- 1Department of Pediatrics, The Affiliated Suqian First People's Hospital of Nanjing Medical University, Suqian, China
- 2Department of Neonatology, Qinghai University Affiliated Hospital, Xining, China
- 3Department of Obstetrics and Gynecology, Qinghai University Affiliated Hospital, Xining, China
- 4Department of Neonatology, The Affiliated Obstetrics and Gynecology Hospital of Nanjing Medical University, Nanjing, China
Introduction: The reference interval for pulse oxygen saturation (SpO2) in neonates born at high altitudes has not been defined to date. The purpose of this study was to systematically review published studies and determine the reference interval of SpO2 in neonates at different altitudes.
Methods: Databases of PubMed, Embase, Cochrane Library, Clinicaltrials.Gov, Chinese National Knowledge Infrastructure Database, Wanfang Database, Chinese Science Technology Journals Database, and Chinese Clinical Trial Registry were searched for studies reporting SpO2 in healthy neonates at different altitudes. Retrieval time was from inception of the database to August 16, 2021. The Agency for Healthcare Research and Quality checklist was used to evaluate the quality of studies. Python v3.8 was used to analyze the data. This systematic review was drafted in accordance with the guidelines of the Preferred Reporting Items for Systematic Reviews and Meta-Analyses (PRISMA) statement.
Results: Seven cross-sectional studies, published between 1991 and 2020, were identified. They were from US, Mexico, Israel, Ecuador, and China. Three studies were rated as high quality and four as moderate quality. The mean SpO2 (with standard deviation or standard error) of neonates born in 40 different altitudes (ranging from 25 meters to 3,100 meters) were obtained. The prediction equation for calculation of the lower limit of the reference interval was established, and the reference intervals for SpO2 at different altitudes were determined.
Conclusions: In healthy neonates, the lower limit of the reference interval of SpO2 decreases with increase in altitude. High-quality prospective studies are need to confirm our findings.
Introduction
Knowledge of the reference interval of pulse oxygen saturation (SpO2) in neonates is crucial for accurate identification of neonatal hypoxemia (1). At sea level and adjacent altitudes, 2.5% of neonates have SpO2 below 95%, and so this value is used as the cutoff for identifying hypoxemia at these altitudes (2). Establishment of such reference values is important for standardized clinical management (3). However, at present, there is no recommended reference interval for SpO2 in high altitude areas. The standard recommended for the plains is not applicable because atmospheric pressure and partial pressure of oxygen decrease as altitude increases and will therefore cause decrease in SpO2 (4). Guo et al. (5) suggested that the value corresponding to the 2.5th percentile of the SpO2 distribution range in healthy neonates born at different altitudes be used as the cutoff value. Unfortunately, there are not many studies on the SpO2 distribution range in healthy neonates born at different altitudes, especially during the period from 24 h after birth to discharge, which is defined as the stable period after birth. No systematic reviews of published studies have been performed.
The purpose of this study was to review the published data and establish the reference interval for SpO2 in the stable period of healthy neonates at different altitudes. The findings of this study will allow evidence-based medical decision making in high altitude areas.
Methods
This systematic review of all studies on SpO2 in healthy neonates at different altitudes during the stable period was reported according to Preferred Reporting Items for Systematic Reviews and Meta-Analyses (PRISMA) guidelines (6).
Data Sources and Literature Search
To identify articles on SpO2 in neonates in the stable period after birth, we performed a literature search of the databases of PubMed, Embase, Cochrane Library, Clinicaltrials.Gov, Chinese National Knowledge Infrastructure Database (CNKI), Wanfang Database, Chinese Science Technology Journals Database (VIP), and Chinese Clinical Trial Registry (ChiCTR). Papers in any language, published from inception of the database to August 16, 2021, were eligible for consideration. The search terms were “altitude,” “neonate,” and “oximetry,” in combination with Medical Subject Headings (MeSH) terms and other equivalent terms; logical symbols, wildcards, and range operators were used to compile search formulas. The search strategy used in PubMed is described in online Supplementary Material 1.
