- 1Department of Paediatrics and Adolescent Medicine, Aarhus University Hospital, Aarhus, Denmark
- 2Department of Clinical Medicine, Aarhus University, Aarhus, Denmark
Background: Studies have suggested that neurological outcome may differ in newborns with encephalopathy with and without perinatal infection. We aimed to systematically review this association.
Methods: We conducted this systematic review according to the Preferred Reporting Items for Systematic Reviews and Meta-Analyses (PRISMA). Studies were obtained from four databases including Pubmed, Embase, Web of Science, and The Cochrane Database. Newborns with encephalopathy with and without markers of perinatal infection were compared with regard to neurodevelopmental assessments, neurological disorders, and early biomarkers of brain damage. Risk of bias and quality of evidence were assessed by the Newcastle-Ottawa scale and Grading of Recommendations Assessment, Development and Evaluation (GRADE).
Results: We screened 4,284 studies of which eight cohort studies and one case-control study met inclusion criteria. A narrative synthesis was composed due to heterogeneity between studies. Six studies were classified as having low risk of bias, while three studies were classified as having high risk of bias. Across all outcomes, the quality of evidence was very low. The neurological outcome was similar in newborns with encephalopathy with and without markers of perinatal infection.
Conclusions: Further studies of higher quality are needed to clarify whether perinatal infection may affect neurological outcome following newborn encephalopathy.
Systematic Review Registration: https://www.crd.york.ac.uk/prospero/, identifier CRD42020185717.
Introduction
Neonatal encephalopathy due to intrapartum-related events–newborn encephalopathy–is a major contributor to infant mortality and neurodevelopmental morbidity (1). When hypoxia-ischemia is the suspected cause of newborn encephalopathy, therapeutic hypothermia may be applied as neuroprotective treatment (2). However, some 50% of all newborns treated with hypothermia still develop unfavorable neurological outcomes (2, 3).
While hypoxia-ischemia may be commonly involved in newborn encephalopathy, the etiology remains multifactorial (4, 5). Perinatal infection is also associated with newborn encephalopathy (6–14). Ascending bacteria may spread to the fetus or result in fetal inflammatory response syndrome, characterized by systemic and local inflammation in the fetal organs including the brain (15, 16). Bacteria from the birth canal may also be transmitted during delivery leading to early-onset infection with similar activation of the newborn inflammatory system (17). Infection and inflammation in the central nervous system may cause encephalopathy and brain damage per se or act in combination with other insults such as hypoxia-ischemia (18–20). This multiple-hit hypothesis states that one insult could sensitize the fetal or newborn brain in order for secondary insults to have larger clinical impact (20–23). Several animal studies have found that endotoxin-induced inflammation prior to hypoxia-ischemia severely exacerbates brain injury in newborns (24–31). In addition to the possible aggravation of brain damage, both animal (32–37) and clinical studies (38–41) have suggested that infections may affect both the efficacy and safety of therapeutic hypothermia.
Accordingly, perinatal infection may interfere with the prognosis in newborns with encephalopathy. A systematic review of this association in humans may qualify the need for differential treatment in newborns with encephalopathy born by mothers with infection or with infection themselves. Therefore, the aim of this systematic review was to compare newborns with encephalopathy with and without markers of perinatal infection with regard to neurological outcome including neurodevelopmental assessments, neurological disorders, and early biomarkers of brain damage.
Methods
This systematic review was performed in accordance with the Preferred Reporting Items for Systematic Reviews and Meta-analyses (PRISMA) (Supplementary A) (42). The protocol was registered 12th of May 2020 at the International Prospective Register of Systematic Reviews (PROSPERO) (CRD42020185717) (43). Two authors (MA and MVP) independently screened articles, assessed eligibility, extracted data, and analyzed risk of bias and quality of evidence. Any disagreements were resolved by discussion or by a third reviewer (TBH).
Terminology
Neonatal encephalopathy is a broad term including different etiologies, while hypoxic-ischemic encephalopathy may be used when hypoxia-ischemia is the most likely cause of the encephalopathy (44, 45). In this systematic review, we used the term “newborn encephalopathy” to emphasize our interest in insults surrounding the birth and the uncertainty with underlying causes. However, when discussing the individual studies, we continued the use of their specific terminology. In addition, no consensus definition of neonatal sepsis currently exists (46). Positive blood culture remains the golden standard of diagnosis. However, several neonates are believed to have sepsis without ever having isolated the specific pathogen (47, 48). Again, we opted to continue the terminology and definition of sepsis used in each individual study.
Eligibility Criteria
Studies
We only included peer-reviewed studies. Both observational studies and randomized controlled trials were eligible for inclusion, while case reports and case series were excluded. No restrictions by language or publication dates were applied.
Population
Newborns with a gestational age of ≥36 completed weeks or with a birth weight of ≥2500 g.
Exposure
Newborns with a diagnosis of neonatal encephalopathy or hypoxic-ischemic encephalopathy in combination with markers of bacterial infection in the mother or child during the perinatal period. Markers of maternal infection included clinical or histological chorioamnionitis, funisitis, and/or chorionic vasculitis. Markers of early-onset infection included infection proven by culture or molecular testing or suspected infection as assessed by the clinician based on clinical features, decision to initiate and continue antimicrobial treatment, and biomarkers including either white blood-cell counts, neutrophil counts, ratio of immature to total neutrophils, C-reactive protein, procalcitonin, or interleukins (IL-6, IL-8, or IL-10).
Comparators
Newborns with a diagnosis of neonatal encephalopathy or hypoxic-ischemic encephalopathy without the markers of bacterial infection in the mother or child during the perinatal period.
Outcome
The primary outcome was the composite outcome of (1) scales and tools for assessing neurodevelopmental function, (2) neurological disorders including seizure-, motor-, cognitive-, mental-, and behavioral disorders, and (3) mortality. The secondary outcomes were defined as the above outcomes assessed separately except for mortality. Furthermore, we included biomarkers of early brain damage such as magnetic resonance imaging (MRI) measures and MR spectroscopy, as well as conventional and amplitude-integrated electroencephalography (49, 50).
Information Sources and Search Strategy
The search strategy was developed by the authors and tested before use. The primary search was conducted 12 April 2021. Pubmed, Embase, Web of Science, and The Cochrane Database were searched by use of subject headings and free texts related to newborns, encephalopathy, and infection (Supplementary B). Our search was limited to human studies. References and citations from each included study were manually scrutinized for additional relevant studies.
Study Selection
The search results from each database were pooled using Endnote X9® and duplicates were removed. Rayyan QCRI was used in the screening process (51). Studies found by search were screened by title and abstract, while references and citations from included studies were screened by title only. All studies that seemingly met the eligibility criteria or provided insufficient information were extracted for full-text analysis. If any information regarding the eligibility criteria was missing, the study was excluded (Supplementary C).
Data Collection and Data Items
Data were extracted by a predefined data-collection form. To reduce errors and missing data plots, the data form was piloted prior to data extraction. Following items were extracted from each study: (1) title, authors, country, journal, year of publication, references, citations, funding sources, and conflicts of interest; (2) aim of the study, design, setting, and time-period; (3) number and characteristics of the newborns; (4) assessments of encephalopathy and whether the newborns received therapeutic hypothermia; (5) assessments of maternal and newborn infections; (6) assessments of neurological outcome; and (7) statistical methods and results (Supplementary D).
Risk of Bias in Individual Studies
The Newcastle-Ottawa Scale for cohort studies was used to evaluate the risk of bias. The studies were evaluated on the risk of bias in the domains: selection process, comparability between groups, and assessment of outcome. A total of four, two, and three points could be awarded in each domain, respectively. The risk of bias in the studies was rated as “low” when awarded 3–4 points in selection, 1–2 points in comparability, and 2–3 points in outcome; “fair” when awarded 2 points in selection, 1-2 points in comparability, and 2–3 points in outcome; and “high” when awarded 0–1 point in selection or 0 point in comparability or 0–1 point in outcome. When assessing comparability, one point was awarded if the study controlled for malformations. An additional point was awarded if the study controlled for gestational age, birth weight, gender, or metabolic diseases.
