- 1Faculty of Sport and Psychology, Educons University in Novi Sad, Novi Sad, Serbia
- 2Faculty of Sports and Physical Education, University of Belgrade, Belgrade, Serbia
Background and Objectives: School bag (SB) load causes significant changes in the height and symmetry of the intervertebral discs at each level of the spine from T12-L1 to L5-S1. This study aims to determine the change in the size of the lumbar segment angle at a particularly critical point L3-L4 of the spine in relation to the load of the average weight of SB in healthy male children (students) at standing and after 2-minute gait.
Methods: 47 boys, aged 12.2 ± 0.92 years, underwent photogrammetric measurements in the sagittal plane in statics and dynamics, walking on a laboratory treadmill. Measurements were repeated with the weight of SB with a constant load of 6,251 kg, which represents 13.78% of the average body weight of our sample. The lumbar angle (LA) connecting the point of the big toe, the lumbar point L3-L4 and the processus spinosus C7 was measured. In gait, LA was measured in the phases of the middle support and the initial contact of the heel.
Results: T-test of paired samples was used to estimate the change in LA at standing from 4.953° and walking phases from 6.295° to 7.332° in relation to the unloaded state, and the value of the effect size (ES) indicates that the impact of SB load is significant.
Conclusions: Cumulatively, microtraumas caused by SB load significantly affect the increase in intervertebral pressure at the L3-L4 point, which is susceptible to degenerative processes and which can be the cause of lumbar syndrome (LS). Preventive measures are needed in order to lighten SB in this population and introduce up to 10% of students' body weight into the safe zone.
Introduction
Researches, that have dealt with risk factors that affect the degenerative processes of the spine, suggest that this issue requires a complex analysis of their occurrence. In addition to genetic preconditions (1), anthropometric parameters (2) and microtrauma are the most common exogenous factors that can overload the spine during growth and development and thus have an impact on the etiology of degenerative processes (3–7). Exogenous factors, especially in the sensitive period of growth and development, can have a negative impact on posture (8, 9). One of the most frequently mentioned factors that intrigues the scientific public and worries parents is the load conditioned by the weight of the school bag - school backpack (SB) (10–16). A number of studies have identified that students most often carry SB on both shoulders (83%) (17), and that SB weight ranged from 10%–22% of the total body weight of students (18–24). The load caused by SB leads to an increase in axial pressure on the spinal column and causes changes in the height and symmetry of the intervertebral discs of the T12-S1 level, but also changes in the angular values of the intervertebral relations (25). The size, frequency and duration of the load conditioned by SB, additionally increases the risk of creating postural changes in students during the period of rapid growth and development (26, 27).
Previous researches, that have dealt with the problem of SB load on the spinal column and the reaction in the form of changing the angle for the torso segment, analyzed different angles. They have dealt with the angles formed between the points trochanterion (femoris) and acromion and in relation to the reference horizontal (18, 19, 21, 28, 29), i.e., vertical line that intersects the great trochanter of the femur (30–32). A group of German authors examined the angle of inclination of the torso in relation to the horizontal line, where the torso is defined as a vertical line formed by the mean values of anatomical points on its upper (both acromion, jugular fossa and processus spinosus C7) and lower segment (upper sacrum, left and right iliac bone - anterior spina iliaca superior) (33). The angle of inclination of the torso that forms the anatomical points on the big toe, the intervertebral space between L3-L4 and the processus spinosus C7, was the subject of a study that considered the change in angle under different loads due to carrying different types of women's bags (female subjects, aged 19 to 37) (34). In accordance with all the above, the angle of the lumbar segment that defines the anatomical points of the big toe, the intervertebral space L3-L4 and the processus spinosus C7, can be studied by considering additional load in the form of SB and in the student population. The reasons are primarily related to the understanding of anatomical-physiological structure, and forces influencing the lumbar spine segment located on the disc between L3-L4, which also forms the peak of the lordotic curve and is the riskiest place for degenerative changes (35, 36). Intervertebral pressure in resting phases such as standing, sitting without support, as well as all types of lying, is highest in the L3-L4 region (37). Anatomically, L4 has the smallest prosessus transverus and relatively long iliotransversal ligaments that provide less support and stability (38). The multifidus muscle maintains lumbar lordosis by acting as a tendon that helps transmit certain axial compression forces on the disc to the anterior longitudinal ligament by shifting from the compression to the tensile load and further protects the discs by preventing unexpected movements such as twisting and bending (39). Regardless of the more significant role which the paraspinal muscles have in the protection of the L3-L4 segment, the greatest correlation of multifidus muscle atrophy is in that region, which additionally makes this point susceptible to degenerative changes (36).
