- 1Division of Neurology, Children's Hospital of Philadelphia, Philadelphia, PA, United States
- 2Division of Child Neurology, Lucile Packard Children's Hospital at Stanford, Palo Alto, CA, United States
Continuous EEG (cEEG) is a fundamental neurodiagnostic tool in the care of critically ill neonates and is increasingly recommended. cEEG enhances prognostication via assessment of the background brain activity, plays a role in predicting which neonates are at risk for seizures when combined with clinical factors, and allows for accurate diagnosis and management of neonatal seizures. Continuous EEG is the gold standard method for diagnosis of neonatal seizures and should be used for detection of seizures in high-risk clinical conditions, differential diagnosis of paroxysmal events, and assessment of response to treatment. High costs associated with cEEG are a limiting factor in its widespread implementation. Centralized remote cEEG interpretation, automated seizure detection, and pre-natal EEG are potential future applications of this neurodiagnostic tool.
Introduction
Continuous electroencephalography (cEEG) is full-array EEG recording, typically using a minimum of eight electrodes, performed over an extended period to non-invasively assess brain function. It is one of the most widely used forms of neuromonitoring in newborns. By providing real-time information about brain function, including information about spatial localization of brain activity, cEEG can offer rich detail. Furthermore, cEEG is the gold standard method for the diagnosis of seizures in the neonatal period and is widely used for this. The current use of cEEG in the NICU can be understood within the context of its application for background assessment, seizure detection, and how it might be employed in combination with other modalities. There is increasing evidence for the benefits of cEEG in the NICU, with future directions to hone utility.
Guidelines for Use of CEEG in Neonates
While cEEG previously had been limited to use in only highly specialized centers, it is increasingly advised as standard care for all NICUs. World Health Organization (WHO) Guidelines advise that all suspected neonatal seizures should be confirmed by EEG where available (1). This is because neonatal seizures are notoriously difficult to diagnosis reliably through clinical observation alone; EEG is required to confirm diagnosis before initiating pharmacologic treatment. Full-array EEG is the most accurate method for confirming that a clinically suspicious event is epileptic in origin, and the risk of performing EEG on a neonate is minimal (1). In most settings, that EEG will be cEEG, as prolonged recording greatly increases the diagnostic yield for paroxysmal events. In addition to the WHO endorsement of EEG for seizure diagnosis, video cEEG has been particularly recognized as useful. In the updated Neonatal Seizure Classification by the International League Against Epilepsy (ILAE), video cEEG is an integral part of the framework for the diagnosis of neonatal seizures; EEG recording is the first step in diagnosis of seizures in a critically ill neonate at risk of or with clinical suspicion for seizures (2).
Similarly, the American Clinical Neurophysiology Society (ACNS) guidelines recommend the use of cEEG to determine whether paroxysmal events in neonates are seizures (3). In addition, the ACNS guidelines specify that cEEG should be used to detect seizures in newborns who are at high risk for having seizures, including those with known or at high risk for acute brain injury (See Table 1) (3). In cases where neonates with treatment refractory seizures are intentionally placed in burst suppression with medications, EEG should be used to monitor the suppression of the EEG and to detect seizure recurrence (3). Beyond seizure detection, EEG is further recommended to assess for background abnormalities in neonatal encephalopathy as a prognostic tool (3). Recommended duration of monitoring is outlined by EEG indication in the ACNS guidelines: EEG background assessment requires a minimum of 1 h of recording to allow analysis of sleep-wake cycling, neonates at high risk for seizures should have 24 h of cEEG monitoring, and neonates with confirmed seizures should have cEEG until they have been seizure-free for at least 24 h (3).
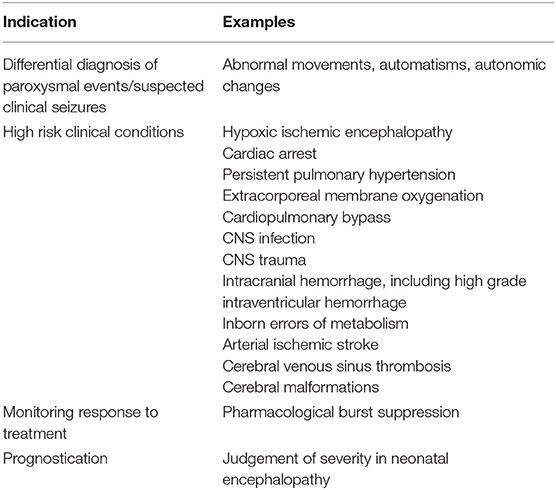
Table 1. Indications for cEEG monitoring in neonates [adapted from Shellhaas, et al. (3)].