Synthesis of Results
No meta-analysis was performed due to the heterogeneity between the studies with regard to the assessment of encephalopathy, infection, and neurological outcome. A narrative synthesis of the results was made in accordance with Popay et al. (52). In studies with inadequate summary measures, a Chi-square test or Fischer's exact test was performed to assess odds ratio with 95% confidence interval. Analyses were performed by GraphPad Prism version 8.0.0 for Mac (GraphPad Software, San Diego, California USA, www.graphpad.com).
Quality of Evidence
The quality of evidence was assessed in accordance with GRADE (53, 54). A total of five domains were evaluated including the risk of bias, directness of evidence, heterogeneity, precision of effect estimates, and risk of publication bias. Due to their observational design, the studies started with an initial rating of low quality. The studies were then downgraded for serious limitations in any of the five domains and would only be upgraded if no downgrading had occurred. Accordingly, the quality of evidence could be rated as high, moderate, low, or very low.
Risk of Bias Across Studies
Publication bias was assessed qualitatively based on the characteristics of the included studies as an insufficient number of studies was included for formal test of asymmetry. Selective reporting bias was assessed by comparing the outcomes reported in the method section and the result section of the included studies.
Results
Study Selection
A total of 4,256 studies were identified across all databases. After removal of duplicates, 3,254 studies were screened for inclusion. Of these, 55 studies were evaluated by full-text and seven studies were eligible for inclusion. Two additional studies were included following screening of 1,030 references and citations from already included studies. This led to inclusion of nine studies for this systematic review (55–63). An overview of the selection process is illustrated in the PRISMA flow diagram (Figure 1).
Study Characteristics
Designs and Populations
Eight cohort studies (55–61, 63) and one case-control study (62) were included. Table 1 provides number, gestational age, birth weight, and gender of the newborns, separated by infection status when possible. Three studies from the Netherlands have overlapping populations (56, 57, 61). Hakobyan et al. (56) only included historical controls (64). Newborns with encephalopathy fulfilled criteria for therapeutic hypothermia in seven studies (55–60, 63), while hypothermia was introduced midtrial in a single study (61). One study was conducted before the implementation of hypothermia (62).
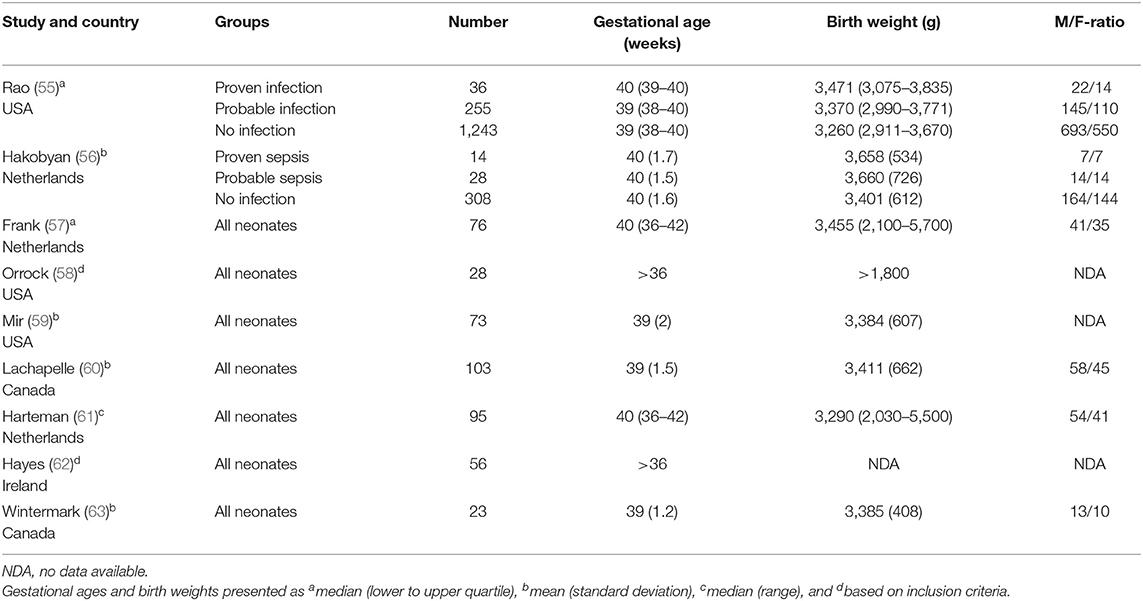
Table 1. Number, gestational age, birth weight, and male to female (M/F) ratio of the newborns in the studies investigating the association between newborn encephalopathy, perinatal infection, and neurological outcome.
Markers of Hypoxia-Ischemia
Table 2 provides the potential markers of hypoxia-ischemia, which was part of the inclusion criteria in each study. Six studies stated that they included newborns with encephalopathy and biochemical criteria suggestive of hypoxia-ischemia including low umbilical arterial pH and high base deficit (55, 58–61, 63). The occurrence of perinatal sentinel events as potential causes of hypoxia-ischemia was part of the criteria in five studies; however, the occurrence was not fully necessary for inclusion (55, 58–60, 63). Three studies solely stated that they included newborns with encephalopathy with signs of asphyxia or due to presumed hypoxia-ischemia (56, 57, 62). However, biochemical markers and other signs of fetal and neonatal distress were presented in the descriptive data of the studies.
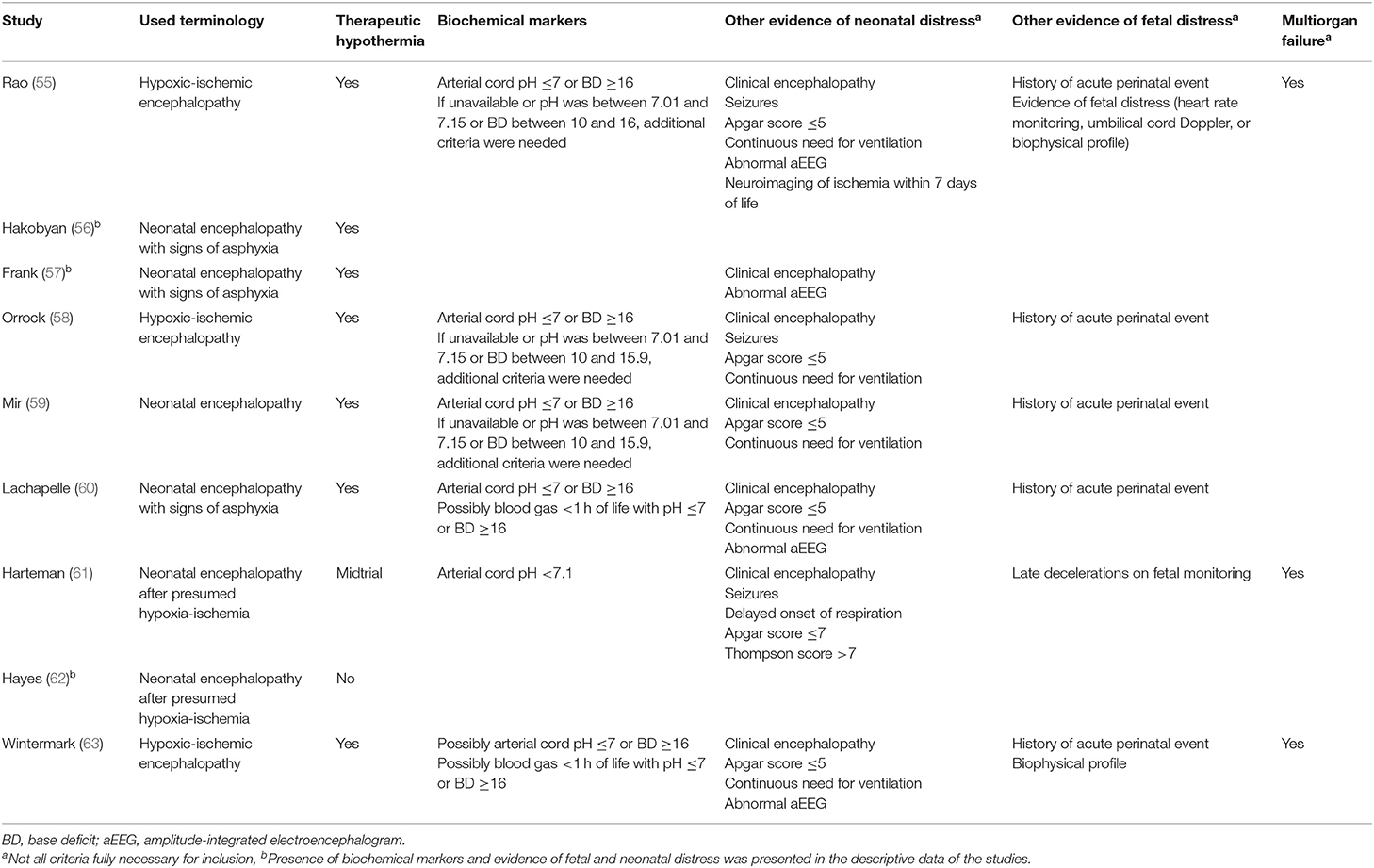
Table 2. Possible markers of hypoxia-ischemia as part of inclusion criteria in the studies investigating the association between newborn encephalopathy, perinatal infection, and neurological outcome.
