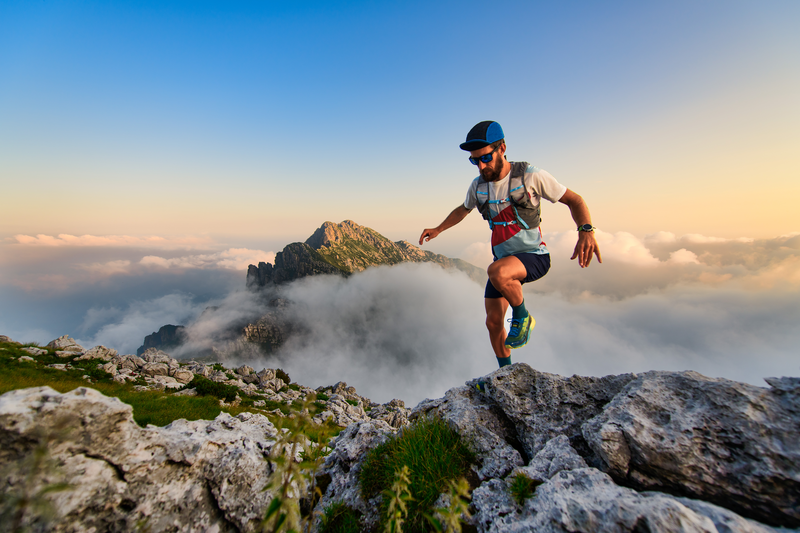
94% of researchers rate our articles as excellent or good
Learn more about the work of our research integrity team to safeguard the quality of each article we publish.
Find out more
REVIEW article
Front. Pediatr. , 19 December 2022
Sec. Pediatric Infectious Diseases
Volume 10 - 2022 | https://doi.org/10.3389/fped.2022.1043251
This article is part of the Research Topic An Update on Pediatric Skeletal System Infections View all 6 articles
Parts of this article's content have been modified or rectified in:
Erratum: Surgery's role in contemporary osteoarticular infection management
The treatment paradigm for osteoarticular infections (OAIs) has changed drastically over the past 80 years, from the advent of penicillin to the use of broad-spectrum antibiotics. Before these drugs, surgery was the only available treatment for OAIs; today, antibiotic therapy is considered the primary response to them. As a result, surgical treatment of OAIs is thus far more rarely indicated, sometimes even considered outdated and obsolete. However, long experience has taught us that many OAI contexts can still benefit from surgical management, constituting an essential complement to medical treatment. The present article seeks to contextualize this discussion by providing a chronological review of the surgical treatments used in cases of OAI and describing the quality of evidence supporting their rehabilitation in well-established situations.
Osteoarticular infections (OAIs) remain very serious affections; they can evolve into major morbidity, including disruptions to subsequent bone development, joint destruction, and permanent articular disability (1–3). Thus, ensuring that clinicians understand an OAI's etiology remains crucial to confirming the diagnosis, prescribing appropriate antibiotic therapy (4), and improving the outcome (5–7). Since the beginning of the 2000s, the widespread use of nucleic acid amplification assays (NAAAs) has substantially improved the detection of low levels of bacteriological agents in clinical samples and has significantly decreased the rate of “culture-negative” OAIs (8, 9). Better recognition of the microbiological causes of infections has drastically changed our understanding of the etiology of OAIs (5–7), concurrently with major changes in diagnostic approaches and therapeutic management.
Despite better overall knowledge of OAIs, some controversies remain concerning which clinical conditions should be treated using surgical procedures. Indeed, there is currently no obvious consensus about the treatment of OAIs, especially about which can be safely treated solely using medicines and which require and will benefit from a surgical approach. All pediatricians and pediatric orthopedists agree that an OAI's clinical presentation and probable outcome will differ depending on its microbiological causes; the treatments required may be diametrically opposed to each other. For example, OAIs due to pyogenic pathogens usually present with a more severe disease course, slower clinical response, and a potentially worse outcome. They may thus require invasive diagnostic and therapeutic procedures and rapid antibiotic treatment (10, 11). OAIs due to Kingella kingae (K. kingae), however, could theoretically be treated with antibiotic therapy alone (5). This literature review attempts to shed light on where to draw the line between cases of OAI for which surgical treatment seems to be the obvious solution and cases in which a surgical procedure would be useless or even excessive.
There are three priority questions when treating a child with an OAI. Does the child need surgery? Which surgical procedure should be considered? Must it be performed urgently? There are also three basic reasons for proposing a surgical intervention for pediatric OAIs: to obtain a bacteriological diagnosis, to ensure control of the infectious source, and to preserve the maximal function of the affected bone segment (12).
