- 1Division of Human Nutrition, Department of Global Health, Faculty of Medicine and Health Sciences, Stellenbosch University, Cape Town, South Africa
- 2Infection Control Africa Network – ICAN, Cape Town, South Africa
- 3Department of Paediatrics and Child Health, Faculty of Medicine and Health Sciences, Stellenbosch University, Cape Town, South Africa
- 4Division of Medical Microbiology, University of Stellenbosch, Cape Town, South Africa
- 5National Health Laboratory Service, Tygerberg Hospital, Cape Town, South Africa
- 6Department of Epidemiology and Data Science, Amsterdam UMC, Amsterdam, Netherlands
- 7Department of Pediatrics, Amsterdam UMC, VU University Medical Center, Amsterdam, Netherlands
Background: Infections caused by drug resistant Gram-negative bacteria (DR-GNB) are a major health concern for hospitalized preterm neonates, globally. The aim of this study was to investigate the effect of a multi-strain probiotic on the incidence of rectal colonization with DR-GNB in preterm neonates.
Methods: A double-blind, placebo-controlled, randomized clinical trial was conducted including 200 neonates, randomly allocated to a multi-strain probiotic (n = 100) or placebo (n = 100).
Results: Fifteen percent of the neonates showed peri-rectal colonization with DR-GNB on the day of enrolment indicating probable maternal-to-neonate (vertical) bacterial transmission or environmental acquisition at time of delivery, with no difference between groups. Acquisition of further DR-GNB colonization was rapid, with an increase from 15% on the day enrolment to 77% by day 7 and 83% by day 14 of life. By day 7 (corresponding to early gut colonization), neonates in the probiotic group were 57% less likely to have peri-rectal DR-GNB colonization [OR: 0.43 (0.20–0.95); p = 0.04] and by day 14 (corresponding to late gut colonization), neonates in the probiotic group were 93% less likely to have peri-rectal DR-GNB colonization [OR: 0.07 (0.02–0.23); p < 0.001].
Conclusion: Hospitalized neonates showed substantial peri-rectal colonization with DR-GNB at enrolment and further rapid acquisition of DR-GNB in the first 2 weeks of life. The use of a multi-strain probiotic was effective in reducing early and late neonatal gut colonization with DR-GNB.
Clinical Trial Registration: The trial was registered at the Pan African Clinical Trial Registry (PACTR202011513390736).
Introduction
Globally infections caused by drug resistant Gram-negative bacteria (DR-GNB) have increased significantly and are a leading public health concern, especially in neonates (1, 2). Both early-onset and healthcare-associated infections (HAI) can be caused by antimicrobial resistant (AMR) bacteria (3, 4). In low-and-middle income countries (LMIC), most neonatal HAI-blood stream infection (BSI) episodes are caused by GNB, with high rates of infection-attributable mortality, morbidity, and increased healthcare costs (5, 6).
The most frequently cultured Gram-negative (GN) pathogens from neonatal BSI include Klebsiella pneumoniae, Escherichia coli, Enterobacter spp., Serratia marcescens, Acinetobacter baumannii and Pseudomonas aeruginosa (7–9). Factors contributing to neonatal colonization and/or infection with GN pathogens include poor compliance with hand hygiene, equipment and environmental cleaning recommendations, overcrowding, low nurse to patient ratios, overuse of broad spectrum antibiotics and delay in introducing breastmilk (10–12). A recent study in the neonatal wards at Tygerberg Hospital, South Africa showed that the highest aerobic colony counts were seen in moist surfaces, e.g., sinks, milk kitchen surfaces, humidifiers, and suction tubing. The organisms cultured were mainly Enterococci, S. marcescens, K. pneumoniae, S. aureus and A. baumannii (11). Due to their prematurity and absence of a suckling reflex, preterm neonates are mainly fed via a feeding tube. A review by Parker et al. indicated that feeding tubes can potentially contain pathogenic as well as antibiotic resistant bacteria (13).
A diverse community of organisms can be found in the gastrointestinal tract, referred to as the gut microbiome. Gut colonization is often delayed in preterm neonates, and these neonates also have limited number of species present (14). The most frequent species first seen in preterm neonates are Enterobacteria and Streptococci with a delayed colonization with Bifidobacteria (15). The lower the gestational age at birth, the slower the microbiome development and the lower the likelihood of Bifidobacteria predominance of the gut microbiome (16). Analysis of microflora in stool samples of extremely premature infants showed that the microflora of preterm infants changes progressively during the first 8 weeks of life, but that the diversity is exceptionally low. Concerning is the colonization with Staphylococci during the first 4 weeks of life, which poses an elevated risk for late onset sepsis (17). An increased risk of both necrotizing enterocolitis (NEC) and late-onset sepsis (LOS) have been observed in neonates with an altered microbiome (18). A predominance of aerobic cocci and reduced abundance of Bifidobacteria increases the risk of sepsis, while an increase in Enterobacter spp. and other Proteobacteria and reduction or absence of Bifidobacteria increases the risk of NEC and sepsis (16).
The large intestine can be a reservoir for many potential HAI pathogens including Enterobacterales, Enterococci, Clostridioides difficile and Candida species. Human-to-human spread of resistant bacteria can occur (19). Stephens et al. indicated that entire microbial communities and antibiotic-resistant bacteria can be spread via fomite transmission (20). Clinical infection may occur following intestinal acquisition of these pathogens, particularly in the presence of disruption to the gastrointestinal epithelial integrity and overgrowth of gut pathogen (19, 21). These conditions occur more frequently in preterm and low birth weight neonates, and in neonates with indwelling devices, prolonged hospital stay and prior antimicrobial use (21–23). In addition, most GN pathogens in LMIC exhibit AMR, making the treatment of HAI episodes difficult, e.g., extended beta-lactamase (ESBL) producing strains of Klebsiella pneumonia and Escherichia coli (24) and more recently emergence of carbapenem-resistant Enterobacterales (25).