Markers of Perinatal Infection
Seven studies investigated newborns born by mothers with markers of infection (57–63). Six studies stated that histological chorioamnionitis, funisitis, or chorionic vasculitis were assessed by a blinded pathologist (57, 59–63), while one study stated that the diagnoses were obtained from placental pathology reports (58). Two studies investigated newborns with markers of infection (55, 56). Rao et al. (55) identified infections within seven days of life. Proven infection was defined by a positive culture (blood, urine, cerebrospinal fluid, airways), while suspected newborns had negative cultures but still received antimicrobial treatment for 7 to 10 days. Hakobyan et al. (56) included newborns who all showed clinical signs of sepsis within 48 h. In addition, the newborns had positive blood cultures, positive surface cultures, or elevated C-reactive protein (≥50 mg/L). All newborns received antimicrobial treatment for at least 7 days.
Neurological Outcomes
Tables 3, 4 provide timing of neurological assessment in each study, which spanned from soon after birth to around 24 months of life. Neurodevelopmental outcomes were investigated in three studies (56, 57, 59). Hakobyan et al. and Frank et al. (56, 57) defined adverse neurological outcome as death, neurological disability (e.g., cerebral palsy), or abnormal Griffiths' neurodevelopmental quotient (<88 or 85) or Bayley Scales of Infant and Toddler Development-III (Bayley-III) score (<85). Mir et al. (59) also investigated a composite outcome consisting of death or low Bayley-III score (<85). MRI findings were investigated in seven studies (55, 57, 58, 60–63). Rao et al. (55) obtained MRI findings classified as either normal, abnormal, cortical injury, deep gray matter injury, or white matter injury. Frank et al. and Harteman et al. (57, 61) classified patterns of brain injury by MRI as normal, white matter/watershed injury, basal-ganglia-thalamus injury, or injury in both. Classification in similar manner based on Barkovich et al. (65) was used in three studies (58, 60, 62). Wintermark et al. (63) identified MRI findings showing evidence of hypoxic-ischemic brain injury. Two studies used postmortem brain examinations or cerebral ultrasound when MRI was unavailable (57, 60).
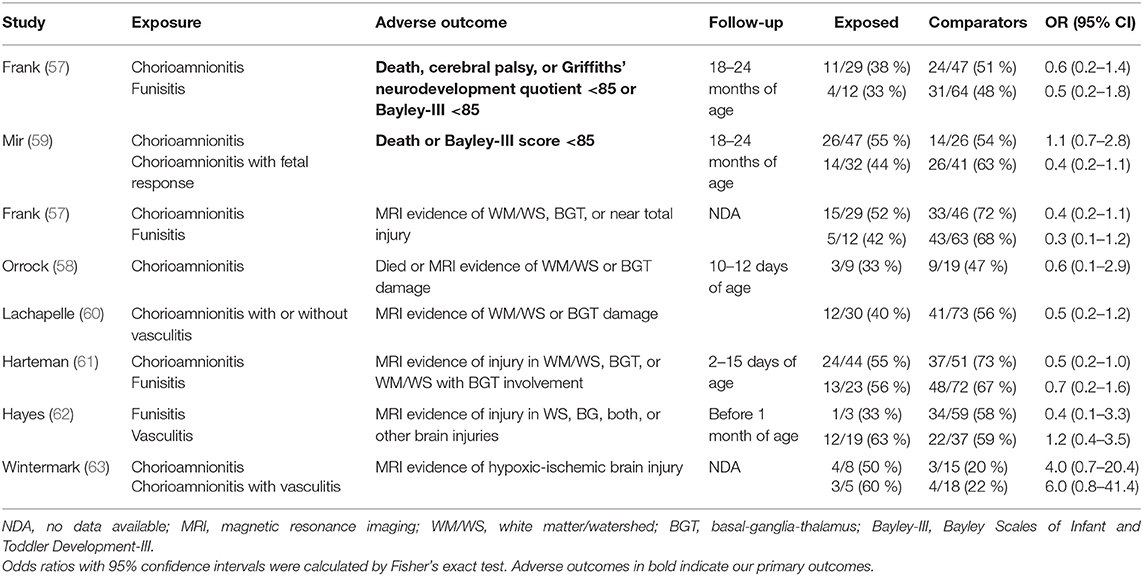
Table 3. Odds ratios (OR) with 95% confidence intervals (CI) of unfavorable neurological outcome between newborns with encephalopathy with and without maternal infection.
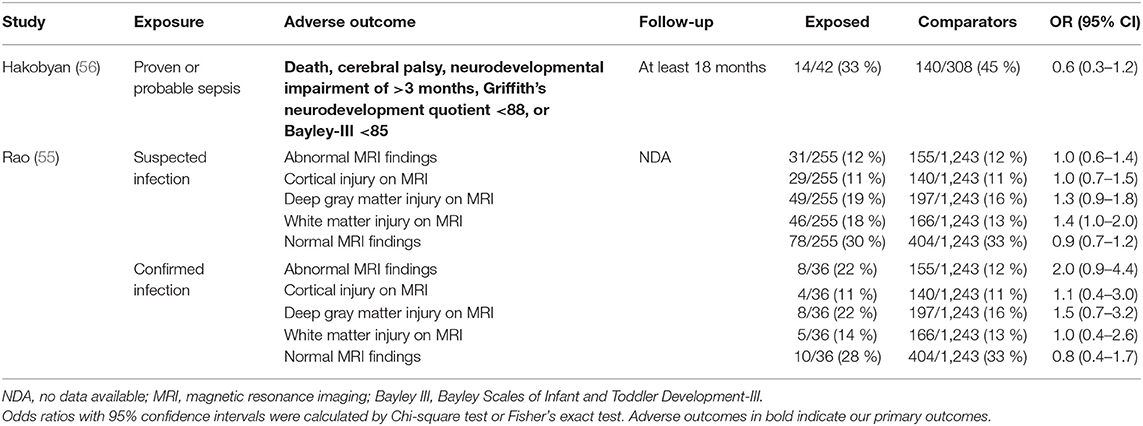
Table 4. Odds ratios (OR) with 95% confidence intervals (CI) of unfavorable neurological outcome in newborns with encephalopathy with and without early-onset infection.
Risk of Bias Within Studies
Table 5 provides an overview of the points awarded by the Newcastle-Ottawa Scale. The studies failed to report on several of the selected risk factors for quality assessment and three studies failed to report on any of these (59, 60, 63). Rao et al. (55) excluded newborns with major congenital anomalies and found no difference between newborns with and without early-onset infection with regard to gestational age and gender, but observed that newborns with infections had higher birth weights. Hakobyan et al. (56) found no difference between newborns with and without early-onset sepsis with regard to gestational age, birth weight, and gender. Orrock et al. (58) excluded newborns with major congenital abnormalities. Three studies excluded newborns with either chromosomal or metabolic disorders (57, 61, 62). In total, six studies were classified as having low risk of bias (55–58, 61, 62), while three studies were classified as having high risk of bias (59, 60, 63).