Across each of these guidelines, there is consistent endorsement of cEEG use in neonates. At a minimum this should be to confirm diagnosis when seizures are suspected clinically; however, there are several recommended uses beyond seizure confirmation.
Continuous EEG for Background Assessment
EEG monitoring allows for assessment of background brain activity in neonates as a reflection of brain health or injury. There are expected normal EEG backgrounds for awake and asleep states in neonates; this pattern matures with increasing gestational age (see Figures 1–3) with the criteria for normal discontinuity varying with gestational age (see Figures 1, 3) until reaching the expected continuous mixed activity seen at term equivalent age during wakefulness (see Figure 2) (4). There are named features called “graphoelements” which similarly indicate normal brain maturation and function when present and can indicate abnormality when absent or when not consistent with the neonate's conceptional age (4). Encoche frontales, which are normal frontal sharp transients, are an example of a normal neonatal graphoelement (see Figures 2, 4). When the EEG background is not as expected for age, it can indicate dysmaturity, acute injury, or other underlying abnormality. Commonly observed abnormal patterns include excess discontinuity, which describes a pattern with low-amplitude activity (<25 microvolts) sustained for longer than expected for age (see Figure 4). Burst suppression is a particularly worrisome pattern; this describes an invariant, extremely low voltage recording interrupted only by high amplitude bursts of activity, lacking any normal features. An entirely suppressed (<10 μV) and featureless tracing is another severely abnormal and concerning background pattern (see Figure 5). The term electrocerebral inactivity, formerly electrocerebral silence, is a term that can be applied only when specific technical requirements are met, which are different from the requirements for a standard neonatal cEEG recording (4). EEG background is typically symmetric; focal or hemispheric abnormalities should raise suspicion for a focal brain lesion (see Figure 6). In all cases, EEG background abnormalities can be useful for reflecting brain function at a single point in time. cEEG monitoring confers the additional advantage of revealing the trajectory of brain function over time, which may be more important. For example, in neonatal encephalopathy, it is common for the EEG background to be excessively discontinuous in the first hours after birth. However, early recovery, with improvement of discontinuity and gradual return to a normal background is associated with good prognosis. In contrast, an EEG which shows worsening discontinuity reflects an overall worsening of condition. In this way, cEEG for background assessment provides information about brain function at the current time, but also about how brain function is changing.
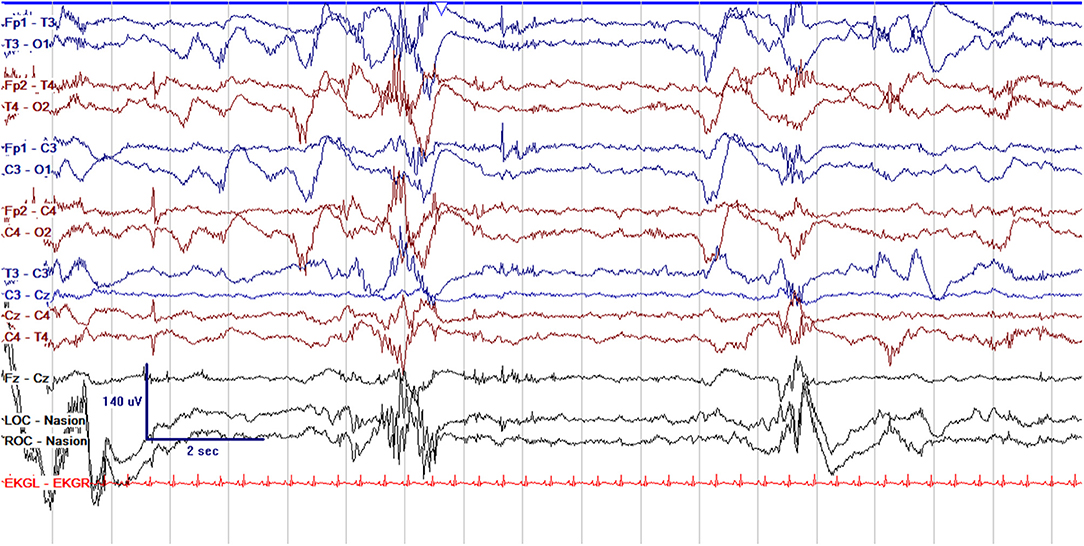
Figure 1. Normal cEEG in a preterm neonate, a 2 day old former 29 + 3 week gestational age (GA) [29 + 5 week post-menstrual age (PMA)] neonate with normal discontinuity for gestational age. In neonates with GA < 30 weeks, interburst intervals can be up to 35 s and <25 μV. In GA 30–33 weeks, interburst intervals can be a maximum of 20 s with voltage <25 μV. In 34–36 week GA neonates, interburst intervals can be up to 10 s and ~25 μV.