A probiotic, as defined by the World Health Organization (WHO) is a live organism, which provides a benefit to the host when provided in adequate quantities (26). Probiotics can either be transient colonizers that alter the environment to allow for more permanent colonization by important commensal species or colonize the gut and modulate the gut microbiota (27, 28). They compete with other bacteria for nutrients and space, promote mucosal barrier function, inhibit mucosal pathogen adherence, and interact with the innate and adaptive immune system. Furthermore, probiotics may help to decolonize intestinal carriage of DR-GNB, through the production of antimicrobial substances, nitric oxide, and hydrolysis of pathogen receptors (29, 30). The ecosystem services framework can be applied to evaluate the neonatal gut microbiome. Through this system the goods and services obtained from the neonatal gut microbiome, can be categorized as supporting (capacity to colonize the gut), provisioning (ability of access and metabolize resources), or regulating (establish stable populations) (31).
In a systematic review and meta-analysis, Dermyshi et al. showed that probiotics (a single Lactobacillus species or a mixture of 2–3 bacterial species) reduced neonatal BSI rates by 12% and 19% respectively (32). Robertson et al. showed that a combination of Lactobacillus and Bifidobacterium supplementation led to a decreased incidence of both NEC and LOS (33). Although the modest benefits of probiotics for neonatal BSI prevention are promising, the optimal microbial strains, combinations, dosing, timing, and duration of supplementation, has not been definitively elucidated. Furthermore, it is not known whether probiotic supplementation in preterm neonates may modify the type, rate of acquisition, and antimicrobial-resistance patterns of perirectal colonizing GNB. We conducted a sub-study nested within a randomized controlled trial (RCT) to answer the question: does administration of a multi-strain probiotic reduce perirectal colonization with DR-GNB in preterm neonates?
Methods
Study design
A double-blind, placebo-controlled, randomized clinical trial was conducted.
Study setting
The study was conducted in Tygerberg Hospital (TBH), Cape Town, South Africa. TBH has a capacity of 1,384 beds, with 132 neonatal beds including a 12-bed medical/surgical neonatal intensive care unit (NICU). Participants were recruited over a 6-month period (19 January to 27 June 2021).
Study participants
Postnatally male and female preterm neonates, with a birth weight between 750 and 1,500 g and a gestational age <37 weeks were recruited, within 72 h of life. Neonates with major congenital malformations (including major gastro-intestinal abnormalities or surgery of the gastro-intestinal tract), birth asphyxia/neonatal encephalopathy, early onset sepsis [C-reactive protein (CRP) >10 mg/L in the first 72 h of life] and preterm neonates up for adoption, were excluded. Written parental informed consent was obtained.
Sampling and randomization
Consecutive sampling was used: every preterm neonate meeting the inclusion criteria was selected for inclusion until the required sample size (200 neonates) was achieved. A pre-determined randomization sequence obtained from a statistician at Stellenbosch University was used to randomly allocate neonates to either the probiotic (intervention) group (n = 100) or placebo group (n = 100). The manufacturer of the probiotic packaged the products (probiotic and placebo) in identical fashion, except for a distinguishing pink and green sticker to facilitate allocation concealment. The randomization list indicated in series whether the infant should be allocated to the pink or green group. Every participant received their own product bottle, marked with their unique hospital sticker, in order to avoid cross-contamination and to ensure that the correct treatment was administered every day. The researcher, neonatal ward staff and laboratory staff were blinded to the allocation of probiotic versus placebo.
Procedures
The probiotic used consists of Lactobacillus acidophilus, Bifidobacterium bifidum and Bifidobacterium infantis marketed as Labinic™ (Biofloratech, Surrey, United Kingdom). A standard dose of 0.2 ml was administered daily, providing approximately 2 billion colony forming units (CFUs) per day (approximately 0,67 × 109 CFUs of each of the three organisms). The placebo consisted of medium chain triglyceride (MCT) oil and Aerosil 200, a stabilizer also used in Labinic.
Supplementation with the probiotic or placebo was initiated with the start of oral feeds. Supplementation was delayed if the neonate was nil per os (NPO) and discontinued if a neonate developed necrotizing enterocolitis (NEC) (Bells stage II or more). The probiotic/placebo was administered once daily in the neonate's feed (mother's own breast milk/donor breast milk/infant formula) before administration of the feed via an orogastric tube or if applicable, orally. Neonates were followed up from birth to a maximum of 28 days or death or discharge to peripheral hospitals or home, whichever came first. At this time point supplementation was discontinued.
Data collected at enrolment included estimated gestational age (early/late ultrasound or foot length), gender, birth weight, type of delivery and Apgar scores. Medical notes and laboratory data were reviewed daily, and data collected on type and volume of feeds received, anthropometric measurements, medication prescribed and when Kangaroo Mother Care (KMC) was initiated.
Microbiological sampling and analysis
Peri-rectal swabs were collected at enrolment within 72 h of birth (day of enrolment), day 7 of life and day 14 of life reflecting baseline colonization status (probable maternally derived organism acquisition), early gut colonization and late gut colonization respectively. Lautenbach et al. indicated that peri-rectal swabs have a high sensitivity (90%) as well as specificity (100%) to correctly identify GNB (E. coli in their study) compared to stool samples (19). Since our study population was preterm infants, peri-rectal swabs would be the least invasive method to identify the DR-GNB. Sigma transwabs (MWE medical wire, United Kingdom) were used to perform peri-rectal swabs. The swab was pre-moistened in the Amies liquid transport medium of the swab tube and then swabbed twice around the peri-rectal area while rotating the swab tip. Swab tips were inoculated onto selective media (ChromID ESBL and ChromID CarbaSmart, BioMerieux, France) for isolation of ESBL- and carbapenem resistant GNB [including carbapenemase-producing Enterobacterales (CPE)], respectively. The plates were incubated aerobically for 18–24 h at 35°C and read the next morning. The presence of ESBL- and carbapenem-resistant organisms was indicated by the growth of pigmented colonies on the agar plate. The organism was then re-cultured on blood agar for 18–24 h at 35°C to ensure a pure colony. The organisms were then further identified to species level using API10E strips (BioMerieux, France). The manufacturer instructions were followed.