Identifying the pathogen responsible for an infection is essential since this is a precondition to tailoring a definitive antibiotic treatment regarding the therapy type, route, and duration (4). Since clinical outcomes may differ depending on the microbiological causes of an OAI, the first phase of a well-managed treatment is identifying the germ and understanding its characteristics. Indeed, identifying the incriminated pathogen will enable, in certain clinical situations, an exploration of whether it will express virulence factors such as antimicrobial genes, exotoxins, genes encoding microbial surface components recognizing adhesive matrix molecules, capsule genes, clonal complexes, or an accessory gene regulator (agr) group (13–18). Although a wide variety of pathogens can cause OAIs, the majority of complications and sequelae are due to Streptococcus species and Staphylococcus aureus (SA). Greater disease severity and complication rates have been described for bacterial isolates expressing the agr III and agr IV loci or exhibiting elevated vancomycin minimum inhibitory concentrations (13–18). In addition, studies from the mid-2000s suggested that MRSA was associated with a more severe infection phenotype than MSSA (19–22). Finally, in the past decade, the worldwide emergence of infections caused by Panton–Valentine leukocidin (PVL)-producing strains has been reported as responsible for multiple severe bone lesions (23). The risk of complications resulting from MSSA or MRSA PVL+ strains is high, with increased risks of subperiosteal abscesses, pyomyositis, and necrosing fasciitis, as well as a high incidence of deep vein thrombosis. Knowledge of pathogen characteristics is therefore crucial since the rate of orthopedic sequelae in the literature varies from 33% to 85%, depending on the strain responsible for the OAI (24).
Early recognition of the pathogen and its virulence factors may also condition the need and indication for complementary surgical treatment. Indeed, with certain pathogens (SA, Streptococcus pneumoniae), surgical treatment may be strongly recommended, if not even unavoidable, especially if they express specific virulence factors. On the contrary, OAIs due to less aggressive pathogens, such as K. kingae, should not require surgical procedures unless it is to exclude the involvement of pyogenic germs. Thus, the need to identify the pathogen responsible for an OAI seems to constitute a sufficient element for performing, at least, an arthrocentesis or a bone punction.
Numerous procedures have been developed to avoid unnecessary surgical procedures for bacterial diagnostic purposes. For children younger than 4 years old (in which more than 90% of OAIs are caused by K. kingae), an alternate, non-invasive diagnostic approach has been proposed involving taking an oropharyngeal swab and subjecting it to a sensitive molecular assay looking specifically for this microorganism. Compatible clinical and biological pictures, coupled with a positive K. kingae-specific NAAA from an oropharyngeal specimen, provide strong evidence that this microorganism is responsible for an OAI (25). However, it should be kept in mind that a positive test is not irrefutable proof of the disease's etiology since around 10% of young children carry the organism. Contrarily, failure to detect K. kingae deoxyribonucleic acid sequences with this sensitive molecular test virtually rules it out as the OAI's etiology (25). This diagnostic strategy is currently used efficiently and routinely worldwide with children under 4 years of age.
Infectious source control is undoubtedly one of the main reasons for considering a surgical procedure. Bacteremia is quite common in acute pediatric osteoarticular infections and may be present in even more than 50% of the cases (26). Controlling the source of an infection necessarily involves preventing and treating bacteremia due to pyogenic pathogens, with a special mention for S. aureus. SA is currently considered as the leading cause of the bacteremia responsible for significant morbidity and mortality, and this regardless of the primary site of infection (27–31). SA bacteremia is, whatever its cause, frequently associated with severe metastatic infections, such as infective endocarditis, septic arthritis, osteomyelitis, and serious complications like sepsis and even septic shock—adverse outcomes that may prove challenging to manage (30, 31). Bacteremia The prevalence of metastatic infections among pediatric patients with SA bacteremia has been estimated to range between 29% and 52% (29). It has also been demonstrated that positive control blood cultures persisting beyond 48 h after the first blood culture triples the possibility of secondary sites of infection (28, 29, 32). Another study demonstrated that the onset of orthopedic complications was related to positive blood cultures and to the persistence of fever for more than 4 days, especially in children whose source control had been delayed (15).
As with most infections, bacterial sources of infection hold to the Latin aphorism “Ubi pus, ibi evacua”, meaning that wherever there is a collection of pus in the body, it should be evacuated as soon as possible, thus avoiding bacteremia, sepsis, and any subsequent complications. Thus, purulent synovial fluid, a bone or subperiosteal abscess, and necrotizing pandiaphysitis are cases in which surgical treatment is not an option but a therapeutic necessity. In these situations, isolated medical treatment will likely lead to therapeutic failure or complications. The best treatment will necessarily involve a surgical procedure aimed at substantially reducing the bacterial load by draining abscess collections and improving blood flow to the infectious site. This last parameter appears to be crucial since it will condition how well antibiotics penetrate infected and ischemic areas, leading to subpotent antibiotic concentrations and, thus, a reduced ability of antimicrobial agents to eradicate infection (33).
As functional impairment appears closely correlated to complications, the preservation of maximal function should focus on their prevention. The onset of complications and subsequent sequelae is thought to be related to inappropriate treatment of acute infections (34). Most authors consider diagnostic and therapeutic delays to be major risk factors for sequelae. Although a 4-day delay has been suggested as “acceptable” for the treatment of uncomplicated osteomyelitis, septic arthritis caused by pyogenic bacteria should be deemed an emergency and thus treated within the first 6–12 h of its onset (35).
Orthopedic complications after OAIs include chronic osteomyelitis, pathological fractures, partial or complete growth arrest with a limb length discrepancy and/or angular deformity, avascular necrosis, articular cartilage degeneration, chronic dislocation, and joint destruction. Few precise figures on the prevalence of post-infectious orthopedic sequelae are currently available. However, it is recognized that chronic osteomyelitis may develop in around 10% of acute osteomyelitis cases due to MSSA (36–39), whereas pathological fractures have been described in approximately 5% of cases sustaining hematogenous acute osteomyelitis (HAO) caused by the same pathogen (15, 40).
