- 1Pediatric Critical Care Medicine, Children’s Mercy Hospital, University of Missouri-Kansas City School of Medicine, Kansas City, MO, United States
- 2Division of Critical Care, Department of Pediatrics, Baylor College of Medicine, Houston, TX, United States
- 3Texas Children’s Hospital, Houston, TX, United States
- 4Department of Biomedical Engineering, Texas Children's Hospital, Houston, TX, United States
The metabolic response to injury and stress is characterized initially by a decreased energy expenditure (Ebb phase) followed by an increased metabolic expenditure (Flow phase). Indirect calorimetry is a methodology utilized to measure energy expenditure and substrate utilization by measuring gas exchange in exhaled air and urinary nitrogen. The use of indirect calorimetry in critically ill patients requires precise equipment to obtain accurate measurements. The most recent guidelines suggested that measured energy expenditure by indirect calorimetry be used to determine energy requirements. This article reviews the methodological and clinical use of indirect calorimetry in critically ill pediatric patients.
Introduction
The metabolic response to injury and stress, from a critical illness, is represented by systemic manifestations. The initial response after an injury is characterized by a decrease in metabolic expenditure (Ebb phase) during the first 2–3 days, this response is intended to preserve energy; it is immediately followed by a flow phase, where there is a considerable increase in metabolic expenditure and a catabolic response that is proportional to the initial injury (1–9). The measurement of metabolic expenditure and the changes in the oxidation of carbohydrates, proteins, and lipids in a critically ill patient can be obtained by using the indirect calorimetry method (10–13).
Methodology for the measurement of metabolic expenditure
Indirect calorimetry: Gas exchange method
Indirect calorimetry (IC) is a method used to measure metabolic expenditure and substrate utilization through the measurement of gas exchange of exhaled air and urinary nitrogen. This method is based on 3 premises: (1) inspired oxygen (O2) is used in its totality to oxidize different substrates, (2) exhaled carbon dioxide (CO2) is a product of the complete combustion of these substrates, and, (3) non-protein nitrogen deposed in urine is a product of free amino acids oxidation (14–18).
The gas exchange methodology measures oxygen consumption (VO2) and carbon dioxide production (VCO2) by quantifying the differences of O2 and CO2 between inspired and exhaled gas. The following formulas include the Haldane transformation:
FEO2 is the concentration of expired O2, FIO2 is the concentration of inspired oxygen, FECO2 is the concentration of expired CO2, and VE is the expired volume of air over time in milliliters per minute (ml/min).
The values for VO2 and VCO2 are converted into a caloric equivalent of resting metabolic rate (RMR) using formulas created by Weir (18):
The RMR is adjusted by taking into consideration the protein metabolism which is quantified by using the total urinary nitrogen (TUN) level over 24 h (19, 20):
These values are used to calculate the oxidation indexes of different substrates (carbohydrates, protein, and lipids) by using Conzolazio's constants; the TUN level is measured over 24 h (21). The measured gas exchange represents the composition of oxidized substrates, 1 kcal requires 0.236, 0.214, and 0.199 L of oxygen to oxidize protein, carbohydrates, and lipids respectively. Similarly, 1 kcal produces 0.190, 0.151, and 0.199 L of carbon dioxide from the oxidation of protein, carbohydrates, and lipids respectively. When oxidative reactions occur in the human body, energy is released in corresponding units of adenosine triphosphate (ATP). For every 1 mol of oxidized glucose, palmitate, and amino acids, 3.00, 2.85, and 2.25 units of ATP are produced respectively. These values help explain a fundamental concept of physiology: the most efficient way to utilize oxygen in the production of energy (adenosine triphosphate) is via the oxidation of glucose; the oxidation of lipids and protein requires higher levels of oxygen. It is important to clarify that by simultaneously using IC and TUN, it is possible to calculate a “net” oxidative index of carbohydrates, proteins, and lipids (19–23). Nitrogen excretion in the urine measured as urea nitrogen has been shown to be highly variable in critically ill adults and children (23–26), therefore, it is recommended to measure total urinary nitrogen by the Kjeldahl method to obtain accurate measurements and minimize variability (20, 22, 27).
The VCO2/VO2 relationship represents the respiratory quotient (RQ) and its value is constant and unique for each substrate, it is important to point out that the RQ does not represent a change in the absolute values of VO2 and VCO2, which are indices that impact the cardiopulmonary function. The corresponding RQ values for the net oxidation of carbohydrates, protein, and lipids are 1.00, 0.80, and 0.70 respectively. The RQ index that does not involve protein contribution (npRQ) has a value of 0.70–1.0, where values >1.0 indicate the synthesis of fat from glucose substrate, a process called lipogenesis. Several clinical trials in patients admitted to the pediatric critical care unit (PICU) have reported that fluctuations in metabolism or an excessive glucose caloric intake, may alter npQR values (22, 28–32).
The relative percentage of metabolized substrates is obtained using the following formulas:
(1) Carbohydrates (%): [(carbohydrates + lipids)/corrected RMR] × [(97.4 npRQ—68.9)/(59.4 npRQ—30.8)]
(2) Lipids (%): [(carbohydrates + lipids)/corrected RMR] × [[38.1 (1-npRQ)]/(59.4 npRQ—30.8)]
(3) Proteins (%): Protein/corrected RMR. Protein = 6.25 × 4.2 × UUN (g/day).
Methodology for the quantification of metabolic expenditure
Metabolic expenditure calculations may be obtained by using either an open or closed system.
Open circuit method
The patient is connected to a spirometry system with 100% oxygen via a mouth adaptor, facial mask, or endotracheal tube, which is then connected to a kymograph. During the patient's inspiration and exhalation, oxygen is consumed, and carbon dioxide is produced. The carbon dioxide and water vapor are absorbed by the system, therefore, the changes in volume detected by the spirometer represent the consumption of oxygen by the lungs of the subject. This technique is not free of errors, and these may result in incorrect metabolic measurements. Another limitation of this system is its short measurement time (generally <10 min), which is not long enough for oxygen and carbon dioxide deposits to stabilize in the human body. Additionally, the metabolic consequences of breathing 100% oxygen on carbon dioxide absorption via soda lime have not been well documented. Currently is no longer used in critically ill patients.
Closed circuit method
This method has been used for the continuous quantification of metabolic expenditure in both subjects with spontaneous respiration and mechanical ventilation. During the measurement, the patient's head is positioned in a transparent plastic chamber with no leaks (canopy) and breathing room air at a constant flow. As the patient exhales, gas is collected in a mixing chamber, and the values of oxygen and carbon dioxide are quantified. The subject's VO2 and VCO2 are calculated by multiplying the airflow in ml/min (room air at a 0.21 oxygen concentration) by the absolute values of the differences in concentrations between O2 and CO2 in inspired air minus exhaled air. A key factor to consider when using this method is that higher airflow will decrease the differences of O2 between inhaled and exhaled air, therefore the equipment used to analyze O2 and CO2 levels must have the appropriate sensitivity to detect small differences in exhaled and inhaled air.
Using indirect calorimetry during mechanical ventilation
The use of IC for the measurement of VO2 and VCO2 during mechanical ventilation requires of a full understanding of the mechanical ventilator and metabolic equipment being utilized (33). The most important components of the metabolic system are the volume measurement devices (pneumotachograph, turbine, hot wire, ultrasonic, etc.) and the gas analyzers for oxygen and carbon dioxide (paramagnetic, zirconia, fuel cell, mass spectrometry, etc.); therefore, metabolic systems must-have components of high precision to obtain acceptable measurements. The current metabolic systems include precise gas analyzers with faster response time (zirconia, fuel cell, paramagnetic, etc.) and advanced electronic components; this has allowed to improve the precision and facilitate the process to obtain these measurements. The two most used systems to measure metabolic expenditure in patients receiving mechanical ventilation are (1) Mixing-chamber (Figure 1) and (2) Breath-by-breath technique (Figure 2), the last system requires fast response gas analyzers and high precision volume measurement devices. The main features that any metabolic system should have to measure metabolic expenditure in patients on mechanical ventilation are listed in Table 1.