Study outcomes
The primary outcome was acquisition and colonization by day 14 post-enrolment with ESBL- and/or carbapenem-resistant GNB (for the purposes of this study collectively referred to as drug resistant GNB (DR-GNB).
Statistical analysis
The total sample size of the main study was 200, with 100 neonates per group (treatment and placebo groups). It was estimated using a published decrease (17%) in the proportion of perirectal colonization with drug-resistant bacteria (34). This sample size was estimated to detect a significant difference between the groups being compared (with Type I error at 0.05 and power at 80%). The total sample size required was allowing a 12% margin for study participant lost-to-follow-up. Continuous and categorical variables were compared using student t tests and Chi square tests respectively. A p-value below 0.05 was considered statistically significant. Logistic generalized estimating equations (GEE) were used to calculate the odds ratios for acquiring DR-GNB peri-rectal colonization by day 7 and day 14 post-enrolment. Both crude and adjusted analyses were performed. Adjustments were made for sepsis risk (infants with a CRP >10 mg/L and positive blood culture), HIV exposure, delivery mode, birthweight, gestational age, day of start of feeds, day of start of KMC and antibiotic use. Finally, we investigated whether the effect of probiotic supplementation on the presence of peri-rectal colonization with DR-GNB on average over time was different for infants with a different gestational age by adding the interaction between gestational age and product to the GEE analysis.
Ethical approval
Ethical approval was granted by the Human Research Ethics Committee of the Faculty of Health Sciences of Stellenbosch University as well as Tygerberg Academic Hospital (S20/07/178). The trial was also registered at the Pan African Clinical Trial Registry (PACTR202011513390736).
Role of the funding source
The funders of the trial had no role in the trial design, data collection, data analysis, data interpretation or writing of the report.
Results
Study participants
A total of 709 neonates were screened for eligibility, after excluding the neonates that did not meet the inclusion criteria, 200 neonates were enrolled in the study (100 in each of the probiotic and placebo groups) (Figure 1). Of the 200 enrolled neonates, 163 (82%) completed the first 14 days of the study, 80 (80%) in the probiotic arm and 83 (83%) in the placebo arm.
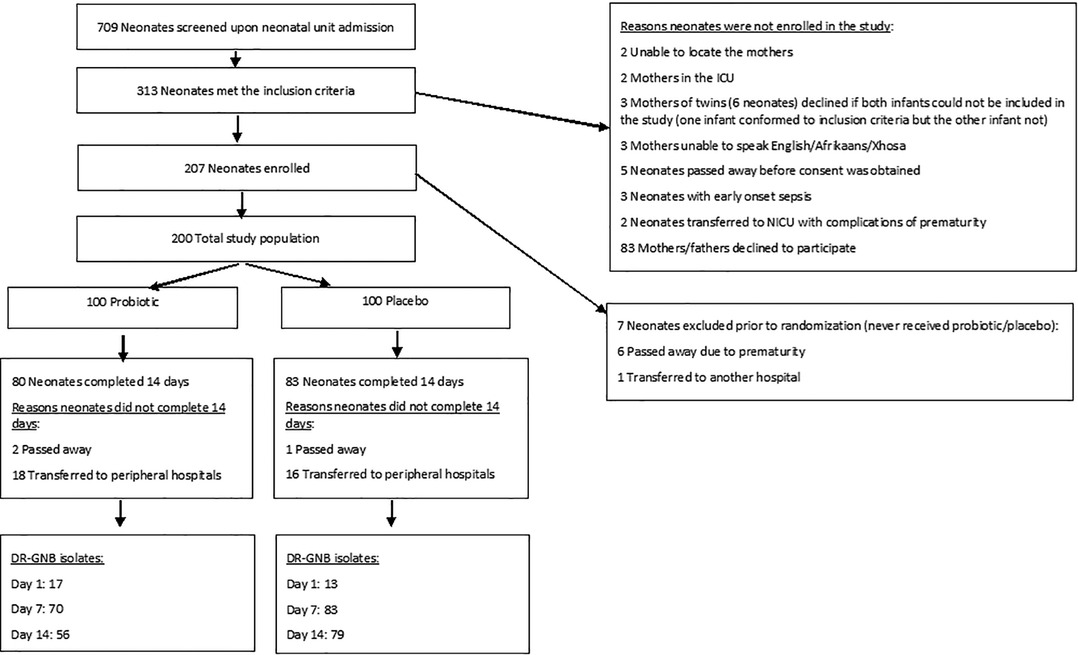
Figure 1. Flow diagram of the neonates included in the clinical trial, reasons for non-inclusion, randomization information as well as outcome. ICU, intensive care unit; NICU, neonatal intensive care unit; DR-GNB, drug-resistant Gram-negative bacilli.
Demographics of the study participants
The demographic for the study participants were not significantly different as described in Table 1. The mean gestational age at birth between the two groups was similar—probiotic group 29 weeks; ±13.9 days (range 25–36 weeks) and the placebo group 30 weeks; ±13.5 days (range 25–34 weeks). The mean birthweight was also similar between the two groups—probiotic 1,174 g; ±226 g (range 780 –1,500 g) and placebo 1,150 g; ±230 g (range 750 –1,495 g). The majority of infants were born via caesarean section (n = 146; 73%). KMC was initiated on average at day 9.2 (range 3–21 days) in the probiotic group, and 9.1 (range 2–21 days) in the placebo group. Day of first feed was also similar between the probiotic and placebo group: 3.1 days; ±1.125 days (range 0–6 days) versus 3.0 days; ±0.985 days (range 2–6 days) respectively.