The large weight of SB on the student's back changes the center of gravity of the body and conditions the adjustment to the posterior load (40). As the external load increases, the flexion of the torso increases significantly in response to the motor control mechanism to move the combined center of gravity of the body and the bag forward in order to maintain balance (21, 29, 40–43). The combination of external load and degree of torso flexion leads to increased pressure on the intervertebral discs (44–47).
This study aims to determine the change in the size of the angle of the lumbar segment of the spine in relation to the load of the average weight of SB in healthy male children (students) aged 10 to 13 years. In accordance with the reviewed literature, the hypothesis of this study is that the load of the SB significantly increases the flexion of the torso, and thus the compression of the vertebral discs. The flexion of the torso is related to the reduction of the lumbar angle formed by the examined anatomical points of the big toe, the lumbar points L3-L4 and the processus spinosus C7 (LA). The task of this research is to define the acute effect of SB load when standing and after 2-minute gait.
Materials and methods
Examinees
This study was a 2-factor repeated-measures design, with the participants serving as their own controls, a sample of 47 male students aged 10–13 years, mean age 12.2 ± 0.92 years, mean height 155.2 ± 8.0 cm and average body weight 45.3 ± 8.7 kg (Table 1). All students are of the same nationality, live in the same city and in the same municipality and attend the same school. Population and age were selected for this study to exclude factors of full demorphism in changes in the musculoskeletal system during the second sensitive period associated with the impact of accelerated growth and development (48). The phase of accelerated growth is a period in which posture irregularities often occur (49) and is accompanied by rapid bone growth and certain limitations of muscle elasticity, a characteristic primarily of postural muscles (50). The basic criteria for inclusion were that they always carry SB symmetrically over both shoulders, that they do not have any acute injuries and diseases and do not engage in any organized physical activity outside the school, except for physical education classes.
Study design and procedures
The experimental factor of this study represents the load in the form of SB, was defined on the basis of the weight which is conditioned by the content of SB, and in relation to the weekly school schedule (5 days a week, for 6 school lessons). The measured load of SB was 6,251 kg, that is, the load was an average 13.78% of the body weight of each subject. In addition to identifying the workload on a weekly basis, examinees were monitored from school entry to the classroom to identify how the workload was distributed, i.e., how respondents carried SB, and what time period was required to put the load down. It was found that the respondents, in 85% of cases, carried the load, SB, on both shoulders, and that on average it took about 120 s from entering the school to putting the load down (this time measure was used to assess variables in dynamic conditions).
The size of the lumbar segment (LA) angle at the L3-L4 level and its changes, caused by the additional SB load in statics and dynamics, were identified. The LA angle is defined by anatomical points: the tip of the big toe, the intervertebral space L3-L4 and the processus spinosus C7. Identification of LA size in relation to SB load in statics and dynamics was performed by photogrammetric measurement in the sport diagnostic laboratory. In the statics, the values of the angle, without and with the load SB, were identified. The values of angles in dynamics (without and with the load) were identified when walking in the phase of the middle support and the phase of initial contact of the heel, and at a speed of 4 km/h on the treadmill because it represents a comfortable, normal walking speed of children (51). In order to familiarize the subjects with the test in which angles are identified in dynamic conditions, they were exposed to a 5-minute walk (4 km/h) without load, prior to the measurement procedure (52).
The study was conducted following the ethical standards of the Declaration of Helsinki. The school and the parents have given the permission for their children's participation in this research. We have an Institutional Review Board approval document given by the Ethical Board of the Faculty of Sport and Psychology, Novi Sad, no. 423-2/2022, dated 12.05.2022.
Variables
The variables in this study were analyzed in the sagittal plane. The flexion of the torso was analyzed on the basis of the defined lumbar angle (LA) which forms the anatomical points of the big toe, the lumbar points L3-L4 and the processus spinosus C7 in: (1) Statics (a) without SB; and (b) with SB; (2) Dynamics in the phase of middle support with the right foot (a) without SB and (b) with SB; (3) Dynamics in the phase of middle support with the left foot (a) without SB and (b) with SB; (4) Dynamics in the phase of initial contact of the heel with the right foot (a) without SB and (b) with SB and (5) Dynamics in the phase of initial contact of the heel with the left foot (a) without SB and (b) with SB. The middle phase of the support was analyzed at the moment when the weight of the body was transferred to the standing leg, and the opposite, swinging leg, was off of the ground. The initial contact was analyzed at the moment when the heel of the front foot touched the ground (48, 53).