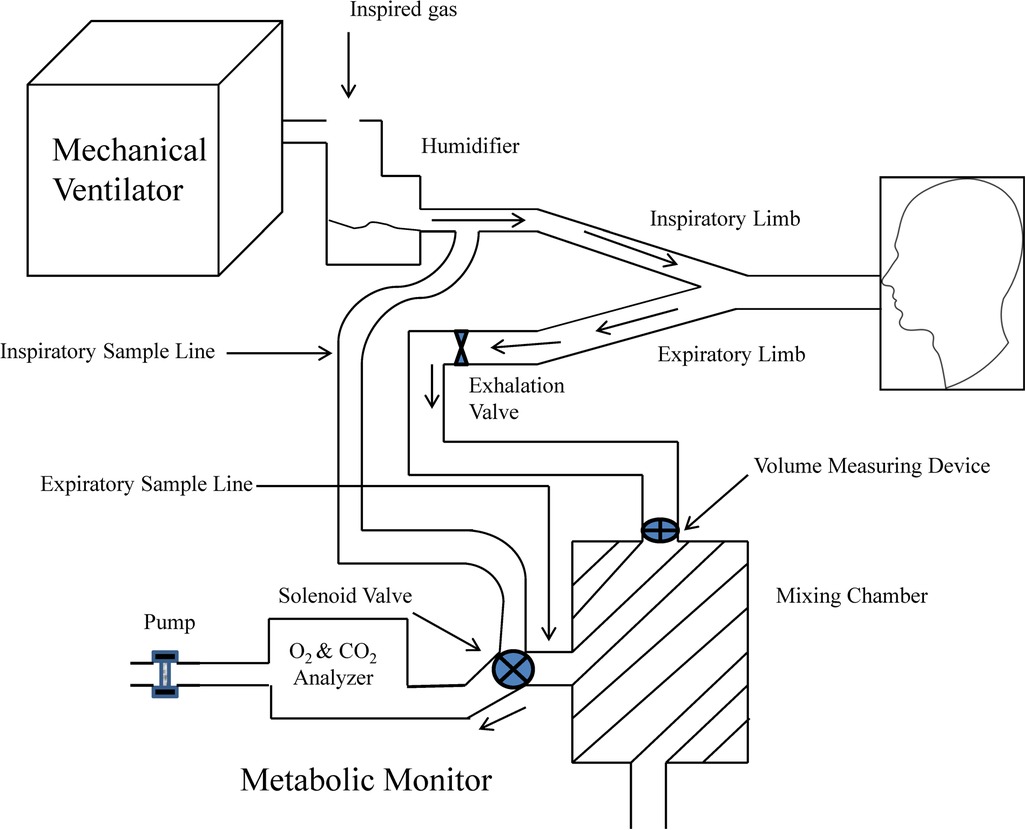
Figure 1. Graphic representation of metabolic equipment with mixing-chamber and its interface with mechanical ventilation. Modified from Weissman and Kemper (33).
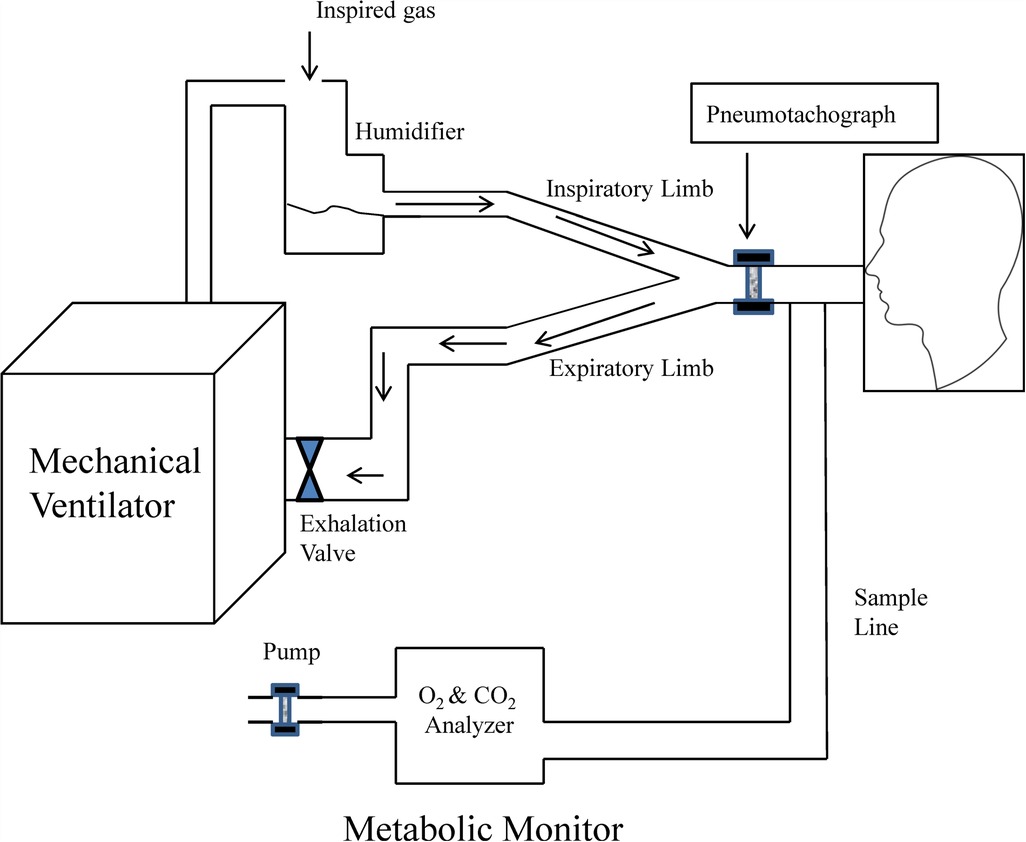
Figure 2. Graphic representation of metabolic equipment with breath-by-breath analysis and its interface with mechanical ventilation. Modified from Weissman and Kemper (33).
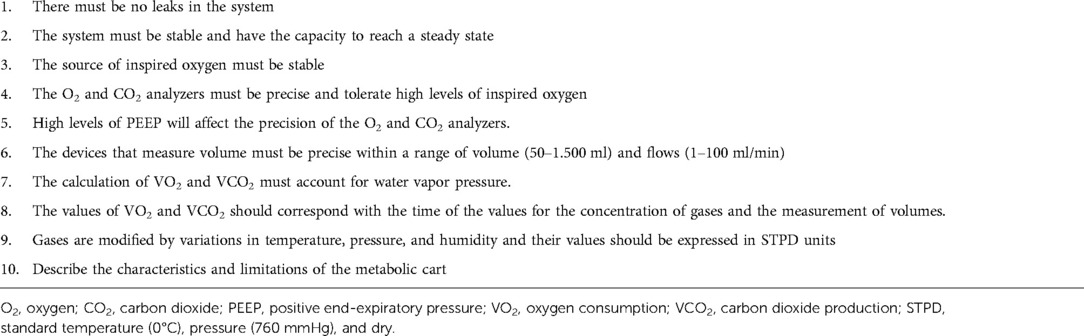
Table 1. Characteristics of the metabolic system used for the measurement of energy expenditure during mechanical ventilation.
