- 1Unità Operativa Complessa di Neonatologia, Fondazione Policlinico Universitario A. Gemelli IRCCS, Rome, Italy
- 2Section of Hygiene, Institute of Public Health, Università Cattolica del Sacro Cuore, Rome, Italy
- 3Department of Woman and Child Health and Public Health, Università Cattolica del Sacro Cuore, Rome, Italy
Objective: To evaluate the effect of volume guarantee (VG) combined with high-frequency oscillatory ventilation (HFOV) on respiratory and other physiological parameters immediately after lung recruitment and surfactant administration in HFOV elective ventilated extremely low gestational age newborns (ELGAN) with respiratory distress syndrome (RDS).
Design: Observational study.
Setting: Tertiary neonatal intensive care unit.
Patients: Twenty-two ELGANs of 25.5 ± 1.1 weeks of gestational age requiring invasive mechanical ventilation and surfactant administration for RDS during the first 6 h of life.
Interventions: All infants intubated in delivery room, were managed with elective HFOV and received surfactant after a lung recruitment manoeuver. Eleven infants received HFOV + VG and were compared with a control group of 11 infants receiving HFOV alone. HFOV was delivered in both groups by Dräger Babylog VN500 ventilator (Dräger, Lubeck, Germany).
Main Outcome Measures: Variations and fluctuations of delivered high-frequency tidal volume (VThf), fluctuation of pressure amplitude (ΔP) and partial pressure of CO2 (pCO2) levels after recruitment manoeuver and immediately after surfactant administration, in HFOV + VG vs. HFOV ventilated infants.
Results: There were no significant differences in the two groups at starting ventilation with or without VG. The mean applied VThf per kg was 1.7 ± 0.3 ml/kg in the HFOV group and 1.7 ± 0.1 ml/kg in the HFOV + VG group. Thirty minutes after surfactant administration, HFOV group had a significant higher VThf/Kg than HFOV + VG (2.1 ± 0.3 vs. 1.6 ± 0.1 ml/kg, p < 0.0001) with significantly lower pCO2 levels (43.1 ± 3.8 vs. 46.8 ± 1.5 mmHg, p = 0.01), 54.4% of patients having pCO2 below 45 mmHg. Measured post-surfactant ΔP values were higher in HFOV group (17 ± 3 cmH2O) than in HFOV + VG group (13 ± 3 cmH2O, p = 0.01).
Conclusion: HFOV + VG maintains pCO2 levels within target range and reduces VThf delivered variations more consistently than HFOV alone after surfactant administration.
Introduction
Despite a shift toward non-invasive respiratory support, mechanical ventilation and surfactant administration in the first hours of life may be life-saving in preterm infants with respiratory distress syndrome (RDS), especially in extremely low gestational age newborns (ELGAN) (gestational age ≤ 27 weeks) (1, 2). In the last years, high-frequency oscillatory ventilation (HFOV) has been increasingly used in preterm infants with RDS, because early HFOV could reduce risk of bronchopulmonary dysplasia (BPD) (3), especially if associated with an open lung strategy (4, 5).
HFOV offers highly effective oxygenation and clearance of waste gas, despite use of tidal volumes at or below dead space volume (6, 7). The CO2 diffusion (gas transport) coefficient (DCO2) is a vital variable in HFOV and is calculated as frequency times the square of tidal volume during HFOV (VThf) (8, 9). Recently, weight-corrected DCO2 ([ml/kg]2/s) has been proposed to reduce inter-individual variability (10). Consequently, VThf is crucial for CO2 elimination with a larger impact in comparison to tidal volume during conventional mechanical ventilation. The VThf is determined by the amplitude of the pressure oscillations (ΔP), and it is delivered to the lungs around a constant mean airway pressure (MAP). As the same ΔP can be associated with very different level of chest oscillations and VThf in different babies, and even in the same baby over the course of pulmonary disease, the same ΔP can result in very large variations of VThf and unexpected variations of CO2 removal (11, 12).
Volume guarantee (VG), a form of volume-targeted ventilation, is known to improve neonatal prognosis and has been a well-established respiratory management for preterm infants when combined with conventional ventilation (13–16). In the last few years, volume-guarantee modality has been combined with HFOV (HFOV+VG), demonstrating an attenuation of the fluctuations in SpO2 and CO2, which can prevent hypoxemia and hypocapnia (17). Iscan et al. compared HFOV and HFOV + VG in the same preterm infants (24–32 weeks' gestation) with RDS, intubated within the first 6 h of life and treated with surfactant. The HFOV + VG period reported a reduction in fluctuations of VThf, in the number of out-of-target pCO2 levels and hypoxia events compared with HFOV group (18). This is confirmed in detail by Belteki et al. (19) recording every VThf from the ventilator and showing that the tidal volume of the oscillations varies in the short term but is kept very close to the long term objective. However, according to the present literature, a specific optimal VThf has not yet been recommended, as it varies with the instantaneous characteristics of individuals and frequencies (20, 21).
