- 1Neonatal Unit, Homerton Healthcare NHS Foundation Trust, London, United Kingdom
- 2Neonatal Unit, Imperial College Healthcare NHS Trust and Imperial College London, London, United Kingdom
- 3Department of Medical Physics and Biomedical Engineering, Faculty of Engineering Sciences, University College London, London, United Kingdom
- 4Barts and The London School of Medicine and Dentistry, Queen Mary University of London (QMUL), London, United Kingdom
There is no ideal single gut tissue or inflammatory biomarker available to help to try and identify Necrotising Enterocolitis (NEC) before its clinical onset. Neonatologists are all too familiar with the devastating consequences of NEC, and despite many advances in neonatal care the mortality and morbidity associated with NEC remains significant. In this article we review Near Infrared Spectroscopy (NIRS) as a method of measuring regional gut tissue oxygenation. We discuss its current and potential future applications, including considering its effectiveness as a possible new weapon in the early identification of NEC.
What is known?
• Necrotising Enterocolitis (NEC) has significant morbidity and mortality
• There is currently no reliable and effective gut biomarker to predict NEC in routine clinical practice
• Near Infrared Spectroscopy (NIRS) is a non-invasive method of measuring regional tissue oxygenation
• Changes in splanchnic oxygenation could suggest the onset of gut tissue injury
What is new?
• Near Infra-Red Spectroscopy (NIRS) could detect perfusion changes prior to development of NEC allowing opportunity for timely intervention, but further research is needed to examine if early intervention aided by gut NIRS measurements can improve outcomes
• Potentially a machine learning algorithm encompassing routine clinical, haematological, and biochemical parameters, in conjunction with gut related biomarkers and gut regional NIRS would be able to detect NEC prior to clinical manifestations
Introduction
Necrotising Enterocolitis (NEC) occurs in 14% infants under 26 weeks gestation and 10% of babies born before 31 weeks gestation (1). Despite advances in neonatal care NEC continues to have a significant mortality and morbidity and with increasing survival of those more immature infants the population at risk of NEC is ever increasing. Although there has been a plethora of research studies examining the various blood and tissue biomarkers and their effectiveness in diagnosing gut tissue injury, none of the current biomarkers are in regular clinical use and most have some inherent difficulty with their measurement. Due to these difficulties, recent interest has turned to non-biochemical markers of gut hypoxia and ischaemia. This article reviews Near Infrared Spectroscopy (NIRS)—its current clinical use in Neonatology, its potential use in the identification and management of NEC, as well as exploring how effectively it could be implemented into routine clinical practice.
Background and introduction to NIRS
Currently tissue perfusion and oxygenation of patients in Neonatal Intensive Care Units (NICU) is indirectly monitored using systemic blood pressure, heart rate, capillary refill time and evidence of end organ perfusion including lactate level, renal function, and urine output, which are often late signs of any disease process causing impaired perfusion. NIRS is a non-invasive method of contemporaneously measuring regional tissue oxygenation (rSO2) at the bedside. Changes in rSO2 measured by NIRS may reflect the balance between oxygen delivery and consumption (2).
Principles of use
NIRS exploits the differential absorption of light of different biological compounds (e.g., haemoglobin) in the near-infrared region of the electromagnetic spectrum. The principle of how NIRS works in humans is best described by Cohn (3). NIRS monitors use near infrared light at wavelengths of relatively low optical absorption for oxyhemoglobin (HbO2) and deoxyhemoglobin (HHb), generally 700–850 nm, thus allowing near infrared light to penetrate and probe deeper into the tissue. The near infrared range is also where the absorption spectra of HbO2 and HHb are maximally separated with minimal overlap with that of water (980 nm) (4). NIRS can measure the ratio of HbO2 to HHb and the rSO2 can be derived using the equation: rSO2 = HbO2/(HbO2 + HHb).
Measurements using NIRS are specific to the region where the sensors are placed, and the rSO2 reflects perfusion and metabolism. They are not temperature or pulsatility dependent and because they are measured in “real-time” they could allow earlier detection of changes in oxygenation, blood flow and perfusion of the tissue. Tissue oxygenation measurements using NIRS are irrespective of systole or diastole; and as only 20% of blood volume is intra-arterial, measurements are primarily indicative of the venous oxygenation. The tissue microcirculation contains arteries, veins and capillaries meaning that the rSO2 represents a “weighted average” of SO2 in these compartments, with approximately 75%–85% of the signal originating from venules. As opposed to pulse-oximeters which subtract out non-pulsatile flow, NIRS monitors focus on the total light signal and the measured rSO2 reflects the balance of local tissue oxygen supply and demand meaning it is considered complementary to pulse oximetry (5).
Although regional tissue oxygenation measurements are venous weighted, by using peripheral pulse oximetry as a measure of arterial oxygenation, Fractional tissue oxygen extraction (FTOE) can be calculated. FTOE allows the estimation of the balance between oxygen delivery and consumption, at the site that the NIRS device is placed, can be calculated from the rSO2 and arterial oxygen saturation (SaO2) by the equation (6): FTOE = [(SaO2−rSO2)/SaO2] × 100. In addition, if cerebral and splanchnic oxygenation are measured simultaneously then the Cerebral Splanchnic Oxygenation Ratio (CSOR—which compares splanchnic oxygenation with cerebral oxygenation) can be calculated (6).
There are various NIRS monitors from different manufacturers which use different algorithms to measure tissue oxygenation. Different machines measure either the regional oxygen saturation (rSO2) or tissue oxygenation index (TOI), but both essentially reflect the ratio of HbO2 to total Hb (HbO2 + HHb).
Current neonatal applications of NIRS
During the unique neonatal period following birth, the infant undergoes dramatic physiological changes during transition from intra- to extra-uterine life, involving significant changes in their haemodynamics which affect tissue oxygenation and would be reflected in rSO2. NIRS could be valuable in neonates; small size and thin layers covering tissues mean that NIRS measurements at depths of <5 cm can easily reach organs including the brain, kidney, and gut. This means that NIRS monitoring of these organs could yield invaluable physiological information not available in adults as these organs are comparatively less superficial (7). The additional advantage of NIRS is the ability of simultaneous oxygenation measurements of multiple sites (8, 9).