Microbiological sampling and analysis
The breakdown of the DR-GNB organisms cultured over the day enrolment, day 7 and day 14 of life are described in Table 2. At enrolment (<72 h after birth), 15% of neonates were already perirectally colonized with DR-GNB. There was no difference in colonization status between the probiotic and placebo arms at enrolment (17% versus 13% respectively; p = 0.553). By day 7, 71% of the probiotic group and 83% of the placebo group were colonized with DR-GNB (p = 0.060). The difference was more pronounced by day 14 with DR-GNB colonization present in 70% of the probiotic group and 95% of the placebo group (p < 0.001).
The three most frequently cultured organisms included Klebsiella pneumoniae, Acinetobacter spp. and Serratia marcescens. The relative abundance of these is demonstrated in Figure 2. On the day of enrolment the most common DR-GNB isolated in both groups were K. pneumoniae (n = 8; 40% in the probiotic group and n = 6; 37.5% in the placebo group) and Acinetobacter spp. (n = 8; 40% in the probiotic group and n = 6; 37% in the placebo group), with lower S. marcescent numbers (n = 1; 5% in the probiotic group and n = 2; 13% in the placebo group). There was no difference in pathogen spectrum between study arms (p = 0.553).
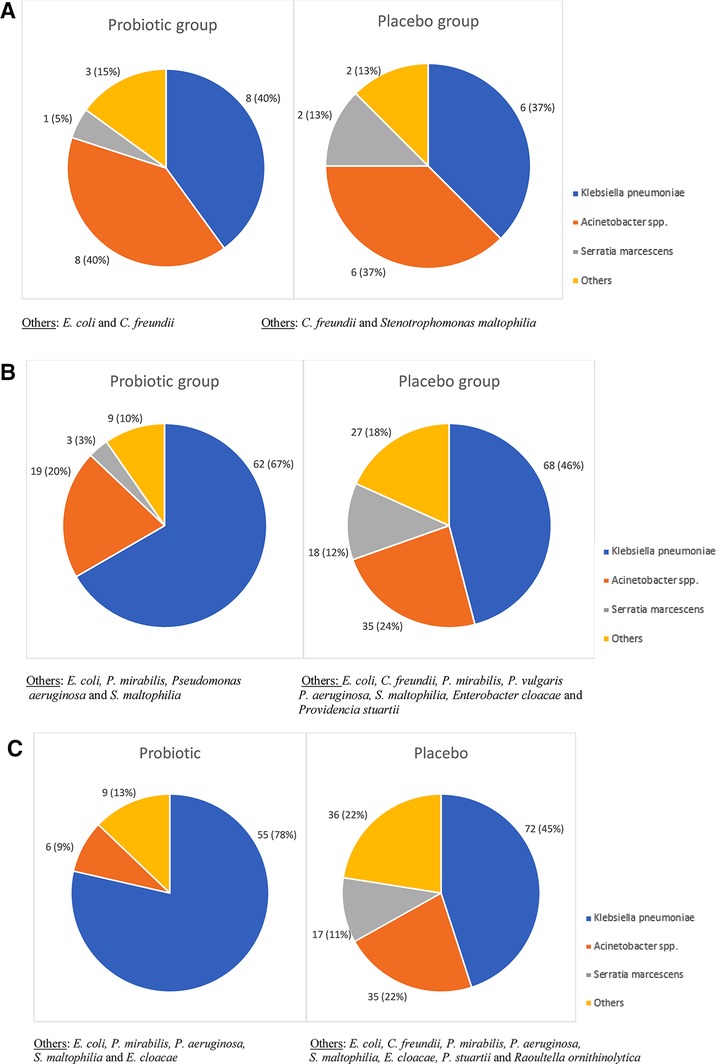
Figure 2. (A–C) Drug-resistant Gram-negative pathogens isolated from rectal swabs in preterm neonates by day of swab collection. (A) DR-GNB pathogens isolated from the day of enrolment swab. (B) DR-GNB pathogens isolated from the day 7 swab. (C) DR-GNB pathogens isolated from the day 14 swab.
At day 7 of life, K. pneumoniae (n = 62; 67% in the probiotic group and n = 68; 46% in the placebo group) and Acinetobacter spp. (n = 19; 20% in the probiotic group and n = 35; 24% in the placebo group) remained the most frequently isolated DR-GNB. There was a difference, although not statistically significant in the prevalence of peri-rectal carriage of DR-GNB between the two groups (p = 0.060). Of note, was a significant difference in the prevalence of S. marcescens carriage (n = 3; 3% in the probiotic group versus n = 18; 12% in the placebo groups; p = 0.032).
At day 14 of life, K. pneumoniae remained the dominant peri-rectal colonizing DR-GNB in the probiotic group (n = 55; 78%) and placebo group (n = 72; 45%), with Acinetobacter spp. in higher numbers in the placebo group (n = 35; 22%) than the probiotic group (n = 6; 9%). Of interest to note that there was no S. marcescens isolated cultured in the probiotic group, compared to n = 17; 11% in the placebo group. There was a significant difference in the overall DR-GNB carriage prevalence between the two groups (p < 0.001), as well as a difference in the frequency of Acinetobacter spp. and S. marcescens isolated from the two groups (p = 0.039 and p < 0.001 respectively) (Figure 2).