In our previous study aimed to evaluate the changes in end-expiratory lung volume during an oxygenation-guided stepwise recruitment procedure in HFOV without VG, mean VThf significantly increased between the start and the end of the recruitment with a slight not significant reduction of mean pCO2 values, possible effect of the increased lung compliance (22).
To our knowledge, changes in VThf and the effects of HFOV + VG during surfactant administration in preterm infants with RDS have not been thoroughly studied.
HFOV open lung recruitment maneuver and surfactant administration are followed by improved alveolar ventilation potentially resulting in volutrauma and hypocapnia (23–25). Volutrauma can contribute to BPD, and hypocapnia can alter cerebral blood flow increasing the risk of intra-ventricular hemorrhage (IVH), periventricular leukomalacia (PVL), and poor neurodevelopmental outcomes (24–30). In newborn animal model of surfactant deficiency, the use of HFOV combined with VG ventilation demonstrated benefits of setting the VThf instead of ΔP to modify CO2 removal from the lung (31). Volume guarantee combined with HFOV may be clinically advantageous, especially in conditions in which lung compliance can change rapidly, such as during and immediately after surfactant treatment.
The aim of the present study was to investigate the effect of VG combined to HFOV on respiratory and other physiological parameters in HFOV electively ventilated ELGAN with RDS immediately after surfactant administration, when lung compliance can change rapidly.
Materials and Methods
Patients
This is a single center, observational study conducted in our third level neonatal intensive care unit (NICU). This study was approved by the Ethics Committee of Fondazione Policlinico Universitario A. Gemelli IRCCS, Roma—Università Cattolica del Sacro Cuore (ID 4425). The inclusion criteria were as follows: inborn premature infants with a gestational age (GA) between 24 and 27 weeks (ELGAN), requiring endotracheal intubation at birth with a diagnosis of RDS, electively HFOV ventilated and receiving surfactant treatment in the first 6 h of life, and after a recruitment maneuver as open lung strategy using oxygenation as indirect marker for lung volume (5). In our NICU, we started using HFOV + VG during clinical care in June 2017, because of technical improvement led by Dräger specialists and the increased experience and skills of the entire neonatal staff with the Dräger Babylog VN500 ventilator (Dräger Medical, Lubeck, Germany), using the HFOV + VG modality.
In fact, before June 2017, all ELGANs that needed mechanical ventilation were managed by HFOV without VG. A historical control group of ELGANs, born between June 2016 and June 2017 and electively ventilated in HFOV, was then selected and compared with a group of ELGANs prospectively studied in the period June 2017–June 2018, electively ventilated in HFOV + VG.
Exclusion criteria were as follows: outborn patients, presence of major congenital malformations, hydrops fetalis inherited disorders of metabolism, congenital pneumonia (positive bronchoalveolar lavage fluid culture at birth), pulmonary hypertension (confirmed by echocardiography), suspected pulmonary hypoplasia (based on clinician interpretation of a chest radiograph with small and hypoplastic-appearing lungs; premature rupture of membranes and/or oligohydramnios documented on antenatal ultrasound 3 or more weeks prior to delivery), severe circulatory shock (prolonged capillary filling, reduced strength of peripheral pulses, cool skin, lethargy, hypotension, oliguria, increasing lactate concentrations, and metabolic acidosis), and >20% endotracheal tube leak to negate the effect of leakage (Figure 1).
HFOV and Open Lung Ventilation Strategy
HFOV is the primary mode of mechanical ventilation in our NICU to manage preterm newborns with GA ≤ 27 weeks and/or BW < 1,000 g affected by RDS because of the results of our previous randomized controlled trial comparing the effects of HFOV vs. conventional mechanical ventilation (32).
In all studied infants, HFOV was delivered by Dräger Babylog VN500 (Dräger Medical, Lubeck, Germany) and started at a MAP of 8–10 cmH20, a frequency of 15 Hz, and an inspiratory/espiratory ratio of 1:2 (I:E = 1:2). The FiO2 was initially set to ensure adequate oxygen saturation (SpO2 89–94%).
In all infants, frequency (15 Hz) and I:E = 1:2 remained unchanged during the studied ventilation periods.
The goals of respiratory management were to maintain pH 7.30–7.45, pCO2 45–55 mmHg (5.9–7.2 kPa), pO2 50–70 mm Hg (6.6–9.3 kPa), and SpO2 89–94%.
In all studied infants, HFOV or HFOV + VG was combined with an open lung ventilation strategy aiming to recruit and stabilize most collapsed alveoli/sacculi, using oxygenation as an indirect parameter for lung volume (33, 34).
The MAP was increased stepwise by 1–2 cmH2O every 2–3 min as long as SpO2 improves. The FiO2 was reduced stepwise, keeping SpO2 within the target range. The recruitment procedure was stopped if the FiO2 did not exceed 0.25 or if oxygenation no longer improved or there were signs of lung hyperinflation (capillary refill time >3 s and/or hypotension). The corresponding MAP was the pre-surfactant opening continuous distending pressure (CDPO). Next, the MAP was reduced stepwise by 1–2 cmH2O every 2–3 min until the SpO2 was deteriorated (of at least 2–3 points), and the corresponding MAP was the pre-surfactant closing pressure (CDPC). Finally, the lung was recruited again by returning to the known CDPO for 2–3 min and then stabilized setting MAP to 2 cmH2O above the CDPC at pre-surfactant optimal pressure (CDPOPT) for at least 3 min.