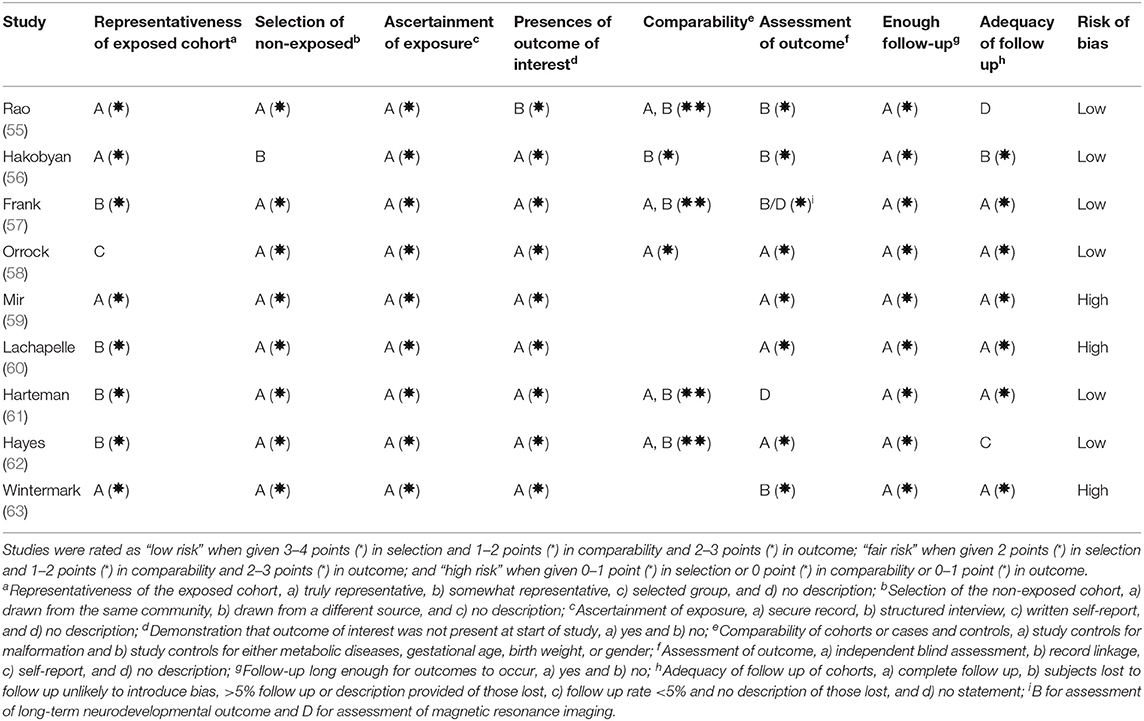
Table 5. Points awarded by the Newcastle-Ottawa Scale to the included studies investigating the association between newborn encephalopathy, perinatal infection, and neurological outcome.
Results of Individual Studies
Maternal Infection
A summary of the results is presented in Table 3. The studies found no statistically significant difference in neurological outcome between newborns with encephalopathy born by mothers with and without markers of infection (57–63). Frank et al. and Mir et al. (57, 59) found tendencies toward more favorable neurodevelopment in newborns born by mothers with chorioamnionitis or funisitis. With regard to neuroimaging, most studies also reported tendencies toward more favorable MRI findings in newborns with maternal infections (57, 58, 60–62).
Early-Onset Infection
A summary of the results is presented in Table 4. Hakobyan et al. (56) found no difference in death or unfavorable neurodevelopment between newborns with neonatal encephalopathy with and without markers of early-onset sepsis. Rao et al. (55) similarly found no difference in MRI findings between newborns with hypoxic-ischemic encephalopathy with and without proven or suspected early-onset infections.
Therapeutic Hypothermia
The studies found no statistically significant difference between hypothermia-treated newborns with encephalopathy with and without markers of perinatal infection (55–60, 63).
Quality of Evidence
We found that the quality of evidence across all outcomes was very low according to the GRADE assessment. This was mainly due to a concern related to risk of bias within the studies and the small number of included newborns. Furthermore, early MRI findings as proxy measures for neurodevelopment subjected the studies to downgrading for indirectness (53, 54).
Risk of Bias Across Studies
Selective reporting bias was not identified. Several of the studies reported no difference in neurological outcome between newborns with and without markers of perinatal infections. Furthermore, the association between perinatal infections and neurological outcome was not the primary aim in several studies (55, 57–63). Therefore, publication bias seems improbable.
Discussion
Summary of Evidence
Based on the included studies, the presence of perinatal infection does not seem to impact neurological outcome in newborns with encephalopathy. However, the quality of evidence was very low.
Markers of maternal infection have been associated with newborn encephalopathy (6–12). However, we found no further association with worse neurological outcome. Some studies on maternal infection were excluded from this systematic review, despite having some relevance (Supplementary C). Nelson et al. (66) found that the combination of perinatal sentinel events and markers of maternal infection was associated with an increased risk of cerebral palsy compared with perinatal sentinel events alone. Jenster et al. (67) investigated newborns with clinical and biochemical evidence of hypoxia-ischemia including 5-min Apgar score ≤5 and arterial cord pH <7.1 and base deficit >10. By contrast, they found that chorioamnionitis was associated with more favorable MRI findings at around 5 days of age and more favorable neurodevelopment assessed by Bayley-II or III at around 30 months of age. Early-onset infections may be more prevalent in newborns with encephalopathy and are associated with increased mortality (13, 14). However, as with maternal infections, we found no difference in neurological outcome between newborns with encephalopathy with and without early-onset infection. Both included studies on early-onset infections had a limited number of newborns with positive cultures. Hakobyan et al. (56) analyzed the combined number of newborns with documented and suspected sepsis. Only a smaller fraction (<1/3) considered to have an infection had positive culture, making the diagnosis less well defined. Furthermore, the study only included historical controls, which further limits the quality of evidence. Again, some studies on early-onset infection with some relevance for the topic were excluded (Supplementary C). Scheidegger et al. (68) found no difference in the Sarnat Staging during the first days of life between newborns with hypoxic-ischemic encephalopathy with and without early-onset sepsis (69). However, Jenster et al. (67) found early-onset sepsis to be associated with worse neuromotor function at around 30 months of age in newborns with clinical and biochemical markers of hypoxia-ischemia. In addition, when combining newborns with encephalopathy treated with and without hypothermia from a recent randomized controlled trial in low- and middle-income countries, culture-positive early- and late-onset sepsis were associated with increased risk of death or disability at 18 months (39).
Animal studies have suggested that infectious and inflammatory exposure before hypoxia-ischemia exacerbate newborn brain injury (24–31). Several biological mechanisms have been suggested to explain these findings (70–86). However, this systematic review of human studies was not able to substantiate these findings. This may be due to more heterogeneity inherent in clinical compared to experimental studies. A previous study found perinatal sentinel events, as potential causes of hypoxia-ischemia, only to be present in some 15% of newborns with encephalopathy (8). The timing between different perinatal insults may also have influenced the neurological outcome. Animal studies have found that lipopolysaccharides from Escherichia coli administered 4 h, 6 h, and 72 h before hypoxia-ischemia result in more severe brain damage, while lipopolysaccharides administered 24 h before hypoxia-ischemia had neuroprotective effects (24, 25, 86, 87). Therefore, both positive and negative conditioning may occur in the fetal or newborn brain when exposed to multiple insults (88). This may explain the findings of the included study by Harteman et al. (61), who found that newborns with hypoxic-ischemic encephalopathy born by mothers with chorioamnionitis had the highest incidence of both normal and most severe MRI findings.
Several newborns with encephalopathy due to presumed hypoxia-ischemia still develop unfavorable neurological outcomes despite treatment with therapeutic hypothermia (2, 3). Several reviews have postulated that therapeutic hypothermia may be contraindicated in newborns with encephalopathy and infection (19, 20, 89, 90). This systematic review found similar neurological outcome between hypothermia-treated newborns with encephalopathy with and without markers of perinatal infection (55–60, 63). However, due to the very low quality of evidence across all outcomes, this concern may still be valid. To our knowledge, no randomized clinical trial has sufficiently investigated the neuroprotective effect of hypothermia in newborns with encephalopathy and perinatal infection, although no association between hypothermia and the risk of sepsis has been found (2). Therapeutic hypothermia has been shown to delay C-reactive protein response and to suppress white blood-cell count (91, 92). These findings may partly explain the reduced efficacy of hypothermia observed in low- and middle-income countries (where infections may be more prevalent) (38, 39), and the increased risk of mortality and prolonged shock observed in hypothermia-treated adults with meningitis and sepsis (40, 41). Furthermore, animal studies have found hypothermia to have limited neuroprotective effect following lipopolysaccharide-sensitized hypoxia-ischemia (32–37, 93). Both studies on early-onset infections included in this systematic review reported an overrepresentation of Gram-positive bacteria (55, 56). Contrarily, animal studies have found hypothermia to have some neuroprotective effect following sensitization by endotoxins deriving from Gram-positive bacteria (34, 94).