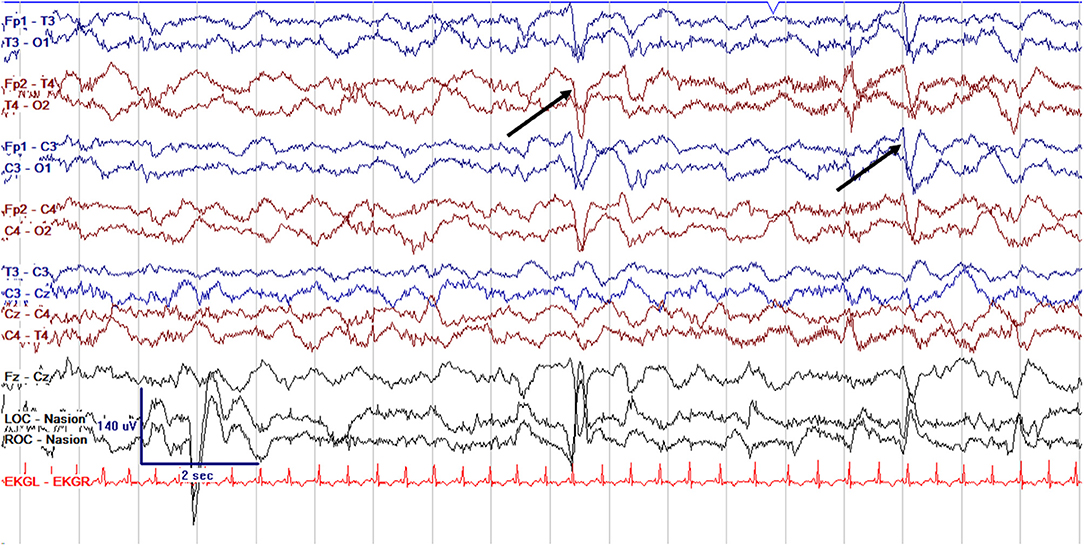
Figure 2. Normal cEEG in a term neonate in the awake state, a 3 day old former 39 + 0 week GA (39 + 3 week PMA) with normal continuous activity of variable voltage and frequency with normal graphoelements (encoche frontales–normal frontal sharps, arrows).