Studies involving cerebral oxygenation are outside the scope of this article but most research on the clinical application of NIRS focuses on cerebral measurements with many researchers having examined the effect of cerebral measurements on clinical outcomes (10–14). However, there is now increasing interest in its use for measuring splanchnic oxygenation. Although the number of studies available examining splanchnic oxygenation, especially over the last ten years, is large, they involve only a small number of infants (very large NIRS studies are still those involving less than 100 infants). The length of time the NIRS measurements were recorded also varies amongst studies and a lot of the studies only involve spot samples. Another issue with the current body of evidence is that the studies in the literature use different NIRS machines. The two most used machines are INVOS (rSO2) and the NIRO-300 (TOI); these measurements are different and therefore non-comparable between studies. Both these machines use different algorithms leading to intrinsically different oxygenation values. Furthermore, the sample volumes between the different machines also differ—the probe design is different meaning that the light source and detector spacing will be slightly different and, therefore, the tissue volumes that the probes sample will be different. Because the regional oxygenation is a weighted average of the arterial oxygenation and the venous oxygenation; if the sample volume is different the ratio of arterial to venous compartment volume could be slightly different, giving different results between the different NIRS machines. Consequently, when examining the literature surrounding NIRS it is important to identify which device was used and not to compare values between different monitors. To overcome this problem, it is more useful to compare percentage change from the baseline rather than the absolute value.
Use of NIRS to identify splanchnic oxygenation and gut injury
In Neonatology NIRS more recently has been used to monitor splanchnic oxygenation (srSO2) to predict NEC and guide decisions regarding initiating feeds. Table 1 summarises the available neonatal studies on the use of NIRS to measure splanchnic oxygenation in neonates (9, 15–28). Table 2 summarises the literature available specifically for the use of NIRS for predicting or diagnosing NEC (15, 27, 29–39).
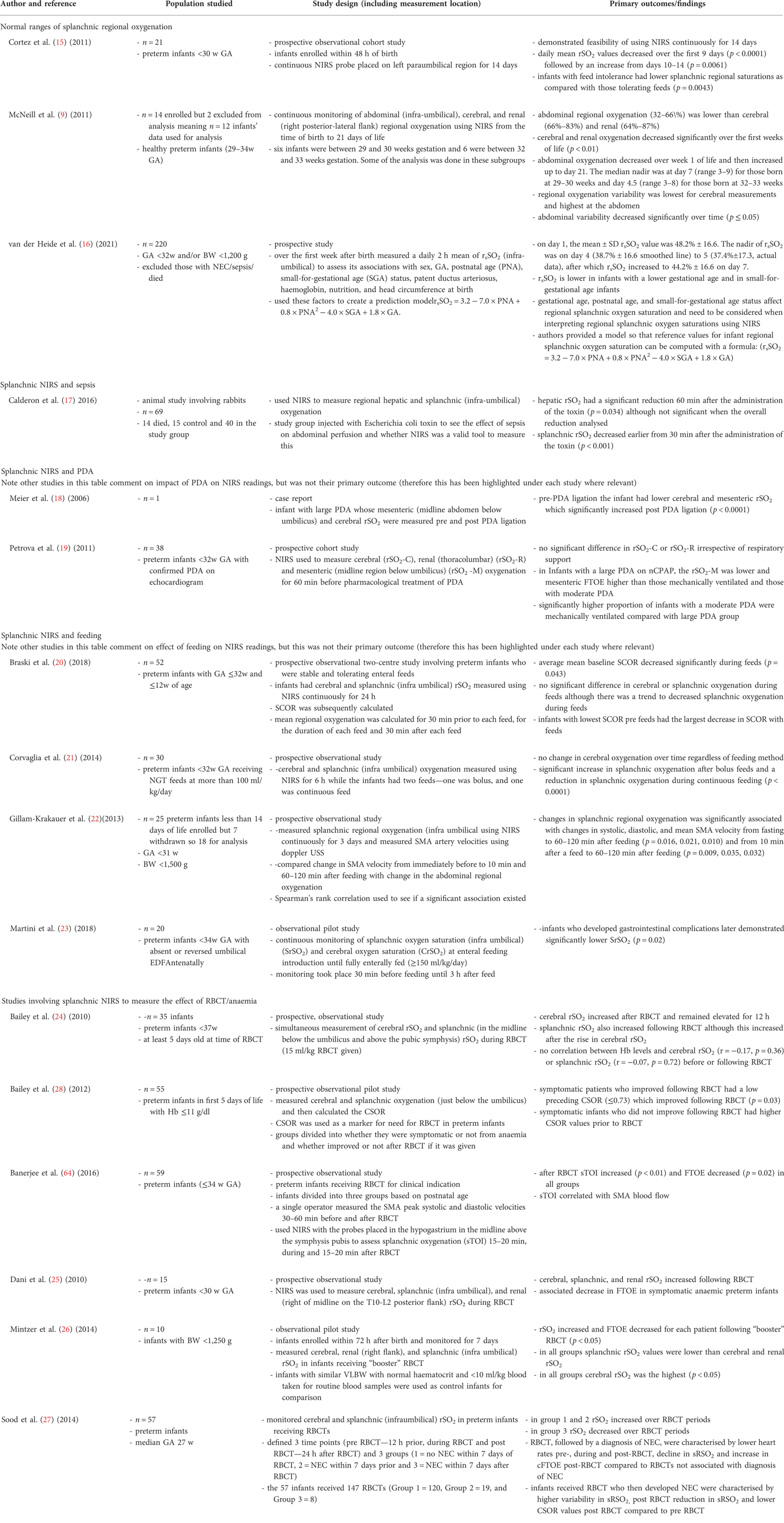
Table 1. Summary of the available literature on the use of Near Infrared Spectroscopy (NIRS) to measure splanchnic oxygenation in neonates.
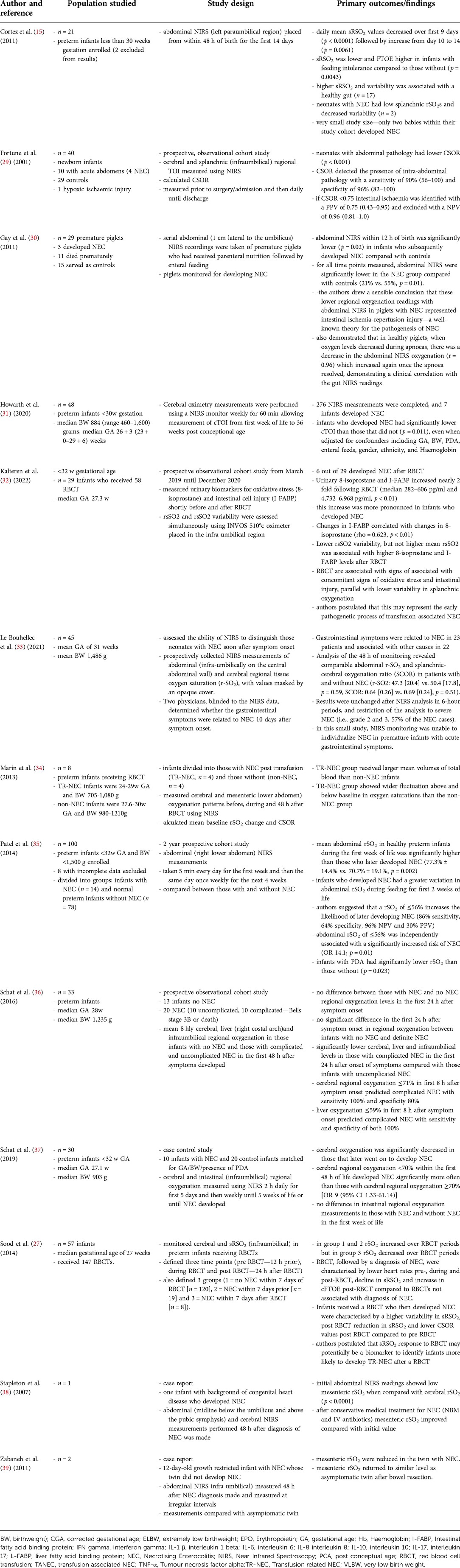
Table 2. Summary of the available literature on the use of Near Infrared Spectroscopy (NIRS) in predicting/diagnosing NEC in neonates.