However, the worst complications are certainly those due either to an injury of the germinal cells (which can lead to irreversible growth arrest) or to avascular epiphyseal necrosis as a sequela of septic shock, articular septic joint tamponade, or infectious vascular insults. Penetration of the growth plate by a pathogen (transphyseal osteomyelitis) can affect the germinal cells of parts or the entire physis. This can lead to irreversible growth arrest (complete epiphysiodesis) or to an axial deviation of the limb (partial epiphysiodesis). As mentioned, avascular epiphyseal necrosis is probably the most serious issue in osteoarticular infections. Necrosis predominantly affects the epiphyses of large bones, such as the proximal ends of the femur and humerus. In sepsis and septic shock, the normal anticoagulative state within the epiphyseal vasculature is disrupted, resulting in a hypercoagulable state characterized by microvascular thrombi, fibrin deposition, neutrophil extracellular trap (NET) formation, and endothelial injury. Thus, blood shunt and prolonged hypo-oxygenation during every phase of shock and multiorgan failure may explain the hypoperfusion of the epiphysis responsible for avascular necrosis, growth arrest, and epiphyseal dysmorphism. Although these complications were initially linked to meningococcemia, they are now associated with septic shock events caused by other organisms (e.g., Pneumococcus, gram-negative bacilli, Streptococci) that occur in pediatric populations, especially when the infectious source is initially badly controlled.
The course of septic arthritis, whether treated or not, varies substantially depending on the germ that causes it. In cases of septic arthritis caused by a pyogenic microorganism, the clinical course is characterized by an inflammatory cascade. This results in a huge influx of immune cells and neutrophils to the infection site, macrophage activation, and the release of inflammatory cytokines such as IL-1B, IL-16, TNF-a, MIP-2, and granulocyte-macrophage colony-stimulating factor (GM-CSF) (41, 42). Phagocytosis of bacteria by macrophages, synoviocytes, and polymorphonuclear leukocytes will release more lysosomal enzymes and reactive oxygen species and will further induce the liberation of cytokines (43–45). The high levels of cytokines finally induce the release of host matrix metalloproteinases and lysosomal enzymes, which are responsible for further joint degradation (46, 47). It is recognized that cartilage destruction starts to occur as early as 8 h after infection (48), even if intravenous antibiotic therapy is started within the first 24 h of infection, since significant glycosaminoglycan destruction and collagen disruption may be noted very early on during septic arthritis.
Even more worryingly, the antigen-induced inflammatory response may persist and continue to damage the joint architecture after the infection has been cleared (49, 50). In addition to enzymes from bacteria, white cells, and synovium, the cartilage's own chondrocytes may also play a part in its destruction (51). An ultrastructural analysis of chondrocytes in experimentally produced septic arthritis showed an increase in lysosomal electron-dense bodies, suggestive of the production of proteolytic enzymes in both the superficial and deep layers of articular cartilage (51). In addition, it is now recognized that chondrocytes respond to IL-1 by breaking down the surrounding proteoglycan matrix, even in mature cartilage without sepsis (52–54).
However, septic arthritis due to less aggressive pathogens, such as Kingella or Moraxella species, only presents with mild clinical signs and few moderate laboratory findings. When bacteriological diagnostic is certain, most septic arthritis due to these germs would benefit from antibiotic treatment alone without additive surgical procedures.
Recognition of the causal germ is essential because it will condition subsequent therapeutic management. Thus, given the pathophysiological mechanisms of cartilage destruction, it would seem irrational not to carry out surgical irrigation and debridement of an infected native joint, especially when pyogenic pathogens are suspected or clearly involved. The mode of surgical treatment for septic arthritis is itself a source of controversy involving which joint is affected, the suspected pathogen, and the patient's age. Drainage and lavage of a septic joint may be performed rapidly either using a simple syringe injection–aspiration procedure for septic arthritis with minimally inflammatory joint fluid (55), or using arthrotomy, or arthroscopy for joints with significant purulent effusion (56–59).
It is important to remember that before the development of antibiotics, surgery was considered the only therapy available for treating HAO. Surgical procedures revolved around coherent concepts such as trepanation for facilitating pus drainage (60, 61), decreasing the intraosseous pressure responsible for bone infarction, and, above all, debridement to remove all infective and necrotic material (62, 63). Following the introduction of penicillin and broad-spectrum antibiotics, reports described drastically reduced mortality rates; however, many authors noted that the incidence of complications (especially the development of chronic osteomyelitis) was very high when using penicillin alone with the usual supportive measures but without surgical drainage (64, 65). Indeed, these authors concluded that, despite the use of effective antibiotic treatments, surgical treatments still had a crucial role to play, and their experiences resulted in an acceptance that many specific criteria remained formal indications for surgical procedures. They described clinical situations, still relevant today, for which surgical intervention seemed to be appropriate (64, 65). Their guidelines recommended that soft tissue abscesses had to be incised and drained, as did subperiosteal abscesses. Interestingly, they believed the subperiosteal space should be considered as a compartment and that the pressure exerted in that space was considerable in cases of HAO, which could lead to an equivalent of compartment syndrome, interrupting circulation and producing extensive necrosis of the bone (64, 65). Their thinking revolved around the crucial concept that antibiotics could not prevent permanent bone damage once blood supply had been interrupted (64, 65). The guidelines also suggested that metaphyseal bone abscesses should be incised and drained and that metaphyseal bone decompression could decrease the bone pressure capable of interrupting circulation and producing extensive necrosis (64, 65). Finally, they recommended that the medullary canal should be decompressed in patients with continuing bone pain, sepsis, or failure to respond to antibiotic therapy.