Tools
For the purposes of this research, two instruments were used: (1) The system (hardware and software) of the German company Contemplas was used for the estimated variables in statics. Templo software with 2D in 3 View protocol. The cameras used in the postural analysis are from the manufacturer “IDS Imaging”, model UI164xLE-C, type uEye, Firmware V1.0, USB interface, max. resolution 1280 × 1024, frequency 50 Hz. (2) For the estimated variables in dynamics, on the treadmill, Templo - Software of the German company GMBH Contemplas with software module: General Motion Analysis. Two high-speed cameras were used - Basler full HD 240 Hz. The laboratory treadmill tape is Sprintex CALLIS Z6000, with a maximum speed of 22 km per hour with a possible elevation of 15%. The lamella technology of the laboratory treadmill belt, on which we performed the test, allows a natural step, provides the best ergonomic experience of walking and running with minimal impact on the joints (51, 52). This treadmill belt has no sliding speed, it has a true zero starting speed, which is very convenient for testing and measurements. In both procedures, retroreflective markers with a diameter of 19 mm were placed on the anatomical points of the subjects for imaging. Both systems are non-invasive and completely safe for testing. The drawing and calculation of angles was done with the help of Software, marking pre-marked anatomical points with the help of retroreflective markers. On the basis of previously defined and marked anatomical points, drawing angles with tools in the software was carried out by two diagnosticians independently of each other, and under the control of a third certified photogrammetric expert who, in the case of differently plotted angles, with expert argumentation, made the final judgment on the position of the arms of the angle.
Data analysis
The obtained results were statistically analyzed in the program SPSS V.26. package. Descriptive statistics were applied, t-test of paired samples was used to identify differences in LA with and without SB in statics and dynamics. Calculate the value of Cohen's d and the effect size (ES) correlation, rYλ, using the t-test value for a between subjects t-test and the degrees of freedom: Cohen's d = 2t / √ (df); rYλ= √/[t2 / (t2 + df)] (54).
Results
Table 2 shows the results of the t-test of paired samples which tested the difference in the size of the LA of the subjects, observed in the sagittal plane, and measured in statics. A statistically significant decrease in LA was found during SB load. The average decrease in LA was 4.953°, and the value of the effect size (ES = 0.634) indicates that the impact of the SB load is significant.
Tables 3, 4 show the results of the t-test of paired samples, which tested the difference in the size of LA of the subjects, observed in the sagittal plane, and measured in dynamics. In this test, LA was observed during the middle support with the right / left foot, i.e., in the phase of initial contact. For the phase of the middle support on the right foot (Table 3), a statistically significant decrease in LA was determined during SB load. The average decrease in LA was 6.295°, and the value of the effect size (ES = 0.706) indicates that the impact of the SB load is significant. The value of the change in LA at the phase of the middle support with the left foot (Table 3) indicates a statistically significant decrease in LA during SB load. The average decrease in LA was 6.664°, and the value of the effect size (ES = 0.792) indicates that the impact of the SB load is significant (Table 3).
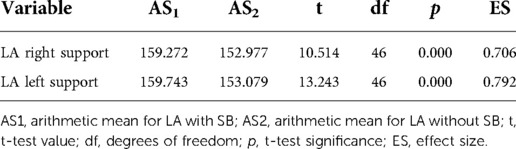
Table 3. Influence of SB load on the change in the size of LA at the phase of the middle support for the right/left leg.
Table 4 shows the values for LA with and without SB and in the initial contact phase for the right/left leg. Statistically significant values can be observed for both left and right leg. The average reduction of LA at the initial contact for the right leg was 7.332°, and the value of the effect size (ES = 0.782) indicates that the impact of the SB load is significant. Also, a statistically significant change of the value in LA with the load in the phase of initial contact for the left leg is also observed. The average decrease in LA was 7.106°, and the value of the effect size (ES = 0.790).
Discussion
In this study, the effects of short-term SB load on the lumbar segment, i.e., the change in angle in the L3-L4 level of students aged 10–13 years, in statics and dynamics, were investigated. For all examinees, the average weight of SB (6,251 kg) was defined, which is coordinated with the weekly school schedule. The load of SB at the group level was 13,78% of the average body weight of the subjects. This value can also represent a limitation of the study, because the load is not defined in relation to body mass and for each subject individually. At the group level in accordance with the defined workload of SB, it was found that there were changes in LA in statics with load, that is, the measured angle was reduced by an average of 4,953° and the value of ES (0.634) indicates that the impact of the load is significant. This result is in accordance with previous studies of the impact of SB load, which ranged from 10%–20% of the average body weight of the subjects (20–23). In the studies that treated the problem of changing the angle of the torso under load, it was found that the forward inclination of the torso in relation to the vertical line, caused by the load SB, ranges from 3.02° to 6.8° (31, 55, 56).