The appropriate interpretation of the measured parameters of IC requires an understanding of the premises and technical aspects of this methodology. The continued advancement of technology and the manufacturing of highly precise portable metabolic carts have made the use of indirect calorimetry in critically ill patients a reality, this includes subjects on mechanical ventilation. There are several factors that can make the real-world application and interpretation of this methodology difficult in an intensive care unit, among these are: (1) the calculation model, (2) calorimetric factors, (3) leak around the endotracheal tube, (4) fraction of inspired oxygen above 0.60, (5) elevated levels of positive end-expiratory pressure (PEEP), generally >10 cm H2O, (6) limited stability of gas analyzers, (7) inability to reach a steady state, and (8) human factors. The duration of the indirect calorimetry study is extremely important when measuring metabolic expenditure to reach a steady state, this is defined as a variation coefficient [(standard deviation/average) × 100] less than 10% which represents an equivalent to energy expenditure over 24 h. Clinical studies in critically ill adults and children have found that a shorter duration of indirect calorimetry measurements ranging from 3 to 5 min is adequate to reach a steady state with precise findings, from a clinical standpoint; other authors have found that indirect calorimetry measurements lasting 30 min may correspond to 24-h studies.
The total energy expenditure (TEE) in healthy subjects can be divided into three components: basal energy expenditure (BEE), thermogenesis, and physical work. The BEE represents 60%–70% of the TEE, thermogenesis equals 10% and physical work corresponds to 20%–30% of the TEE. During times of stress or injury, the values of these components can change in relation to the degree of injury, diet, and physical activity. Diet-induced thermogenesis (DIT) is defined as the amount of energy required to absorb, process and store nutrients and is equal to the increase in energy expenditure as it relates to the post-absorption state. Doubling the intake of calories compared to the REE during the acute phase of disease produces a 10%–20% increase in the DIT, with an increase in VO2 and VCO2 that will be reflected in the increased ventilatory support and stress on the cardiovascular system in the critically ill patient (27, 32, 34–39); which is why the current recommendations are to not administer an excess of calories compared to REE during the acute disease phase or immediate post-operative phase unless an indirect calorimetry study has been performed (3, 32, 38–41).
The possible benefits of using indirect calorimetry in critically ill patients are (1) quantification of energy expenditure in patients unresponsive to medical management whose nutritional plan is based solely on estimated energy requirement formulas, (2) measurement of energy expenditure in critically ill patients with organ failure and prolonged use of parenteral nutrition, (3) to evaluate the effects of parenteral nutrition on the respiratory system of patients with acute and chronic respiratory failure on ventilatory support, and (4) to measure the consumption of oxygen during the weaning phase of respiratory support. An article by Mehta et al. (32), described indications for the measurement of resting energy expenditure (REE) in the pediatric intensive care unit: these recommendations are incorporated into the clinical guidelines for nutritional support of the critically ill pediatric patient, published by the American Society of Parenteral and Enteral Nutrition (A.S.P.E.N.) (39, 41). In conclusion, indirect calorimetry allows us to understand the process of energy utilization during periods of acute stress and injury, although this has not been shown to improve outcomes in clinical studies. More research is needed to analyze the benefits of indirect calorimetry, its uses in calculating energy requirements, preventing overfeeding or underfeeding, and its cost-benefit relationship in the critical care unit.
Other methods to quantify energy expenditure
Stable isotopes
Procedures used to measure energy expenditure in humans include indirect and direct calorimetry, stables isotopes, 24-h cardiac frequency monitoring, and the use of monitors to measure physical activity. Direct calorimetry quantifies the dissipation of heat during a state of rest, where the heat that is lost is equal to the energy that is produced. Indirect calorimetry quantifies heat produced based on gas exchange and calculated energy expenditure at rest. Total energy expenditure (TEE) considers physical activity and can be measured by using doubly labeled water. This method uses stable isotopes (2H2O, H218O, NaH13CO3) to quantify energy expenditure (42–46).
The method for using doubly labeled water with stable isotopes was developed 50 years ago and is based on the differences in the production of 2H2O and H218O in the fluid compartment of the human body (47–49). After a period of equilibrium, 2H and 18O dissolve in this fluid compartment, while 18O is eliminated via respiration in the form of carbon dioxide. The VCO2 is calculated by subtracting the difference in the production of both isotopes. By using a respiratory coefficient (VCO2/VO2) of 0.85, the metabolic expenditure is estimated by using the values for VO2 and VCO2. This doubly labeled water technique has been validated against indirect calorimetry and is now considered the gold standard for measuring TEE in ambulatory subjects. The sources of error using this method are due to analytical errors of the mass spectrophotometer when measuring the percentage of enrichment of the isotopes, biological variability in the enrichment of the isotopes, division of the isotope during the production of carbon dioxide and water vapor, total body water measurement and the use of an estimated or calculated 24-h respiratory coefficient. It is impossible to implement this technique with patients in the critical care unit given their constant changes in fluid over various body compartments, hence it is reserved for outpatient measurements.
Previously, the production of carbon dioxide during respiration has served as an index for substrate oxidation and energy expenditure. The isotopic dilution technique consists of the incorporation and dilution of the 13C marker in bicarbonate which is marked with the isotope NaH13CO3 (50–55). The quantification of the degree of dilution of the isotope in the exhaled air or in the blood is used to calculate the level of carbon dioxide production. Energy expenditure calculation requires the quantity of released energy per liter of carbon dioxide production or the energy equivalents of CO2 (Eq E CO2) that reflect the coefficient value of the dietary intake (56). Two fundamental factors that may affect this technique are (1) errors in the measurement of labeled bicarbonate and (2) the possibility that labeled bicarbonate may not dissolve with the CO2 produced in the mitochondria. Requiring a precise knowledge of the bicarbonate correction factors necessary for physiologic function (fractional recovery) is another limitation. Several clinical studies have estimated fractional recovery of premature neonates and pediatric patients in critical care between 0.63 and 0.95. It is necessary to emphasize the importance of quantifying fractional recovery values of bicarbonate according to the specific populations being studied, without solely relying on values obtained in adults. In conclusion, isotopic methods and indirect calorimetry are considered complementary methods since the former requires VCO2 which is measured using the latter.
Fick method
In patients with thermodilution catheters in the pulmonary artery, we can measure VO2 and VCO2 through the measurement of cardiac output (CO) and differences in arterial and venous oxygen content and carbon dioxide by using the Fick method. The Fick method is based on the formula: VO2 Fick = CO × [(SaO2 – SvO2) × Hb × 0.0139 + (PaO2 – PVO2)× 0.003], CO is cardiac output (L/min); Hb is the value of hemoglobin (g/L); SatO2 and SVO2 correspond to oxygen saturation (%) of arterial and mixed venous blood; PaO2 and PVO2 represent partial pressure of oxygen (torr) in arterial and mixed venous blood, respectively. The problems related to a precise measurement using the Fick Method regarding the quantification of VO2, include: (1) values lower than the total oxygen consumption since this method does not take into consideration the VO2 of bronchial and Thebesian veins, (2) it may not show changes in VO2 during the initial stages of injury, and (3) the formulas for calculation of oxygen consumption and oxygen transport are mathematically coupled.
Some research studies have concluded that, compared to the Fick Method, indirect calorimetry is the non-invasive method of choice for measuring VO2 in adults. Nowadays, the use of the Fick Method in critically ill children is limited since there are few indications for the placement of pulmonary artery thermodilution catheters and their insertion is technically difficult in small children.
Methods for calculating caloric needs
Critically ill pediatric patients admitted to an ICU have different caloric needs when compared to healthy children; in relation to their metabolism, growth index, comorbidities, and preexisting energy reserves; hence it is difficult to evaluate the metabolic requirements of this patient population. Therefore, the serial monitoring of their metabolic energy expenditure during the course of the disease is of utmost importance. When you do not have access to the specific equipment required to measure energy expenditure in a pediatric patient in the ICU you may cautiously use reference values to estimate their caloric needs.
Reference values for individuals less than 18 years old
The reference values commonly recommended for estimating basal energy expenditure in children and adolescents include:
1. Harris-Benedict Equation: (16) published in 1919 it is one of the most commonly used equations to estimate basal energy expenditure; it was derived from measurements performed on 94 newborns and 239 individuals (136 men and 103 women) older than 16 years of age. This equation has never been validated in pediatric patients.