It is important to highlight that to date this has only been on limited numbers and there is an inherent problem with measuring srSO2 non-invasively using NIRS, in that the amount of gas (abdominal distension) and faecal content effects the measurement (27). In preterm infants these factors are constantly changing; respiratory support commonly given to preterm infants such as continuous positive airway pressure (CPAP) frequently causes abdominal distension, and in the first few days of life, they are known to not open their bowels regularly when they are on limited enteral feeds.
The validity of NIRS-derived srSO2 measurements for examining splanchnic perfusion and oxygenation has already been confirmed as srSO2 strongly correlates with gastric pH, serum lactate and systemic mixed venous saturations (2). In a clinical validation study of 59 preterm infants receiving blood transfusion compared with 12 control infants it was demonstrated that splanchnic NIRS is a reliable technique to inform changes in srSO2 following blood transfusion (40). A recent small study by Gillam-Krakauer et al. using Doppler confirmed that splanchnic NIRS reflects blood flow to the small intestine (22). In their study srSO2 was continuously measured for 3 days in stable infants born at 25–31 weeks gestation (n = 18) and they then compared changes in Superior Mesenteric Artery (SMA) velocity from immediately before to 10 min and 60–120 min after feeding with change in abdominal rSO2 over the same time. They reported that a change in abdominal rSO2 was significantly associated with change in systolic, diastolic and mean SMA velocity from fasting to 60–120 min after feeding (p = 0.016, 0.021, 0.010) and from 10 min after a feed to 60–120 min after feeding (p = 0.009, 0.035, 0.032).
Several studies have looked at the potential of NIRS in identifying gut injury with varying levels of success as we have summarised in Table 2 (15, 29–31, 34–39). There are a few studies to particularly highlight; a recent prospective cohort observational study (31) in appropriately grown preterm infants born at less than 30 weeks of gestational age demonstrated that cerebral oxygenation was significantly lower and FTOE significantly higher in infants who developed NEC across the first 10 weeks of life even when adjusted for potential confounding factors. This may suggest an underlying mechanistic relation between NEC and their worse neurodevelopmental outcome. The same study also found that splanchnic oxygenation was significantly lower and FTOE significantly higher in those infants who developed NEC compared to those without NEC (41, 42). NIRS continuous monitoring could therefore potentially help to identify infants with low cerebral or splanchnic oxygenation allowing earlier treatment and targeted neurodevelopmental interventions.
Studies using NIRS have shown that neonates with an acute abdomen, suggesting mesenteric ischaemia, have a lower Cerebral Splanchnic Oxygenation Ratio (CSOR), suggesting the CSOR could be used to identify gut injury (29). Fortune et al. (29) specifically looked at 40 neonates; 10 with acute abdomens (4 of these had NEC), 29 with normal abdomens and 1 with cerebral HIE. They found that the acute abdomen group had a significantly lower median CSOR value of 0.66 (0.45–0.69), p < 0.001 and that the CSOR had a 90% (56%–100%) sensitivity to detect splanchnic ischaemia in neonates; specifically, they reported that a CSOR of <0.75 was highly predictive of intestinal ischaemia and the need for surgical intervention. If continuous cerebral and splanchnic NIRS were being measured in an individual neonate, then should the CSOR drop this could alert clinicians to an increased severity of NEC and likely need for surgical intervention. However, a complicating factor in preterm infants is that the CSOR may be unreliable in the presence of intraventricular haemorrhage (IVH), which is a common complication of preterm birth. Infants with IVH would be expected to have impaired cerebral autoregulation which could affect the cerebral regional oxygenation (crSO2) and consequently the CSOR (15).
Schat et al. (36) examined 33 infants and compared the mean 8 h cerebral, liver and infraumbilical rSO2 and FTOE divided into infants with no NEC (n = 13), definite NEC (n = 20) and infants with uncomplicated (n = 10) and complicated NEC (n = 10) in the first 48 h after onset of symptoms that were felt to be suspicious for NEC. They found no significant differences in the first 24 h after onset of symptoms in rSO2 and FTOE between infants with no NEC and definite NEC. In preterm infants with complicated NEC, they demonstrated significantly lower cerebral, liver, and infraumbilical rSO2 and higher FTOE within 24 h after onset of symptoms compared with infants with uncomplicated NEC. Their results showed that crSO2 ≤ 71% and liver rSO2 ≤ 59% in the first 8 h after onset of symptoms predicted the onset of complicated NEC with a sensitivity of 1.0 and specificity of 0.8, and a sensitivity of 1.0 and specificity of 1.0, respectively. This suggests that NIRS could differentiate complicated NEC from uncomplicated NEC, but it did not differentiate between definite NEC and no NEC in preterm infants with clinical signs suspicious of NEC.
In addition to absolute changes in regional oxygenation more subtle changes have been identified in infants with NEC; in preterm neonates, a pattern of low srSO2 as well as high fluctuations in mesenteric oxygenation patterns were more pronounced in infants with NEC, especially before NEC onset (15, 27, 34). Cortez et al. (15) looked at 21 preterm infants born at less than 30 weeks gestation (2 excluded due to artifacts); who had abdominal NIRS placed from within 48 h of birth for the first 14 days of life. They demonstrated that daily mean srSO2 values decreased over the first 9 days of life (p < 0.0001), followed by increase from day 10–14 (p = 0.0061). The srSO2 was lower and FTOE higher in infants with feeding intolerance compared to those without (p = 0.0043). A higher srSO2 and variability was associated with a healthy gut (n = 17) and low srSO2 and decreased variability was noted in neonates with NEC (n = 2). NIRS has also been used to examine the relationship between red blood cell transfusion (RBCT) and gut injury to try and resolve the debate as to whether RBCT cause gut injury and NEC (27, 34) (Table 2). A recent case control study (n = 72) by Kalteren et al. (32) reported that RBCT were associated with concomitant signs of oxidative stress and intestinal injury as measured by raised levels of urinary 8-isoprostane and I-FABP respectively, along with lower variability in splanchnic oxygenation. The authors therefore postulated that this may represent the early pathogenetic process of transfusion-associated NEC. However, larger prospective trials are needed to substantiate conclusions as to whether this accurately reflects the onset of gut injury, as nearly all the current evidence is from non-randomised trials with only small study numbers.