Effects of probiotic supplementation
Infants supplemented with probiotics showed a significant reduction in the likelihood of peri-rectal colonization with DR-GNB at day 7 and 14 of life (Table 3). When adjusting for other variables (septic risk, HIV exposure, delivery mode, birthweight, gestational age, day of start of feeds, day of start of KMC and antibiotic use), the odds for isolating one/more DR-GNB on a peri-rectal swab at day 14 in the probiotic group was 0.24 as high as the odds for peri-rectal carriage of DR-GNB in the placebo group (95% CI: 0.12–0.47; p < 0.001).
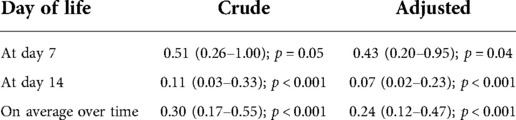
Table 3. Crude and adjusted odds ratios for the effect of probiotic supplementation on the presence of peri-rectal colonization with DR-GNB over time.
The positive effects of probiotic supplementation appeared to be age dependent with more pronounced effects seen in preterm infants born < 32 weeks gestation (Table 4).
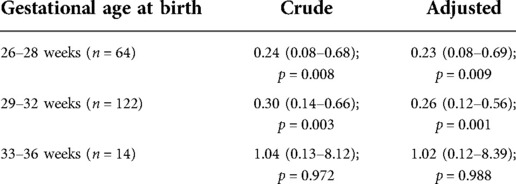
Table 4. Crude and adjusted odds ratios for the effect of probiotic supplementation on the presence of peri-rectal colonization with DR-GNB on average over time stratified by the infant's gestational age at birth.
Discussion
Antimicrobial resistance in Gram negative bacilli is of concern worldwide. Giuffre et al. conducted a 5-year prospective cohort surveillance study in Italy. They described an upward trend in the prevalence of intestinal colonization by DR-GNB (23). ESBL and carbapenem-resistant Enterobacteriaceae (CRE) both present a huge clinical problem worldwide as it causes a high morbidity and mortality rates (35). Literature describes that colonization with MDR-GNB is a risk factor for the development of BSI—around 50% of infants colonized with MDR-GNB, (especial ESBL-producing bacteria) can develop BSI (36, 37).
This double-blind RCT investigated the effect of a multi-strain probiotic on the prevalence of peri-rectal colonization with DR-GNB in preterm neonates receiving a multi-strain probiotic supplementation versus placebo. On the day of enrolment, fifteen percent of the neonates showed peri-rectal colonization with either ESBL-producing or carbapenem resistant GNB, indicating probable maternal-to-neonate (vertical) bacterial transmission or environmental acquisition at time of delivery, with no difference between groups. On day 7 (corresponding to early gut colonization), and day 14 (corresponding to late gut colonization) neonates in the probiotic group were less likely to have peri-rectal DR-GNB colonization compared to the placebo group.
The probiotic and placebo group started off with K. pneumoniae and Acinetobacter spp. as the two main DR-GNB organisms cultured. The pattern in DR-GNB organisms in the probiotic group changed over time showing K. pneumoniae dominance over Acinetobacter spp. In contrast the DR-GNB in the placebo group retained a higher proportion of Acinetobacter spp. and S. marcescens over time. A 10-year retrospective analysis of neonatal HA-BSI data from TBH showed that the following bacteria (Klebsiella species, Staphylococcus aureus, S. marcescens, Enterococcus species and Acinetobacter baumannii) were the pathogens most frequently cultured (38).
Previous studies found encouraging results for probiotic use to reduce carriage of pathogens in the neonatal gastrointestinal tract. One postulated mechanism of effect is that probiotic bacteria produce bacteriocins that enhance mucosal integrity and reduce pathogenic bacterial proliferation and resistance (39). Probiotic bifidobacterial strains can reduce AMR gene carriage by 90% compared to controls, e.g., Casaburi et al. showed that Bifidobacterium infantis can change the neonate gut microbiome and lower the abundance of common gut phyla such as Proteobacteria and Firmicutes (which include the genera Escherichia and Clostridium) (40). Nguyen et al. also found that the supplementation of Bifidobacterium infantis led to a decrease of Klebsiella, Staphylococcaci and Enterobacteriaceae in preterm infants. Further there was a high abundance of Bifidoacteria and most importantly a decreased in enteric inflammation (41). Roy et al. supplemented preterm neonates (<37 weeks, <2,500 g at birth) with Bifidobacterium infantis, Lactobacillus, and Bifidobacterium lactis. They showed that the supplemented group had a reduction in enteral fungal colonization, a reduction in fungal sepsis, earlier establishment of full enteral feeds and a reduced duration in hospital stay (30). Esaissaen et al. supplemented extremely preterm infants with Bifidobacterium longum and Lactobacillus acidophilus. They indicated that these infants had a high relative abundance of Bifidobacterium at 1 week of age, after receiving the supplement for only a few days, despite the high usage of antibiotics. Thus, the probiotic supplement induced colonization resistance and alleviated the harmful effects of antibiotic use on the gut microbiome (42). Van Best et al. studied the effect of probiotics on the developing microbiome. They concluded that probiotic supplementation reduced the abundance of organisms that are known to induce proinflammatory responses (43). Duar et al. used the ecosystem services framework to show that Bifidobacterium longum subsp. infantis, a gut symbiont, can effectively use human milk oligosaccharides (HMO) and change it into organic acids (mainly lactate and acetate). These organic acids alter the intestinal environment and prohibit the growth of pH-sensitive populations, known as enteric pathogens, of which many carry antibiotic resistant genes. Only B. infantis have the complete pathway necessary for intracellular HMO-transport and degradation (31).