Inclusion and Exclusion Criteria
Articles were eligible for inclusion in this review if (1) the study participants were healthy asymptomatic neonates born at a specified altitude; (2) a pulseoximetry (POX) was used to measure SpO2 on the right hand (pre-ductal) and/or either foot (post-ductal); (3) measurement was made during the stable period, i.e., 24 h after birth to before discharge; and (4) measurement was made while the neonate was inhaling room air (i.e., no oxygen supplementation). Studies were excluded if (1) they did not mention the measurement site (pre-ductal or post-ductal); (2) measurement was made within 24 h of birth; (3) data could not be extracted; (4) gestational age was <34 weeks; or (5) altitude was not specified.
Data Collection and Extraction
Two researchers independently conducted the literature search and extracted and cross-checked the data. Disagreements were settled by discussion and, if necessary, by consultation with the third researcher. Duplicate articles were first eliminated. Then, the researchers read the titles and abstracts of all articles and discarded obviously irrelevant papers. Finally, the full text of the remaining papers were read, and studies that satisfied all eligibility criteria were selected.
The following data were extracted from the selected articles: (1) basic information regarding the study, i.e., name of first author, publication date, study period, study location and altitude, sample size; (2) baseline characteristics of the study participants and the methods used, i.e., gestational age, POX type, measurement site (hand, foot), time of measurement, resting state at the time of measurement, and summary statistics. If multiple SpO2 measurements had been made during the stable period, only the values recorded closest to the time of birth was extracted. Similarly, if measurements had been made during different neonatal resting states (e.g., awake but quiet, light sleep, deep sleep, and so on), only the values recorded in the awake but quiet state was extracted.
Quality Assessment
Study quality was assessed using the checklist of the Agency for Healthcare Research and Quality (AHRQ) (7). This checklist comprises 11 questions that assess different aspects of the study such as definition of information sources, inclusion and exclusion criteria, the time period and continuity of identifying patients, blinding of personnel, quality assurance, confounding and missing data, patient response rates and completeness of response. The response to each question may be “yes,” “no,” or “unclear.” A score of 1 point is assigned for “yes” and 0 points for “no” or “unclear.” Thus, the total score can range from 0 to 11, with 8–11 indicating high quality, 4–7 indicating moderate quality, and 0–3 indicating low quality. In case of discordance in scoring, the third researcher's decision was final.
Statistical Analysis
The mean ± standard deviation (SD) of SpO2, or the 2.5th percentile value of the SpO2 distribution range, were recorded. If the study results were expressed as the mean value ± standard error (SE), the SD was derived using the formula: (where n is the sample size). If the data showed a skewed distribution, the lower limit of the reference interval was the value corresponding to the 2.5th percentile in the distribution range of SpO2 of healthy neonates born at different altitudes. If the data were normally distributed, the lower limit value corresponds to the −2SD value (mean value-2*SD). Therefore, the reference interval of SpO2 was defined as: the lower limit value < SpO2 ≤ 100%.
All data were entered into Microsoft Excel. Python v3.8 was used to read the data in Microsoft Excel for analysis. Then, the curve-fitting function in the numerical calculation library was used to perform polynomial fitting on the lower limit values. Next, the altitude data were taken as the parameters of the fitted function, and the predictive equations were obtained for the lower limit values.
Results
Study Selection
A total of 376 articles were initially retrieved. From these, 116 duplicate articles and 213 obviously irrelevant articles were first screened out. Of the remaining 47 articles, seven satisfied all eligibility criteria and were included in this systematic review (5, 8–13). Figure 1 shows the literature screening process.
Study Characteristics
All seven articles were cross-sectional studies: three were from the US, one from Mexico, one from Israel, one from Ecuador, and one from China. One study was in Spanish and the rest were in English. The sample sizes ranged from 15 to 41,097, and the altitudes ranged from 25 to 3,100 meters above sea level. Four studies did not specify the study period. Three studies did not specify the neonatal resting state during measurement. Table 1 summarizes the characteristics of the seven studies.
Quality Assessment
By the AHRQ scores, three studies were graded as high quality and four as moderate quality (Table 2).