2. Formulas published by the Food and Agriculture Organization of the United Nations (FAO), and the World Health Organization (WHO) of the United Nations (57); these formulas were used in clinical studies including 6,100 participants.
3. Talbot published his equation for calculating basal energy expenditure in 1938, based on measurements performed on 3,000 pediatric subjects; 2,220 females and 800 males (17).
4. Schofield Equations (58); are based on the results of the clinical study performed by the FAO and WHO. It's important to mention that, due to the variability of the metabolic state of critically ill children during their ICU stay, these reference values may result in an overestimation or underestimation of the patient's actual energy requirements. This was demonstrated by Kyle et al. (59), by showing that in a group of 240 critically ill children admitted to an ICU for longer than 48 h, a negative metabolic balance during the first 8 days of the ICU stay was obtained by using the values from the Schofield Equation.
Correction factors
With the use of correction factors for stress and activity, in addition to basal energy expenditure equations, there have been published evaluations overestimating or underestimating the metabolic needs of critically ill patients. This highlights the need for the use of indirect calorimetry as a tool for the quantification of these metabolic needs in this patient population (2, 3, 5, 11, 15, 23, 28, 30, 38, 39, 60–66).
Coss-Bu et al., evaluated the basal energy expenditure values measured by indirect calorimetry in 55 children on mechanical ventilation; the corresponding values using the Harris-Benedict and Talbot equations of each patient were corrected with two factors of 1.3 and 1.5 (2). The corrected basal energy expenditure results obtained were compared to the resting energy expenditure measured by indirect calorimetry and found to have vast differences, thus concluding that indirect calorimetry is the only precise method to measure the metabolic requirements of critically ill pediatric patients.
Predictive equations
It's been shown that the use of formulas or predictive equations is more appropriate than the use of reference values, these equations have been developed using multiple linear regression models that include different variables (gender, body weight, height, body temperature, heart rate, inotropic infusion rate, diagnosis of sepsis, ICU length of stay, mechanical ventilation, etc.), performed in both adults and pediatric patients admitted to an ICU (67–71). Although, these predictive formulas should be used with caution given the variability of the metabolic state of these critically ill patients during their hospital stay.
Use of carbon dioxide production to estimate energy expenditure
Recent studies in critically ill adults and children have reported on a simplified formula to calculate basal energy expenditure based solely on the measurement of the production of carbon dioxide or a derivation of different values of the respiratory coefficient (0.80, 0.85, and 0.89) (72–76).
These calculations have been made possible thanks to the measurement of carbon dioxide concentrations on mechanical ventilators currently used in intensive care units. VCO2 es calculated by taking into consideration minute ventilation and the concentration of exhaled carbon dioxide. The consensus that several authors reached was that the measurement of basal energy expenditure based solely on VCO2 in the absence of VO2 measurements is simple and attractive and could be used in lieu of predictive equations, although indirect calorimetry, which includes both the measurement of VCO2 and VO2, remains the recommended form of calculating energy expenditure in critically ill patients on mechanical ventilation.
Use of indirect calorimetry in critically ill children
Targeted indirect calorimetry
Basal energy expenditure measured by indirect calorimetry is the gold standard for the measurement of metabolic needs in critically ill patients. This recommendation is included in the most recent pediatric nutritional clinical guidelines of A.S.P.E.N., S.C.C.M. (41), and E.S.P.E.N. (77), which propose obtaining indirect calorimetry calculations in patients with fluctuating metabolic alterations or who have evidence of malnutrition. These guidelines highlight the importance of identifying imbalances between caloric intake and measured energy expenditure with the goal of avoiding an excessive or inadequate nutritional consumption and this way preventing any adverse consequences that this might entail. Given the ever-changing nature of metabolic conditions that are reflected in a patient's energy requirements through the course of their disease, the calculation of their resting energy expenditure should be obtained serially, and corresponding changes must be incorporated to their caloric intake.
The current clinical nutrition guidelines for critically ill pediatric patients that were recently published by A.S.P.E.N. and S.C.C.M. state that indirect calorimetry plays a vital role in patients admitted to the PICU whose metabolic needs are constantly changing, whether they be hypermetabolic or hypometabolic. In a clinical study by Metha et al. (32), in critically ill pediatric patients admitted to a PICU it was found that indirect calorimetry in high-risk patients helped prevent accumulated excesses and deficits in metabolic balance. Kyle et al. (40), published a prospective observational study that included 150 critically ill children and was meant to determine how many of them met the criteria for indirect calorimetry measurements during their first week of IC stay. The results showed that 75% of the enrolled patients were candidates for the use of indirect calorimetry and that one-third of these patients had two or more criteria for its use. With this study, the authors highlighted even more the importance of performing multi-center clinical studies to establish factors that determine the cost-benefit ratio of the use of indirect calorimetry in patients admitted to a critical care unit.
Measuring energy expenditure in children with congenital heart disease
Several authors reported measurements of energy expenditure using indirect calorimetry in children after correction for congenital heart disease (CHD) (6, 78–81). De Wit et al. (78), reported energy expenditure measurements in 21 mechanically ventilated children, the children who underwent cardiopulmonary bypass (CPB) had REE of 73.6 ± 14.45 vs. 58.3 ± 10.29 kcal/kg/day in children who underwent non-bypass surgery, suggesting that CPB exposure increased energy expenditure and the need to increase caloric requirements postoperatively. Also, none of the predictive formulas correlated with measured energy expenditure. Mehta et al. (6), measured REE using an in-line IC in 30 children with single-ventricle physiology after Fontan surgery, mean REE at 8 h after surgery was 57 ± 20 kcal/kg/day, also, measured REE had poor correlation with equation-estimated energy expenditure. The authors concluded that in the absence of IC, using formulas to estimate caloric requirements will increase the risk of overfeeding. Floh et al. (79), reported IC measurements in 111 children after CPB for CHD, measured REE decreased from 51 to 45 kcal/kg/day at 72 h postoperatively, indicating an increased inflammatory condition after cardiopulmonary bypass. Zhang et al. (81), reported a review of measured REE using IC in children after surgery for CHD, they concluded that daily indirect calorimetry provides the best estimate of energy needs for this population of children. Roebuck et al. (80), reported results of REE in 107 children following CPB for correction of CHD. Several formulas were compared vs. REE; Dietary Reference Intake, Harris Benedict, Schofield, and the World Health Organization. All the equations incorrectly estimated REE after surgery, with a predominance of overestimation, suggesting that IC remains the best method of estimating REE after CPB. In summary, all these studies indicate poor agreement of the use of formulas to estimate energy expenditure in children after CPB for correction of CHD, making the use of indirect calorimetry the ideal method to estimate caloric needs for this population.
Energy expenditure in children with burns
Several studies have published measurements of energy expenditure in children with burns (43, 82–85), and exfoliative skin disorders (86, 87). Goran et al. (43), measured TEE in 15 children with burns using the doubly labeled water technique; TEE was 1.18 ± 0.17 times the REE measured by indirect calorimetry, and significant correlation among the two methods was found (r2 = 0.92, p = 0.002). the authors recommended that optimal nutrition support will be adjusting the REE by a factor of 1.2 in convalescent burned children. Gore et al. (82), used IC in 74 children with ≥40% total body surface area (TBSA) burns and compared to formulas. The authors concluded that formulas overestimate caloric needs in children with severe burns and that measured REE requires an additional correction factor of 30% to maintain body weight. Mayes et al. (84), measured REE in 48 children younger than 10 years of age with burns (∼ mean 30% TBSA). The study found that formulas for children with burns overpredicted energy needs and when indirect calorimetry is used in burn patients in this age group, the application of a 30% activity factor is recommended. Liusuwan et al. (83), reported values of REE in 10 children with burns (35%–97% of TBSA). The authors compared the measured REE multiplied by a factor of 1.3 with three formulas (Harris Benedict, Mayes, and World Health Organization) multiplied by a factor of 2. The results indicated that the accuracy of these formulas in predicting measured REE adjusted by a factor of 1.3 was variable and larger studies are needed to improve the prediction of energy needs in burned children. Suman et al. (85), measured REE in 91 severely burned children between 3 and 18 years of age (average of 60% TBSA). The authors compared measured REE vs. Harris Benedict, Schofield, and World Health Organization formulas without correction factor. The results showed the three formulas underestimated measured REE by indirect calorimetry with the mean percent of predicted calculated from the three equations similar on average at 152% (1,203–1,219 vs. 1,851 kcal/day from the measurement). The Bland-Altman analysis showed poor agreement between measured REE and the predicted using the three equations. The authors concluded that indirect calorimetry be used to determine REE in severely burned children. In summary, energy expenditure is elevated in children with burns and is proportional to the body surface area affected. The use of formulas to predict energy needs in this population is not accurate making indirect calorimetry the recommended method to estimate energy requirements in children with burns; this recommendation is endorsed by several scientific organizations who have published nutrition support guidelines for patients with burns (88–94).