A limitation of the study may be that culture-based methods using selective media were used to determine carriage status. While culture-based detection is likely less sensitive than molecular assays, the ChromID ESBL selective agar used in this study has been shown to have sensitivity of 88% (44), and the CarbaSmart a sensitivity of 90% (45). It is likely that a small proportion of children carrying MDR-GNB may have been missed using this approach, as opposed to a molecular screen. On the other hand, use of culture allows for identification of the resistant pathogens, as opposed to only detecting resistance genes in the sample. While culture will miss fastidious organisms, the medium we used supports the growth of common facultative Gram-negative pathogens very well. We did not characterize the resistance genes present in the cultured isolates which would have provided additional epidemiological information; this was a consequence of resource constraints. A maternal peri-rectal swab would have provided additional information regarding the maternal to neonate bacterial transfer, and future studies including both maternal and environmental swabs would be valuable in describing the relative contributions of maternal microflora and environmental flora to the carriage of resistance in neonates.
Conclusion
Hospitalized neonates showed substantial peri-rectal colonization with DR-GNB at enrolment and further rapid acquisition of DR-GNB in the first 2 weeks of life. The use of a multi-strain probiotic was effective in reducing early and late neonatal gut colonization with DR-GNB.
Data availability statement
The raw data supporting the conclusions of this article will be made available by the authors, without undue reservation.
Ethics statement
The studies involving human participants were reviewed and approved by Human Research Ethics Committee of the Faculty of Health Sciences of Stellenbosch University as well as Tygerberg Academic Hospital (S20/07/178). Written informed consent to participate in this study was provided by the participants' legal guardian/next of kin.
Author contributions
MS conceptualized and designed the study, coordinated the study, conducted the data collection and analysis, drafted the first version of the manuscript, and revised the manuscript. EN conceptualized and designed the study, reviewed, and revised the manuscript, and approved the final manuscript as submitted. AB conceptualized and designed the study, reviewed, and revised the manuscript, and approved the final manuscript as submitted. AD conceptualized and designed the study, reviewed, and revised the manuscript, and approved the final manuscript as submitted. AW assisted with the laboratory training, reviewed, and revised the manuscript, and approved the final manuscript as submitted. JT assisted with the statistical analysis, reviewed, and revised the manuscript, and approved the final manuscript as submitted. EN conceptualized and designed the study, reviewed, and revised the manuscript, and approved the final manuscript as submitted. MW conceptualized and designed the study, reviewed, and revised the manuscript, and approved the final manuscript as submitted. All authors contributed to the article and approved the submitted version.
Funding
Funding for this study was received from the South African Medical Research Council (SAMRC) self-initiated research grant to AD for laboratory costs, Harry Crossley Foundation and VU University of Amsterdam. AD is supported by a National Institutes of Health Fellowship (K43TW010682).
Acknowledgments
The author would like to thank Kedisaletse Moloto who assisted with the laboratory training.
Conflict of interest
The authors declare that the research was conducted in the absence of any commercial or financial relationships that could be construed as a potential conflict of interest.
Publisher's note
All claims expressed in this article are solely those of the authors and do not necessarily represent those of their affiliated organizations, or those of the publisher, the editors and the reviewers. Any product that may be evaluated in this article, or claim that may be made by its manufacturer, is not guaranteed or endorsed by the publisher.
References
1. Laxminarayan R, Duse A, Wattal C, Zaidi AKM, Wertheim HFL, Sumpradit N, et al. Antibiotic resistance-the need for global solutions. Lancet Infect Dis. (2013) 13(12):70318–9. doi: 10.1016/S1473-3099(13)70318-9
2. Exner M, Bhattacharya S, Christiansen B, Gebel J, Goroncy-Bermes P, Hartemann P, et al. Antibiotic resistance: what is so special about multidrug-resistant Gram-negative bacteria? GMS Hyg Infect Control. (2017) 12:1–24. doi: 10.3205/dgkh000290
3. Sehgal R, Gaind R, Chellani H, Agarwal P. Extended-spectrum beta lactamase-producing Gram-negative bacteria: clinical profile and outcome in a neonatal intensive care unit. Ann Trop Paediatr. (2007) 27(1):45–54. doi: 10.1179/146532807X170501
4. Didier C, Streicher MP, Chognot D, Campagni R, Schnebelen A, Messer J, et al. Late-onset neonatal infections: incidences and pathogens in the era of antenatal antibiotics. Eur J Pediatr. (2021) 171(4):681–7. doi: 10.1007/s00431-011-1639-7