In our study, the phases of the middle support and the initial contact for the left and right foot when walking on a treadmill in a time frame of 110–120 s, were also analyzed. The change of LA at SB load was also identified, and the values for both tested phases ranged from 6.295° to 7.332°, while the ES value ranged from 0.706 to 0.792, which indicates that the effect of load for all four variables tested in dynamics was significant. Studies that have treated the problem of SB load (10%–20% of total body weight) in dynamic conditions and changes in the forward inclination of the of the torso in relation to the axial line, identified values ranging from 4.55° to as high as 19.80° (18, 19, 21, 28–30, 32, 33), which is also confirmed in this study. Differences in the range of angle changes in these studies can be explained by various experimental factors such as: anthropological differences in children (students) from different areas, gender, whether the dynamics were analyzed in the laboratory or open field, time spent in the dynamics after which the change in the inclination of the torso was analyzed, the weight and composition of the load filled SB (sand, weights, books, etc.) and the height at which their center of gravity was adjusted relative to the back of the subjects, etc. Therefore, the closest study to this one in relation to experimental factors is the study of Lee, Hong and Robinson on 15 boys, average age 10.36 years, with SB load of average weight of 10% of body weight, after 1 min gait on the treadmill, verified the change of the torso angle of 4.55° in relation to the torso angle without load SB (28). The results of studies in 10-year-old boys walking on a treadmill, where the average weight of SB was 15% and 20% of body weight, also identified a great influence on the change in the angle of the torso. In the same studies, when the load was 10% of the body weight of the subjects, i.e., without the SB load, there was no significant effect on the change in the angle of the torso (19, 21). The studies that have treated different weights, i.e., SB load on subjects aged 10–13 years, found that any load greater than 10% relative to average body weight has a large impact on torso inclination and LA change (18, 30).
The child's skeleton has a large amount of cartilage, significantly in the region of the spine as a prerequisite for growth. These cartilaginous regions consist of articular cartilage, epiphyses, and apophysis (24). Each of these structures is subject to different types of injury. Articular cartilage is susceptible to current stress, while the epiphyses and apophysis are more susceptible to recurrent microtrauma (57). Studies in vivo measuring intervertebral pressure, measured a pressure of about 0.3 MPa at the L3-L4 level (range 0.27–0.33 MPa) (37). When the torso was bent forward, an increase in intervertebral pressure of 2.5–3.6 times was found (45). The combination of intervertebral disc compression and torso flexion pre-induced by SB weight is a microtrauma that will cause an increase in intervertebral pressure (46, 47). Increased intervertebral pressure may be the cause of the accelerated process of disc degeneration (58, 59). In such a degenerated disc, the fluid level decreases, while the nucleus pulposus is not able to maintain uniform pressure on the adjacent fibrous ring and end plates (46, 47, 60). The fibrous ring consists of several nerve endings in its posterior part (61). The concentration of stress due to compression and flexion of the torso is maximal in the posterior part of the fibrous ring and irritates nerve endings that mechanically cause acute but can also be the cause of chronic lower back pain - lumbar syndrome (LS) (60, 62). LS is a major problem in the population of 40% of young people aged 9 to 18 worldwide (20, 63). The load of SB, which is greater than 10% of the total body weight (as is the case in this study), has a negative effect and contributes to the development of LS in students (20, 64). The fact that the painful condition in LS is associated with SB load was identified in 82% of students aged 11 to 14 years (65). These data are worrying because LS in youth plays an important role in the development of chronic LS in adulthood (20, 66). However, the negative impact of SB can be prevented, primarily by limiting the weight to a maximum of 10% in relation to the total body weight of the child, which can have a positive impact on the spine and thus on quality of life in later years (67). Also, it was found that the design of SB (55) and the acquisition of knowledge about its proper wearing (68, 69) can significantly mitigate its negative effects during statics and dynamics.
Conclusions
The innovativeness of this study is reflected in the fact that for the first time in children (students), the change of LA at the level of L3-L4 with and without SB load was analyzed by photometric method in statics and dynamics. The study found that the average weight of SB was 13.78% of the average body weight of the tested sample. The results showed that the influence of SB load on LA change is great both in statics and dynamics, which is in line with previous research where SB load is greater than 10% of body weight, and, as such, causes a negative effect in terms of degenerative changes. Slightly greater changes in LA in dynamics relative to statics point to differences in the motor control strategies children use to maintain balance. Cumulatively, the microtrauma caused by SB load significantly increases the intervertebral pressure in a very critical segment, L3-L4 (which is susceptible to degenerative processes) and which can be the cause of LS. Preventive measures are needed in order to lighten SB in this population and introduce up to 10% of the child's body weight to the safe zone, as well as designing the most anatomically functional SB and educating about its proper carrying.