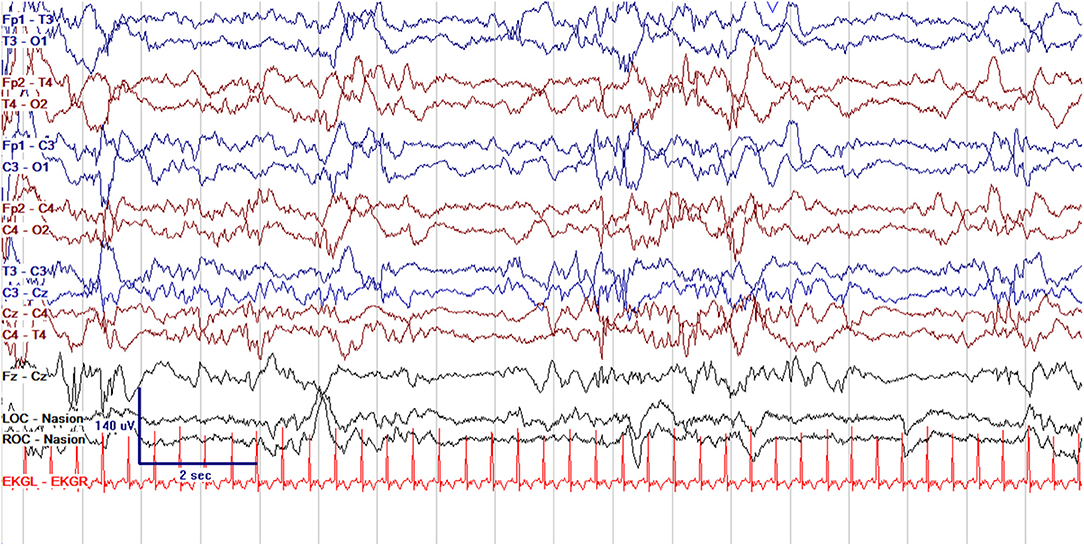
Figure 3. Normal cEEG in the same term neonate in quiet sleep, with normal discontinuity (trace alternans) with interburst intervals lasting up to 6 s and of voltage >25 μV.
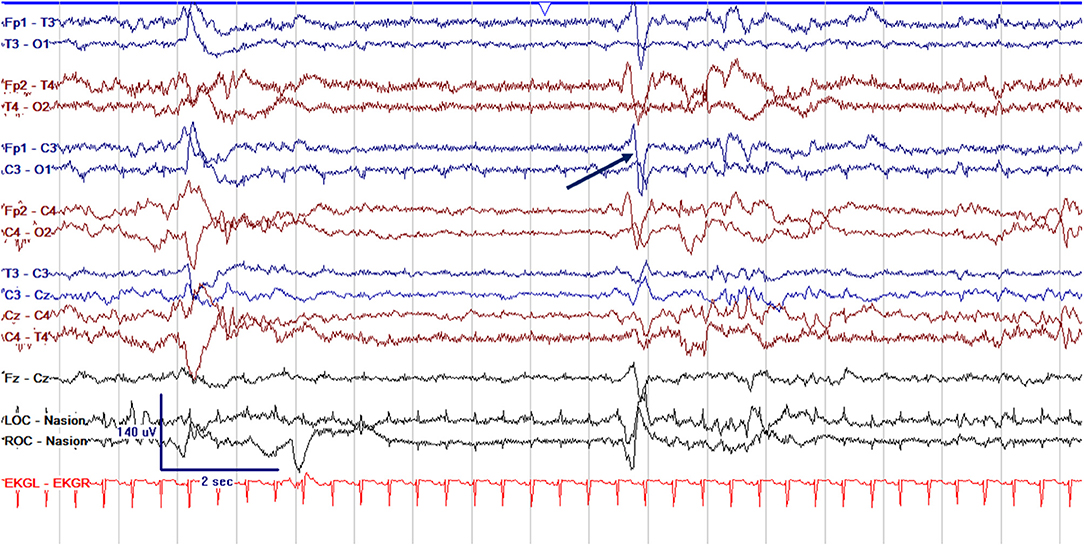
Figure 4. Abnormal excessively discontinuous cEEG background in a term neonate, a 1 day old former 39 + 0 week GA (39 + 1 PMA) with interburst intervals lasting >6 s with voltages <25 μV. Normal graphoelements, encoche frontales–normal frontal sharps, are seen (arrow).