5. Harder T, Seidel J, Eckmanns T, Weiss B, Haller S. Predicting late-onset sepsis by routine neonatal screening for colonisation by Gram-negative bacteria in neonates at intensive care units: a protocol for a systematic review. BMJ Open. (2017) 7(3):1–7. doi: 10.1136/bmjopen-2016-014986
6. Abel-Hady H, Hawas S, El-Daker M, El-Kady R. Extended-spectrum B-lactamase producing Klebsiella pneumoniae in neonatal intensive care unit. J Perinatol. (2008) 28(10):685–90. doi: 10.1038/jp.2008.73
7. Bizzarro MJ, Gallagher PG. Antibiotic-resistant organisms in the neonatal intensive care unit. Semin Perinatol. (2007) 31(1):26–32. doi: 10.1053/j.semperi.2007.01.004
8. Liu J, Fang Z, Yu Y, Ding Y, Liu Z, Zhang C, et al. Pathogens distribution and antimicrobial resistance in bloodstream infections in twenty-fve neonatal intensive care unitsin China, 2017–2019. Antimicrob Resist Infect Control. (2021) 10(121):1–11. doi: 10.1186/s13756-021-00989-6
9. Thomas R, Ondongo-Ezhet C, Motsoaledi N, Sharland M, Clements M, Velahi S. Incidence and all-cause mortality rates in neonates infected with carbapenem resistant organism. Front Trop Dis. (2022) 3:1–9. doi: 10.3389/fitd.2022.832011
10. Anthony M, Beford-Russell A, Cooper T, Fry C, Heath PT, Kennea H, et al. Managing and preventing outbreaks of Gram-negative infections in UK neonatal units. Arch Dis Child Fetal Neonatal Ed. (2013) 98(6):F549–53. doi: 10.1136/archdischild-2012-303540
11. Dramowski A, Aucamp M, Bekker A, Pillay A, Moloto K, Whitelaw AC, et al. NeoCLEAN: a multimodal strategy to enhance environmental cleaning in a resource-limited neonatal unit. Antimicrob Resist Infect Control. (2021) 10(35):1–9. doi: 10.1186/s13756-021-00905-y
12. Camacho-Gonzalez A, Spearman PW, Stoll BJ. Neonatal infectious diseases: evaluation of neonatal sepsis. Pediatr Clin North Am. (2013) 60(2):367–89. doi: 10.1016/j.pcl.2012.12.003
13. Parker LA, Magalhaes M, Desorcy-Scherer K, Lamberti MT, Lorca GL, Neu J. Neonatal feeding tube colonization and the potential effect on infant health: a review. Front Nutr. (2022) 24(9):1–19. doi: 10.3389/fnut.2022.775014
14. Gewolb IH, Schwalbe RS, Taciak VL, Harrison TS, Panigrahi P. Stool microflora in extremely low birthweight infants. Arch Dis Child Fetal Neonatal Ed. (1999) 80(3):F167–73. doi: 10.1136/fn.80.3.F167
15. Sakata H, Yoshioka H, Fujita K. Development of the intestinal flora in very low birth weight infants compared to normal full-term newborns. Eur J Pediatr. (1985) 144:186–90. doi: 10.1007/BF00451911
16. Korpela K, Blakstad EW, Moltu SJ, Strommen K, Nakstad B, Ronnestad AE, et al. Intestinal microbiota development and gestational age in preterm neonates. Sci Rep. (2018) 6(8):1–9. doi: 10.1038/s41598-018-20827-x
17. Jacquot A, Neveu D, Aujoulat F, Mercier G, Marchandin H, Jumas-Bilak E. Dynamics and clinical evolution of bacterial gut microflora in extremely premature patients. J Pediatr. (2011) 158(3):390–6. doi: 10.1016/j.jpeds.2010.09.007
18. Stewart CJ, Embleton ND, Marrs ECL, Smith DP, Nelson A, Abdulkadir B, et al. Temporal bacterial and metabolic development of the preterm gut reveals specific signatures in health and disease. Microbiome. (2016) 4(67):1–10. doi: 10.1186/s40168-016-0216-8
19. Lautenbach E, Harris AD, Perencevich EN, Nachamkin I, Tolomeo P, Metlay JP. Test characteristics of perirectal and recal swab compared to stool sample for detection of fluoroquinalone-resistant eschericia coli in the gastrointestinal tract. Antimicrob Agents Chemother. (2005) 49(2):798–800. doi: 10.1128/AAC.49.2.798-800.2005
20. Stephens B, Azimi P, Thoemmes MS, Heidarinejad M, Allen GJ. Microbial exchagne via fomites and implications for human health. Curr Pollut Rep. (2019) 5(4):198–213. doi: 10.1007/s40726-019-00123-6
21. Turner P, Pol S, Soeng S, Sar P, Neou L, Chea P, et al. High pevalence of antimicrobial-resistant Gram-negative colonization in hospitalized cambodian infants. Pediatr Infect Dis J. (2016) 35(8):856–61. doi: 10.1097/INF.0000000000001187
22. Donskey CJ. The role of the intestinal tract as a reservoir and source for transmission of nosocomial pathogens. Clin Infect Dis. (2004) 39(2):219–26. doi: 10.1086/422002
23. Giuffre M, Geraci DM, Bonura C, Saporito L, Graziano G, Insinga V, et al. The inceasing challenge of multidrug-resistant Gram-negative bacilli. Medicine. (2016) 95(10):1–10. doi: 10.1097/MD.0000000000003016
24. Bulabula A, Dramowski A, Mehtar S. Maternal colonization or infection with extended-spectrum-beta-lactamase-producing Enterobacteriaceae in Africa: a systematic review and meta-analysis. Int J Infect Dis. (2017) 64:58–66. doi: 10.1016/j.ijid.2017.08.015
25. Yin L, He L, Miao J, Yang W, Wang X, Ma J, et al. Carbapenem-resistant enterobacterales colonization and subsequent infection in a neonatal intensive care unit in Shanghai, China. Infect Prev Pract. (2021) 3(3):1–9. doi: 10.1016/j.infpip.2021.100147
26. WHO and FAO. Food and Agriculture Organization and World Health Organization Expert Consultation. Evaluation of health and nutritional properties of powder milk and live lactic acid bacteria. Córdoba, Argentina; 2001.