Surgical management of HAO remains challenging. Despite abundant publications on this subject, debate continues between those recommending surgery and those considering it excessive. Unfortunately, the debate focuses on whether or not to perform a therapeutic surgical procedure, whereas the right question would be which cases of HAO and, above all, which pathogens require it.
There is growing evidence that surgical drainage is not an option, but it may appear as a real obligation, especially when radiological investigations highlight the presence of pus in the soft tissues, the subperiosteal space, the diaphysis cavity, or even the metaphysis (64–69). Surgical management's role in cases of HAO should be to improve the local environment for antibiotic delivery by decompressing and washing the subperiosteal/metaphyseal abscess cavity, decreasing the bacterial load, and removing devitalized necrotic tissue, thereby preventing the progression of infection to chronic osteomyelitis and lesions of the physis/epiphysis (64–69). Adequate debridement of devitalized tissue can lead to a large bony defect, also called dead space, whose management is crucial, with the objective being to replace dead infected bone with vascularized tissue. The specifications for surgery, therefore, include 6 objectives that medical treatment cannot provide (64–69):
- Draining/decompressing the bone and subperiosteal space
- Reducing the bacterial load
- Removing necrotic tissue
- Improving bone vascularization
- Avoiding physeal/epiphyseal lesions
- Preventing evolution toward chronic osteomyelitis
Surgery thus remains a cornerstone of treatment for children with HAO with subperiosteal, soft tissue, or bone abscesses, especially when they present late after the onset of symptoms. In these cases, prompt surgical decompression with sequential intravenous antibiotics gives the maximum chance of an excellent outcome.
Finally, it is important to note that even the greatest study which validated the medical treatment of osteomyelitis reported the need for surgical procedures; in fact, Peltola et al. affirmed in their paper that “surgery was kept at minimum” and that “most children underwent only the diagnostic percutaneous aspiration or drilling, and only 24% had no surgery” (70).
Indications for the surgical treatment of OAIs also suggest an urgent need for high-quality imaging, which is of paramount importance to confirm the diagnosis, define the degree of infection, plan surgical procedures, and control the response to therapy. Magnetic resonance imaging (MRI) is currently considered the most highly sensitive means of investigating and detecting OAI, especially in its early days. MRI is also probably the best means of detecting soft tissue abscesses. Gadolinium-enhanced sequences help to outline zones of necrosis (71), and are useful for detecting abscesses, whether subperiosteal or intraosseous (67). However, recent reports, demonstrating pathological retention in brain tissue of children exposed to gadolinium-based contrast agents (GBCAs) should encourage radiologists to avoid its unnecessary use in pediatric patients (72–74). MRI can also help to plan treatment, particularly the percutaneous drainage of fluid collections and surgical debridement; it enables an assessment of the extent of necrotic tissue and the definition of dangerous contiguous structures that will need customized management to avoid morbidity and complications (75). In addition, contrast-enhanced sequences of MRI can be used to clearly identify extension of the infection into soft tissues or to detect real childhood pyomyositis.
Thus, we believe it is reasonable to assume that, alongside recognizing the infection's causative germ, MRI is a decisive element in deciding on the need for a surgical procedure.
Emerging technologies such as positron emission tomography (PET)–MRI should improve the diagnosis of bone infections even more (76). However, hybrid PET–MRI scanners are very expensive, and their widespread clinical implementation thus remains problematic. It seems preferable to use MRI as a primary tool for uncomplicated unifocal cases, whereas cases with (potentially) multifocal disease, or a contraindication for MRI, could prefer PET.
The early diagnosis of OAI and the commencement of antimicrobial treatment are crucial to good patient outcomes, as is the control of the infectious focus. This therapeutic approach may be sufficient in certain cases, but it took being risky to want to generalize them to all OAI. In fact, many OAI still require surgical procedures for their diagnosis, focus control, and function preservation.
Open access funding was provided by the University Of Geneva.
GM, DC: participated to the study design, conceived and coordinated the study, collected data, and drafted the manuscript. BC, OV, NG, GM, CS, and RD: collected data, and drafted the manuscript. All authors contributed to the article and approved the submitted version.
The authors declare that the research was conducted in the absence of any commercial or financial relationships that could be construed as a potential conflict of interest.
All claims expressed in this article are solely those of the authors and do not necessarily represent those of their affiliated organizations, or those of the publisher, the editors and the reviewers. Any product that may be evaluated in this article, or claim that may be made by its manufacturer, is not guaranteed or endorsed by the publisher.
The Supplementary Material for this article can be found online at: https://www.frontiersin.org/articles/10.3389/fped.2022.1043251/full#supplementary-material.
1. Gutierrez K. Bone and joint infection. In: Long SS PL, Prober CG, eds. Principles and practice of pediatric infectious disease. 2nd ed. Philadelphia, PA: Churchill Livingstone (2003). p. 467–74.