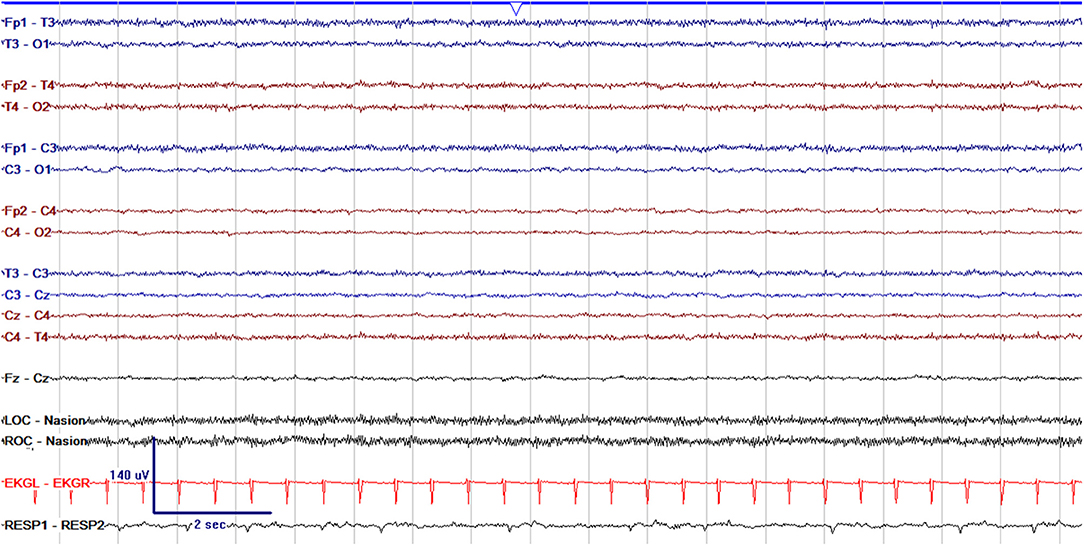
Figure 5. Severely abnormal cEEG background which is entirely suppressed with voltage <10 μV and featureless in a 1 day old former 37 + 6 week GA (37 + 7 week PMA) neonate.
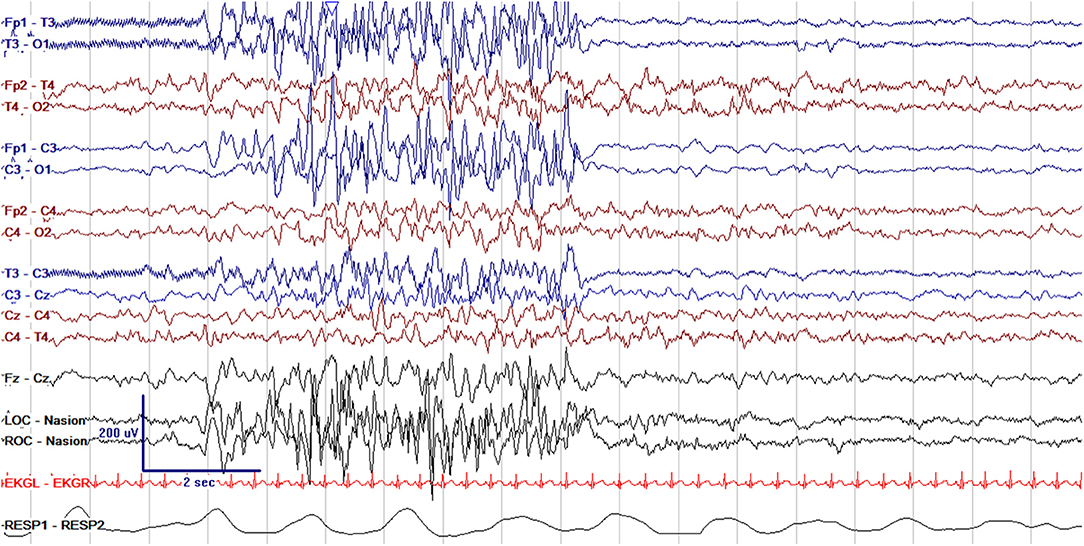
Figure 6. Abnormal asymmetric cEEG with discontinuous high amplitude bursts of epileptiform activity in the left hemisphere and more normal continuity and voltage in the right hemisphere in a 10 day old former 39 + 1 week GA (40 + 4 week PMA) with left hemimegalencephaly.