Practical issues with using NIRS as a potential biomarker for NEC onset
While there is some promising evidence in the literature, it is not conclusive and there are several issues that we must address first before we can consider NIRS as a potential biomarker for NEC onset and these include:
Lack of normal ranges
Most importantly, there is currently no large population-based normative data for regional saturation of various organs in infants which therefore subsequently limits its use in identifying gut injury, until we have established normal splanchnic oxygenation for infants of various gestation and postnatal ages (2). There have been numerous studies looking into establishing normal ranges using the two most common NIRS monitors and there is an impact of gestational and postnatal age, but these studies were all relatively small (9, 15, 43–47) (Table 1). Furthermore, most studies, do not include the micropremies between 22 and 24 weeks gestation, and it is this subgroup that are the highest risk of the associated morbidity and mortality with NEC and potentially will benefit the most from new technologies such as NIRS in monitoring their haemodynamic status. However, without first establishing normal ranges for such infants, detecting deviations from the norm and onset of possible NEC is problematic. Therefore, research should focus on the use of routine NIRS in extreme preterm infants and micropremies, so we can start constructing normative ranges. A recent study by van der Heid et al. (16) has brought us one step further as they conducted a relatively large NIRS study on preterm infants (n = 220, <32 w and/or <1,500 g BW) and showed that gestational age, postnatal age, and small-for-gestational age status affect regional splanchnic oxygen saturation and need to be taken into account when interpreting regional splanchnic oxygen saturations using NIRS. In their study they provided a model so that reference values for infant regional splanchnic oxygen saturation can be computed with their formula based on those factors.
A further issue that hinders the availability of normal ranges is that there are differing locations used for “abdominal” NIRS measurements; all of these locations have been used in the available studies and there is no consistent placement; some use liver, some infra-umbilical and some supra-umbilical. At present no one has conclusively confirmed which is the more reliable for detecting changes in abdominal regional oxygenation, although the infra-umbilical region is the most used (Table 1). Perhaps more importantly when trying to establish normal ranges the measurement location used significantly influences the regional saturation measurement obtained and there is no correlation between the three locations (48). Therefore, in addition to having to review the data from studies in relation to the device used, clinicians must also be aware of the measurement location that was used for the measurements.
Cost and training implications
To consider NIRS as a possible NEC biomarker we must remember that for this to work NIRS monitors would need to be used routinely, which would require a significant expenditure for each neonatal unit as well as the need for training medical and nursing staff on how to use it and interpret the readings. However, it is not inconceivable that with the ever-increasing body of evidence regarding the usefulness and clinical application of NIRS in predicting changes in gut blood flow and gut injury that it may be cost effective to use NIRS. By allowing earlier detection of NEC and initiation of treatment the impact of this disease could be reduced by decreasing the inevitable neonatal intensive care cot days and, more importantly, the long-term health burden for affected infants.
Safety of NIRS
NIRS is transcutaneous, non-invasive and does not cause harm to patients, therefore it is appropriate to continue to explore its usefulness in predicting NEC. The light intensities used are not harmful to the tissue, particularly when only used for short periods; furthermore NIRS is not known to cause skin burns even if applied for a longer period (48, 49). Current sensors for neonates are well tolerated due to their smaller size and because they are lined with a skin friendly adhesive. To provide further skin protection in extremely premature patients probes can be attached to a light-permeable skin barrier without interference with measurements (9).
Accuracy of NIRS measurements
Before its use in human studies, NIRS was initially used in laboratory and subsequently animal studies (50, 51). NIRS has been validated by using a newborn piglet model where the carotid, renal and mesenteric arteries were occluded and then re-perfused which caused fast, simultaneous changes in rSO2 of the affected end-organs (51). NIRS has since been validated in adult intensive care patients, those undergoing ECMO or cardiac surgery by comparing central venous samples with NIRS values (52–56). Its effectiveness and validity in measuring splanchnic oxygenation in preterm infants and the clinical implications of this were extensively examined in a recent systematic review article by Seager et al. (57). In a study by Menke et al. (58) two observers repeated a total of 500 NIRS measurements in 25 neonates and they performed a baseline measurement to assess the physiological variation in every neonate. They reported that inter-patient variance contributed most to the total variance, while the interobserver variance had the smallest effect. They demonstrated that crSO2 showed a good reproducibility, with an inter-measurement variance slightly but not significantly higher than the physiological baseline variation. Banerjee et al. measured gut oxygenation using NIRS in 71 preterm infants (59 receiving blood transfusion and 12 controls) and reported that NIRS is a reliable method of measuring changes in splanchnic tissue oxygenation and therefore gut tissue perfusion (59).
However, there are many factors that can affect the accuracy of the rSO2 obtained including the specific device used but also, unsurprisingly, given it is transcutaneous, the placement of the sensor is important. Placement of the sensor over fatty deposits, hair, bony protuberances, nevi, hematomas or broken skin, or application of pressure to the sensor may result in inaccurate readings. For measuring splanchnic oxygenation there are other factors that inherently affect the measurement reliability (48) because the gut is moving, can be filled with varying degrees of gas or stool and is near the stomach and bladder, all of which can affect results, and all of these are exacerbated in preterm infants given their size. It has previously been described that meconium interferes with the NIRS measurements (60) and depending on the gestational and chronological age of preterm infants, and whether an ileus is present or not, the amount of meconium present in the bowel will differ significantly. The currently available NIRS devices do not account for the absorption by stool and given the absorption spectra of meconium this can alter the optical signal from the NIRS device meaning that the measured regional oxygenation value will differ depending on the amount of meconium present in the bowel.
In addition, movements of the infant and consequently pull on the NIRS probes are more likely. The measurement accuracy of splanchnic oxygenation is affected by the amount of gaseous distension and faecal matter present in the abdomen which in a preterm is continually changing as they mature, due to the initiation of enteral feeds and depends on the level of respiratory support they are requiring; with non-invasive methods such as CPAP and Vapotherm being well known to increase abdominal distension.
