injury in neonatal rats. Pediatr Res. (2012) 71:583–9. doi: 10.1038/pr.2012.14
293. Lauterbach R, Szymura-Oleksiak J. Nebulized pentoxifylline in successful treatment of five premature neonates with bronchopulmonary dysplasia. Eur J Pediatr. (1999) 158:607. doi: 10.1007/s004310051158
294. Lauterbach R, Szymura-Oleksiak J, Pawlik D, Warchoł J, Lisowska-Miszczyk I, Rytlewski K. Nebulized pentoxifylline for prevention of bronchopulmonary dysplasia in very low birth weight infants: a pilot clinical study. J Matern Fetal Neonatal Med. (2006) 19:433–8. doi: 10.1080/14767050600736754
295. Curis E, Nicolis I, Moinard C, Osowska S, Zerrouk N, Bénazeth S, et al. Almost all about citrulline in mammals. Amino Acids. (2005) 29:177–205. doi: 10.1007/s00726-005-0235-4
296. Scott JA, Maarsingh H, Holguin F, Grasemann H. Arginine therapy for lung diseases. Front Pharmacol. (2021) 12:627503. doi: 10.3389/fphar.2021.627503
297. Vadivel A, Aschner JL, Rey-Parra GJ, Magarik J, Zeng H, Summar M, et al. L-citrulline attenuates arrested alveolar growth and pulmonary hypertension in oxygen-induced lung injury in newborn rats. Pediatr Res. (2010) 68:519–25. doi: 10.1203/PDR.0b013e3181f90278
298. Montgomery AM, Bazzy-Asaad A, Asnes JD, Bizzarro MJ, Ehrenkranz RA, Weismann CG. Biochemical screening for pulmonary hypertension in preterm infants with bronchopulmonary dysplasia. Neonatology. (2016) 109:190–4. doi: 10.1159/000442043
299. Piersigilli F, Lam TT, Vernocchi P, Quagliariello A, Putignani L, Aghai ZH, et al. Identification of new biomarkers of bronchopulmonary dysplasia using metabolomics. Metabolomics. (2019) 15:20. doi: 10.1007/s11306-019-1482-9
300. Lauterbach R, Pawlik D, Lauterbach JP. L-citrulline supplementation in the treatment of pulmonary hypertension associated with bronchopulmonary dysplasia in preterm infant: A case report. SAGE Open Med Case Rep. (2018) 6:2050313X18778730. doi: 10.1177/2050313X18778730
301. Hallman M, Saugstad OD, Porreco RP, Epstein BL, Gluck L. Role of myoinositol in regulation of surfactant phospholipids in the newborn. Early Hum Dev. (1985) 10:245–54. doi: 10.1016/0378-3782(85)90055-6
302. Phelps DL, Ward RM, Williams RL, Watterberg KL, Laptook AR, Wrage LA. et al. Pharmacokinetics and safety of a single intravenous dose of myo-inositol in preterm infants of 23-29 wk. Pediatr Res. (2013) 74:721–9. doi: 10.1038/pr.2013.162
303. Howlett A, Ohlsson A, Plakkal N. Inositol in preterm infants at risk for or having respiratory distress syndrome. Cochrane Database Syst Rev. (2019) 7:CD000366. doi: 10.1002/14651858.CD000366.pub4
304. Ley D, Hallberg B, Hansen-Pupp I, Dani C, Ramenghi LA, Marlow N, et al. rhIGF-1/rhIGFBP-3 in preterm infants: a phase 2 randomized controlled trial. J Pediatr. (2019) 206:56-65.e8. doi: 10.1016/j.jpeds.2018.10.033
305. Vadivel A, Alphonse RS, Etches N, van Haaften T, Collins JJ, O'Reilly M, et al. Hypoxia-inducible factors promote alveolar development and regeneration. Am J Respir Cell Mol Biol. (2014) 50:96–105. doi: 10.1165/rcmb.2012-0250OC
306. Collins JJP, Tibboel D, de Kleer IM, Reiss IKM, Rottier RJ. The future of bronchopulmonary dysplasia: emerging pathophysiological concepts and potential new avenues of treatment. Front Med (Lausanne). (2017) 4:61. doi: 10.3389/fmed.2017.00061
307. Nadeau-Vallée M, Chin PY, Belarbi L, Brien MÈ, Pundir S, Berryer MH, et al. Antenatal suppression of IL-1 protects against inflammation-induced fetal injury and improves neonatal and developmental outcomes in mice. J Immunol. (2017) 198:2047–62. doi: 10.4049/jimmunol.1601600
308. Royce SG, Nold MF, Bui C, Donovan C, Lam M, Lamanna E, et al. Airway remodeling and hyperreactivity in a model of bronchopulmonary dysplasia and their modulation by il-1 receptor antagonist. Am J Respir Cell Mol Biol. (2016) 55:858–68. doi: 10.1165/rcmb.2016-0031OC
309. Zhang ZQ, Hong H, Li J, Li XX, Huang XM. MicroRNA-214 promotes alveolarization in neonatal rat models of bronchopulmonary dysplasia via the PlGF-dependent STAT3 pathway. Mol Med. (2021) 27:109. doi: 10.1186/s10020-021-00374-4
Keywords: bronchopulmonary dysplasia, BPD, chronic lung disease, neonates, preterm, neonatal pharmacology
Citation: Sakaria RP and Dhanireddy R (2022) Pharmacotherapy in Bronchopulmonary Dysplasia: What Is the Evidence? Front. Pediatr. 10:820259. doi: 10.3389/fped.2022.820259
Received: 22 November 2021; Accepted: 26 January 2022;
Published: 09 March 2022.
Edited by:
Graeme R. Polglase, Monash University, AustraliaReviewed by:
Christopher Baker, University of Colorado, United StatesHelen Christou, Brigham and Women's Hospital and Harvard Medical School, United States
Copyright © 2022 Sakaria and Dhanireddy. This is an open-access article distributed under the terms of the Creative Commons Attribution License (CC BY). The use, distribution or reproduction in other forums is permitted, provided the original author(s) and the copyright owner(s) are credited and that the original publication in this journal is cited, in accordance with accepted academic practice. No use, distribution or reproduction is permitted which does not comply with these terms.
*Correspondence: Rishika P. Sakaria, cnNha2FyaWEmI3gwMDA0MDt1dGhzYy5lZHU=