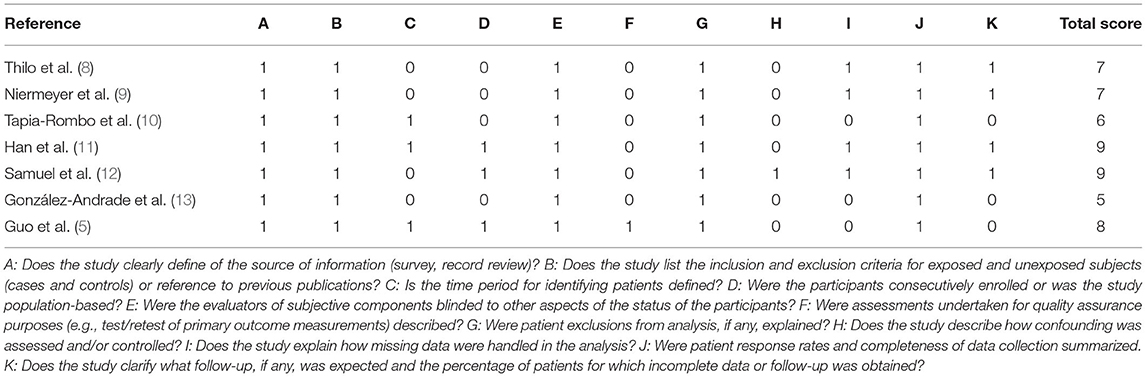
Table 2. Quality assessment of studies by the Agency for Healthcare Research and Quality (AHRQ) scoring system.
Data Extraction
The mean (with SD or SE) SpO2 of healthy neonates born at 40 different altitudes were collected from the seven studies. Of these, three studies had only post-ductal data. Table 3 presents all SpO2 data expressed as means ± SD.
Data Analysis
Because the altitude varied between the studies, meta-analysis could not be performed. The pre-ductal and post-ductal data were analyzed separately. The altitude, mean (±SD) SpO2, and sample size were entered into Microsoft Excel, and the −2SD value of SpO2 for each altitude was calculated. The “xlrd” module of Python v3.8 was used to read the altitude column data, mean column data, and −2SD column data in the Microsoft Excel file; this was stored in the “list” collection. Then the curve-fitting function “polyfit” in the “numpy” numerical calculation library was used to perform polynomial fitting based on the least squares method on the −2SD column data. The “polyfit” function has three required parameters, namely abscissa data, ordinate data, and polynomial order, and its return value is the fitting function. After comparison, a third-order polynomial was selected as the fitting function. Then the “list” set of altitude data was used as the parameter of the fitting function, and the prediction equations for the lower limits for pre-ductal and post-ductal SpO2 were obtained:
For pre-ductal SpO2:
For post-ductal SpO2:
where H stands for altitude, in meters.
After the lower limits for pre-ductal and post-ductal SpO2 at each altitude was calculated, the smallest integer of the two values was selected as the final lower limit of SpO2 at each altitude. For example, at altitude 2,500 meters, the lower limit values of pre-ductal and post-ductal SpO2 were 89.3 and 89.25%, respectively, and so the reference interval was: 89% < SpO2 ≤ 100%.
Finally, the “pyplot” function in the visualization library “Matplotlib” was used to draw a −2SD scatter plot and a fitting curve of the lower limit values. For comparison, a scatter plot of the mean values and a fitting curve of the mean values was also drawn using the same method (Figure 2). Mean values scatter plot in Figure 2 is drawn from collected data, and mean values curve is obtained by curve fitting the collected mean values. The scatter plot of −2SD values in Figure 2 is calculated and drawn from the collected data, and lower limit values curve is obtained by curve fitting the −2SD values. As is clear from Figure 2, both pre-ductal and post-ductal SpO2 show a clear downward trend with increasing altitude.