2. Pääkkönen M, Peltola H. Simplifying the treatment of acute bacterial bone and joint infections in children. Expert Rev Anti-Infect Ther. (2011) 9(12):1125–31. doi: 10.1586/eri.11.140
3. Yagupsky P. Microbiological diagnosis of skeletal system infections in children. Curr Pediatr Rev. (2019) 15:154–63. doi: 10.2174/1573396315666190408114653
4. Luhmann JD, Luhmann SJ. Etiology of septic arthritis in children: an update for the 1990s. Pediatr Emerg Care. (1999) 15(1):40–2. doi: 10.1097/00006565-199902000-00012
5. Coulin B, Demarco G, Spyropoulou V, Juchler C, Vendeuvre T, Habre C, et al. Osteoarticular infection in children. Bone Joint J. (2021) 103(3):578–83. doi: 10.1302/0301-620X.103B3.BJJ-2020-0936.R2
6. Juchler C, Spyropoulou V, Wagner N, Merlini L, Dhouib A, Manzano S, et al. The contemporary bacteriologic epidemiology of osteoarticular infections in children in Switzerland. J Pediatr. (2018) 194:190–6. doi: 10.1016/j.jpeds.2017.11.025
7. Samara E, Spyropoulou V, Tabard-Fougère A, Merlini L, Valaikaite R, Dhouib A, et al. Kingella kingae and osteoarticular infections. Pediatrics. (2019) 144(6):e20191509. doi: 10.1542/peds.2019-1509
8. DeMarco G, Chargui M, Coulin B, Borner B, Steiger C, Dayer R, et al. Kingella kingae osteoarticular infections approached through the prism of the pediatric orthopedist. Microorganisms. (2022) 10(1):25. doi: 10.3390/microorganisms10010025
9. Ceroni D, Dayer R, Steiger C. Are we approaching the end of pediatric culture-negative osteoarticular infections? Future Microbiol. (2019) 14:917–9. doi: 10.2217/fmb-2019-0141
10. Basmaci R, Lorrot M, Bidet P, Doit C, Vitoux C, Penneçot G, et al. Comparison of clinical and biologic features of Kingella kingae and Staphylococcus aureus arthritis at initial evaluation. Pediatr Infect Dis J. (2011) 30(10):902–4. doi: 10.1097/INF.0b013e31821fe0f7
11. Ceroni D, Cherkaoui A, Combescure C, François P, Kaelin A, Schrenzel J. Differentiating osteoarticular infections caused by Kingella kingae from those due to typical pathogens in young children. Pediatr Infect Dis J. (2011) 30(10):906–9. doi: 10.1097/INF.0b013e31821c3aee
12. Arnold JC, Bradley JS. Osteoarticular infections in children. Infect Dis Clin North Am. (2015) 29(3):557–74. doi: 10.1016/j.idc.2015.05.012
13. Cheung GYC, Bae JS, Otto M. Pathogenicity and virulence of Staphylococcus aureus. Virulence. (2021) 12:547–69. doi: 10.1080/21505594.2021.1878688
14. Zhang Y, Xu D, Shi L, Cai R, Li C, Yan H. Association between agr type, virulence factors, biofilm formation and antibiotic resistance of Staphylococcus aureus isolates from pork production. Front Microbiol. (2018) 9:1876. doi: 10.3389/fmicb.2018.01876
15. McNeil JC, Vallejo JG, Kok EY, Sommer LM, Hultén KG, Kaplan SL. Clinical and microbiologic variables predictive of orthopedic complications following Staphylococcus aureus acute hematogenous osteoarticular infections in children. Clin Infect Dis. (2019) 69(11):1955–61. doi: 10.1093/cid/ciz109
16. Kok EY, Vallejo JG, Sommer LM, Rosas L, Kaplan SL, Hulten KG, et al. Association of vancomycin mic and molecular characteristics with clinical outcomes in methicillin-susceptible Staphylococcus aureus acute hematogenous osteoarticular infections in children. Antimicrob Agents Chemother. (2018) 62(5):e00084–18. doi: 10.1128/AAC.00084-18
17. Pichon B, Hill R, Laurent F, Larsen AR, Skov RL, Holmes M, et al. Development of a real-time quadruplex PCR assay for simultaneous detection of nuc, panton-valentine leucocidin (PVL), mecA and homologue mecALGA251. J Antimicrob Chemother. (2012) 67:2338–41. doi: 10.1093/jac/dks221
18. Pérez-Montarelo D, Viedma E, Larrosa N, Gómez-González C, Ruiz de Gopegui E, et al. Molecular epidemiology of Staphylococcus aureus bacteremia: association of molecular factors with the source of infection. Front Microbiol. (2018) 9:2210. doi: 10.3389/fmicb.2018.02210
19. Arnold SR, Elias D, Buckingham SC, Thomas ED, Novais E, Arkader A, et al. Changing patterns of acute hematogenous osteomyelitis and septic arthritis: emergence of community-associated methicillin-resistant Staphylococcus aureus. J Pediatr Orthop. (2006) 26(6):703–8. doi: 10.1097/01.bpo.0000242431.91489.b4