NIRS is also susceptible to motion artefact caused by relative motion between the NIRS optical fibers and the skin of the region where they are placed. Relative motion will cause a rapid shift in the optical coupling between the NIRS optical fibers and the body area where the probe is placed (e.g., scalp or abdomen), which typically results in a period of high-frequency noise in the recorded NIRS data. In preterm infants this motion can be unavoidable; some critically ill preterm infants require high frequency oscillation ventilation (HFOV) which because of the chest “wobble” from the vibrations can be transmitted to the abdomen as well. Furthermore, even in more stable preterm infants movement can affect NIRS readings; NIRS motion artefact has been reported to be approximately 15% (61). Motion artifacts in NIRS data are often relatively easy to identify using a combination of observation of the subject during NIRS recording and visual inspection of the resulting data. However, there is no universal approach to whether remove or correct these (62). In most NIRS studies around 10% of infants are excluded because of motion artefacts (63, 64), but there have been recent studies demonstrating that you can avoid needing to exclude any infants due to motion artefact by using consistent probe placement (31, 47).
The accuracy of NIRS information is also affected by light scattering, the concentration of haemoglobin (Hb) and the level of melanin and bilirubin in the skin (65, 66). In neonatal intensive care, an obvious difficulty is in the first few days of life most preterm infants become jaundiced and many need phototherapy. Overhead phototherapy and the bilirubin level itself will affect the NIRS readings and, in some cases, the sensors will not work at all if the light is too bright near the sensors. Changes in Hb levels in preterm infants over the first few weeks of life have been shown to change NIRS measurements by a significant amount; 30%–50% (9, 65–67). Therefore, these factors would need to be considered when interpreting any NIRS measurements.
Finally it is well known that there is a high variability of the NIRS measurements in the abdominal region, although this has been shown to decrease over time (9, 47, 48, 68) This high variability limits the accuracy of NIRS measurements for both the detection of NEC onset and establishing normal ranges. This higher variability seen at the abdominal region is to be expected given the inherent limitations we have already discussed, as there is a plethora of factors which can alter its accuracy and cause high variability, including gut peristalsis, faecal and air content, as well as the impact of nursing cares including nappy changes. There are emerging techniques looking at ways to improve the reliability and accuracy of NIRS measurements by reducing this variability and Isler et al. (60) recently published their study where they examined the optical properties of faeces of preterm infants to enable a more accurate measurement, considering the effect of stool on the NIRS measurements. Therefore, it is conceivable that in the future corrections such as this will be commercially available for all NIRS devices and subsequently allow for more accurate NIRS abdominal regional oxygenation measurements.
Summary of effectiveness
NIRS is non-invasive and safe (48, 49, 69); but the accuracy of splanchnic oxygenation measured is affected by the structure and content of the bowels (48). NIRS can detect alterations in splanchnic oxygenation and perfusion, potential allowing earlier recognition of bowel ischaemia and gut injury such as NEC than currently used methods. However, its widespread application is limited given that current literature evidence has involved mostly very small studies, using both term and preterm infants of various gestations and postnatal ages as well and with varying NIRS monitors (2). One limitation of NIRS is that repeated measures within subject standard deviation is about 5% to 6% (70) and there is a methodological bias between sensors from the two most commonly used monitors- INVOS-5100 and NIRO-300 (71) and therefore attention needs to be paid to the type of sensor used and the device used when interpreting any regional oxygenation results. Because authors report studies on regional oxygenation using different NIRS monitors the emphasis should be on using NIRS to report trends in regional oxygenation (72).
Conclusion
Despite advances in neonatal care, the severity of NEC and its morbidity and mortality remain high. As a neonatal community our research must focus on identifying this devastating disease prior to symptom onset.
Continuous splanchnic NIRS monitoring may benefit the clinical outcomes of preterm infants because NIRS has the potential to alert clinicians to when relative regional hypoxia occurs for an individual neonate allowing time for prompt interventions to ameliorate their hypoxia, and potentially reduce their long-term impairments. However further trials involving larger numbers of infants are needed to examine if NIRS monitoring coupled with intervention can improve outcomes. Perhaps the real answer is using a machine learning algorithm combining routine clinical, laboratory and gut tissue biomarkers along with NIRS on each individual preterm neonate in intensive care; therefore, we need larger scale observational studies and randomised trials combining NIRS with the more successful tissue biomarkers including intestinal fatty acid binding proteins (IFABPs), which are the most studied gut biomarker, and other clinical parameters to review their effectiveness in predicting NEC onset in larger numbers of infants.
While there is currently no conclusive evidence regarding NIRS and its ability to predict NEC there are promising small studies and the option of having a non-invasive method of monitoring gut injury is an exciting concept and justifies further research in this area.
Author contributions
The idea for this article was conceptualised by CH, NA and JB. CH wrote the original manuscript and this was then reviewed and edited by NA, JB, and TL. All authors contributed to the article and approved the submitted version.
Conflict of interest
The authors declare that the research was conducted in the absence of any commercial or financial relationships that could be construed as a potential conflict of interest.
Publisher's note
All claims expressed in this article are solely those of the authors and do not necessarily represent those of their affiliated organizations, or those of the publisher, the editors and the reviewers. Any product that may be evaluated in this article, or claim that may be made by its manufacturer, is not guaranteed or endorsed by the publisher.