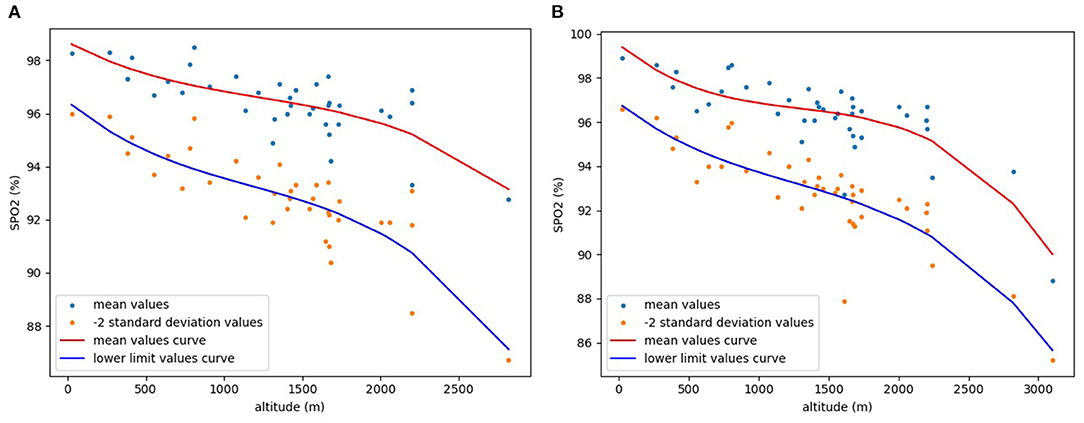
Figure 2. Relationship between altitude and mean and lower limit values of SpO2 in healthy neonates. (A) pre-ductal; (B) post-ductal.
Discussion
This study aimed to determine the reference intervals for neonatal SpO2 at different altitudes. The results showed that the lower limit of the reference interval decreases as altitude increases. It is clear that the reference interval used in the plains is inappropriate for higher altitudes.
Physiologically, the most significant changes in the neonatal period are in the path of intracardiac blood flow and pulmonary circulation. Immediately after birth, the lungs change from being fluid filled to air filled. Pulmonary blood flow increases, and the foramen ovale and ductus arteriosus close, preventing shunting of blood. The respiratory system must respond to a rapid increase in metabolic rate and a gradual change in partial pressure of oxygen from fetal to postnatal period, while continuing the final steps of structural maturation of the alveolar gas exchange unit. Oxygen plays a crucial role in regulating this sequence of events (14).
At high altitudes, atmospheric pressure is lower. This means that air in a given space expands and, although the oxygen proportion remains the same, the molecules are more dispersed. In the lungs, the number of oxygen molecules in alveoli are reduced, and so there is decreased transfer of oxygen from the alveoli to the bloodstream (15). This is the reason for the fall in SpO2 with increase in altitude.
In this review, we only included studies reporting SpO2 levels during the period from 24 h after birth to before discharge. Studies reporting SpO2 within 24 h of birth were excluded because SpO2 levels fluctuate during this period (16). In a study conducted at sea level on healthy neonates, Toth et al. found that the mean post-ductal SpO2 was 67% at 2 min after birth, but rose gradually over 14 min to reach 95% (17). Many studies (18–20) on the use of SpO2 to screen for congenital heart disease have reported significantly higher false-positive rate when screening is performed within 24 h of birth than when it is performed after 24 h of birth; this too suggests that SpO2 levels are unstable during the first 24 h after birth, a period when most of the transition from fetal circulation to neonatal circulation is occurring.
In this study, we excluded preterm infants with gestational age <34 weeks; only full-term or late preterm infants were included. Ravert et al. (21) compared SpO2 in term infants and premature infants born at altitudes of 1,371–2,484 meters, and found that preterm infants tend to have higher SpO2; however, the authors did not specify the exact gestational age. But, multiple studies (16, 22) on healthy late preterm infants and term infants showed that there was no difference in SpO2 between them after birth.
There may be differences between pre-ductal and post-ductal SpO2 of neonates at different altitudes. Habib et al. studied healthy neonates born at an altitude of 1,640 meters and found significant difference between pre-ductal and post-ductal SpO2 levels in the first 20 min of life (23). A multicenter study from Yunnan, China, also reported significant differences between pre-ductal and post-ductal SpO2 levels in healthy neonates during the stable period (i.e., 24 h after birth to before discharge) (5). During screening of neonates for conditions such as congenital heart disease, it is necessary to determine both pre-ductal and post-ductal SpO2 (24). We therefore collected the data for both pre-ductal and post-ductal SpO2, and built separate prediction equations. Currently, there is no high-quality evidence to indicate whether the larger or smaller integer should be chosen as the lower limit of the reference interval. To minimize the possibility misdiagnosis of hypoxemia in healthy neonates, we chose the smaller integer as the lower limit. Incidentally, the difference between values was not large.