Strengths and Limitations
We conducted this systematic review according to the PRISMA guidelines (42). We followed a preregistered protocol. We conducted a systematic search across four different databases. To minimize bias, each step was performed independently by two reviewers including screening of studies, data collection, and risk of bias and quality of evidence assessment. However, several limitations have to be considered. Newborn encephalopathy may arise from different etiologies including hypoxia-ischemia, infection and inflammation, placental pathologies, and more (5). These factors may alone or together affect the fetal or newborn brain. In the included studies, newborn encephalopathy may have occurred due to various factors and interactions. However, most studies presented criteria suggestive of both hypoxia-ischemia and perinatal infection, indicating that these factors were involved to some degree. The assessments of neurological outcomes may also be problematic. Most studies reported MRI findings, which not necessarily correlates with the neurodevelopmental outcome (95, 96). Furthermore, the longest follow-up time in the included studies was around 24 months of age. It would have been interesting to observe whether any differences in neurological outcome would develop throughout childhood and adolescence (97). Furthermore, due to inadequate reporting in several studies, we were unable to reject that the reference groups also contained mothers or newborns exposed to perinatal infections. This have likely biased the studies toward findings of no difference. At last, most included studies also contained small number of newborns and the comparability between newborns with and without perinatal infections was limited. This led to imprecision of the effect sizes and a high possibility of risk of bias. A meta-analysis could have increased the precision; however, the included studies were deemed to heterogenous.
Conclusion
We found no difference in neurological outcome between newborns with encephalopathy with and without markers of perinatal infection. However, the current quality of evidence within this subject is very low. Therefore, further studies are needed with larger sample sizes, longer follow-up time, less risk of bias, and more detailed description of populations with reports of possible etiologies and interactions.
Data Availability Statement
The original contributions presented in the study are included in the article/Supplementary Material, further inquiries can be directed to the corresponding author.
Author Contributions
MA, MP, TA, KK, and TH designed the study. MA and MP undertook data collection and analysis. MA drafted the manuscript. All authors have critically reviewed the drafted manuscript and have approved the manuscript and agree to be accountable for all aspects of the work.
Funding
This study was supported by Aarhus University (Graduate School of Health) and The Elsass Foundation (19-3-0577).
Conflict of Interest
The authors declare that the research was conducted in the absence of any commercial or financial relationships that could be construed as a potential conflict of interest.
Publisher's Note
All claims expressed in this article are solely those of the authors and do not necessarily represent those of their affiliated organizations, or those of the publisher, the editors and the reviewers. Any product that may be evaluated in this article, or claim that may be made by its manufacturer, is not guaranteed or endorsed by the publisher.
Supplementary Material
The Supplementary Material for this article can be found online at: https://www.frontiersin.org/articles/10.3389/fped.2021.787804/full#supplementary-material
References
1. Lee AC, Kozuki N, Blencowe H, Vos T, Bahalim A, Darmstadt GL, et al. Intrapartum-related neonatal encephalopathy incidence and impairment at regional and global levels for 2010 with trends from 1990. Pediatr Res. (2013) 74 Suppl 1:50–72. doi: 10.1038/pr.2013.206
2. Jacobs SE, Berg M, Hunt R, Tarnow-Mordi WO, Inder TE, Davis PG. Cooling for newborns with hypoxic ischaemic encephalopathy. Cochrane Database Syst Rev. (2013) (1):CD003311. doi: 10.1002/14651858.CD003311.pub3
3. Edwards AD, Brocklehurst P, Gunn AJ, Halliday H, Juszczak E, Levene M, et al. Neurological outcomes at 18 months of age after moderate hypothermia for perinatal hypoxic ischaemic encephalopathy: synthesis and meta-analysis of trial data. BMJ. (2010) 340:c363. doi: 10.1136/bmj.c363
4. Kurinczuk JJ, White-Koning M, Badawi N. Epidemiology of neonatal encephalopathy and hypoxic-ischaemic encephalopathy. Early Hum Dev. (2010) 86:329–38. doi: 10.1016/j.earlhumdev.2010.05.010
5. Aslam S, Strickland T, Molloy EJ. Neonatal encephalopathy: need for recognition of multiple etiologies for optimal management. Front Pediatr. (2019) 7:142. doi: 10.3389/fped.2019.00142
6. Badawi N, Kurinczuk JJ, Keogh JM, Alessandri LM, O'Sullivan F, Burton PR, et al. Intrapartum risk factors for newborn encephalopathy: the Western Australian case-control study. BMJ. (1998) 317:1554–8. doi: 10.1136/bmj.317.7172.1554
7. Impey L, Greenwood C, MacQuillan K, Reynolds M, Sheil O. Fever in labour and neonatal encephalopathy: a prospective cohort study. BJOG. (2001) 108:594–7. doi: 10.1111/j.1471-0528.2001.00145.x
8. Nelson KB, Bingham P, Edwards EM, Horbar JD, Kenny MJ, Inder T, et al. Antecedents of neonatal encephalopathy in the Vermont Oxford Network Encephalopathy Registry. Pediatrics. (2012) 130:878–86. doi: 10.1542/peds.2012-0714
9. Tann CJ, Nakakeeto M, Willey BA, Sewegaba M, Webb EL, Oke I, et al. Perinatal risk factors for neonatal encephalopathy: an unmatched case-control study. Arch Dis Child Fetal Neonatal Ed. (2018) 103:F250–6. doi: 10.1136/archdischild-2017-312744
10. Blume HK, Li CI, Loch CM, Koepsell TD. Intrapartum fever and chorioamnionitis as risks for encephalopathy in term newborns: a case-control study. Dev Med Child Neurol. (2008) 50:19–24. doi: 10.1111/j.1469-8749.2007.02007.x
11. Parker SJ, Kuzniewicz M, Niki H, Wu YW. Antenatal and Intrapartum Risk Factors for Hypoxic-Ischemic Encephalopathy in a US Birth Cohort. J Pediatr. (2018) 203:163–9. doi: 10.1016/j.jpeds.2018.08.028
12. Novak CM, Eke AC, Ozen M, Burd I, Graham EM. Risk Factors for Neonatal Hypoxic-Ischemic Encephalopathy in the Absence of Sentinel Events. Am J Perinatol. (2019) 36:27–33. doi: 10.1055/s-0038-1639356
13. Tann CJ, Nkurunziza P, Nakakeeto M, Oweka J, Kurinczuk JJ, Were J, et al. Prevalence of bloodstream pathogens is higher in neonatal encephalopathy cases vs. controls using a novel panel of real-time PCR assays. PLoS ONE. (2014) 9:e97259. doi: 10.1371/journal.pone.0097259
14. Tann CJ, Martinello KA, Sadoo S, Lawn JE, Seale AC, Vega-Poblete M, et al. Neonatal Encephalopathy With Group B Streptococcal Disease Worldwide: Systematic Review, Investigator Group Datasets, and Meta-analysis. Clin Infect Dis. (2017) 65:S173–89. doi: 10.1093/cid/cix662
15. Pacora P, Chaiworapongsa T, Maymon E, Kim YM, Gomez R, Yoon BH, et al. Funisitis and chorionic vasculitis: the histological counterpart of the fetal inflammatory response syndrome. J Matern Fetal Neonatal Med. (2002) 11:18–25. doi: 10.1080/jmf.11.1.18.25
16. Gisslen T, Singh G, Georgieff MK. Fetal inflammation is associated with persistent systemic and hippocampal inflammation and dysregulation of hippocampal glutamatergic homeostasis. Pediatr Res. (2019) 85:703–10. doi: 10.1038/s41390-019-0330-y
17. Russell NJ, Seale AC, O'Sullivan C, Le Doare K, Heath PT, Lawn JE, et al. Risk of Early-onset neonatal group B Streptococcal disease with maternal colonization worldwide: systematic review and meta-analyses. Clin Infect Dis. (2017) 65:S152–s159. doi: 10.1093/cid/cix655
18. Hagberg H, Mallard C, Ferriero DM, Vannucci SJ, Levison SW, Vexler ZS, et al. The role of inflammation in perinatal brain injury. Nat Rev Neurol. (2015) 11:192–208. doi: 10.1038/nrneurol.2015.13