Use of the respiratory quotient to adjust caloric intake
Several studies have reported on the use of the RQ to adjust the caloric intake and on the metabolic evaluations in critically ill patients (22, 28–32, 95). The results of these studies have shown that indirect calorimetry is vital to modifying individual nutrition plans in patients admitted to the PICU, especially in patients with pulmonary disease, given the fact that an excess of dietary carbohydrates may result in an increase in respiratory work from an increase in VCO2 and make the process to wean from mechanical ventilation more difficult.
The RQ has been used as an indicator of excess or deficient caloric intake in critically ill pediatric patients with variable results (28–32). Mehta et al. (32), published results of indirect calorimetry in 14 pediatric patients admitted to the PICU. The RQ of patients classified as hypermetabolic [RMR/Basal metabolic rate (BMR) > 110%] [n = 7, 50.3 ± 64.2 (SD) kg] was 0.85 ± 0.03 with a caloric intake of 1,464 ± 1,008 kcal/day; and the hypometabolic patients (RMR/BMR < 90%) [n = 7, 44.5 ± 59 kg] had an RQ of 0.94 ± 0.06 with a caloric intake of 935 ± 559 kcal/day. The authors highlighted the importance of using indirect calorimetry to adjust individual nutritional support during the patient's PICU stay. Three clinical studies in pediatric patients have determined sensitivity and specificity values of RQ as a guide to assess for excessive or deficient caloric intake. The study by Dokken et al. (28), included 30 patients on mechanical ventilation admitted to the PICU; reported an RQ value of <0.85 as having a sensitivity and specificity of 27% and 87%, respectively; and an RQ value of >1.0, had sensitivity and specificity of 21% and 98%, respectively. The report by Hulst et al. (29), involved 95 PICU patients and reported sensitivity and specificity for an RQ value of <0.85 (deficient caloric intake), as being 63% and 89%, respectively; while the sensitivity and specificity for an RQ value of >1.0 (excess caloric intake) was 21% and 97%, respectively. Kerklaan et al. (30), studied 78 pediatric patients on mechanical ventilation and concluded that the identification of patients with excess or deficient caloric intake by way of IC and RQ values depended on the definition that was used to categorize the patients. Liusuwan et al. (31), reported on 74 pediatric patients with burns over 20% of their total body surface area with a sensitivity and specificity for an RQ value of <0.85, of 40% and 77%, respectively, and for an RQ value of >1.0, sensitivity and specificity values of 23% and 85%, respectively. Based on these studies, it can be determined that the RQ in critically ill pediatric patients has a low sensitivity and adequate specificity in identifying patients who suffer from an excess or deficient caloric intake.
In conclusion, indirect calorimetry remains the gold standard for measuring energy expenditure in critically ill patients. It is important to understand the methodology behind this technique, as well as the cost-benefit ratio to optimize its use in a critical care setting with patients who have changing metabolic needs.
Author contributions
Substantial contributions to conception and design, acquisition of data, or analysis and interpretation of data: JS-G, PZ, MM, DW, and JC-B. Drafting the article or revising it critically for important intellectual content: JS-G, PZ, MM, DW, and JC-B. Final approval of the version to be published: JS-G, PZ, MM, DW, and JC-B. All authors contributed to the article and approved the submitted version.
Conflict of interest
The authors declare that the research was conducted in the absence of any commercial or financial relationships that could be construed as a potential conflict of interest.
Publisher's note
All claims expressed in this article are solely those of the authors and do not necessarily represent those of their affiliated organizations, or those of the publisher, the editors and the reviewers. Any product that may be evaluated in this article, or claim that may be made by its manufacturer, is not guaranteed or endorsed by the publisher.
References
1. Coss-Bu JA, Hamilton-Reeves J, Patel JJ, Morris CR, Hurt RT. Protein requirements of the critically ill pediatric patient. Nutr Clin Pract. (2017) 32(1_suppl):128S–41S. doi: 10.1177/0884533617693592
2. Coss-Bu JA, Jefferson LS, Walding D, David Y, Smith EO, Klish WJ. Resting energy expenditure in children in a pediatric intensive care unit: comparison of harris-benedict and talbot predictions with indirect calorimetry values. Am J Clin Nutr. (1998) 67(1):74–80. doi: 10.1093/ajcn/67.1.74
3. Coss-Bu JA, Mehta NM. Energy metabolism. In: Caballero B, Finglas P, Toldrá F, editors. The encyclopedia of food and health. 2nd ed. Oxford: Academic Press (2016). p. 503–10.
4. Mehta NM. Energy expenditure: how much does it matter in infant and pediatric chronic disorders? Pediatr Res. (2015) 77(1–2):168–72. doi: 10.1038/pr.2014.180
5. Mehta NM. Estimating energy expenditure and metabolism at the bedside-still shooting in the dark? Pediatr Crit Care Med. (2020) 21(8):776–7. doi: 10.1097/PCC.0000000000002360
6. Mehta NM, Costello JM, Bechard LJ, Johnson VM, Zurakowski D, McGowan FX, et al. Resting energy expenditure after fontan surgery in children with single-ventricle heart defects. J Parenter Enteral Nutr. (2012) 36(6):685–92. doi: 10.1177/0148607112445581
7. Orellana RA, Coss-Bu JA. Energy and macronutrient requirements in the critically ill child. In: Goday PS, Mehta NM, editors. Pediatric critical care nutrition. 1st ed. New York, NY: McGraw-Hill Education Medical (2015). p. 33–58.
8. Orellana RA, Coss-Bu JA. Nutrition and gastrointestinal emergencies. In: Watson RS, Thompson AE, editors. Pediatric intensive care. Pittsburgh critical care medicine. New York, NY: Oxford University Press (2017). p. 193–207.
9. Orellana RA, Kyle UG, Coss-Bu JA. Nutritional assessment and feeding in the icu. In: Stockwell JA, Preissig CM, editors. Comprehensive critical care: Pediatric. Mount Porspect, IL, United States: Society of Critical Care Medicine (2012). p. 931–48.