Whenever possible, we preferred to record SpO2 values measured in the awake but quiet state. SpO2 during sleep can be low due to insufficient ventilation. It is not certain if there is a difference in SpO2 between sleeping and awake states at high altitudes. In a study of neonates born at altitudes of 1,371–2,484 meters, Ravert et al. found no significant differences in SpO2 values measured at different resting states (21). However, a study on infants aged 1–4 months living at 3,200 meters altitude did find differences in SpO2 recorded during awake vs. sleeping states (25). Whether the contradictory results are due to differences in altitude or differences in the ages of the study participants is unclear.
The prediction equation obtained in this systematic review can help guide medical decision making in high altitude areas by sensitively identifying hypoxemia in infants with conditions such as pneumonia, sepsis, and congenital heart disease. In a study conducted in Albuquerque, New Mexico, at an altitude of 1,646 meters, Rao et al. used SpO2 of 95% as the threshold to screen for congenital heart disease, and reported a false positive rate of 1.5%, which was much higher than the rate at sea level (26). According to our prediction equation, the lower limit of the reference interval of SpO2 at an altitude of 1,646 meters is 92%. However, the sensitivity of using 92% as the threshold needs further study. Previously proposed prediction equations to obtain the SpO2 threshold for diagnosis of hypoxemia in children were based on meta-regression analysis (3); we believe that the curve-fitting method we used is more accurate.
Our study has many limitations. In theory, when the data follow a normal distribution, −2SD corresponds to the 2.5th percentile. All studies in this systematic review provided the mean ± standard deviation or standard error. However, we found that many studies had +2SD values (mean values + 2*SD) exceeding the theoretical maximum of 100%, suggesting a skewed distribution. Ideally, the results of these studies should have been described using the median and interquartile range, and gave the 2.5th percentile value. We used the curve-fitting method to fit the data, but the accuracy of this approach needs to be verified. Another limitation was that the highest altitude in this study was only 3,100 meters; moreover, for three altitudes, pre-ductal data were not available. The accuracy of the prediction equation for higher altitudes needs to be verified. A third limitation was that factors such as season, ethnicity, POX type, and neonatal resting state during measurement varied between the studies; this may have affected the results. A study from Lhasa, Tibet, found that Tibetan infants had higher SpO2 than Han infants at birth and during the first 4 months (27); obviously, altitude is not the only factor affecting SpO2 in neonates.
Conclusions
This is the first systematic review of SpO2 in neonates at different altitudes during the stable period. The lower limit of the reference interval of SpO2 in healthy neonates tends to decrease as altitude increases. Further research on this topic is needed. Future studies should take into account all factors that could influence SpO2 levels, and if the research results are skewed distribution, specific values of the 2.5th percentile in the studies population can be given so as to have a higher quality meta-analysis and systematic review.
Data Availability Statement
The raw data supporting the conclusions of this article will be made available by the authors, without undue reservation.
Author Contributions
BW, JZ, and Z-BY designed this work. BW, Y-ZW, and Z-HL extracted the data. BW, JZ, NW, and Z-BY analyzed the data. BW wrote the manuscript. JZ and Z-BY supervised the work. All authors contributed to the article and approved the submitted version.
Conflict of Interest
The authors declare that the research was conducted in the absence of any commercial or financial relationships that could be construed as a potential conflict of interest.
Publisher's Note
All claims expressed in this article are solely those of the authors and do not necessarily represent those of their affiliated organizations, or those of the publisher, the editors and the reviewers. Any product that may be evaluated in this article, or claim that may be made by its manufacturer, is not guaranteed or endorsed by the publisher.
Acknowledgments
We would like to thank XiaoXiang Han from the School of Medical Instrument and Food Engineering, University of Shanghai for Science and Technology for guidance on the statistical methods used in our study.