Surfactant was then administered at the CDPOPT (Curosurf, Chiesi) via a closed system catheter at a dose of 200 mg/kg.
Surfactant treatment in open lung HFOV ventilated preterm infants with RDS causes rapid increase (minutes) and subsequent stabilization of lung volume and increases maximal compliance of the lung, but at lower airway pressures (23).
Consequently, to avoid possible overdistension and to profit from the increased compliance after surfactant treatment, after a stabilization period of 5–10 min, MAP was reduced by 1–2 cmH2O and CDPC, CDPO, and CDPOPT were once more determined post-surfactant.
HFOV and HFOV + VG Ventilation Strategy
In the HFOV group, ΔP was initially set at 15 cm H2O and then increased if necessary until the infant's chest was seen to be visibly vibrating. Operators adjusted ΔP up or down in increments of 1–2 cm H2O as necessary, if pCO2 values were outside the target range, to achieve a VThf of 1.5–2.0 ml/kg.
In the HFOV + VG group, the target range of VThf was 1.5–1.8 ml/kg and ΔPmax limit was set 15–20% above the average ΔP needed to achieve it, frequency was set at 15 Hz, and inspiratory/espiratory ratio was 1:2. The set VThf/Kg was adjusted by the clinicians up or down in increments of 0.2 ml/kg as frequently as necessary, if pCO2 values were outside of the target range.
All patients received a loading dose of caffeine (20 mg/kg) immediately after admission to the NICU, then maintenance therapy (35), and Remifentanil by continuous intravenous infusion at a dose of 0.075 μg/kg/min to provide analgesia during HFOV while preserving spontaneous respiratory activity (36).
Data Collection
Data of the historical HFOV group were collected from ventilation sheets where doctors of our Unit usually report vital signs and respiratory and ventilation parameters during surfactant administration in ventilated patients.
Demographic data on patient and maternal characteristics were collected from each patient.
Information was collected on MAP, FiO2, ΔP, VThf per weight, frequency, DCO2 per weight, SpO2, and pCO2 at different time points: start of ventilation in the NICU, at the pre-surfactant time (after the stabilization period at CDPOPT pre-surfactant time), and at 30 min post-surfactant (after the stabilization period at CDPOPT post-surfactant time). To assess the severity of lung disease at the start of ventilation, the oxygenation index (OI): (MAPxFiO2/PaO2x100) was calculated for each patient.
In all patients, a capillary blood sample after adequate heel warming was obtained for gas analysis at each of the three study phases (baseline, pre-surfactant, and post-surfactant).
Statistical Analysis
Values were expressed as mean and SD or median and range for continuous variables or absolute frequency and percentages for categorical variables. Continuous variables were compared with parametric (Student's t-test) or non-parametric (Mann–Whitney U-test) tests, as appropriate. Categorical variables were compared by using a two-tailed Fisher's exact test. A 2-tailed value of p < 0.05 was considered significant. Pearson's correlation analysis was performed to determine the correlations between selected parameters.
Data were analyzed using commercial statistical software (GraphPad Prism version 8.0.0, San Diego, California, USA).
Results
Between 1 June 2016 and 30 June 2018, a total of 68 inborn ELGAN with a diagnosis of RDS requiring on-going intensive care were admitted to our NICU. Thirty-five of these ELGAN required endotracheal intubation at birth and were electively ventilated by HFOV.
Thirteen of these infants were excluded for the following reasons: congenital pneumonia (n = 2), severe circulatory shock (n = 3, two septic shocks, one recipient baby of twin-to-twin transfusion syndrome), hydrops fetalis in congenital syphilis (n = 1), severe pulmonary hypertension in lung hypoplasia secondary to prolonged premature rupture of membranes (n = 4), >30% endotracheal tube leak (n = 2), and esophageal atresia (n = 1).
Of the remaining 22 patients with 25.5 ± 1.1 weeks of GA and 721 ± 115 g of birth weight, 11 were born between 1 June 2016 and 15 June 2017 and electively ventilated in HFOV (HFOV group), and 11 were born between 15 June 2017 and 30 June 2018 and electively ventilated in HFOV combined with VG (HFOV + VG group). No significant differences were observed between the two groups in terms of demographic and clinical characteristics (Table 1), including the severity of the lung disease, as demonstrated by oxygenation index (OI) (MAPxFiO2/PaO2x100 values at baseline; Table 2). All patients were intubated with 2.5 mm endotracheal tube. In all infants, frequency (15 Hz) and inspiratory/espiratory ratio of 1:2 remained unchanged during the studied ventilation periods.
The study population ventilator parameters at baseline are shown in Table 2. There were not significant differences in the 2 groups at baseline between ventilation modes in terms of MAP, ΔP, VThf per kg, DCO2, pCO2, frequency, and I:E. The mean applied VThf/Kg was 1.7 ± 0.3 ml/kg in the HFOV group and 1.7 ± 0.1 in the HFOV + VG group.