19. Nelson KB, Penn AA. Is infection a factor in neonatal encephalopathy? Arch Dis Child Fetal Neonatal Ed. (2015) 100:F8–f10. doi: 10.1136/archdischild-2014-306192
20. Fleiss B, Tann CJ, Degos V, Sigaut S, Van Steenwinckel J, Schang AL, et al. Inflammation-induced sensitization of the brain in term infants. Dev Med Child Neurol. (2015) 57 Suppl 3:17–28. doi: 10.1111/dmcn.12723
21. Kendall G, Peebles D. Acute fetal hypoxia: the modulating effect of infection. Early Hum Dev. (2005) 81:27–34. doi: 10.1016/j.earlhumdev.2004.10.012
22. Hagberg H, Gressens P, Mallard C. Inflammation during fetal and neonatal life: implications for neurologic and neuropsychiatric disease in children and adults. Ann Neurol. (2012) 71:444–57. doi: 10.1002/ana.22620
23. Peebles DM, Wyatt JS. Synergy between antenatal exposure to infection and intrapartum events in causation of perinatal brain injury at term. BJOG. (2002) 109:737–9. doi: 10.1111/j.1471-0528.2002.01019.x
24. Eklind S, Mallard C, Arvidsson P, Hagberg H. Lipopolysaccharide induces both a primary and a secondary phase of sensitization in the developing rat brain. Pediatr Res. (2005) 58:112–6. doi: 10.1203/01.PDR.0000163513.03619.8D
25. Eklind S, Mallard C, Leverin AL, Gilland E, Blomgren K, Mattsby-Baltzer I, et al. Bacterial endotoxin sensitizes the immature brain to hypoxic–ischaemic injury. Eur J Neurosci. (2001) 13:1101–6. doi: 10.1046/j.0953-816x.2001.01474.x
26. Froen JF, Amerio G, Stray-Pedersen B, Saugstad OD. Detrimental effects of nicotine and endotoxin in the newborn piglet brain during severe hypoxemia. Biol Neonate. (2002) 82:188–96. doi: 10.1159/000063610
27. Yang L, Sameshima H, Ikeda T, Ikenoue T. Lipopolysaccharide administration enhances hypoxic-ischemic brain damage in newborn rats. J Obstet Gynaecol Res. (2004) 30:142–7. doi: 10.1111/j.1447-0756.2003.00174.x
28. Martinello KA, Meehan C, Avdic-Belltheus A, Lingam I, Ragab S, Hristova M, et al. Acute LPS sensitization and continuous infusion exacerbates hypoxic brain injury in a piglet model of neonatal encephalopathy. Sci Rep. (2019) 9:10184. doi: 10.1038/s41598-019-46488-y
29. Ikeda T, Mishima K, Aoo N, Egashira N, Iwasaki K, Fujiwara M, et al. Combination treatment of neonatal rats with hypoxia-ischemia and endotoxin induces long-lasting memory and learning impairment that is associated with extended cerebral damage. Am J Obstet Gynecol. (2004) 191:2132–41. doi: 10.1016/j.ajog.2004.04.039
30. Mottahedin A, Svedin P, Nair S, Mohn CJ, Wang X, Hagberg H, et al. Systemic activation of Toll-like receptor 2 suppresses mitochondrial respiration and exacerbates hypoxic-ischemic injury in the developing brain. J Cereb Blood Flow Metab. (2017) 37:1192–8. doi: 10.1177/0271678X17691292
31. Stridh L, Mottahedin A, Johansson ME, Valdez RC, Northington F, Wang X, et al. Toll-like receptor-3 activation increases the vulnerability of the neonatal brain to hypoxia-ischemia. J Neurosci. (2013) 33:12041–51. doi: 10.1523/JNEUROSCI.0673-13.2013
32. Osredkar D, Sabir H, Falck M, Wood T, Maes E, Flatebo T, et al. Hypothermia does not reverse cellular responses caused by lipopolysaccharide in neonatal hypoxic-ischaemic brain injury. Dev Neurosci. (2015) 37:390–7. doi: 10.1159/000430860
33. Osredkar D, Thoresen M, Maes E, Flatebo T, Elstad M, Sabir H. Hypothermia is not neuroprotective after infection-sensitized neonatal hypoxic-ischemic brain injury. Resuscitation. (2014) 85:567–72. doi: 10.1016/j.resuscitation.2013.12.006
34. Falck M, Osredkar D, Maes E, Flatebo T, Wood TR, Sabir H, et al. Hypothermic neuronal rescue from infection-sensitised hypoxic-ischaemic brain injury is pathogen dependent. Dev Neurosci. (2017) 39:238–47. doi: 10.1159/000455838
35. Chevin M, Guiraut C, Maurice-Gelinas C, Deslauriers J, Grignon S, Sebire G. Neuroprotective effects of hypothermia in inflammatory-sensitized hypoxic-ischemic encephalopathy. Int J Dev Neurosci. (2016) 55:1–8. doi: 10.1016/j.ijdevneu.2016.09.002
36. Chevin M, Guiraut C, Sebire G. Effect of hypothermia on interleukin-1 receptor antagonist pharmacodynamics in inflammatory-sensitized hypoxic-ischemic encephalopathy of term newborns. J Neuroinflammation. (2018) 15:214. doi: 10.1186/s12974-018-1258-6
37. Martinello KA, Meehan C, Avdic-Belltheus A, Lingam I, Mutshiya T, Yang Q, et al. Hypothermia is not therapeutic in a neonatal piglet model of inflammation-sensitized hypoxia-ischemia. Pediatr Res. (2021) 1–12. doi: 10.1038/s41390-021-01584-6
38. Pauliah SS, Shankaran S, Wade A, Cady EB, Thayyil S. Therapeutic hypothermia for neonatal encephalopathy in low- and middle-income countries: a systematic review and meta-analysis. PLoS ONE. (2013) 8:e58834. doi: 10.1371/journal.pone.0058834
39. Thayyil S, Pant S, Montaldo P, Shukla D, Oliveira V, Ivain P, et al. Hypothermia for moderate or severe neonatal encephalopathy in low-income and middle-income countries (HELIX): a randomised controlled trial in India, Sri Lanka, and Bangladesh. The Lancet Global Health. (2021) 9:e1273–85. doi: 10.1016/S2214-109X(21)00264-3
40. Mourvillier B, Tubach F, van de Beek D, Garot D, Pichon N, Georges H, et al. Induced hypothermia in severe bacterial meningitis: a randomized clinical trial. JAMA. (2013) 310:2174–83. doi: 10.1001/jama.2013.280506
41. Itenov TS, Johansen ME, Bestle M, Thormar K, Hein L, Gyldensted L, et al. Induced hypothermia in patients with septic shock and respiratory failure (CASS): a randomised, controlled, open-label trial. Lancet Respir Med. (2018) 6:183–92. doi: 10.1016/S2213-2600(18)30004-3
42. Liberati A, Altman DG, Tetzlaff J, Mulrow C, Gotzsche PC, Ioannidis JP, et al. The PRISMA statement for reporting systematic reviews and meta-analyses of studies that evaluate health care interventions: explanation and elaboration. J Clin Epidemiol. (2009) 62:e1–34. doi: 10.1016/j.jclinepi.2009.06.006
43. Chien PF, Khan KS, Siassakos D. Registration of systematic reviews: PROSPERO. BJOG. (2012) 119:903–5. doi: 10.1111/j.1471-0528.2011.03242.x
44. Chalak L, Ferriero DM, Gressens P, Molloy E, Bearer C. A 20 years conundrum of neonatal encephalopathy and hypoxic ischemic encephalopathy: are we closer to a consensus guideline? Pediatr Res. (2019) 86:548–9. doi: 10.1038/s41390-019-0547-9
45. Molloy EJ, Bearer C. Neonatal encephalopathy versus hypoxic-ischemic encephalopathy. Pediatr Res. (2018) 84:574. doi: 10.1038/s41390-018-0169-7
46. Molloy EJ, Wynn JL, Bliss J, Koenig JM, Keij FM, McGovern M, et al. Neonatal sepsis: need for consensus definition, collaboration and core outcomes. Pediatr Res. (2020) 88:2–4. doi: 10.1038/s41390-020-0850-5
47. Fjalstad JW, Stensvold HJ, Bergseng H, Simonsen GS, Salvesen B, Ronnestad AE, et al. Early-onset sepsis and antibiotic exposure in term infants: a nationwide population-based study in Norway. Pediatr Infect Dis J. (2016) 35:1–6. doi: 10.1097/INF.0000000000000906
48. Drageset M, Fjalstad JW, Mortensen S, Klingenberg C. Management of early-onset neonatal sepsis differs in the north and south of Scandinavia. Acta Paediatr. (2017) 106:375–81. doi: 10.1111/apa.13698
49. van Laerhoven H, de Haan TR, Offringa M, Post B, van der Lee JH. Prognostic tests in term neonates with hypoxic-ischemic encephalopathy: a systematic review. Pediatrics. (2013) 131:88–98. doi: 10.1542/peds.2012-1297
50. Lally PJ, Montaldo P, Oliveira V, Soe A, Swamy R, Bassett P, et al. Magnetic resonance spectroscopy assessment of brain injury after moderate hypothermia in neonatal encephalopathy: a prospective multicentre cohort study. Lancet Neurol. (2019) 18:35–45. doi: 10.1016/S1474-4422(18)30325-9
51. Ouzzani M, Hammady H, Fedorowicz Z, Elmagarmid A. Rayyan-a web and mobile app for systematic reviews. Syst Rev. (2016) 5:210. doi: 10.1186/s13643-016-0384-4
52. Popay J, Roberts HM, Sowden A, Petticrew M, Arai L, Rodgers M, et al. Guidance on the Conduct of Narrative Synthesis in Systematic Reviews. Lancaster: Institute for Health Research.