Data availability statement
The raw data supporting the conclusions of this article will be made available by the authors, without undue reservation.
Ethics statement
The studies involving human participants were reviewed and approved by Ethical Board of the Faculty of Sport and Psychology, Novi Sad, no. 423-2/2022, dated 12.05.2022. Written informed consent to participate in this study was provided by the participants’ legal guardian/next of kin.
Author contributions
MB, and RR.; methodology, MB, MK.; software, MB, MK validation, RR, ZA.; formal analysis, MB, RR; investigation, MB, MK; resources, MB.; data curation, MB MK.; writing—original draft preparation, MB; writing—review and editing, RR, ZA; visualization, MK.; supervision, RR, ZA.; project administration, RR.; funding acquisition, MB, ZA. All authors contributed to the article and approved the submitted version.
Acknowledgments
The authors thank the participants for their contribution to the study.
Conflict of interest
The authors declare that the research was conducted in the absence of any commercial or financial relationships that could be construed as a potential conflict of interest.
Publisher's note
All claims expressed in this article are solely those of the authors and do not necessarily represent those of their affiliated organizations, or those of the publisher, the editors and the reviewers. Any product that may be evaluated in this article, or claim that may be made by its manufacturer, is not guaranteed or endorsed by the publisher.
References
1. Ala-Kokko L. Genetic risk factors for lumbar disc disease. Ann Med. (2002) 34:42–7. doi: 10.1080/078538902317338634
2. Videman T, Levalahti E, Battié MC. The effects of anthropometrics, lifting strength,and physical activities in disc degeneration. Spine. (2007) 32:1406–13. doi: 10.1097/BRS.0b013e31806011fa
3. Miller JA, Schmatz C, Schultz AB. Lumbar disc degeneration: correlation with age, sex, and spine level in 600 autopsy specimens. Spine (Phila Pa 1976). (1988) 13:173–8. doi: 10.1097/00007632-198802000-00008
4. Videman T, Nurminen M, Troup JD. Volvo award in clinical sciences. Lumbar spinal pathology in cadaveric material in relation to history of back pain, occupation, and physical loading. Spine (Phila Pa 1976). (1990) 15:728–40. doi: 10.1097/00007632-199008010-00002
5. Videman T, Battié MC. The influence of occupation on lumbar degeneration. Spine. (1999) 24:1164–8. doi: 10.1097/00007632-199906010-00020
6. Hassett G, Hart DJ, Manek NJ, Doyle DV, Spector TD. Risk factors for progression of lumbar spine disc degeneration. Arthritis Rheum. (2003) 48:3112–7. doi: 10.1002/art.11321
7. Macedo LG, Battié MC. The association between occupational loading and spine degeneration on imaging – a systematic review and meta-analysis. BMC Musculoskelet Disord. (2019) 20:1–15. doi: 10.1186/s12891-019-2835-2
8. O’ Sullivan PB, Smith AJ, Beales DJ, Straker LM. Association of biopsychosocial factors with degree of slump in sitting posture and self-report of back pain in adolescents: a cross selectional-stydy. Phys Ther. (2011) 91:470–83. doi: 10.2522/ptj.20100160
9. Latalski M, Bylina J, Fatuga M, Repko M, Filipović M, Jarosz JM, et al. Risk factors of postural defects in children at school age. Ann Agric Envrion Med. (2013) 20:583–7.
10. Vitiello A, Pollard H. Backpack design: the use of ratings of perceived exertion (Borg scale) – a review. Chiropractic J Australia. (2002) 32:91–8.
11. Cottalorda J, Rahmani A, Diop M, Gautheron V, Ebermeyer E, Belli A. Influence of school bag carrying on gait kinetics. J Pediatric Orthopaedics. (2003) 12:357–64. doi: 10.1097/01.bpb.0000078270.58527
12. Cardon G, Balague F. Backpacks and spinal disorders in school children. Eura Medicophys. (2004) 40:15–20. PMID: 16030489
13. Negrini S, Politano E, Carabalona R, Tartarotti L, Marchetti M. The backpack load in schoolchildren: clinical and social importance, and efficacy of a community-based educational intervention. Eur Medicophys. (2004) 40:185–90.