References
1. Costeloe K, Hardy P, Juszczak E, Wilks M, Millar MR. Bifidobacteriu, breve BBG-001 in very preterm infants: a randomised controlled phase 3 trial. Lancet. (2016) 387:649–60. doi: 10.1016/S0140-6736(15)01027-2
2. Sood BG, McLaughlin K, Cortez J. Near-infrared spectroscopy: applications in neonates. Semin Fetal Neonatal Med. (2015) 20(3):164–72. doi: 10.1016/j.siny.2015.03.008
3. Cohn SM, Crookes BA, Proctor KG. NIRS In resuscitation. J Trauma. (2003) 54:S199–S202. doi: 10.1097/00005373-200301000-00029
4. Ghosh A, Elwell C, Smith M. Review article: cerebral near-infrared spectroscopy in adults: a work in progress. Anesth Analg. (2012) 115(6):1373–83. doi: 10.1213/ANE.0b013e31826dd6a6
5. Chakravarti S, Srivastava S, Mittnacht AJ. Near infrared spectroscopy (NIRS) in children. Semin Cardiothorac Vasc Anesth. (2008) 12:70–9. doi: 10.1177/1089253208316444
6. van Vonderen JJ, Roest AAW, Siew ML, Walther FJ, Hooper SB, te Pas AB. Measuring physiological changes during the transition to life after birth. Neonatology. (2014) 105:230–42. doi: 10.1159/000356704
7. Vretzakis G, Georgopoulou S, Stamoulis K, Stamatiou G, Tsakiridis K, Zarogoulidis P, et al. Cerebral oximetry in cardiac anesthesia. J Thorac Dis. (2014) 6(Suppl. 1):S60–9. doi: 10.3978/j.issn.2072-1439.2013.10.2
8. Hoffman G, Stuth E, Berens R. Two-site NIRS transcutaneous oximetry as a non-invasive indicator of mixed venous oxygen saturation in cardiac neonates. Anesthesiology. (2003) 99(A1393). doi: 10.3978/j.issn.2072-1439.2013.10.22
9. McNeill S, Gatenby JC, McElroy S, Engelhardt B. Normal Cerebral, renal and abdominal regional oxygen saturations using near-infrared spectroscopy in preterm infants. J Perinatol. (2011) 31(1):51–7. doi: 10.1038/jp.2010.71
10. Alderliesten T, van Bel F, van der Aa NE, Steendijk P, van Haastert IC, de Vries LS, Groenendaal F, Lemmers P. Low cerebral oxygenation in preterm infants is associated with adverse neurodevelopmental outcome. J Pediatr. (2019) 207:109–16. doi: 10.1016/j.jpeds.2018.11.038
11. Balegar KK, Stark MJ, Briggs N, Andersen CC. Early cerebral oxygen extraction and the risk of death or sonographic brain injury in very preterm infants. J Pediatr. (2014) 164(3):475–80.e1. doi: 10.1016/j.jpeds.2013.10.041
12. Sørensen L, Maroun L, Børch K, Lou H, Greisen G. Neonatal cerebral oxygenation is not linked to foetal vasculitis and predicts intraventricular haemorrhage in preterm infants. Acta Paediatr. (2008) 97(11):1529–34. doi: 10.1111/j.1651-2227.2008.00970.x
13. Toet MC, Lemmers PM, van Schelven LJ, van Bel F. Cerebral oxygenation and electrical activity after birth asphyxia: their relation to outcome. Pediatrics. (2006) 117:333–9. doi: 10.1542/peds.2005-0987
14. Verhagen EA, ter Horst HJ, Keating P, Martijn A, Van Braeckel KNJA, Bos AF. Cerebral oxygenation in preterm infants with germinal matrix-intraventricular hemorrhages. Stroke. (2010) 41(12):2901–7. doi: 10.1161/STROKEAHA.110.597229
15. Cortez J, Gupta M, Amaram A, Pizzino J, Sawhney M, Sood B. Non invasive evaluation of splanchnic tissue oxygenation using near-infrared spectroscopy in preterm neonates. J Matern Fetal Neonatal Med. (2011) 24:574–82. doi: 10.3109/14767058.2010.511335
16. van der Heide M, Dotinga BM, Stewart RE, Kalteren WS, Hulscher JBF, Reijneveld SA, Bos AF, Kooi EMW. Regional splanchnic oxygen saturation for preterm infants in the first week after birth: reference values. Pediatr Res. (2021) 90(4):882–7. doi: 10.1038/s41390-020-01323-3
17. Calderon B, Rojas-Scheffer L, Encinas Jose, Ruza F. Monitoring splanchnic hypoperfusion with near-infrared spectroscopy in a rabbit model of septic shock. Med Sci Technol. (2016) 57:29–37. doi: 10.12659/MST.896517
18. Meier SD, Eble BK, Stapleton GE, Morales DL, Chang AC, Andropoulos DB. Mesenteric oxyhemoglobin desaturation improves with patent ductus arteriosus ligation. J Perinatol. (2006) 26:562–4. doi: 10.1038/sj.jp.7211559
19. Petrova A, Bhatt M, Mehta R. Regional tissue oxygenation in preterm born infants in association with echocardiographically significant patent ductus arteriosus. J Perinatol. (2011) 31:460–4. doi: 10.1038/jp.2010.200
20. Braski K, Weaver-Lewis K, Loertscher M, Ding Q, Sheng X, Baserga M. Splanchnic-cerebral oxygenation ratio decreases during enteral feedings in anemic preterm infants: observations under near-infrared spectroscopy. Neonatology. (2018) 113(1):75–80. doi: 10.1159/000481396
21. Corvaglia L, Martini S, Battistini B, Rucci P, Aceti A, Faldella G. Bolus vs. continuous feeding: effects on splanchnic and cerebral tissue oxygenation in healthy preterm infants. Pediatr Res. (2014) 76(1):81–5. doi: 10.1038/pr.2014.52
22. Gillam-Krakauer M, Cochran CM, Slaughter JC, Polavarapu S, McElroy SJ, Hernanz-Schulman M, Engelhardt B. Correlation of abdominal rSO2 with superior mesenteric artery velocities in preterm infants. J Perinatol. (2013) 33(8):609–12. doi: 10.1038/jp.2013.3
23. Martini S, Aceti A, Beghetti I, Faldella G, Corvaglia L. Feed-related splanchnic oxygenation in preterm infants with abnormal antenatal Doppler developing gut complications. J Pediatr Gastroenterol Nutr. (2018) 66:755–9. doi: 10.1097/MPG.0000000000001804
24. Bailey SM, Hendricks-Muñoz KD, Wells JT, Mally P. Packed red blood cell transfusion increases regional cerebral and splanchnic tissue oxygen saturation in anemic symptomatic preterm infants. Am J Perinatol. (2010) 27(6):445–53. doi: 10.1055/s-0030-1247598
25. Dani C, Pratesi S, Fontanelli G, Barp J, Bertini G. Blood transfusions increase cerebral, splanchnic, and renal oxygenation in anemic preterm infants. Transfusion. (2010) 50(6):1220–6. doi: 10.1111/j.1537-2995.2009.02575.x
26. Mintzer JP, Parvez B, Chelala M, Alpan G, LaGamma EF. Monitoring regional tissue oxygen extraction in neonates <1250 g helps identify transfusion thresholds independent of hematocrit. J Neonatal Perinatal Med. (2014) 7:89–100. doi: 10.3233/NPM-1477213