Considering data after the recruitment maneuver (i.e., pre-surfactant administration), HFOV group had a significantly higher VThf/Kg than HFOV + VG (1.8 ± 0.3 vs. 1.6 ± 0.1, p = 0.03) with higher VThf/Kg variability (in terms of SD), not corresponding to a significant difference in pCO2 values (Figure 2 and Table 2).
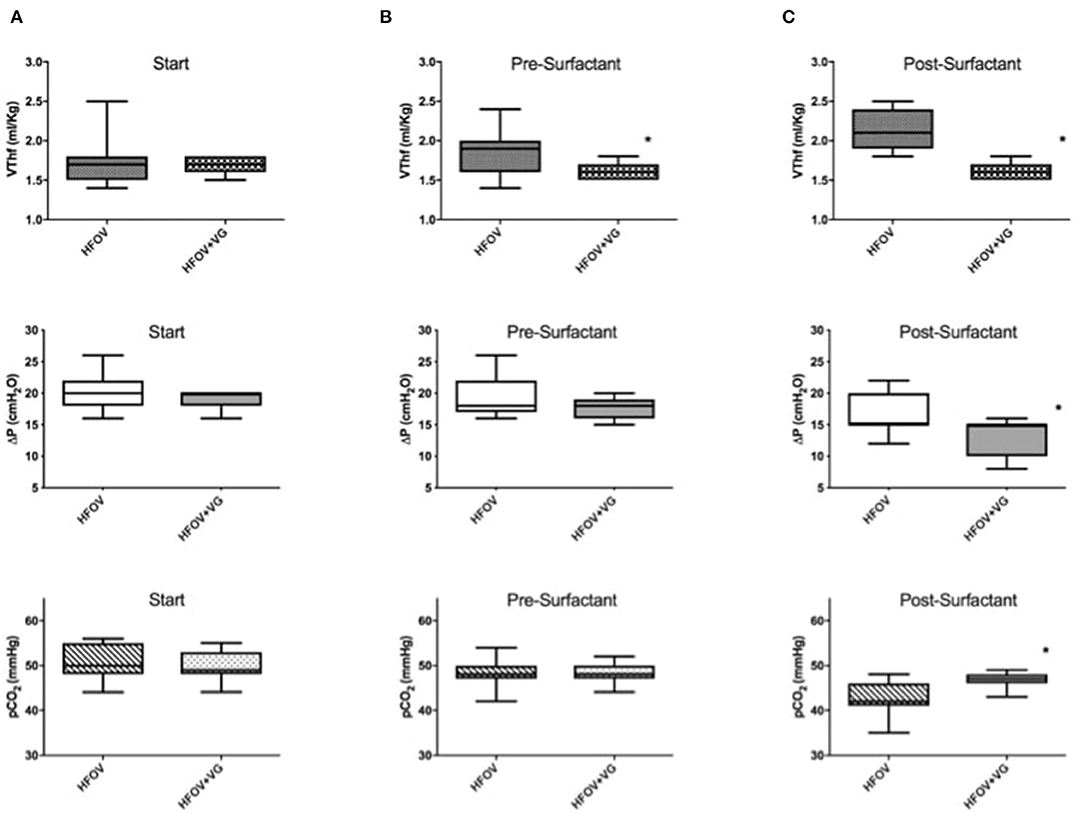
Figure 2. Evaluation of VThf (ml/kg), amplitude level (ΔP) and pCO2 in HFOV and HFOV + VG groups. (A) At start: no significant differences of the three parameters between the two groups were observed. (B) Pre-surfactant administration (after completing the lung recruitment maneuver): there is a significant difference between the two groups in terms of VThf/kg (p = 0.03). (C) Post-surfactant administration: there are significant differences between the two groups in terms of VThf/kg (p < 0.0001), ΔP (p = 0.005), and pCO2 (p = 0.006). *Represents statistically significant differences.
After surfactant administration, HFOV group, compared to HFOV + VG group, had significantly higher VThf/Kg (2.1 ± 0.3 vs. 1.6 ± 0.1; p < 0.0001) with significantly lower pCO2 values (43.1 ± 3.8 vs. 46.8 ± 1.5; p = 0.006). Moreover, six patients of HFOV group (54.4%) reached a pCO2 value below target of 45 mmHg and one patient with pCO2 below 35 mmHg of pCO2 (Figure 2).
A significant negative correlation was found between VThf/Kg values and the corresponding pCO2 after surfactant administration in all patients of both groups (r: −0.69; n:21, p < 0.0001; Figure 3).