14. Al-Hazzaa HM. How much load do Saudi school boys carry on their shoulder. Saudi Med J. (2006) 27:1567–71.17013484
15. Chow D, Leung K, Holmes A. The effects of load carriage and bracing on the balance of schoolgirls with adolescent idiopathic scoliosis. Eur Spine J. (2007) 16:1351–8. doi: 10.1007/s00586-007-0333-y
16. Ismaila SO, Oriolowo KT. Determination of safe backpack mass for students in tertiary institutions. Proceedings of the 2015 international conference on industrial engineering and operations managemen, Dubai: Piscataway (2015). p. 2526–9.
17. Talbott NR, Bhattacharya A, Davis KG, Shukla R, Levin L. School backpacks: it's More than just a weight problem. Work. (2009) 34:481–94. doi: 10.3233/WOR-2009-0949
18. Pascoe DD, Pascoe DE, Wang YT, Shim DM, Kim CK. Influence of carrying book bags on gait cicle and posture youths. Ergonomics. (1997) 40:631–41. doi: 10.1080/001401397187928
19. Hong Y, Brueggemann GB. Changes in gait patterns in 10-year-old boys with increasing loadswhen walking on a treadmill. Gait Posture. (2000) 11:254–9. doi: 10.1016/S0966-6362(00)00055-2
20. Negrini S, Carabalona R. Backpack on ! Schoolchildren's Perceptions of load, association with back pain and factors determining the load. Spine. (2002) 27:187–95. doi: 10.1097/00007632-200201150-00014
21. Hong Y, Cheung C. Gait and posture responses to backpack load during level walkingin children. Gait Posture. (2003) 17:28–33. doi: 10.1016/S0966-6362(02)00050-4
22. Cavallo CM, Hlavaty TM, Tamase MGM. A pilot study for the development of a primaryprevention program: what is the average weight of a fourth graders backpack? Work. (2003) 20:137–58. Corpus ID: 35490437
23. Forjuoh SN, Schuchmann JA, Lane BL. Correlates of heavy backpack use by elementary school children. Public Health. (2004) 118:532–5. doi: 10.1016/j.puhe.2003.10.010
24. Vaghela NP, Parekh SK, Padsala D, Patel D. Effect of backpack loading on cervical and sagittal shoulder posture in standing and after dynamic activity in school going children. J Family Med Prim Care. (2019) 8:1076–81. doi: 10.4103/jfmpc.jfmpc_367_18
25. Neuschwander TB, Cutrone J, Macias BR, Cutrone S, Murthy G, Chambers H, et al. The effect of backpacks on the lumbar spine in children: a standing magnetic resonance imaging study. Spine. (2010) 35:83–8. doi: 10.1097/BRS.0b013e3181b21a5d
26. Chan SC, Ferguson SJ, Gantenbein-Ritter B. The effects of dynamic loading on the intervertebral disc. Eur Spine J. (2011) 20:1796–812. doi: 10.1007/s00586-011-1827-1
27. Cosma G, Ilinca I, Rosu L, Burileanu A. Physical exercise and it s role in a correct postural alignment. Discobolul Phys Educ Sport Kinetother J. (2015) 1:58–64.
28. Li JX, Hong Y, Robinson PD. The effect of load carriage on movement kinematics and respiratory parameters in children during walking. Eur J Appl Physiol. (2003) 90:35–43. doi: 10.1007/s00421-003-0848-9
29. Li JX, Hong Y. Age difference in trunk kinematics during walking with different backpack weights in 6-to 12-year-old children. Res Sports Med. (2004) 12:135–42. doi: 10.1080/15438620490460486
30. Goodgold S, Mohr K, Samant A, Parke T, Burns T, Gardner L. Effects of backpack load and task demand on trunk forward lean: pilot findings on two boys. Work. (2002) 18:213–20.12441561
31. Kistner F, Fiebert I, Roach K, Moore J. Postural compensations and subjective complaints due to backpack loads and wear time in schoolchildren. Pediatr Phys Ther. (2013) 25:15–24. doi: 10.1097/PEP.0b013e31827ab2f7