27. Sood BG, Cortez J, Mclaughlin K, Gupta M, Amaram A, Kolli M, et al. Near infrared spectroscopy as a biomarker for necrotizing enterocolitis following red blood cell transfusion. J Near InfraRed Spectrosc. (2014) 22(6):375–88. doi: 10.1255/jnirs.1135
28. Bailey SM, Hendricks-Muñoz KD, Mally P. Splanchnic-cerebral oxygenation ratio as a marker of preterm infant blood transfusion needs. Transfusion. (2012) 52:252–60. doi: 10.1111/j.1537-2995.2011.03263.x
29. Fortune PM, Wagstaff M, Petros AJ. Cerebro-splanchnic oxygenation ratio (CSOR) using near infrared spectroscopy may be able to predict splanchnic ischaemia in neonates. Intensive Care Med. (2001) 27(8):1401–7. doi: 10.1007/s001340100994
30. Gay AN, Lazar DA, Stoll B, Naik-Mathuria B, Mushin OP, Rodriguez MA, Burrin DG, Olutoye OO. Near-infrared spectroscopy measurement of abdominal tissue oxygenation is a useful indicator of intestinal blood flow and necrotizing enterocolitis in premature piglets. J Pediatr Surg. (2011) 46(6):1034–40. doi: 10.1016/j.jpedsurg.2011.03.025
31. Howarth C, Banerjee J, Leung T, Eaton S, Morris JK, Aladangady N. Cerebral oxygenation in preterm infants with necrotizing enterocolitis. Pediatrics. (2020) 146(3):e20200337. doi: 10.1542/peds.2020-0337
32. Kalteren WS, Bos AF, Bergman KA, van Oeveren W, Hulscher JBF, Kooi EMW. The short-term effects of RBC transfusions on intestinal injury in preterm infants. Pediatr Res. (2022):1–7. doi: 10.1038/s41390-022-01961-9
33. Le Bouhellec J, Prodhomme O, Mura T, Jacquot A, Combes C, Gamon L, et al. Near-infrared spectroscopy: a tool for diagnosing necrotizing enterocolitis at onset of symptoms in preterm neonates with acute gastrointestinal symptoms? Am J Perinatol. (2021) 38(S 01):e299–e308. doi: 10.1055/s-0040-1710033
34. Marin T, Moore J, Kosmetatos N, Roback JD, Weiss P, Higgins M, McCauley L, Strickland OL, Josephson CD. Red blood cell transfusion –related necrotising enterocolitis in very low birthweight infants: a near infrared spectroscopy investigation. Transfusion. (2013) 53:2650–8. doi: 10.1111/trf.12158
35. Patel AK, Lazar DA, Burrin DG, Smith EO, Magliaro TJ, Stark AR, Brandt ML, Zamora IJ, Sheikh F, Akinkuotu AC, Olutoye OO. Abdominal near infrared spectroscopy measurements are lower in preterm infants at risk for necrotizing enterocolitis. Pediatr. Crit. Care Med. (2014) 15:735–41. doi: 10.1097/PCC.0000000000000211
36. Schat TE, Schurink M, van der Laan ME, Hulscher JB, Hulzebos CV, Bos AF, Kooi EM. Near-infrared spectroscopy to predict the course of necrotizing enterocolitis. PLoS ONE. (2016) 11(5):e0154710. doi: 10.1371/journal.pone.0154710
37. Schat TE, van Zoonen AGJF, van der Laan ME, Mebius MJ, Bos AF, Hulzebos CV, Boezen HM, Hulscher JBF, Kooi EMW. Early cerebral and intestinal oxygenation in the risk assessment of necrotizing enterocolitis in preterm infants. Early Hum Dev. (2019) 131:75–80. doi: 10.1016/j.earlhumdev.2019.03.001
38. Stapleton GE, Eble BK, Dickerson HA, Andropoulos DB, Chang AC. Mesenteric oxygen desaturation in an infant with congenital heart disease and necrotizing enterocolitis. Tex Heart Inst J. (2007) 34(4):442–4. PMID: 18172526; PMCID: PMC2170498.18172526
39. Zabaneh RN, Cleary JP, Lieber CA. Mesentric oxygen saturations in premature twins with and without necrotizing enterocolitis. Pediatr Crit Care Med. (2011) 12:e404–6. doi: 10.1097/PCC.0b013e3181fe4278
40. Banerjee J, Leung T, Aladangady NA. Is Near Infrared Spectroscopy a reliable technique to measure gut perfusion in preterm infants? J Paediatr Neonatal Individualized Med. (2017) 6(2):e060244. doi: 10.7363/060244
41. Howarth C. The effect of anaemia and red blood cell transfusion on gut tissue injury in preterm infants. In: Blizard Institute (2021). QMUL. 375 p.
42. Howarth C, Morris J, Mifsud C, Banerjee J, Leung T, Eaton S, et al. 3rd congress of joint European neonatal societies (jENS 2019): near infrared spectroscopy and gut biomarkers in preterm infants—can they predict NEC? (abstract 44). Pediatr Res. (2019) 86:1–64. doi: 10.1038/s41390-019-0521-6
43. Naulaers G, Morren G, Van Huffel S, Casaer P, Devlieger H. Cerebral tissue oxygenation index in very premature infants. Arch Dis Child Fetal Neonatal Ed. (2002) 87:F189–92. doi: 10.1136/fn.87.3.F189
44. Baik N, Urlesberger B, Schwaberger B, Schmölzer GM, Mileder L, Avian A, Pichler G. Reference ranges for regional cerebral tissue oxygen saturation and fractional oxygen extraction in neonates during immediate transition after birth. J Pediatr. (2013) 163:1558–63. doi: 10.1016/j.jpeds.2013.07.007
45. Roche-Labarbe N, Surova A, Carp S, Patel M, Boas D, Grant P, et al.. Noninvasive optical measures of CBV, StO2, CBF index, and rCMRO2 in human premature neonates’ brains in the first six weeks of life. Hum Brain Mapp. (2010) 31:341–52. doi: 10.1002/hbm.20868
46. Takami T, Sunohara D, Kondo A, Mizukaki N, Suganami Y, Takei Y, Miyajima T, Hoshika A. Changes in cerebral perfusion in extremely LBW infants during the first 72 h after birth. Pediatr Res. (2010) 68(5):435–9. doi: 10.1203/PDR.0b013e3181f2bd4d
47. Howarth CN, Leung TS, Banerjee J, Eaton S, Morris JK, Aladangady N. Regional cerebral and splanchnic tissue oxygen saturation in preterm infants—longitudinal normative measurements. Early Hum Dev. (2022) 165:105540. doi: 10.1016/j.earlhumdev.2022.105540
48. Schat TE, van der Laan ME, Schurink M, Hulscher JB, Hulzebos CV, Bos AF, Kooi EM. Abdominal near-infrared spectroscopy in preterm infants: a comparison of splanchnic oxygen saturation measurements at two abdominal locations. Early Hum Dev. (2014) 90(7):371–5. doi: 10.1016/j.earlhumdev.2014.04.008
49. Scheeren TW, Schober P, Schwarte LA. Monitoring tissue oxygenation by near infrared spectroscopy (NIRS): background and current applications. J Clin Monit Compu. (2012) 26:279–87. doi: 10.1007/s10877-012-9348-y