10. da Rocha EE, Alves VG, da Fonseca RB. Indirect calorimetry: methodology, instruments and clinical application. Curr Opin Clin Nutr Metab Care. (2006) 9(3):247–56. doi: 10.1097/01.mco.0000222107.15548.f5
11. Flancbaum L, Choban P, Sambucco S, Verducci J, Burge J. Comparison of indirect calorimetry, the fick method, and prediction equations in estimating the energy requirements of critically ill patients. Am J Clin Nutr. (1999) 69:461–6. doi: 10.1093/ajcn/69.3.461
12. Lev S, Cohen J, Singer P. Indirect calorimetry measurements in the ventilated critically ill patient: facts and controversies–the heat is on. Crit Care Clin. (2010) 26(4):e1–9. doi: 10.1016/j.ccc.2010.08.001
13. Volp ACP, de Oliveira FCE, Duarte Moreira Alves R, Esteves EA, Bressan J. Energy expenditure: components and evaluation methods. Nutr Hosp. (2011) 26(3):430–40. doi: 10.1590/S0212-16112011000300002
14. Ferrannini E. The theoretical bases of indirect calorimetry: a review. Metab Clin Exp. (1988) 37(3):287–301. doi: 10.1016/0026-0495(88)90110-2
15. Framson CM, LeLeiko NS, Dallal GE, Roubenoff R, Snelling LK, Dwyer JT. Energy expenditure in critically ill children. Pediatr Crit Care Med. (2007) 8(3):264–7. doi: 10.1097/01.PCC.0000262802.81164.03
16. Harris JA, Benedict FG. A biometric study of human basal metabolism. Proc Natl Acad Sci. (1918) 4(12):370–3. doi: 10.1073/pnas.4.12.370
17. Talbot FB. Basal metabolism standards for children. Am J Dis Child. (1938) 55(3):455–9. doi: 10.1001/archpedi.1938.01980090003001%J.
18. Weir JB. New methods for calculating metabolic rate with special reference to protein metabolism. J Physiol. (1949) 109(1-2):1–9. doi: 10.1113/jphysiol.1949.sp004363
19. Frayn KN. Calculation of substrate oxidation rates in vivo from gaseous exchange. J Appl Physiol Respir Environ Exerc Physiol. (1983) 55(2):628–34. doi: 10.1152/jappl.1983.55.2.628
20. Konstantinides FN, Konstantinides NN, Li JC, Myaya ME, Cerra FB. Urinary urea nitrogen: too insensitive for calculating nitrogen balance studies in surgical clinical nutrition. J Parenter Enteral Nutr. (1991) 15(2):189–93. doi: 10.1177/0148607191015002189
21. Consolazio CF, Johnson RE, Pecora LJ. Physiological measurement of metabolic functions in man. In: Handbok of physiology. New York: McGraw-Hill (1963). p. 313–7.
22. Coss-Bu JA, Klish WJ, Walding D, Stein F, Smith EO, Jefferson LS. Energy metabolism, nitrogen balance, and substrate utilization in critically ill children. Am J Clin Nutr. (2001) 74(5):664–9. doi: 10.1093/ajcn/74.5.664
23. Joosten KF, Verhoeven JJ, Hazelzet JA. Energy expenditure and substrate utilization in mechanically ventilated children. Nutrition. (1999) 15(6):444–8. doi: 10.1016/s0899-9007(99)00081-7
24. Makk LJ, McClave SA, Creech PW, Johnson DR, Short AF, Whitlow NL, et al. Clinical application of the metabolic cart to the delivery of total parenteral nutrition. Crit Care Med. (1990) 18(12):1320–7. doi: 10.1097/00003246-199012000-00003
25. Mickell JJ. Urea nitrogen excretion in critically ill children. Pediatrics. (1982) 70(6):949–55. doi: 10.1542/peds.70.6.949
26. Verhoeven JJ, Hazelzet JA, van der Voort E, Joosten KF. Comparison of measured and predicted energy expenditure in mechanically ventilated children. Intensive Care Med. (1998) 24(5):464–8. doi: 10.1007/s001340050597
27. Coss-Bu JA, Jefferson LS, Walding D, David Y, Smith EO, Klish WJ. Resting energy expenditure and nitrogen balance in critically ill pediatric patients on mechanical ventilation. Nutrition. (1998) 14(9):649–52. doi: 10.1016/s0899-9007(98)00050-1
28. Dokken M, Rustøen T, Stubhaug A. Indirect calorimetry reveals that better monitoring of nutrition therapy in pediatric intensive care is needed. J Parenter Enteral Nutr. (2015) 39(3):344–52. doi: 10.1177/0148607113511990
29. Hulst JM, van Goudoever JB, Zimmermann LJ, Hop WC, Büller HA, Tibboel D, et al. Adequate feeding and the usefulness of the respiratory quotient in critically ill children. Nutrition. (2005) 21(2):192–8. doi: 10.1016/j.nut.2004.05.020
30. Kerklaan D, Hulst JM, Verhoeven JJ, Verbruggen SC, Joosten KF. Use of indirect calorimetry to detect overfeeding in critically ill children: finding the appropriate definition. J Pediatr Gastroenterol Nutr. (2016) 63(4):445–50. doi: 10.1097/MPG.0000000000001197
31. Manotok R, Palmieri TL, Greenhalgh DG. The respiratory quotient has little value in evaluating the state of feeding in burn patients. J Burn Care Res. (2008) 29(4):655–9. doi: 10.1097/BCR.0b013e31817db9e3
32. Mehta NM, Bechard LJ, Leavitt K, Duggan C. Cumulative energy imbalance in the pediatric intensive care unit: role of targeted indirect calorimetry. J Parenter Enteral Nutr. (2009) 33(3):336–44. doi: 10.1177/0148607108325249
33. Weissman C, Kemper M. Metabolic measurements in the critically ill. Crit Care Clin. (1995) 11(1):169–97. doi: 10.1016/S0749-0704(18)30091-5
34. Askanazi J. Respiratory changes induced by the large glucose loads of total parenteral nutrition. J Am Med Assoc. (1980) 243:1444. doi: 10.1001/jama.1980.03300400028023
35. Covelli HD, Black JW, Olsen MS, Beekman JF. Respiratory failure precipitated by high carbohydrate loads. Ann Intern Med. (1981) 95(5):579–81. doi: 10.7326/0003-4819-95-5-579
36. Guenst JM, Nelson LD. Predictors of total parenteral nutrition-induced lipogenesis. Chest. (1994) 105(2):553–9. doi: 10.1378/chest.105.2.553
37. Liposky JM, Nelson LD. Ventilatory response to high caloric loads in critically ill patients. Crit Care Med. (1994) 22(5):796–802. doi: 10.1097/00003246-199405000-00014
38. Mehta NM, Bechard LJ, Dolan M, Ariagno K, Jiang H, Duggan C. Energy imbalance and the risk of overfeeding in critically ill children. Pediatr Crit Care Med. (2011) 12(4):398–405. doi: 10.1097/PCC.0b013e3181fe279c
39. Mehta NM, Compher C. Directors ASPENBo. A.S.P.E.N. Clinical guidelines: nutrition support of the critically ill child. J Parenter Enteral Nutr. (2009) 33(3):260–76. doi: 10.1177/0148607109333114
40. Kyle UG, Arriaza A, Esposito M, Coss-Bu JA. Is indirect calorimetry a necessity or a luxury in the pediatric intensive care unit? J Parenter Enteral Nutr. (2012) 36(2):177–82. doi: 10.1177/0148607111415108
41. Mehta NM, Skillman HE, Irving SY, Coss-Bu JA, Vermilyea S, Farrington EA, et al. Guidelines for the provision and assessment of nutrition support therapy in the pediatric critically ill patient: society of critical care medicine and American society for parenteral and enteral nutrition. Pediatr Crit Care Med. (2017) 18(7):675–715. doi: 10.1097/PCC.0000000000001134
42. Goran MI, Carpenter WH, Poehlman ET. Total energy expenditure in 4- to 6-yr-old children. Am J Physiol. (1993) 264(5 Pt 1):E706–11. doi: 10.1152/ajpendo.1993.264.5.E706
43. Goran MI, Peters EJ, Herndon DN, Wolfe RR. Total energy expenditure in burned children using the doubly labeled water technique. Am J Physiol. (1990) 259(4 Pt 1):E576–85. doi: 10.1152/ajpendo.1990.259.4.E576