Supplementary Material
The Supplementary Material for this article can be found online at: https://www.frontiersin.org/articles/10.3389/fped.2021.771750/full#supplementary-material
References
1. Tekleab AM, Sewnet YC. Role of pulse oximetry in detecting critical congenital heart disease among newborns delivered at a high altitude setting in Ethiopia. Pediatric Health Med Ther. (2019) 10:83–8. doi: 10.2147/PHMT.S217987
2. Hoffman JIE. Is pulse oximetry useful for screening neonates for critical congenital heart disease at high altitudes? Pediatr Cardiol. (2016) 37:812–7. doi: 10.1007/s00246-016-1371-1
3. Subhi R, Smith K, Duke T. When should oxygen be given to children at high altitude? A systematic review to define altitude-specific hypoxaemia. Arch Dis Child. (2009) 94:6–10. doi: 10.1136/adc.2008.138362
4. Tian YP, Hu XJ, Ma XJ, Gu Q, Ge XL, Yang M, et al. [The distribution and variance of neonatal pulse oxygen saturation at different altitudes]. Zhonghua Yi Xue Za Zhi. (2021) 101:1410–4. doi: 10.3760/cma.j.cn112137-20200831-02504
5. Guo F, Tang S, Guo T, Bartell S, Detrano R. Revised threshold values for neonatal oxygen saturation at mild and moderate altitudes. Acta Paediatr. (2020) 109:321–6. doi: 10.1111/apa.14962
6. Moher D, Liberati A, Tetzlaff J, Altman DG. Preferred reporting items for systematic reviews and meta-analyses: the PRISMA statement. Ann Intern Med. (2009) 151:264–9. doi: 10.7326/0003-4819-151-4-200908180-00135
7. Rostom A, Dubé C, Cranney A, Saloojee N, Sy R, Garritty C, et al. Celiac Disease. Evidence Reports/Technology Assessments, No. 104. Appendix D. Quality Assessment Forms. Rockville MD: Agency for Healthcare Research and Quality (US) (2004).
8. Thilo EH, Park-Moore B, Berman ER, Carson BS. Oxygen saturation by pulse oximetry in healthy infants at an altitude of 1610 m (5280 ft). What is normal? Am J Dis Child. (1991) 145:1137–40. doi: 10.1001/archpedi.1991.02160100069025
9. Niermeyer S, Shaffer EM, Thilo E, Corbin C, Moore LG. Arterial oxygenation and pulmonary arterial pressure in healthy neonates and infants at high altitude. J Pediatr. (1993) 123:767–72. doi: 10.1016/S0022-3476(05)80857-1
10. Tapia-Rombo CA, Rosales-Cervantes MGI, Saucedo-Zavala VJ, Ballesteros-del Olmo JC, Sánchez-García L, Santos-Vera I. [Oxygen peripheral saturation using pulse oximetry among healthy term newborns at Mexico's altitude (2240 m)]. Gac Med Mex. (2008) 144:207–12.
11. Han LM, Klewer SE, Blank KM, Seckeler MD, Barber BJ. Feasibility of pulse oximetry screening for critical congenital heart disease at 2643-foot elevation. Pediatr Cardiol. (2013) 34:1803–7. doi: 10.1007/s00246-013-0716-2
12. Samuel TY, Bromiker R, Mimouni FB, Picard E, Lahav S, Mandel D, et al. Newborn oxygen saturation at mild altitude versus sea level: implications for neonatal screening for critical congenital heart disease. Acta paediatrica. (2013) 102:379–84. doi: 10.1111/apa.12155
13. González-Andrade F, Echeverría D, López V, Arellano M. Is pulse oximetry helpful for the early detection of critical congenital heart disease at high altitude? Congenit Heart Dis. (2018) 13:911–8. doi: 10.1111/chd.12654
14. Niermeyer S. Cardiopulmonary transition in the high altitude infant. High Alt Med Biol. (2003) 4:225–39. doi: 10.1089/152702903322022820
15. Schierholz E. Flight physiology: science of air travel with neonatal transport con-siderations. Adv Neonatal Care. (2020) 10:196–9. doi: 10.1097/ANC.0b013e3181e94709