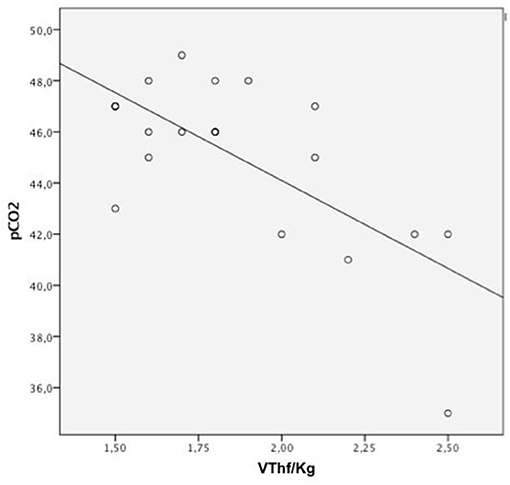
Figure 3. Pearson correlation analysis between VThf/kg, pCO2 significant correlation between all VThf/kg and pCO2 levels (Pearson coefficient: r = −0.69, p < 0.0001).
In both groups, the ΔP significantly decreases after surfactant administration, but comparing the 2 groups, the ΔP post-surfactant is lower in HFOV + VG with 13 ± 3 cm H2O vs. 17 ± 3 cm H2O; p = 0.01 (Figure 2 and Table 2).
Data on weight corrected gas transport coefficient (DCO2) in the HFOV and HFOV + VG groups show a mean DCO2 measurement significantly different, with higher values in HFOV both pre-surfactant (51.6 ± 16.3 vs. 39.4 ± 5.1; p = 0.03) and post-surfactant (69.5 ± 16.4 vs. 39.5 ± 5.8; p < 0.0001).
Discussion
To our knowledge, this is the first paper of comparison between HFOV and HFOV + VG during surfactant administration in extremely preterm neonates with RDS electively HFOV ventilated. Considering the last literature, a multicentre, randomized, controlled trial, IN-REC-SUR-E (37), demonstrated how surfactant administration after lung recruitment with HFOV decreased the need for MV in the first 72 h of life in extremely preterm infants compared to standard IN-SUR-E technique. Volume recruitment maneuver improves surfactant distribution and pulmonary gas exchange as also seen in animal studies (38). Conditions where alveolar ventilation is improved and lung compliance changes rapidly, like during open lung HFOV surfactant treatment, can result in volutrauma and hypocapnia (23–25). During HFOV, the same pressure amplitude and frequency can result in different chest oscillations and tidal volume due to changes in lung mechanics and patient–ventilator interactions. Data published on HFOV + VG in preterm infants constantly report the feasible use and a better maintenance of VThf and pCO2 in the target range (19–22). The aim of our study was to evaluate VG combined to HFOV during surfactant administration.
Avoiding volutrauma is a desirable goal in ventilation, with immediate and long term benefits associated to the prevention of both lung injury with BPD and hypocapnia that can alter cerebral blood flow with increased risk of intra-ventricular hemorrhage (IVH), periventricular leukomalacia (PVL), and poor neurodevelopmental outcomes (26–30).
In our study variations of VThf and ΔP were evaluated during surfactant administration, i.e., a rapidly lung compliance change phase, and the low and stable VThf/Kg observed in HFOV + VG patients cause a significant low DCO2 values in this group. The mean VThf/Kg levels used in our study, corresponding to normocapnic blood gases, were 1.5–1.8 ml/kg. We used a constant frequency of 15 Hz to reduce the determinants of DCO2. As Mukerji et al. demonstrated in artificial lung model, frequency has a direct relationship with CO2 elimination when tidal volume is fixed. Using low delivered tidal volumes and high frequencies may allow for improved ventilation efficacy, while minimizing lung injury (39).
Recently, it is reported that it is possible to use lower delivered tidal volumes during HFOV combined with VG and higher frequencies to allow minimizing lung injury. In experimental models, the protective effect of HFOV + VG has been proved when using smaller volumes and very high frequencies (40) which have been successfully used in preterm newborns in a pilot study of González-Pacheco (41). In a recent retrospective cohort study investigating the high-frequency parameters corresponding with adequate ventilation, the median high-frequency tidal volume corrected by birth weight was 1.63 ml/kg for frequencies 15 Hz, and tidal volumes were inversely correlated with frequencies used (21).
To date, there are no starting ventilation parameters defined for VG and frequency in HFOV ventilation. Few studies support the need for higher VThf during HFOV ranging between 1.75 and 1.90 ml/kg using a constant frequency of 10 Hz (18, 42). Another study in an almost homogeneous group of preterm infants, already treated with surfactant, used an average VThf of 1.64 ± 0.25 ml/kg (20).
In our highly homogeneous population of extremely preterm infants with RDS evaluated before and after surfactant administration, an HFOV + VG starting ventilator setting with VThf 1.5–1.8 ml/kg and a respiratory rate of 15 Hz has been demonstrated to be safe and efficacious.
This is a retrospective study, so the method of data recording is a first limitation. Another important limitation is the small number of patients evaluated in two different study periods. However, in our unit, HFOV + VG is nowadays the elective and routine modality of invasive ventilation and it is not possible to perform a randomized controlled trial. Finally, our patients received surfactant at a mean age of 2.5 h. The duration of the HFOV recruitment maneuver (average 20–30 min) may have contributed to the delay in surfactant administration, which is usually recommended soon after intubation.