20. Bocchini CE, Hulten KG, Mason EO Jr, Gonzalez BE, Hammerman WA, Kaplan SL. Panton-valentine leukocidin genes are associated with enhanced inflammatory response and local disease in acute hematogenous Staphylococcus aureus osteomyelitis in children. Pediatrics. (2006) 117(2):433–40. doi: 10.1542/peds.2005-0566
21. Carrillo-Marquez MA, Hulten KG, Hammerman W, Mason EO, Kaplan SL. USA 300 is the predominant genotype causing Staphylococcus aureus septic arthritis in children. Pediatr Infect Dis J. (2009) 28(12):1076–80. doi: 10.1097/INF.0b013e3181adbcfe
22. Hawkshead JJ 3rd, Patel NB, Steele RW, Heinrich SD. Comparative severity of pediatric osteomyelitis attributable to methicillin-resistant versus methicillin-sensitive Staphylococcus aureus. J Pediatr Orthop. (2009) 29(1):85–90. doi: 10.1097/BPO.0b013e3181901c3a
23. Del Giudice P, Tattevin P, Etienne J. Community-acquired methicillin-resistant Staphylococcus aureus: review. Presse Med. (2012) 41(7–8):713–20. doi: 10.1016/j.lpm.2011.10.022
24. lharreborde B. Sequelae of pediatric osteoarticular infection. Orthop Traumatol Surg Res. (2015) 101(S1):S129–37. doi: 10.1016/j.otsr.2014.07.029
25. Ceroni D, Dubois-Ferriere V, Cherkaoui A, Renzi G, Combescure C, Lamah L, et al. Detection of Kingella kingae osteoarticular infections in children by oropharyngeal swab PCR. Pediatrics. (2013) 131(1):e230–5. doi: 10.1542/peds.2012-0810
26. Pääkkönen M, Kallio MJ, Kallio PE, Peltola H. CRP vs ESR, WBC and ALP in diagnosing bacteremia in bone and joint infections. J Paediatr Child Health. (2013) 49:189–92. doi: 10.1111/jpc.12122
27. Vogel M, Schmitz RP, Hagel S, Pletz MW, Gagelmann N, Scherag A, et al. Infectious disease consultation for Staphylococcus aureus bacteremia – a systematic review and meta-analysis. J Infect. (2016) 72(1):19–28. doi: 10.1016/j.jinf.2015.09.037
28. Praino ML, Neyro SE, Procopio A, Vázquez M, Rey S, Marone S, et al. Localización metastásica en niños con bacteriemia por Staphylococcus aureus. Arch Argent Pediatr. (2012) 110(4):331–4. doi: 10.5546/aap.2012.331
29. Praino ML, Neyro SE, Procopio A, Vázquez M, Rey S, Marone S, et al. Metastatic infections in pediatric patients with Staphylococcus aureus bacteremia assisted at a children's hospital in La Plata, Argentina. Arch Argent Pediatr. (2021) 119(6):408–13. doi: 10.5546/aap.2021.eng.408
30. Shorr AF, Tabak YP, Killian AD, Gupta V, Liu LZ, Kollef MH. Healthcare-associated bloodstream infection: a distinct entity? Insights from a large U.S. database. Crit Care Med. (2006) 34(10):2588–95. doi: 10.1097/01.CCM.0000239121.09533.09
31. Wyllie DH. Mortality after Staphylococcus aureus bacteraemia in two hospitals in oxfordshire, 1997-2003: cohort study. Br Med J. (2006) 333(7562):281. doi: 10.1136/bmj.38834.421713.2F
32. Le J, Dam Q, Tran T, Nguyen A, Adler-Shohet FC, Kim S, et al. Epidemiology and hospital readmission associated with complications of Staphylococcus aureus bacteremia in pediatrics over a 25- year period. Epidemiol Infect. (2017) 145(12):2631–9. doi: 10.1017/S0950268817001571
33. Anwar H, Dasgupta MK, Costerton JW. Testing the susceptibility of bacteria in biofilms to antibacterial agents. Antimicrob Agents Chemother. (1990) 34(11):2043–6. doi: 10.1128/AAC.34.11.2043
34. Wald ER. Risk factors for osteomyelitis. Am J Med. (1985) 78(6b):206–12. doi: 10.1016/0002-9343(85)90386-9
35. Herman MJ, James McCarthy J, Willis RB, Pizzutillo PD. Top 10 pediatric orthopaedic surgical emergencies: a case-based approach for the surgeon on call. Instr Course Lect. (2011) 60:373–95.21553787
36. Zhang T, Yu S, Lv X, Gan Y, Luo Y, Li T. Paediatric osteomyelitis and septic arthritis pathogen distribution and antimicrobial resistance in a single centre: a 15-year retrospective analysis. J Trop Pediatr. (2022) 68(3):fmac038. doi: 10.1093/tropej/fmac038
37. Dich VQ, Nelson JD, Haltalin KC. Osteomyelitis in infants and children: a review of 163 cases. Am J Dis Child. (1975) 129(11):1273–8. doi: 10.1001/archpedi.1975.02120480007004
38. Martínez-Aguilar G, Avalos-Mishaan A, Hulten K, Hammerman W, Mason EO Jr, Kaplan SL. Community-acquired, methicillin-resistant and methicillin-susceptible Staphylococcus aureus musculoskeletal infections in children. Pediatr Infect Dis J. (2004) 23(8):701–6. doi: 10.1097/01.inf.0000133044.79130.2a