Evolution of cEEG background has been used to guide prognostication in Hypoxic Ischemic Encephalopathy (HIE). An early normal EEG background at 6, 12, and 24 h of life is predictive of a favorable outcome at 2 years of age (5). Conversely, abnormal background with sustained suppression or burst suppression at 72 h is predictive of death or severe disability in >90% (5–8). Prior to the widespread implementation of therapeutic hypothermia for neuroprotection in HIE, severe EEG background abnormalities at 6 h of age were associated with poor neurodevelopmental outcomes, while normal background at 6 h was associated with normal outcomes (5). Further, recovery of the background within 24 h was associated with improved outcomes (5). In the era of cooling, the time points at which EEG background is useful for prognostication have changed. Discontinuity on EEG for >30 s per min (equating to 50% or greater discontinuity) at 24 and 48 h of age was associated with MRI injury and worse neurodevelopmental outcome in term neonates with HIE who underwent therapeutic hypothermia (8). In another study, severe background abnormalities on EEG (discontinuous activity with interburst intervals 10–60 s, severe attenuation of background activity, no sleep-wake cycles, isoelectric EEG with activity <5 μV, or severe discontinuity with interburst interval >60 s) at 36 and 48 h of age was associated with severe injury on MRI and abnormal neurodevelopmental outcome in term neonates with HIE who underwent cooling (7). A large meta-analysis found that EEG background patterns of burst suppression, low voltage, and flat trace were the most predictive of abnormal neurodevelopmental outcome, with pooled estimates of sensitivity and specificity of 87 and 82% for burst suppression, 92 and 99% for low voltage, and 78 and 99% for flat tracing, however there was some variation in the definitions used for these background patterns across studies (6). The Total Body Hypothermia for Neonatal Encephalopathy Trial (TOBY) demonstrated a positive predictive value of severely abnormal aEEG for death or disability at 18 months of 56% (9).
EEG background can also be used to predict the occurrence of seizures, with higher sensitivity and specificity in models combining both clinical and EEG data (10–12). In a historical sample of 2,000 neonates, poor mental status with lethargy or coma and moderate to severe EEG background abnormalities were associated with seizures on EEG (10). A more contemporary sample of 210 neonates found that seizure prediction models combining clinical and EEG characteristics yielded a higher area under the curve of 83% compared to 66% with clinical variables alone and 76% with EEG variables alone (12). Neonates with clinically suspected seizures had higher risk compared to neonates with encephalopathy alone, and abnormal EEG background conferred higher risk for seizures, with more severe abnormalities having higher risk (excessively discontinuous background OR 7.10, burst suppression OR 19.45, and depressed/undifferentiated OR 27.78 compared to normal EEG) (12). By contrast, a normal EEG background and presence of sleep-wake cycling were associated with a low likelihood of seizures (OR 0.12 and OR 0.29, respectively) (12). In this sample of 210 neonates, only 2 with normal EEG background had seizures, and both had known brain injury (12). A different sample of 90 term neonates with HIE similarly found that initial EEG background was strongly associated with risk for seizures, with more severe background abnormalities conferring higher risk, independent of treatment with antiseizure medications prior to EEG (11). In this sample, however, 12% of neonates with normal initial EEG background did have seizures, though further clinical details of these subjects were not provided (11).
As the brain develops with increasing gestational age, the cEEG background demonstrates expected maturational changes, as seen in Figures 1–3. With brain maturation, there is expected evolution with increasing continuity, the appearance of sleep cycling, increasing hemispheric synchrony, and PMA-specific graphoelements (13). Brain dysfunction in preterm neonates may lead to altered rates of brain maturation with dysmaturity on EEG, a gap between the actual PMA of the neonate and the PMA suggested by the appearance of their EEG (4, 14, 15). cEEG allows the most accurate assessment of brain maturation, with visualization of graphoelements and synchrony (13, 16). EEG dysmaturity in pre-term infants has been associated with worse developmental outcomes at 12 and 24 months of age (15).
cEEG background can also be suggestive of specific diagnoses. Focal attenuation of one region or hemisphere is suggestive of focal dysfunction in that region, such as ischemia from an arterial stroke or venous sinus thrombosis. Focal epileptiform activity may be seen in cases of focal brain malformation, including extensive polymicrogyria or hemimegalencephaly (see Figure 6). Severe background abnormalities with burst suppression on EEG and refractory seizures in a neonate with encephalopathy of unknown etiology suggest a diagnosis of epileptic encephalopathy with likely genetic cause; mutations in the KCNQ2 gene are the most common cause of neonatal onset genetic epilepsy and epileptic encephalopathy (17–19).