44. Jensen CL, Butte NF, Wong WW, Moon JK. Determining energy expenditure in preterm infants: comparison of 2 h(2)18o method and indirect calorimetry. Am J Physiol. (1992) 263(3 Pt 2):R685–92. doi: 10.1152/ajpregu.1992.263.3.R685
45. Roberts SB, Coward WA, Schlingenseipen KH, Nohria V, Lucas A. Comparison of the doubly labeled water [2h2(18)O] method with indirect calorimetry and a nutrient-balance study for simultaneous determination of energy expenditure, water intake, and metabolizable energy intake in preterm infants. Am J Clin Nutr. (1986) 44(3):315–22. doi: 10.1093/ajcn/44.3.315
46. Westerterp KR, Lafeber HN, Sulkers EJ, Sauer PJ. Comparison of short term indirect calorimetry and doubly labeled water method for the assessment of energy expenditure in preterm infants. Biol Neonate. (1991) 60(2):75–82. doi: 10.1159/000243391
47. Coward WA. Stable isotopic methods for measuring energy expenditure. The doubly-labelled-water [2h2(18)O] method: principles and practice. Proc Nutr Soc. (1988) 47(3):209–18. doi: 10.1079/pns19880037
48. Schoeller DA. Energy expenditure from doubly labeled water: some fundamental considerations in humans. Am J Clin Nutr. (1983) 38(6):999–1005. doi: 10.1093/ajcn/38.6.999
49. Seale J, Miles C, Bodwell CE. Sensitivity of methods for calculating energy expenditure by use of doubly labeled water. J Appl Physiol. (1989) 66(2):644–52. doi: 10.1152/jappl.1989.66.2.644
50. Elia M, Fuller N, Murgatroyd P. The potential use of the labelled bicarbonate method for estimating energy expenditure in man. Proc Nutr Soc. (1988) 47(3):247–58. doi: 10.1079/pns19880042
51. Irving CS, Wong WW, Shulman RJ, Smith EO, Klein PD. [13c]Bicarbonate kinetics in humans: intra- vs. Interindividual variations. Am J Physiol. (1983) 245(2):R190–202. doi: 10.1152/ajpregu.1983.245.2.R190
52. Kien CL. Isotopic dilution of CO2 as an estimate of CO2 production during substrate oxidation studies. Am J Physiol. (1989) 257(2 Pt 1):E296–8. doi: 10.1152/ajpendo.1989.257.2.E296
53. Van Aerde JE, Sauer PJ, Pencharz PB, Canagarayar U, Beesley J, Smith JM, et al. The effect of energy intake and expenditure on the recovery of 13CO2 in the parenterally fed neonate during a 4-hour primed constant infusion of NaH13CO3. Pediatr Res. (1985) 19(8):806–10. doi: 10.1203/00006450-198508000-00005
54. Verbruggen SC, Coss-Bu J, Wu M, Schierbeek H, Joosten KF, Dhar A, et al. Current recommended parenteral protein intakes do not support protein synthesis in critically ill septic, insulin-resistant adolescents with tight glucose control. Crit Care Med. (2011) 39(11):2518–25. doi: 10.1097/CCM.0b013e3182257410
55. Wolfe RR, Shaw JH, Nadel ER, Wolfe MH. Effect of substrate intake and physiological state on background 13CO2 enrichment. J Appl Physiol Respir Environ Exerc Physiol. (1984) 56(1):230–4. doi: 10.1152/jappl.1984.56.1.230
56. Elia M. Energy equivalents of CO2 and their importance in assessing energy expenditure when using tracer techniques. Am J Physiol. (1991) 260(1 Pt 1):E75–88. doi: 10.1152/ajpendo.1991.260.1.E75
57. Energy and Protein Requirements. Report of a joint fao/who/unu expert consultation. World Health Organ Tech Rep Ser. (1985) 724:1–206.3937340
58. Schofield WN. Predicting basal metabolic rate, new standards and review of previous work. Hum Nutr Clin Nutr. (1985) 39(Suppl 1):5–41.4044297
59. Kyle UG, Jaimon N, Coss-Bu JA. Nutrition support in critically ill children: underdelivery of energy and protein compared with current recommendations. J Acad Nutr Diet. (2012) 112(12):1987–92. doi: 10.1016/j.jand.2012.07.038
60. Beggs MR, Garcia Guerra G, Larsen BMK. Do picu patients meet technical criteria for performing indirect calorimetry? Clin Nutr ESPEN. (2016) 15:80–4. doi: 10.1016/j.clnesp.2016.06.003
61. Jotterand Chaparro C, Moullet C, Taffé P, Laure Depeyre J, Perez MH, Longchamp D, et al. Estimation of resting energy expenditure using predictive equations in critically ill children: results of a systematic review. J Parenter Enteral Nutr. (2018) 42(6):976–86. doi: 10.1002/jpen.1146
62. Jotterand Chaparro C, Taffé P, Moullet C, Laure Depeyre J, Longchamp D, Perez MH, et al. Performance of predictive equations specifically developed to estimate resting energy expenditure in ventilated critically ill children. J Pediatr. (2017) 184:220–6.e5. doi: 10.1016/j.jpeds.2016.12.063
63. Meyer R, Kulinskaya E, Briassoulis G, Taylor RM, Cooper M, Pathan N, et al. The challenge of developing a new predictive formula to estimate energy requirements in ventilated critically ill children. Nutr Clin Pract. (2012) 27(5):669–76. doi: 10.1177/0884533612448479
64. Smallwood CD, Mehta NM. Gas exchange measurement during pediatric mechanical ventilation–agreement between gas sampling at the airway and the ventilator exhaust. Clin Nutr. (2013) 32(6):988–92. doi: 10.1016/j.clnu.2013.03.009
65. Sy J, Gourishankar A, Gordon WE, Griffin D, Zurakowski D, Roth RM, et al. Bicarbonate kinetics and predicted energy expenditure in critically ill children. Am J Clin Nutr. (2008) 88(2):340–7. doi: 10.1093/ajcn/88.2.340
66. Vazquez Martinez JL, Martinez-Romillo PD, Diez Sebastian J, Ruza Tarrio F. Predicted versus measured energy expenditure by continuous, online indirect calorimetry in ventilated, critically ill children during the early postinjury period. Pediatr Crit Care Med. (2004) 5(1):19–27. doi: 10.1097/01.Pcc.0000102224.98095.0a
67. Frankenfield DC, Omert LA, Badellino MM, Wiles CE 3rd, Bagley SM, Goodarzi S, et al. Correlation between measured energy expenditure and clinically obtained variables in trauma and sepsis patients. J Parenter Enteral Nutr. (1994) 18(5):398–403. doi: 10.1177/0148607194018005398
68. Goran MI, Broemeling L, Herndon DN, Peters EJ, Wolfe RR. Estimating energy requirements in burned children: a new approach derived from measurements of resting energy expenditure. Am J Clin Nutr. (1991) 54(1):35–40. doi: 10.1093/ajcn/54.1.35
69. Hwang TL, Huang SL, Chen MF. The use of indirect calorimetry in critically ill patients–the relationship of measured energy expenditure to injury severity score, septic severity score, and apache ii score. J Trauma. (1993) 34(2):247–51. doi: 10.1097/00005373-199302000-00013
70. Swinamer DL, Grace MG, Hamilton SM, Jones RL, Roberts P, King EG. Predictive equation for assessing energy expenditure in mechanically ventilated critically ill patients. Crit Care Med. (1990) 18(6):657–61. doi: 10.1097/00003246-199006000-00017
71. White MS, Shepherd RW, McEniery JA. Energy expenditure in 100 ventilated, critically ill children: improving the accuracy of predictive equations. Crit Care Med. (2000) 28(7):2307–12. doi: 10.1097/00003246-200007000-00021
72. Kagan I, Zusman O, Bendavid I, Theilla M, Cohen J, Singer P. Validation of carbon dioxide production (VCo(2)) as a tool to calculate resting energy expenditure (ree) in mechanically ventilated critically ill patients: a retrospective observational study. Crit Care. (2018) 22(1):186. doi: 10.1186/s13054-018-2108-8