32. Zhou N, Yan J, Chen W, Hu C, Zhang X. Effects of daily used bags on biomechanics characteristic of elementary students. Leather Footwear J. (2016) 16:265–74. doi: 10.24264/lfj.16.4.2
33. Hell AK, Braunschweig L, Grages B, Brunner R, Romkes J. Einfluss des schulrucksackgewichtes bei grundschulkindern: gang, muskelaktivität, haltung und stabilität [the influence of backpack weight in school children: gait, muscle activity, posture and stability. Orthopade. (2021) 50:446–54. doi: 10.1007/s00132-020-04047-8
34. Lyu S, LaBat KL. Effects of natural posture imbalance on posture deviation caused by load carriage. Int J Ind Ergon. (2016) 56:115–23. doi: 10.1016/j.ergon.2016.09.006
35. Pritchett JW, Bortel DT. Degenerative symptomatic lumbar scoliosis. Spine. (1993) 18:700–3. doi: 10.1097/00007632-199305000-00004
36. Sun D, Liu P, Cheng J, Ma Z, Liu J, Qin T. Correlation between intervertebral disc degeneration, paraspinal muscle atrophy, and lumbar facet joints degeneration in patients with lumbar disc herniation. Musculoskelet Dis. (2017) 18:1–7. doi: 10.1186/s12891-016-1361-8
37. Dreischarf M, Shirazi-Adl A, Arjmand N, Rohlmann A, Schmidt H. Estimation of loads on human lumbar spine: a review of in vivo and computational model studies. J Biomech. (2016) 49:833–45. doi: 10.1016/j.jbiomech.2015.12.038
38. Kirkaldy-Willis WH, Farfan HF. Instability of the lumbar spine. Clin Orthop. (1982) 165:110–23. doi: 10.1097/00003086-198205000-00015
39. Kader DF, Wardlaw D, Smith FW. Correlation between the MRI changes in the lumbar multifidus muscles and leg pain. Clin Radiol. (2000) 55:145–9. doi: 10.1053/crad.1999.0340
40. Grimmer K, Dansie B, Milanese S, Pirunsan U, Trott P. Adolescent standing postural response to backpack loads: a randomized controlled experimental study. BMC Musculoskelet Dis. (2002) 3:1–10. doi: 10.1186/1471-2474-3-10
41. Motmans RREE, Tomlow S, Vissers D. Trunk muscle activity in different modes of carrying schoolbags. Ergonomics. (2006) 49:127–38. doi: 10.1080/00140130500435066
42. Fiolkowski P, Horodyski M, Bishop M, Williams M, Stylianou L. Changes in gait kinematics and posture with the use of a front pack. Ergonomics. (2006) 49:885–94. doi: 10.1080/00140130600667444
43. Chen YL, Nguyen HT, Chen Y. Influence of school bag loads and carrying methods on body strain among young Male students. Int J Ind Ergon. (2021) 82:1–9. doi: 10.1016/j.ergon.2021.103095
44. Rohlmann A, Neller S, Claes L, Bergmann G, Wilke HJ. Influence of a follower load on intradiscal pressure and intersegmental rotation of the lumbar spine. Spine. (2001) 26:557–61. doi: 10.1097/00007632-200112150-00014
45. Takahashi I, Kikuchi S, Sato K, Sato N. Mechanical load of the lumbar spine during forward bending motion of the trunk–A biomechanical study. Spine. (2006) 31:18–23. doi: 10.1097/01.brs.0000192636.69129.fb
46. Zahaf S, Mansouri B. The effect of the posterior loading on the spine of a school child. Adv Cancer Prev. (2016) 1:1–19. doi: 10.4172/2472-0429.1000112
47. Li JQ, Kwong WH, Chan YL, Kawabata M. Comparison of in vivo intradiscal pressure between sitting and standing in human lumbar spine: a systematic review and meta-analysis. Life (Basel, Switzerland). (2022) 12:1–18. doi: 10.3390/life12030457
48. Abernethy B, Kippers V, Hanrahan SJ. In: Schrag M, editor. Human kinetics. Biophysical foundations of human movement. Champaign, IL, United States: Human Kinetics (2013). p. 41–54.