39. Riise ØR, Kirkhus E, Handeland KS, Flatø B, Reiseter T, Cvancarova M, et al. Childhood osteomyelitis-incidence and differentiation from other acute onset musculoskeletal features in a population-based study. BMC Pediatr. (2008) 8:45. doi: 10.1186/1471-2431-8-45
40. Belthur MV, Birchansky SB, Verdugo AA, Mason EO Jr, Hulten KG, Kaplan SL, et al. Pathologic fractures in children with acute Staphylococcus aureus osteomyelitis. J Bone Joint Surg Am. (2012) 94(1):34–42. doi: 10.2106/JBJS.J.01915
41. Ross K, Mehr J, Carothers B, Greeley R, Benowitz I, McHugh L, et al. Outbreak of septic arthritis associated with intra-articular injections at an outpatient practice. Morb Mortal Wkly Rep. (2017 Jul 28) 66(29):777–9. doi: 10.15585/mmwr.mm6629a3
42. Shirtliff ME, Mader JT. Acute septic arthritis. Clin Microbiol Rev. (2002) 15(4):527–44. doi: 10.1128/CMR.15.4.527-544.2002
43. Nade S. Septic arthritis. Best Pract Res Clin Rheumatol. (2003) 17(2):183–200. doi: 10.1016/S1521-6942(02)00106-7
44. Colavite PM, Sartori A. Septic arthritis: immunopathogenesis, experimental models and therapy. J Venom Anim Toxins Incl Trop Dis. (2014) 20:1–8. doi: 10.1186/1678-9199-20-19
45. Bremell T, Abdelnour A, Tarkowski A. Histopathological and serological progression of experimental Staphylococcus aureus arthritis. Infect Immun. (1992) 60(7):2976–85. doi: 10.1128/iai.60.7.2976-2985.1992
46. Kanangat S, Postlethwaite A, Hasty K, Kang A, Smeltzer M, Appling W, et al. Induction of multiple matrix metalloproteinases in human dermal and synovial fibroblasts by Staphylococcus aureus: implications in the pathogenesis of septic arthritis and other soft tissue infections. Arthritis Res Ther. (2006) 8(6):R176. doi: 10.1186/ar2086
47. Nair R, Schweizer ML, Singh N. Septic arthritis and prosthetic joint infections in older adults. Infect Dis Clin North Am. (2017) 31(4):715–29. doi: 10.1016/j.idc.2017.07.013
48. Roy S, Bhawan J. Ultrastructure of cartilage in pyogenic arthritis. Arch Pathol. (1975) 99(1):44–7.1111494
49. Smith RL, Schurman DJ. Bacterial arthritis. A staphylococcal proteoglycan-releasing factor. Arthritis Rheum. (1986) 29(11):1378–86. doi: 10.1002/art.1780291111
50. Smith RL, Schurman DJ, Kajiyama G, Mell M, Gilkerson E. The effect of antibiotics on the destruction of cartilage in experimental infectious arthritis. J Bone Joint Surg Am. (1987) 69(7):1063–8. doi: 10.2106/00004623-198769070-00015
51. Wang J, Wang L. Novel therapeutic interventions towards improved management of septic arthritis. BMC Musculoskelet Disord. (2021) 22(1):530. doi: 10.1186/s12891-021-04383-6
52. McGuire-Goldrins MB, Meats JE, Wood DD, Ihrie EJ, Ebsworth NM, Russell RG. In vitro activation of human interleukin-1-like factor. Arthritis Rheum. (1984) 27(6):654–2. doi: 10.1002/art.1780270608
53. Jasiu HE. Bacterial lipopolysaccharides induce in vitro degradation of cartilage matrix through chondrocyte activation. J Clin Invest. (1983) 72(6):2014–9. doi: 10.1172/JCI111166
54. Gower M, Wood DD, Ihrie EJ, Meats JE, Russell RG. Stimulation by human interleukin-1 of cartilage breakdown and production of collagenase and proteoglyconase by human chondrocytes but not osteoblasts. Biochim Biophys Acta. (1984) 797(2):186–93. doi: 10.1016/0304-4165(84)90121-1
55. Iliadis AD, Ramachandran M. Paediatric bone and joint infection. EFORT Open Rev. (2017) 2(1):7–12. doi: 10.1302/2058-5241.2.160027
56. Donders CM, Spaans AJ, Bessems JHJM, van Bergen CJA. A systematic review of the optimal drainage technique for septic hip arthritis in children. Hip Int. (2022) 32(5):685–93. doi: 10.1177/1120700021989666
57. Donders CM, Spaans AJ, Bessems JHJM, van Bergen CJA. Arthrocentesis, arthroscopy or arthrotomy for septic knee arthritis in children: a systematic review. J Child Orthop. (2021) 15:48–54. doi: 10.1302/1863-2548.15.200129
58. Sanchez AA, Hennrikus WL. Arthroscopically assisted treatment of acute septic knees in infants using the micro-joint arthroscope. Arthroscopy. (1997) 13:350–4. doi: 10.1016/S0749-8063(97)90033-5
59. Agout C, Lakhal W, Fournier J, de Bodman C, Bonnard C. Arthroscopic treatment of septic arthritis of the knee in children. Orthop Traumatol Surg Res. (2015) 101:S333–6. doi: 10.1016/j.otsr.2015.09.007