16. Morgan MC, Maina B, Waiyego M, Mutinda C, Aluvaala J, Maina M, et al. Oxygen saturation ranges for healthy newborns within 24 hours at 1800 m. Arch Dis Child Fetal Neonatal Ed. (2017) 102:F266–8. doi: 10.1136/archdischild-2016-311813
17. Toth B, Becker A, Seelbach-Gobel B. Oxygen saturation in healthy newborn infants immediately after birth measured by pulse oximetry. Arch Gynecol Obstet. (2002) 266:105–7. doi: 10.1007/s00404-001-0272-5
18. Matsumori A, Furukawa Y, Hasegawa K, Sato Y, Nakagawa H, Morikawa Y, et al. Epidemiologic and clinical characteristics of cardiomyopathies in Japan: results from nationwide surveys. Circ J. (2002) 66: 323–36. doi: 10.1253/circj.66.323
19. Nugent AW, Daubeney PEF, Chondros P, Carlin JB, Cheung M, Wilkinson LC, et al. The epidemiology of childhood cardiomyopathy in Australia. N Engl J Med. (2003) 348: 1639–46. doi: 10.1056/NEJMoa021737
20. Oh JH, Hong YM, Choi JY, Kim SJ, Jung JW, Sohn S, et al. Idiopathic cardiomyopathies in Korean children. ~ 9 ~Year Korean Multicenter Study. Circ J. (2011) 75: 2228–34. doi: 10.1253/circj.cj-11-0051
21. Ravert P, Detwiler TL, Dickinson JK. Mean oxygen saturation in well neonates at altitudes between 4498 and 8150 feet. Adv Neonatal Care. (2011) 11:412–7. doi: 10.1097/ANC.0b013e3182389348
22. Ezeanosike O, Oguonu T, Ibeziako N, Ibe B. Oxygen saturation reference value by percutaneous pulse oximetry in asymptomatic newborn babies in Nigeria: a cross-sectional study. Int J Neonatal Screen. (2016) 2:6. doi: 10.3390/ijns2030006
23. Habib HS. Oxygen saturation trends in the first hour of life in healthy full-term neonates born at moderate altitude. Pak J Med Sci. (2013) 29:903–6. doi: 10.12669/pjms.294.3848
24. Kemper AR, Mahle WT, Martin GR, Cooley WC, Kumar P, Morrow WR, et al. Strategies for implementing screening for critical congenital heart disease. Pediatrics. (2011) 128: e1259–67. doi: 10.1542/peds.2011-1317
25. Ucrós S, Granados C, Parejo K, Ortega F, Guillén F, Restrepo S, et al. Oxygen saturation, periodic breathing, and sleep apnea in infants aged 1–4 months old living at 3200 meters above sea level. Arch Argent Pediatr. (2017) 115:54–7. doi: 10.5546/aap.2017.eng.54
26. Rao S, Goens MB, Myers OB, Sebesta EA. Pulse oximetry screening for detection of congenital heart defects at 1646 m in Albuquerque, New Mexico. Cardiol Young. (2020) 30:1851–5. doi: 10.1017/S1047951120002899
Keywords: altitude, pulse oxygen saturation, SpO2, reference interval, predictive equations, systematic review, neonates
Citation: Wang B, Zhang J, Wu Y-Z, Lu Z-H, Wang N and Yu Z-B (2021) Reference Interval for Pulse Oxygen Saturation in Neonates at Different Altitudes: A Systematic Review. Front. Pediatr. 9:771750. doi: 10.3389/fped.2021.771750
Received: 07 September 2021; Accepted: 11 October 2021;
Published: 01 November 2021.
Edited by:
Maximo Vento, La Fe Hospital, SpainCopyright © 2021 Wang, Zhang, Wu, Lu, Wang and Yu. This is an open-access article distributed under the terms of the Creative Commons Attribution License (CC BY). The use, distribution or reproduction in other forums is permitted, provided the original author(s) and the copyright owner(s) are credited and that the original publication in this journal is cited, in accordance with accepted academic practice. No use, distribution or reproduction is permitted which does not comply with these terms.
*Correspondence: Zhang-Bin Yu, emhhbmdiaW55dSYjeDAwMDQwO25qbXUuZWR1LmNu
†These authors have contributed equally to this work and share first authorship