49. Schlösser TP, Vincken KL, Rogers K, Castelein RM, Shah SA. Natural sagittal spino-pelvic alignment in boys and girls before, at and after the adolescent growth spurt. Eur Spine J. (2015) 24:1158–67. doi: 10.1007/s00586-014-3536-z
50. Upasani VV, Hedequist DJ, Hresko MT, Karlin LI, Emans JB, Glotzbecker MP. Spinal deformity progression after posterior segmental instrumentation and fusion for idiopathic scoliosis. J Child Orthop. (2015) 9:29–37. doi: 10.1007/s11832-015-0632-5
51. Romkes J, Bracht-Schweizer K. The effects of walking speed on upper body kinematics during gait in healthy subjects. Gait Posture. (2017) 54:304–10. doi: 10.1016/j.gaitpost.2017.03.025
52. Leardini A, Sawacha Z, Paolini G, Ingrosso S, Nativo R, Benedetti MG. A new anatomically based protocol for gait analysis in children. Gait Posture. (2007) 26:560–71. doi: 10.1016/j.gaitpost.2006.12.018
53. Pirker W, Katzenschlager R. Gait disorders in adults and the elderly. Wien Klin Wochenschr. (2016) 129:81–95. doi: 10.1007/s00508-016-1096-4
54. Becker LA. Effect size calculators. 2000, Retrieved from https://lbecker.uccs.edu/
55. Mackie HW, Legg SJ, Beadle J, Hedderle D. Comparison of four different backpacks intended for school use. Appl Ergon. (2003) 34:257–64. doi: 10.1016/S0003-6870(03)00034-6
56. Brackley HM, Stevenson JM, Selinger JC. Effect of backpack load placement on posture and spinal curvature in prepubescent children. Work. (2009) 32:351–60. doi: 10.3233/WOR-2009-0833
57. Whittfield JK, Legg SJ, Hedderley DL. The weight and use of schoolbags in New Zealand secondary schools. Ergonomics. (2001) 44:819–24. doi: 10.1080/00140130117881
58. Luoma K, Riihimäki H, Luukkonen R, Raininko R, Viikari-Juntura E, Lamminen A. Low back pain in relation to lumbar disc degeneration. Spine. (2000) 25:487–92. doi: 10.1097/00007632-200002150-00016
59. Zhang S, Hu B, Liu W, Wang P, Lv X, Chen S, et al. The role of structure and function changes of sensory nervous system in intervertebral disc-related low back pain. Osteoarthritis Cartilage. (2021) 29:17–27. doi: 10.1016/j.joca.2020.09.002
60. Adams MA, Roughley PJ. What is intervertebral disc degeneration, and what causes it? Spine. (2006) 31:2151–61. doi: 10.1097/01.brs.0000231761.73859.2c
61. Raj Arjun SI, Chandrashekara CV, Parth G. Fracture strength estimation of L3-L4 intervertebral disc using FEA. Vibroeng Procedia. (2019) 27:67–72. doi: 10.21595/vp.2019.20976
62. Mackie HW, Legg SJ. Postural and subjective responses to realistic schoolbag carriage. Ergonomics. (2008) 51:217–31. doi: 10.1080/00140130701565588
63. Calvo-Muñoz I, Gómez-Conesa A, Sánchez-Meca J. Prevalence of low back pain in children and adolescents: a meta-analysis. BMC Pediatr. (2013) 13:1–12. doi: 10.1186/1471-2431-13-14
64. Nicolet T, Mannion AF, Heini P, Cedraschi C, Balague F. No kidding: low back pain and type of container influence adolescents’ perception of load heaviness. Eur Spine J. (2014) 23:794–9. doi: 10.1007/s00586-014-3213-2
65. Shymon SJ, Yaszay B, Dwek JR, Proudfoot JA, Donohue M, Hargens AR. Altered disc compression in children with idiopathic low back pain: an upright magnetic resonance imaging backpack study. Spine. (2014) 39:243–8. doi: 10.1097/BRS.0000000000000114
66. Jones GT, Macfarlane GJ. Epidemiology of low back pain in children and adolescents. Arch Dis Child. (2005) 90:312–6. doi: 10.1136/adc.2004.056812
67. Steele E, Bialocerkowski A, Grimmer K. The postural effects of load carriage on young people – a systematic review. BMS Musculosekelet Disord. (2003) 4:1–7. doi: 10.1186/1471-2474-4-1
68. Feingold AJ, Jacobs K. The effect of education on backpack wearing and posture in a middleschool population. Work. (2002) 18:287–94. PMID: 12441569
Keywords: photogrammetry, sagittal plane, lumbar angle, schoolchildren, standing posture, gait, backpack weight
Citation: Bajin M, Kojić M, Romanov R and Ahmetović Z (2022) Neglected problem: Influence of school bag on lumbar segment in children. Front. Pediatr. 10:1045666. doi: 10.3389/fped.2022.1045666
Received: 15 September 2022; Accepted: 27 October 2022;
Published: 15 November 2022.
Edited by:
Jing Wu, Tianjin University, ChinaReviewed by:
Michał Ginszt, Medical University of Lublin, PolandVadim Byvaltsev, Irkutsk State Medical University, Russia
© 2022 Bajin, Kojić, Romanov and Ahmetović. This is an open-access article distributed under the terms of the Creative Commons Attribution License (CC BY). The use, distribution or reproduction in other forums is permitted, provided the original author(s) and the copyright owner(s) are credited and that the original publication in this journal is cited, in accordance with accepted academic practice. No use, distribution or reproduction is permitted which does not comply with these terms.
*Correspondence: Milan Bajin bWlsYW5iYWppbkB5YWhvby5jb20=
Specialty Section: This article was submitted to Children and Health, a section of the journal Frontiers in Pediatrics