50. Kurth CD, Liu H, Thayer WS, Chance B. A dynamic phantom brain model for NIRS. Phys Med Biol. (1995) 40:20792092. doi: 10.1088/0031-9155/40/12/006
51. Wider M. Hemodynamic management and regional hemoglobin oxygen saturation (rSO2) of the brain, kidney and gut. Neonatal Intensive Care. (2009) 22(5):57-60. https://nicmag.ca/pdf/NIC-22-5-SEP09-web.pdf
52. Abdul-Khaliq H, Troitzsch D, Schubert S, Wehsack A, Böttcher W, Gutsch E, Hübler M, Hetzer R, Lange PE. Cerebral oxygen monitoring during neonatal cardiopulmonary bypass and deep hypothermic circulatory arrest. Thorac Card Surg. (2002) 50:77–81. doi: 10.1055/s-2002-26698
53. Benni PB, Chen B, Dykes FD, Wagoner SF, Heard M, Tanner AJ, Young TL, Rais-Bahrami K, Rivera O, Short BL. Validation of the CAS neonatal NIRS system by monitoring vvECMO patients: preliminary results. Adv Exp Med Biol. (2005) 566:195–201. doi: 10.1007/0-387-26206-7_27
54. Nagdyman N, Fleck T, Barth S, Abdul-Khaliq H, Stiller B, Ewert P, Huebler M, Kuppe H, Lange PE. Relation of cerebral tissue oxygenation index to central venous oxygen saturation in children. Intensive Care Med. (2004) 30(3):468–71. doi: 10.1007/s00134-003-2101-8
55. Rais-Bahrami K, Rivera O, Short BL. Validation of a non invasive neonatal cerebral oximeter in venous venous ECMO patients. J Perinatol. (2006) 26:628–35. doi: 10.1038/sj.jp.7211573
56. Weiss M. NIRS cerebral oxygenation reading in neonates and infants and SvO2. Ped Anesth. (2005) 15:102–9. doi: 10.1111/j.1460-9592.2005.01404.x
57. Seager E, Longley C, Aladangady N, Banerjee J. Measurement of gut oxygenation in the neonatal population using near-infrared spectroscopy: a clinical tool? Arch Dis Child Fetal Neonatal Ed. (2019) 105(1):76–86. doi: 10.1136/archdischild-2018-316750
58. Menke J, Voss U, Möller G, Jorch G. Reproducability of NIRS in neonates. Biol Neonate. (2003) 83:6–11. doi: 10.1159/000067006
59. Banerjee J, Leung TS, Aladangady NA. Is Near Infrared Spectroscopy a reliable technique to measure gut perfusion in preterm infants? in Second Congress of Joint European Neonatal Society Meeting (jENS). (2017). Venice.
60. Isler H, Schenk D, Bernhard J, Kleiser S, Scholkmann F, Ostojic D, Kalyanov A, Ahnen L, Wolf M, Karen T. Absorption spectra of early stool from preterm infants need to be considered in abdominal NIRS oximetry. Biomed Opt Express. (2019) 10(6):2784–94. doi: 10.1364/BOE.10.002784
61. Aladangady N, Leung T, Costeloe K, Delpy D. Measuring circulating blood volume in newborn infants using pulse dye densitometry and indocyanine green. Paediatr Anaesth. (2008) 18(9):865. doi: 10.1111/j.1460-9592.2008.02647.x
62. Cooper RJ, Selb J, Gagnon L, Phillip D, Schytz HW, Iversen HK, Ashina M, Boas DA. A systematic comparison of motion artifact correction techniques for functional near-infrared spectroscopy. Front Neurosci. (2012) 6(147):1–10. doi: 10.3389/fnins.2012.00147
63. Banerjee J, Leung TS, Aladangady N. Cerebral blood flow and oximetry response to blood transfusion in relation to chronological age in preterm infants. Early Hum Dev. (2016) 97:1–8. doi: 10.1016/j.earlhumdev.2015.10.017
64. Banerjee J, Leung TS, Aladangady N. Effect of blood transfusion on intestinal blood flow and oxygenation in extremely preterm infants during first week of life. Transfusion. (2016) 56(4):808–15. doi: 10.1111/trf.13434
65. Wassenaar EB, Van den Brand JG. Reliability of NIRS in people with dark skin pigmentation. J Clin Monit Comput. (2005) 19:195–9. doi: 10.1007/s10877-005-1655-0
66. Madsen PL, Skak C, Rasmussen A, Secher NH. Interference of cerebral near-infrared oximetry in patients with icterus. Anesth Analg. (2000) 90:489–93. doi: 10.1213/00000539-200002000-00046
67. Ferrari M, Mottola L, Quaresima V. Principles, techniques and limitations of NIRS. Can J Appl Physiol. (2004) 29:464–87. doi: 10.1139/h04-031
68. Mintzer JP, Parvez B, Chelala M, Alpan G, LaGamma EF. Quiescent variability of cerebral, renal, and splanchnic regional tissue oxygenation in very low birth weight neonates. J Neonatal Perinatal Med. (2014) 7:199–206. doi: 10.3233/NPM-14814035
69. International Health Terminology Standards Development Organisation. SNOMED CT. (2012). Available from: http://www.ihtsdo.org/snomed-ct
70. Sorensen LC, Greisen G. Precision of measurement of cerebral tissue oxygenation index using near-infrared spectroscopy in preterm neonates. J Biomed Opt. (2006) 11(5):054005. doi: 10.1117/1.2357730
71. Dullenkopf A, Frey B, Baenziger O, Gerber A, Weiss M. Measurement of cerebral oxygenation state in anaesthetized children using the INVOS 5100 cerebral oximeter. Pediatr Anesth. (2003) 13(5):384–91. doi: 10.1046/j.1460-9592.2003.01111.x
Keywords: NEC, biomarker, gut oxygenation, tissue injury, ischaemia, NIRS, regional oxygenation
Citation: Howarth C, Banerjee J, Leung T and Aladangady N (2022) Could Near Infrared Spectroscopy (NIRS) be the new weapon in our fight against Necrotising Enterocolitis?. Front. Pediatr. 10:1024566. doi: 10.3389/fped.2022.1024566
Received: 21 August 2022; Accepted: 17 October 2022;
Published: 8 November 2022.
Edited by:
Gunnar Naulaers, KU Leuven, BelgiumReviewed by:
Troy Markel, Indiana University, United StatesBerndt Urlesberger, Medical University of Graz, Austria
© 2022 Howarth, Banerjee, Leung and Aladangady. This is an open-access article distributed under the terms of the Creative Commons Attribution License (CC BY). The use, distribution or reproduction in other forums is permitted, provided the original author(s) and the copyright owner(s) are credited and that the original publication in this journal is cited, in accordance with accepted academic practice. No use, distribution or reproduction is permitted which does not comply with these terms.
*Correspondence: Claire Howarth claire.howarth@nhs.net
Specialty Section: This article was submitted to Neonatology, a section of the journal Frontiers in Pediatrics