53. Higgins JPT, G.S.e. ([updated March 2011]. The Cochrane Collaboration, 2011.). “Cochrane Handbook for Systematic Reviews of Interventions Version 5.1.0”.
54. Ryan R, Hill S. “How to GRADE the quality of the evidence”. La Trobe University, Melbourne: CCCG (2016). Available online at: http://cccrg.cochrane.org/author-resources.
55. Rao R, Lee KS, Zaniletti I, Yanowitz TD, DiGeronimo R, Dizon MLV, et al. Antimicrobial therapy utilization in neonates with hypoxic-ischemic encephalopathy (HIE): a report from the children's hospital neonatal database (CHND). Journal of Perinatology. (2020) 40:70–8. doi: 10.1038/s41372-019-0527-2
56. Hakobyan M, Dijkman KP, Laroche S, Naulaers G, Rijken M, Steiner K, et al. Outcome of infants with therapeutic hypothermia after perinatal asphyxia and early-onset sepsis. Neonatology. (2018) 115:127–33. doi: 10.1159/000493358
57. Frank CM, Nikkels PG, Harteman JC, van Haastert IC, Benders MJ, Koopman-Esseboom C, et al. Placental pathology and outcome after perinatal asphyxia and therapeutic hypothermia. J Perinatol. (2016) 36:977–84. doi: 10.1038/jp.2016.110
58. Orrock JE, Panchapakesan K, Vezina G, Chang T, Harris K, Wang Y, et al. Association of brain injury and neonatal cytokine response during therapeutic hypothermia in newborns with hypoxic-ischemic encephalopathy. Pediatr Res. (2016) 79:742–7. doi: 10.1038/pr.2015.280
59. Mir IN, Johnson-Welch SF, Nelson DB, Brown LS, Rosenfeld CR, Chalak LF. Placental pathology is associated with severity of neonatal encephalopathy and adverse developmental outcomes following hypothermia. Am J Obstet Gynecol. (2015) 213:849.e841–847. doi: 10.1016/j.ajog.2015.09.072
60. Lachapelle J, Chen M, Oskoui M, Ali N, Brown R, Wintermark P. Placental pathology in asphyxiated newborns treated with therapeutic hypothermia. J Neonatal Perinatal Med. (2015) 8:33–40. doi: 10.3233/NPM-15814068
61. Harteman JC, Nikkels PG, Benders MJ, Kwee A, Groenendaal F, de Vries LS. Placental pathology in full-term infants with hypoxic-ischemic neonatal encephalopathy and association with magnetic resonance imaging pattern of brain injury. J Pediatr. (2013) 163:968–95.e962. doi: 10.1016/j.jpeds.2013.06.010
62. Hayes BC, Cooley S, Donnelly J, Doherty E, Grehan A, Madigan C, et al. The placenta in infants >36 weeks gestation with neonatal encephalopathy: a case control study. Arch Dis Child Fetal Neonatal Ed. (2013) 98:F233–239. doi: 10.1136/archdischild-2012-301992
63. Wintermark P, Boyd T, Gregas MC, Labrecque M, Hansen A. Placental pathology in asphyxiated newborns meeting the criteria for therapeutic hypothermia. Am J Obstet Gynecol. (2010) 203:579 e571–579. doi: 10.1016/j.ajog.2010.08.024
64. Groenendaal F, Casaer A, Dijkman KP, Gavilanes AW, de Haan TR, ter Horst HJ, et al. Introduction of hypothermia for neonates with perinatal asphyxia in the Netherlands and Flanders. Neonatology. (2013) 104: 15–21. doi: 10.1159/000348823
65. Barkovich AJ, Hajnal BL, Vigneron D, Sola A, Partridge JC, Allen F, et al. Prediction of neuromotor outcome in perinatal asphyxia: evaluation of MR scoring systems. AJNR Am J Neuroradiol. (1998) 19:143–9.
66. Nelson KB, Grether JK. Potentially asphyxiating conditions and spastic cerebral palsy in infants of normal birth weight. Am J Obstet Gynecol. (1998) 179:507–13. doi: 10.1016/S0002-9378(98)70387-4
67. Jenster M, Bonifacio SL, Ruel T, Rogers EE, Tam EW, Partridge JC, et al. Maternal or neonatal infection: association with neonatal encephalopathy outcomes. Pediatr Res. (2014) 76:93–9. doi: 10.1038/pr.2014.47
68. Scheidegger S, Held U, Grass B, Latal B, Hagmann C, Brotschi B, et al. Association of perinatal risk factors with neurological outcome in neonates with hypoxic ischemic encephalopathy. J Matern Fetal Neonatal Med. (2019) 1–8. doi: 10.1080/14767058.2019.1623196
69. Sarnat HB, Sarnat MS. Neonatal encephalopathy following fetal distress. A clinical and electroencephalographic study Arch Neurol. (1976) 33:696–705. doi: 10.1001/archneur.1976.00500100030012
70. Wang X, Stridh L, Li W, Dean J, Elmgren A, Gan L, et al. Lipopolysaccharide sensitizes neonatal hypoxic-ischemic brain injury in a MyD88-dependent manner. J Immunol. (2009) 183:7471–7. doi: 10.4049/jimmunol.0900762
71. Yang D, Sun YY, Bhaumik SK, Li Y, Baumann JM, Lin X, et al. Blocking lymphocyte trafficking with FTY720 prevents inflammation-sensitized hypoxic-ischemic brain injury in newborns. J Neurosci. (2014) 34:16467–81. doi: 10.1523/JNEUROSCI.2582-14.2014
72. Kendall GS, Hristova M, Horn S, Dafou D, Acosta-Saltos A, Almolda B, et al. TNF gene cluster deletion abolishes lipopolysaccharide-mediated sensitization of the neonatal brain to hypoxic ischemic insult. Lab Invest. (2011) 91:328–41. doi: 10.1038/labinvest.2010.192
73. Yang D, Sun YY, Nemkul N, Baumann JM, Shereen A, Dunn RS, et al. Plasminogen activator inhibitor-1 mitigates brain injury in a rat model of infection-sensitized neonatal hypoxia-ischemia. Cereb Cortex. (2013) 23:1218–29. doi: 10.1093/cercor/bhs115
74. Yang D, Sun YY, Lin X, Baumann JM, Dunn RS, Lindquist DM, et al. Intranasal delivery of cell-penetrating anti-NF-kappaB peptides (Tat-NBD) alleviates infection-sensitized hypoxic-ischemic brain injury. Exp Neurol. (2013) 247:447–55. doi: 10.1016/j.expneurol.2013.01.015
75. Serdar M, Kempe K, Rizazad M, Herz J, Bendix I, Felderhoff-Muser U, et al. Early pro-inflammatory microglia activation after inflammation-sensitized hypoxic-ischemic brain injury in neonatal rats. Front Cell Neurosci. (2019) 13:237. doi: 10.3389/fncel.2019.00237
76. Savard A, Lavoie K, Brochu ME, Grbic D, Lepage M, Gris D, et al. Involvement of neuronal IL-1beta in acquired brain lesions in a rat model of neonatal encephalopathy. J Neuroinflammation. (2013) 10:110. doi: 10.1186/1742-2094-10-110
77. Savard A, Brochu ME, Chevin M, Guiraut C, Grbic D, Sebire G. Neuronal self-injury mediated by IL-1beta and MMP-9 in a cerebral palsy model of severe neonatal encephalopathy induced by immune activation plus hypoxia-ischemia. J Neuroinflammation. (2015) 12:111. doi: 10.1186/s12974-015-0330-8