Continuous EEG for Seizure Monitoring
Seizures are the most common neurological condition affecting neonates; cEEG is essential to their accurate diagnosis. Neonatal seizures affect 1–5/1,000 live births and have a wide range of etiologies (1, 20). Neonatal seizures are most often subclinical, or electrographic only, meaning they have no outward clinical signs and can be diagnosed solely by EEG (see Figure 7). An estimated 80–90% of seizures in neonates are subclinical, and those that initially have a clinical correlate frequently become subclinical after treatment (20–22). Electroclinical uncoupling, the phenomenon in which clinical seizures transition to subclinical seizures following administration of antiseizure medications, occurs in up to 58% after treatment with phenobarbital or phenytoin (20–22). For all these reasons, clinical diagnosis of neonatal seizures is challenging and often inaccurate; in a study in a large NICU network in Ireland, only 9% of true electrographic seizures were clinically diagnosed, while 73% of non-ictal events were incorrectly identified as seizures (23). A study with video review of events concerning for possible seizure identified only 50% correctly and demonstrated poor interrater agreement with a low Kappa of 0.2 (24).
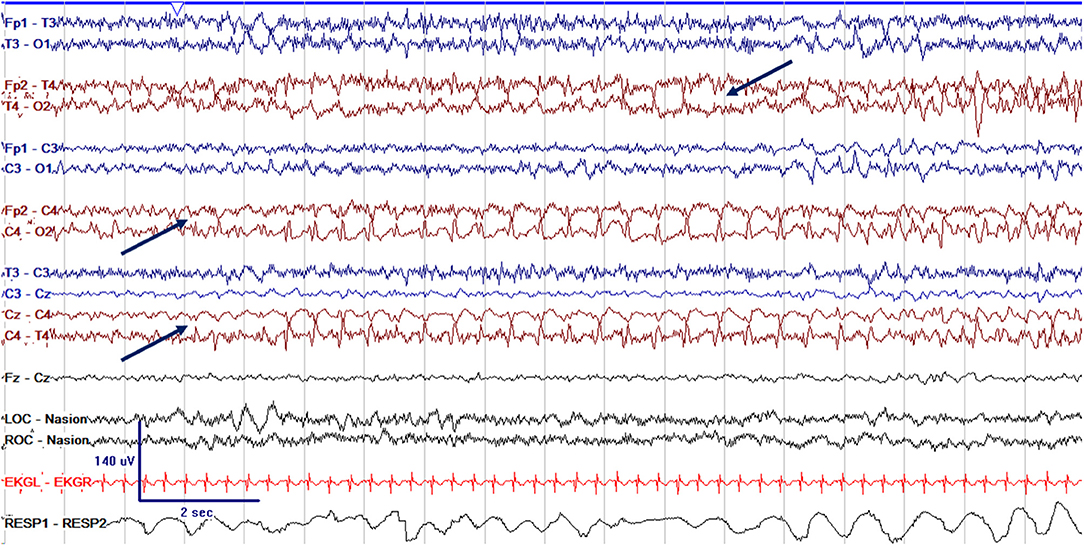
Figure 7. cEEG of a focal electrographic only seizure (arrows) arising from the right central region (C4), with spread to the right temporal region (T4) in a 3 week old former 38 + 4 week GA (41 + 4 week PMA) neonate.
Due to the high rates of subclinical seizures and inability to reliably diagnose seizures clinically in neonates, cEEG is required for accurate diagnosis. WHO, ILAE, and ACNS guidelines all advise that cEEG be used for diagnosis in neonates with clinical suspicion for seizures (1–3). Further, the ILAE and ACNS recommend cEEG for critically ill neonates at risk for seizures (see Table 1) (2, 3). Neonates at high risk for seizures should have 24 h of cEEG monitoring, and neonates with confirmed seizures should continue on cEEG for at least 24 h of seizure freedom (3). A recent large study of term neonates with acute symptomatic seizures further demonstrated the utility of cEEG for neonates at high risk for seizures. The rate of successful response to initial seizure treatment with an ASM was higher in neonates undergoing cEEG for seizure screening due to high-risk clinical conditions compared to neonates undergoing cEEG for confirmation of clinically concerning events for seizure (39% successful initial response to treatment vs. 18% response) (25). The suggested framework for implementation of therapeutic hypothermia for the treatment of HIE from the American Academy of Pediatrics also advises the use of EEG for seizure detection and monitoring and suggests that centers treating HIE have this resource available (26).