73. Kerklaan D, Augustus ME, Hulst JM, van Rosmalen J, Verbruggen S, Joosten KFM. Validation of ventilator-derived VCO2 measurements to determine energy expenditure in ventilated critically ill children. Clin Nutr. (2017) 36(2):452–7. doi: 10.1016/j.clnu.2016.01.001
74. Mehta NM, Smallwood CD, Joosten KF, Hulst JM, Tasker RC, Duggan CP. Accuracy of a simplified equation for energy expenditure based on bedside volumetric carbon dioxide elimination measurement–a two-center study. Clin Nutr. (2015) 34(1):151–5. doi: 10.1016/j.clnu.2014.02.008
75. Mouzaki M, Schwartz SM, Mtaweh H, La Rotta G, Mah K, Herridge J, et al. Can vco(2)-based estimates of resting energy expenditure replace the need for indirect calorimetry in critically ill children? J Parenter Enteral Nutr. (2017) 41(4):619–24. doi: 10.1177/0148607115627143
76. Stapel SN, de Grooth HJ, Alimohamad H, Elbers PW, Girbes AR, Weijs PJ, et al. Ventilator-derived carbon dioxide production to assess energy expenditure in critically ill patients: proof of concept. Crit Care. (2015) 19:370. doi: 10.1186/s13054-015-1087-2
77. Joosten K, Embleton N, Yan W, Senterre T. Espghan/Espen/Espr/Cspen guidelines on pediatric parenteral nutrition: energy. Clin Nutr. (2018) 37(6 Pt B):2309–14. doi: 10.1016/j.clnu.2018.06.944
78. De Wit B, Meyer R, Desai A, Macrae D, Pathan N. Challenge of predicting resting energy expenditure in children undergoing surgery for congenital heart disease. Pediatr Crit Care Med. (2010) 11(4):496–501. doi: 10.1097/PCC.0b013e3181ce7465
79. Floh AA, Nakada M, La Rotta G, Mah K, Herridge JE, Van Arsdell G, et al. Systemic inflammation increases energy expenditure following pediatric cardiopulmonary bypass. Pediatr Crit Care Med. (2015) 16(4):343–51. doi: 10.1097/PCC.0000000000000357
80. Roebuck N, Fan CS, Floh A, Harris ZL, Mazwi ML. A comparative analysis of equations to estimate patient energy requirements following cardiopulmonary bypass for correction of congenital heart disease. J Parenter Enteral Nutr. (2020) 44(3):444–53. doi: 10.1002/jpen.1610
81. Zhang J, Cui YQ, Ma Md ZM, Luo Y, Chen XX, Li J. Energy and protein requirements in children undergoing cardiopulmonary bypass surgery: current problems and future direction. J Parenter Enteral Nutr. (2019) 43(1):54–62. doi: 10.1002/jpen.1314
82. Gore DC, Rutan RL, Hildreth M, Desai MH, Herndon DN. Comparison of resting energy expenditures and caloric intake in children with severe burns. J Burn Care Rehabil. (1990) 11(5):400–4. doi: 10.1097/00004630-199009000-00005
83. Liusuwan RA, Palmieri TL, Kinoshita L, Greenhalgh DG. Comparison of measured resting energy expenditure versus predictive equations in pediatric burn patients. J Burn Care Rehabil. (2005) 26(6):464–70. doi: 10.1097/01.bcr.0000185786.38365.3d
84. Mayes T, Gottschlich MM, Khoury J, Warden GD. Evaluation of predicted and measured energy requirements in burned children. J Am Diet Assoc. (1996) 96(1):24–9. doi: 10.1016/s0002-8223(96)00008-9
85. Suman OE, Mlcak RP, Chinkes DL, Herndon DN. Resting energy expenditure in severely burned children: analysis of agreement between indirect calorimetry and prediction equations using the bland-altman method. Burns. (2006) 32(3):335–42. doi: 10.1016/j.burns.2005.10.023
86. Coss-Bu JA, Jefferson LS, Levy ML, Walding D, David Y, Klish WJ. Nutrition requirements in patients with toxic epidermal necrolysis. Nutr Clin Pract. (1997) 12(2):81–4. doi: 10.1177/011542659701200281
87. Mayes T, Gottschlich M, Khoury J, Warner P, Kagan R. Energy requirements of pediatric patients with Stevens-Johnson syndrome and toxic epidermal necrolysis. Nutr Clin Pract. (2008) 23(5):547–50. doi: 10.1177/0884533608323434
88. Rousseau AF, Losser MR, Ichai C, Berger MM. Espen endorsed recommendations: nutritional therapy in major burns. Clin Nutr. (2013) 32(4):497–502. doi: 10.1016/j.clnu.2013.02.012
89. Brychta P. European practice guidelines for burn care: minimum level of burn care provision in Europe. In: Jeschke MG, Kamolz L-P, Sjöberg F, Wolf SE, editors. Handbook of burns: Acute burn care volume 1. Vienna: Springer Vienna (2012). p. 97–102.
90. Young AW, Graves C, Kowalske KJ, Perry DA, Ryan CM, Sheridan RL, et al. Guideline for burn care under austere conditions: special care topics. J Burn Care Res. (2017) 38(2):e497–509. doi: 10.1097/BCR.0000000000000369
91. Garcia de Lorenzo y Mateos A, Ortiz Leyba C, Sanchez SM. Metabolism, nutrition working group of the spanish society of intensive care M, coronary u. Guidelines for specialized nutritional and metabolic support in the critically-ill patient: update. Consensus semicyuc-senpe: critically-ill burnt patient. Nutr Hosp. (2011) (26 Suppl 2)26:59–62. doi: 10.1590/S0212-16112011000800013
92. McClave SA, Taylor BE, Martindale RG, Warren MM, Johnson DR, Braunschweig C, et al. Guidelines for the provision and assessment of nutrition support therapy in the adult critically ill patient: society of critical care medicine (sccm) and American society for parenteral and enteral nutrition (A.S.P.E.N.). J Parenter Enteral Nutr. (2016) 40(2):159–211. doi: 10.1177/0148607115621863
93. Committee IPG, Steering S, Advisory S. Isbi practice guidelines for burn care. Burns. (2016) 42(5):953–1021. doi: 10.1016/j.burns.2016.05.013
94. Committee IPG, Advisory S, Steering S. Isbi practice guidelines for burn care, part 2. Burns. (2018) 44(7):1617–706. doi: 10.1016/j.burns.2018.09.012
Keywords: basal metabolism, indirect calorimetry, critically ill, oxygen consumption, carbon dioxide production, substrate oxidation, respiratory coefficient, pediatrics
Citation: Silva-Gburek J, Zhu PH, Mansour M, Walding D and Coss-Bu JA (2022) A methodological and clinical approach to measured energy expenditure in the critically ill pediatric patient. Front. Pediatr. 10:1027358. doi: 10.3389/fped.2022.1027358
Received: 24 August 2022; Accepted: 29 September 2022;
Published: 21 October 2022.
Edited by:
Artur Delgado, Hospital das Clínicas, Sao Paulo University, BrazilReviewed by:
Ana Carlotti, Universidade de São Paulo, BrazilVijay Srinivasan, Children's Hospital of Philadelphia, United States
© 2022 Silva-Gburek, Zhu, Mansour, Walding and Coss-Bu. This is an open-access article distributed under the terms of the Creative Commons Attribution License (CC BY). The use, distribution or reproduction in other forums is permitted, provided the original author(s) and the copyright owner(s) are credited and that the original publication in this journal is cited, in accordance with accepted academic practice. No use, distribution or reproduction is permitted which does not comply with these terms.
*Correspondence: Jorge A. Coss-Bu jacossbu@texaschildrens.org
Specialty Section: This article was submitted to Pediatric Critical Care, a section of the journal Frontiers in Pediatrics