In conclusion, although the optimal initial value of VThf cannot be exactly known because it can be influenced by prenatal conditions, sedation, and spontaneous breathing, in a selected population we can suggest safety values of VThf/kg and frequency to obtain normocapnia.
Our results show that HFOV + VG, compared to HFOV alone, avoids increasing VThf just after surfactant administration, reduces VThf fluctuations (as demonstrated by lower SD of mean VThf values), and reduces large pCO2 excursions and risk of hypocapnia.
Due to the lower ΔP and VThf values and to the reduced fluctuation of pCO2 levels, HFOV combined with VG appears to be suitable for extremely preterm infants, compared to HFOV alone, in the management of acute neonatal RDS before and after surfactant administration.
Data Availability Statement
The original contributions presented in the study are included in the article/supplementary material, further inquiries can be directed to the corresponding author/s.
Ethics Statement
The studies involving human participants were reviewed and approved by Comitato Etico, Policlinico Universitario, Agostino Gemelli—Roma. Written informed consent to participate in this study was provided by the participants' legal guardian/next of kin.
Author Contributions
MT, AP, and GV conceptualized and edited the final manuscript. RP supervised the statistical analysis. MT, AP, CT, CA, AL, AB, SC, and ET collaborated in clinical practice and data collection. All authors contributed to the article and approved the submitted version.
Conflict of Interest
The authors declare that the research was conducted in the absence of any commercial or financial relationships that could be construed as a potential conflict of interest.
Publisher's Note
All claims expressed in this article are solely those of the authors and do not necessarily represent those of their affiliated organizations, or those of the publisher, the editors and the reviewers. Any product that may be evaluated in this article, or claim that may be made by its manufacturer, is not guaranteed or endorsed by the publisher.
References
1. Support Support Study Group of the Eunice Kennedy Shriver NICHD Neonatal Research Network, Finer NN, Carlo WA, Walsh MC, Rich W, Gantz MG, et al. Early CPAP versus surfactant in extremely preterm infants. N Engl J Med. (2010) 362:1970–9. doi: 10.1056/NEJMoa0911783
2. Morley CJ, Davis PG, Doyle LW, Brion LP, Hascoet JM, Carliln JB, et al. Nasal CPAP or intubation at birth for very preterm infants. N Engl J Med. (2008) 358:700–8. doi: 10.1056/NEJMoa072788
3. Cools F, Offringa M, Askie LM. Elective high frequency oscillatory ventilation versus conventional ventilation for acute pulmonary dysfunction in preterm infants. Cochrane Database Syst Rev. (2015) 3:CD000104. doi: 10.1002/14651858.CD000104.pub4
4. Courtney SE, Durand DJ, Asselin JM, Hudak ML, Aschner JL, Shoemaker CT, et al. High frequency oscillatory ventilation versus conventional mechanical ventilation for very low birth weight infants. N Engl J Med. (2002) 347:643–52. doi: 10.1056/NEJMoa012750
5. Tana M, Zecca E, Tirone C, Aurilia C, Cota F, Lio A, et al. Target fraction of inspired oxygen during open lung strategy in neonatal high frequency oscillatory ventilation: a retrospective study. Minerva Anestesiol. (2012) 78:151–9.
6. Slutsky AS, Drazen JM Ventilation with small tidal volumes. N Engl J Med. (2002) 347:630–63. doi: 10.1056/NEJMp020082
7. Pillow JJ. High-frequency oscillatory ventilation: mechanisms of gas exchange and lung mechanics. Crit Care Med. (2005) 33(3 Suppl.):S135–41. doi: 10.1097/01.CCM.0000155789.52984.B7
8. Slutsky AS, Kamm RD, Rossing TH, Loring SH, Lehr J, Shapiro AH, et al. Effects of frequency, tidal volume, and lung volume on CO2 elimination in dogs by high frequency (2-30 Hz), low tidal volume ventilation. J Clin Invest. (1981) 68:1475–84. doi: 10.1172/JCI110400
9. Rossing TH, Slutsky AS, Lehr JL, Drinker PA, Kamm R, Drazen JM. Tidal volume and frequency dependence of carbon dioxide elimination by high-frequency ventilation. N Engl J Med. (1981) 305:1375–9. doi: 10.1056/NEJM198112033052303
10. Belteki G, Lin B, Morley CJ. Weight-correction of carbon dioxide diffusion coefficient (DCO2) reduces its inter-individual variability and improves its correlation with blood carbon dioxide levels in neonates receiving high-frequency oscillatory ventilation. Pediatr Pulmonol. (2017) 52:1316–22. doi: 10.1002/ppul.23759