78. Ikeda T, Yang L, Ikenoue T, Mallard C, Hagberg H. Endotoxin-induced hypoxic-ischemic tolerance is mediated by up-regulation of corticosterone in neonatal rat. Pediatr Res. (2006) 59:56–60. doi: 10.1203/01.pdr.0000191140.87314.ce
79. Harding B, Conception K, Li Y, Zhang L. Glucocorticoids protect neonatal rat brain in model of hypoxic-ischemic encephalopathy (HIE). International Journal of Molecular Sciences. (2017) 18. doi: 10.3390/ijms18010017
80. Girard S, Sebire H, Brochu ME, Briota S, Sarret P, Sebire G. Postnatal administration of IL-1Ra exerts neuroprotective effects following perinatal inflammation and/or hypoxic-ischemic injuries. Brain Behav Immun. (2012) 26:1331–9. doi: 10.1016/j.bbi.2012.09.001
81. Girard S, Larouche A, Kadhim H, Rola-Pleszczynski M, Gobeil F, Sebire G. Lipopolysaccharide and hypoxia/ischemia induced IL-2 expression by microglia in neonatal brain. Neuroreport. (2008) 19:997–1002. doi: 10.1097/WNR.0b013e3283036e88
82. Lehnardt S, Massillon L, Follett P, Jensen FE, Ratan R, Rosenberg PA, et al. Activation of innate immunity in the CNS triggers neurodegeneration through a Toll-like receptor 4-dependent pathway. Proc Natl Acad Sci USA. (2003) 100:8514–9. doi: 10.1073/pnas.1432609100
83. Stigger F, Lovatel G, Marques M, Bertoldi K, Moyses F, Elsner V, et al. Inflammatory response and oxidative stress in developing rat brain and its consequences on motor behavior following maternal administration of LPS and perinatal anoxia. Int J Dev Neurosci. (2013) 31:820–7. doi: 10.1016/j.ijdevneu.2013.10.003
84. Brochu ME, Girard S, Lavoie K, Sebire G. Developmental regulation of the neuroinflammatory responses to LPS and/or hypoxia-ischemia between preterm and term neonates: An experimental study. J Neuroinflammation. (2011) 8:55. doi: 10.1186/1742-2094-8-55
85. Wang X, Svedin P, Nie C, Lapatto R, Zhu C, Gustavsson M, et al. N-acetylcysteine reduces lipopolysaccharide-sensitized hypoxic-ischemic brain injury. Ann Neurol. (2007) 61:263–71. doi: 10.1002/ana.21066
86. Dhillon SK, Gunn AJ, Jung Y, Mathai S, Bennet L, Fraser M. Lipopolysaccharide-induced preconditioning attenuates apoptosis and differentially regulates TLR4 and TLR7 gene expression after ischemia in the preterm ovine fetal brain. Dev Neurosci. (2015) 37:497–514. doi: 10.1159/000433422
87. Lin HY, Huang CC, Chang KF. Lipopolysaccharide preconditioning reduces neuroinflammation against hypoxic ischemia and provides long-term outcome of neuroprotection in neonatal rat. Pediatr Res. (2009) 66:254–9. doi: 10.1203/PDR.0b013e3181b0d336
88. Vexler ZS, Mallard C, Hagberg H. Positive and negative conditioning in the neonatal brain. Cond Med. (2018) 1:279–93.
89. Hassell KJ, Ezzati M, Alonso-Alconada D, Hausenloy DJ, Robertson NJ. New horizons for newborn brain protection: enhancing endogenous neuroprotection. Arch Dis Child Fetal Neonatal Ed. (2015) 100:F541–552. doi: 10.1136/archdischild-2014-306284
90. Thoresen M. Who should we cool after perinatal asphyxia? Semin Fetal Neonatal Med. (2015) 20:66–71. doi: 10.1016/j.siny.2015.01.002
91. Jenkins DD, Lee T, Chiuzan C, Perkel JK, Rollins LG, Wagner CL, et al. Altered circulating leukocytes and their chemokines in a clinical trial of therapeutic hypothermia for neonatal hypoxic ischemic encephalopathy*. Pediatr Crit Care Med. (2013) 14:786–95. doi: 10.1097/PCC.0b013e3182975cc9
92. Chakkarapani E, Davis J, Thoresen M. Therapeutic hypothermia delays the C-reactive protein response and suppresses white blood cell and platelet count in infants with neonatal encephalopathy. Arch Dis Child Fetal Neonatal Ed. (2014) 99:F458–463. doi: 10.1136/archdischild-2013-305763
93. Martinello KA, Meehan C, Avdic-Belltheus A, Lingam I, Mutshiya T, Yang Q, et al. Hypothermia is not therapeutic in a piglet model of LPS sensitised neonatal encephalopathy. J Paediatr Child Health. (2019) 55:33–33. doi: 10.1111/jpc.14409_82
94. Falck M, Osredkar D, Maes E, Flatebo T, Wood TR, Walloe L, et al. Hypothermia Is Neuroprotective after Severe Hypoxic-Ischaemic Brain Injury in Neonatal Rats Pre-Exposed to PAM3CSK4. Dev Neurosci. (2018) 40:189–97. doi: 10.1159/000487798
95. Miller SP, Ramaswamy V, Michelson D, Barkovich AJ, Holshouser B, Wycliffe N, et al. Patterns of brain injury in term neonatal encephalopathy. J Pediatr. (2005) 146:453–60. doi: 10.1016/j.jpeds.2004.12.026
96. Shankaran S, McDonald SA, Laptook AR, Hintz SR, Barnes PD, Das A, et al. Neonatal magnetic resonance imaging pattern of brain injury as a biomarker of childhood outcomes following a trial of hypothermia for neonatal hypoxic-ischemic encephalopathy. J Pediatr. (2015) 167:987–993.e983. doi: 10.1016/j.jpeds.2015.08.013
Keywords: neonatal encephalopathy, hypoxic-ischemic encephalopathy, infection, therapeutic hypothermia, neurodevelopment
Citation: Andersen M, Pedersen MV, Andelius TCK, Kyng KJ and Henriksen TB (2021) Neurological Outcome Following Newborn Encephalopathy With and Without Perinatal Infection: A Systematic Review. Front. Pediatr. 9:787804. doi: 10.3389/fped.2021.787804
Received: 01 October 2021; Accepted: 25 November 2021;
Published: 20 December 2021.
Edited by:
Anna Maria Lavezzi, University of Milan, ItalyReviewed by:
Ulrike Mietzsch, University of Washington, United StatesRiffat Mehboob, King Edward Medical University, Pakistan
Copyright © 2021 Andersen, Pedersen, Andelius, Kyng and Henriksen. This is an open-access article distributed under the terms of the Creative Commons Attribution License (CC BY). The use, distribution or reproduction in other forums is permitted, provided the original author(s) and the copyright owner(s) are credited and that the original publication in this journal is cited, in accordance with accepted academic practice. No use, distribution or reproduction is permitted which does not comply with these terms.
*Correspondence: Mads Andersen, Mads.Andersen@clin.au.dk