27. Han S, Lu Y, Xie J, Fei Y, Zheng G, Wang Z, et al. Probiotic gastrointestinal transit and colonizatin after oral administration: a long journey. Front Cell Infect Microbiol. (2021) 11:609722. doi: 10.3389/fcimb.2021.609722
28. Azad AK, Sarker M, Li T, Yin J. Probiotic Species in the modulation of gut microbiota: an overview. BioMed Res Int. (2018) 2018:9478630. doi: 10.1155/2018/9478630
29. Reid G. Probiotics: definition, scope and mechanisms of action. Best Pract Res Clin Gastroenterol. (2016) 30(1):17–25. doi: 10.1016/j.bpg.2015.12.001
30. Roy A, Chaudhuri J, Sarkar D, Ghosh P, Charaborty S. Role of enteric supplementation of probiotics on late-onset sepsis by candida species in preterm low birth weight neonates: a randomized, double blind, placebo-controlled trial. N Am J Med Sci. (2014) 6(1):50–7. doi: 10.4103/1947-2714.125870
31. Duar RM, Henrick BM, Casaburi G, Frese SA. Integrating the ecosystem services framework to define dysbiosis of the breastfed infant gut: the role of B. infantis and human milk oligosaccharides. Front Nutr. (2020) 7:33. doi: 10.3389/fnut.2020.00033
32. Dermyshi E, Wang Y, Yan C, Hong W, Qiu G, Gong X, et al. The “golden age” of probiotics: a systematic review and meta-analysis of randomized and observational studies in preterm infants. Neonatology. (2017) 112(1):9–23. doi: 10.1159/000454668
33. Robertson C, Savva GM, Clapuci R, Jones J, Maimouni H, Brown E, et al. Incidence of necrotising enterocolitis before and after introducing routine prophylactic lactobacilus and bifidobacterium probiotics. Arch Dis Child Fetal Neonatal Ed. (2020) 105(4):F380–6. doi: 10.1136/archdischild-2019-317346
34. Hua XT, Tang J, Mu DZ. [Effect of oral administration of probiotics on intestinal colonization with drug-resistant bacteria in preterm infants]. Zhongguo Dang Dai Er Ke Za Zhi (Chinese). (2014) 16(6):606–9. PMID: 24927436
35. Bar-Yoseph H, Hussein K, Braun E, Mical P. Natural history and decolonization strategies for ESBL/carbapenem-resistant Enterobacteriaceae carriage: systematic review and meta-analysis. J Antimicrob Chemother. (2016) 71(10):2729–39. doi: 10.1093/jac/dkw221
36. Singh N, Patel KM, Leger MM, Short B, Sprague BM, Kalu N, et al. Risk of resistant infections with enterobacteriaceae in hospitalized neonates. Pediatr Infect Dis J. (2002) 21(11):1029–33. doi: 10.1097/00006454-200211000-00010
37. Lukac PJ, Bonomo RA, Logan LK. Extended-spectrum B-lactamase-producing enterobacteriaceae in children: old foe, emerging threat. Clin Infect Dis J. (2015) 60(9):1389–97. doi: 10.1093/cid/civ020
38. Reddy K, Bekker A, Whitelaw AC, Esterhuizen TM, Dramowski A. A retrospective analysis of pathogen profile, antimicrobial resistance and mortality in neonatal hospital-acquired bloodstream infections from 2009 to 2018 at tygerberg hospital, South Africa. Plos One. (2021) 16(1):1–14. doi: 10.1371/journal.pone.0245089
39. Pamer EG. Resurrecting the intestinal microbiota to combat antibiotic-resistant pathogens. Science. (2016) 352(6285):535–8. doi: 10.1126/science.aad9382
40. Casaburi G, Duar RM, Vance DP, Mitchell R, Contreras L, Frese SA, et al. Early-life gut microbiome modulation reduces the abundance of antibiotic-resistant bacteria. Antimicrob Resist Infect Control. (2019) 8(131):1–18. doi: 10.1186/s13756-019-0583-6
41. Nguyen M, Holdbrooks H, Mishra P, Abrantes MA, Eskew S, Garma M, et al. Impact of probiotic B. infantis EVC001 feeding in premature infants on the gut microbiome, nosocomially acquired antibiotic resistance, and enteric inflammation. Front Pediatr. (2021) 9:1–19. doi: 10.3389/fped.2021.618009
42. Esaissaen E, Hjerde E, Cavanagh JP, Pedersen T, Andresen JH, Rettedal SI, et al. Effects of probiotic supplementation on the gut microbiota and antibiotic resistome development in preterm infants. Front Pediatr. (2018) 6:1–16. doi: 10.3389/fped.2018.00001
43. van Best N, Trepels-Kottek S, Savelkoul P, Orlikowsky T, Hornef MW, Penders J. Influence of probiotic supplementation on the developing microbiota of human preterm neonates. Gut Microbes. (2020) 12(1):1–16. doi: 10.1080/19490976.2020.1826747
44. Réglier-Poupet H, Naas T, Carrer A, Cady A, Adam JM, Fortineau N, et al. Performance of chromID ESBL, a chromogenic medium for detection of enterobacteriaceae producing extended-spectrum beta-lactamases. J Med Microbiol. (2008) 57(Pt 3):310–5. doi: 10.1099/jmm.0.47625-0
Keywords: multidrug-resistant Gram negative bacilli, neonate, probiotic, premature (babies), rectal swab
Citation: Sowden M, van Niekerk E, Bulabula ANH, Dramowski A, Whitelaw A, Twisk J and van Weissenbruch MM (2022) Impact of a multi-strain probiotic administration on peri-rectal colonization with drug-resistant Gram-negative bacteria in preterm neonates. Front. Pediatr. 10:1002762. doi: 10.3389/fped.2022.1002762
Received: 25 July 2022; Accepted: 26 September 2022;
Published: 2 November 2022.
Edited by:
Robert Lewis Schelonka, Oregon Health and Science University, United StatesReviewed by:
Brian Scottoline, Oregon Health and Science University, United StatesDonna Rudd, James Cook University, Australia
© 2022 Sowden, van Niekerk, Bulabula, Dramowski, Whitelaw, Twisk and Van Weissenbruch. This is an open-access article distributed under the terms of the Creative Commons Attribution License (CC BY). The use, distribution or reproduction in other forums is permitted, provided the original author(s) and the copyright owner(s) are credited and that the original publication in this journal is cited, in accordance with accepted academic practice. No use, distribution or reproduction is permitted which does not comply with these terms.
*Correspondence: Marwyn Sowden bWFyd3luc0BzdW4uYWMuemE=
Specialty Section: This article was submitted to Neonatology, a section of the journal Frontiers in Pediatrics