11. Miedema M, de Jongh FH, Frerichs I, van Veenendaal MB, van Kaam AH. The effect of airway pressure and oscillation amplitude on ventilation in preterm infants. Eur Respir J. (2012) 40:479–84. doi: 10.1183/09031936.00138311
12. Zimov'a-Herknerov'a M, Plavka R Expired tidal volumes measured by hot-wire anemometer during high-frequency oscillation in preterm infants. Pediatr Pulmonol. (2006) 41:428–33. doi: 10.1002/ppul.20367
13. Klingenberg C, Wheeler KI, McCallion N, Morley CJ, Davis PG. Volume- targeted versus pressure-limited ventilation in neonates. Cochrane Database Syst Rev. (2017) 10:CD003666. doi: 10.1002/14651858.CD003666.pub4
14. Peng W, Zhu H, Shi H, Liu E. Volume-targeted ventilation is more suitable than pressure-limited ventilation for preterm infants: a systematic review and meta-analysis. Arch Dis Child Fetal Neonatal Ed. (2014) 99:F158–65. doi: 10.1136/archdischild-2013-304613
15. Duman N, Tuzun F, Sutcuoglu S, Yesihrmak CD, Kumral A, Ozkan H. Impact of volume gurantee on synchronized ventilation in preterm infants: a randomized controlled trial. Intensive Care Med. (2012) 38:1358–64. doi: 10.1007/s00134-012-2601-5
16. Keszler M. Volume-targeted ventilation: one size does not fit all. Evidence- based recommendations for successful use. Arch Dis Child Fetal Neonatal Ed. (2019) 104:F108–12. doi: 10.1136/archdischild-2017-314734
17. Enomoto M, Keszler M, Sakuma M, Kikuchi S, Katayama Y, Takei A, et al. Effect of volume guarantee in preterm infants on high-frequency oscillatory ventilation: a pilot study. Am J Perinatol. (2017) 34:26–30. doi: 10.1055/s-0036-1584141
18. Iscan B, Duman N, Tuzun F, Kumral A, Ozkan H. Impact of volume guarantee on high-frequency oscillatory ventilation in preterm infants: a randomized crossover clinical trial. Neonatology. (2015) 108:277–82. doi: 10.1159/000437204
19. Belteki G, Morley CJ. high-frequency oscillatory ventilation with volume guarantee: a single -centre experience. Arch Dis Child Fetal Neonatal Ed. (2018) 104:F384–9. doi: 10.1136/archdischild-2018-315490
20. Funda T, Burak D, Merve MC, Burcin I, Nuray D, Hasan O. Volume guarantee high-frequency oscillatory ventilation in preterm infants with RDS: tidal volume and DCO2 levels for optimal ventilation using open-lung strategies. Front Pediatr. (2020) 8:105. doi: 10.3389/fped.2020.00105
21. Solís-García G, González-Pacheco N, Ramos-Navarro C, Sánchez de la Blanca AR, Sánchez-Luna M. Target volume-guarantee in high-frequency oscillatory ventilation for preterm respiratory distress syndrome: low volumes and high frequencieslead to adequate ventilation. Pediatr Pulmonol. (2021) 56:2597–603. doi: 10.1002/ppul.25529
22. Tana M, Polglase GR, Cota F, Tirone C, Aurilia C, Lio A, et al. Determination of lung volume and hemodynamic changes during high-frequency ventilation recruitment in preterm neonates with respiratory distress syndrome. Crit Care Med. (2015) 43:1685–91. doi: 10.1097/CCM.0000000000000967
23. Couser RJ, Ferrara TB, Ebert J, Hoekstra RE, Fangman JJ. Effects of exogenous surfactant therapy on dynamic compliance during mechanical breathing in preterm infants with hyaline membrane disease. J Pediatr. (1990) 116:119–24. doi: 10.1016/S0022-3476(05)81660-9
24. Dinger J, Topfer A, Schaller P, Schwarze R. Functional residual capacity and compliance of the respiratory system after surfactant treatment in premature infants with severe respiratory distress syndrome. Eur J Pediatr. (2002) 161:485–90. doi: 10.1007/s00431-002-0989-6
25. Miedema M, de Jongh FH, Frerichs I, van Veenendaal MB, van Kaam A. Changes in lung volume and ventilation during surfactant treatment in ventilated preterm infants. Am J Respir Crit Care Med. (2011) 184:100–5. doi: 10.1164/rccm.201103-0375OC
26. Björklund LJ, Ingimarsson J, Curstedt T, John J, Werner O, Vilstrp CT. Manual ventilation with a few large breaths at birth compromises the therapeutic effect of subsequent surfactant replacement in immature lambs. Pediatr Res. (1997) 42:348–55. doi: 10.1203/00006450-199709000-00016
27. Wyatt JS1, Edwards AD, Cope M, Delpy DT, McCormick DC, Potter A, et al. Response of cerebral blood volume to changes in arterial carbon dioxide tension in preterm and term infants. Pediatr Res. (1991) 29:553–7. doi: 10.1203/00006450-199106010-00007
28. Meek JH1, Tyszczuk L, Elwell CE, Wyatt JS. Cerebral blood flow increases over the first three days of life in extremely preterm neonates. Arch Dis Child Fetal Neonatal Ed. (1998) 78:F33–7. doi: 10.1136/fn.78.1.F33