60. Amberg S, Ghormley RK. Osteomyelitis among children. J Pediatr. (1934) 5:177–93. doi: 10.1016/S0022-3476(34)80163-1
62. Costa AN. Acêrca do tratamento da osteomielite aguda dos adolescentes. Coimbra Méd. (1936) 8:579–86.
64. Winters JL, Cahen I. Acute hematogenous osteomyelitis: a review of sixty-six cases. J Bone Joint Surg Am. (1960) 42:691–704. doi: 10.2106/00004623-196042040-00013
65. Epps CH Jr, Granger S. Acute hematogenous osteomyelitis. a report of five years experience. J Natl Med Assoc. (1963) 55(5):389–93.14049551
66. Darville T, Jacobs RF. Management of acute hematogenous osteomyelitis in children. Pediatr Infect Dis J. (2004) 23:255–7. doi: 10.1097/01.inf.0000115629.52543.d0
67. Kaplan S. Acute hematogenous osteomyelitis in children: differences in clinical manifestations and management. Pediatr Infect Dis J. (2010) 29:1128–9. doi: 10.1097/INF.0b013e3181f55a75
68. Calhoun JH, Manring MM, Shirtliff M. Osteomyelitis of the long bones. Semin Plast Surg. (2009) 23(2):59–72. doi: 10.1055/s-0029-1214158
69. Kulkarni SL, Mannual S, Patil N, Daragad M. Study to evaluate the role of surgical management in acute osteomyelities in children. Indian J Health Sci Biomed Res. (2022) 15:26–33. doi: 10.4103/kleuhsj.kleuhsj_352_21
70. Peltola H, Pääkkönen M, Kallio P, Osteomyelitis-Septic Arthritis Study Group. Short- versus long-term antimicrobial treatment for acute hematogenous osteomyelitis of childhood: prospective, randomized trial on 131 culture positive cases. Pediatr Infect Dis J. (2010) 29:1123–8. doi: 10.1097/INF.0b013e3181f55a89
71. Kocher MS, Lee B, Dolan M, Weinberg J, Shulman ST. Pediatric orthopedic infections: early detection and treatment. Pediatr Ann. (2006) 35:112–22. doi: 10.3928/0090-4481-20060201-11
72. Ozturk K, Nascene D. Effect of at least 10 serial gadobutrol administrations on brain signal intensity ratios on T1-weighted MRI in children: a matched case-control study. Am J Roentgenol. (2021) 217(3):753–60. doi: 10.2214/AJR.20.24536
73. Ryu YJ, Choi YH, Cheon JE, Lee WJ, Park S, Park JE, et al. Pediatric brain: gadolinium deposition in dentate nucleus and globus pallidus on unenhanced T1-weighted images is dependent on the type of contrast agent. Invest Radiol. (2018) 53(4):246–55. doi: 10.1097/RLI.0000000000000436
74. Sambri A, Spinnato P, Tedeschi S, Zamparini E, Fiore M, Zucchini R, et al. Bone and joint infections: the role of imaging in tailoring diagnosis to improve patients’ care. J Pers Med. (2021) 11:1317. doi: 10.3390/jpm11121317
75. Stanescu AL, Shaw DW, Murata N, Murata K, Rutledge JC, Maloney E, et al. Brain tissue gadolinium retention in pediatric patients after contrast-enhanced magnetic resonance exams: pathological confirmation. Pediatr Radiol. (2020) 50(3):388–96. doi: 10.1007/s00247-019-04535-w
Keywords: osteoarticular, infection, surgery, management, punction, drainage, necrotic, abscess
Citation: De Marco G, Vazquez O, Gavira N, Ramadani A, Steiger C, Dayer R and Ceroni D (2022) Surgery's role in contemporary osteoarticular infection management. Front. Pediatr. 10:1043251. doi: 10.3389/fped.2022.1043251
Received: 13 September 2022; Accepted: 23 November 2022;
Published: 19 December 2022.
Edited by:
Dimitri Van der Linden, Cliniques Universitaires Saint-Luc, BelgiumReviewed by:
Pierre-Louis Docquier, Université Catholique de Louvain, Belgium© 2022 De Marco, Vazquez, Gavira, Ramadani, Steiger, Dayer and Ceroni. This is an open-access article distributed under the terms of the Creative Commons Attribution License (CC BY). The use, distribution or reproduction in other forums is permitted, provided the original author(s) and the copyright owner(s) are credited and that the original publication in this journal is cited, in accordance with accepted academic practice. No use, distribution or reproduction is permitted which does not comply with these terms.
*Correspondence: Dimitri Ceroni ZGltaXRyaS5jZXJvbmlAaGN1Z2UuY2g=
Specialty Section: This article was submitted to Pediatric Infectious Diseases, a section of the journal Frontiers in Pediatrics
Disclaimer: All claims expressed in this article are solely those of the authors and do not necessarily represent those of their affiliated organizations, or those of the publisher, the editors and the reviewers. Any product that may be evaluated in this article or claim that may be made by its manufacturer is not guaranteed or endorsed by the publisher.
Research integrity at Frontiers
Learn more about the work of our research integrity team to safeguard the quality of each article we publish.