29. Resch B, Neubauer K, Hofer N, Resch E, Maurer U, Haas J, et al. Episodes of hypocarbia and early-onset sepsis are risk factors for cystic periventricular leukomalacia in the preterm infant. Early Hum Dev. (2012) 88: 27–31. doi: 10.1016/j.earlhumdev.2011.06.011
30. Greisen G, Munck H, Lou H. Severe hypocarbia in preterm infants and neurodevelopmental deficit. Acta Paediatr Scand. (1987) 76:401–4. doi: 10.1111/j.1651-2227.1987.tb10489.x
31. Sánchez Luna M, Santos González M, Tendillo Cortijo F. High-frequency oscillatory ventilation combined with volume guarantee in a neonatal animal model of respiratory distress syndrome. Crit Care Res Pract. (2013) 2013:593915. doi: 10.1155/2013/593915
32. Vento G, Matassa PG, Ameglio F, Capoluongo E, Zecca E, Tortorolo L, et al. HFOV in premature neonates: effects on pulmonary mechanics and epithelial lining fluid cytokines. A randomized controlled trial. Intensive Care Med. (2005) 31:463–70. doi: 10.1007/s00134-005-2556-x
33. De Jaegere A, van Veenendaal MB, Michiels A, van Kaam AH. Lung recruitment using oxygenation during open lung high-frequency ventilation in preterm infants. Am J Respir Crit Care Med. (2006) 174:639–45. doi: 10.1164/rccm.200603-351OC
34. De Waal K, Evans N, van der Lee J, van Kaam A. Effect of lung recruitment on pulmonary, systemic, and ductal blood flow in preterm infants. J Pediatr. (2009) 154:651–5. doi: 10.1016/j.jpeds.2009.01.012
35. Schimdt B, Roberts RS, Davis P, Doyle LW, Barrington J, Ohlsson A, et al. Caffeine therapy for apnea of prematurity. N Engl J Med. (2006) 354:2112–21. doi: 10.1056/NEJMoa054065
36. Giannantonio C, Sammartino M, Valente E, Cota F, Fioretti M, Papacci P. Remifentanil analgosedation in preterm newborns during mechanical ventilation. Acta Paediatr. (2009) 98:1111–5. doi: 10.1111/j.1651-2227.2009.01318.x
37. Vento G, Ventura ML, Pastorino R, van Kaam AH, Carnielli V, Cools F, et al. Lung recruitment before surfactant administration in extremely preterm neonates with respiratory distress syndrome (IN-REC-SUR-E): a randomised, unblinded, controlled trial. Lancet Respir Med. (2020) 9:P159–66. doi: 10.1016/S2213-2600(20)30179-X
38. Krause MF, Jäkel C, Haberstroh J, Schulte-Mönting J, Leititis JU, Orlowska-Volk M. Alveolar recruitment promotes homogenous surfactant distribution in a piglet model of lung injury. Pediatr Res. (2001) 50:34–43. doi: 10.1203/00006450-200107000-00009
39. Mukerji A, Belik J, Sanchez-Luna M. Bringing back the old: time to reevaluate the high-frequency ventilation strategy. J Perinatol. (2014) 34:464–7. doi: 10.1038/jp.2014.39
40. González-Pacheco N, Sánchez-Luna M, Chimenti-Camacho P, Santos- González M, Palau-Concejo P, Tendillo-Cortijo F. Use of very low tidal volumes during high-frequency ventilation reduces ventilator lung injury. J Perinatol. (2019) 39:730–6. doi: 10.1038/s41372-019-0338-5
41. González-Pacheco N, Sánchez-Luna M, Ramos-Navarro C, Navarro-Pati1o N, de la Blanca AR. Using very high frequencies with very low lung volumes during high- frequency oscillatory ventilation to protect the immature lung. A pilot study. J Perinatol. (2016) 36:306. doi: 10.1038/jp.2015.197
Keywords: HFOV, volume guarantee, ELGAN, respiratory distress syndrome, lung recruitment
Citation: Tana M, Paladini A, Tirone C, Aurilia C, Lio A, Bottoni A, Costa S, Tiberi E, Pastorino R and Vento G (2022) Effects of High-Frequency Oscillatory Ventilation With Volume Guarantee During Surfactant Treatment in Extremely Low Gestational Age Newborns With Respiratory Distress Syndrome: An Observational Study. Front. Pediatr. 9:804807. doi: 10.3389/fped.2021.804807
Received: 29 October 2021; Accepted: 28 December 2021;
Published: 03 March 2022.
Edited by:
Hans Fuchs, University of Freiburg Medical Center, GermanyReviewed by:
Martin Keszler, Women & Infants Hospital of Rhode Island, United StatesClaus Klingenberg, UiT the Arctic University of Norway, Norway
Copyright © 2022 Tana, Paladini, Tirone, Aurilia, Lio, Bottoni, Costa, Tiberi, Pastorino and Vento. This is an open-access article distributed under the terms of the Creative Commons Attribution License (CC BY). The use, distribution or reproduction in other forums is permitted, provided the original author(s) and the copyright owner(s) are credited and that the original publication in this journal is cited, in accordance with accepted academic practice. No use, distribution or reproduction is permitted which does not comply with these terms.
*Correspondence: Giovanni Vento, giovanni.vento@unicatt.it
†These authors have contributed equally to this work and share first authorship