Ultimately, cEEG is most often used in the NICU to screen for and diagnose seizures. While further research is needed, there is evidence to suggest that neonatal seizures are independently associated with worsened developmental outcomes. In this way, cEEG to guide effective neonatal seizure diagnosis and treatment ultimately hopes to reduce the risk of adverse outcomes among neonates at risk. Neonatal seizures are most often acute symptomatic seizures caused by an acute brain injury, such as HIE or arterial ischemic stroke. In addition to the neurodevelopmental impact of the brain injury or condition causing the seizures, the seizures independently are associated with additional risk for further brain injury and worse neurodevelopmental outcomes. Neonates with seizures have more severe MRI brain injury and worse neurodevelopmental outcomes compared to those with a similar brain injury and no seizures, suggesting that the seizures themselves mediate further injury (27–30). Neonatal seizures lead to altered cerebral hemodynamics, increased cerebral metabolic demands above energy supply with decreased phosphocreatine to inorganic phosphate ratios, increased cerebral lactate and choline, and alteration of hippocampal neurons (31–34). Similarly, long-term outcomes are associated with presence of seizures and seizure burden: both clinical and EEG seizures are associated with poorer outcomes including lower IQ, cerebral palsy, and increased mortality (28–30). Higher electrographic seizure burden is associated with worse MRI injury and worse neurodevelopmental outcomes including motor and language delays, post-neonatal epilepsy, and cerebral palsy (27, 35–37). Given these neurodevelopmental consequences of EEG seizures in neonates, accurate diagnosis is essential. EEG is employed to guide treatment and in hopes of improving outcomes.
Continuous EEG in Combination With Amplitude-Integrated EEG
While amplitude-integrated EEG (aEEG) is useful for bedside screening for seizures, cEEG is preferred for confirming a diagnosis of seizures. The use of both tools together allows teams to benefit from the particular strengths of each. Bedside monitoring with aEEG for seizures allows caregivers to directly review the simplified aEEG tracing without reliance on a neurophysiologist for interpretation. This can be an incredibly helpful tool. At the same time, cEEG remains necessary because aEEG used alone will miss some electrographic seizures and may lead to incorrect diagnosis of seizures in other cases. Neonatal seizures are often focal, brief, and of a slow frequency. Because the aEEG tracing filters out slow frequencies and compresses the time scale, very slow seizures with discharges at a frequency of <2 Hz or brief seizures lasting <30 s are not detected; seizures lasting 90 s appear for only 1.4 mm on the display (see Table 2) (38). aEEG also uses a limited number of channels which allows rapid application and bedside interpretation, but misses seizures that occur in locations not covered by the limited aEEG montage, including the frontal and posterior regions (39). The sensitivity and specificity of aEEG is also variable and highly dependent upon the skill level in interpretation, with reported sensitivity ranging from 40 to 85% and specificity ranging from 50 to 90% (40–47). Interrater agreement of aEEG seizure interpretation is also poor, with a Kappa value of only 0.3 (48). When considering the role of aEEG for neonatal seizures, it is best used as a screening tool when there is a high incidence of seizures and a high skill level of interpretation, with confirmation by cEEG (see Table 2) (40). In settings where cEEG is not readily available, aEEG and cEEG are best used in combination, with aEEG for seizure screening when there is a sufficiently high seizure prevalence and high skill level of interpretation and cEEG for seizure confirmation.
Impact of CEEG in the NICU
Even as there is an association between seizure burden and adverse outcomes, there have remained questions about the benefits of cEEG use in the NICU, particularly when weighed against cost and other barriers. The balance of the potential benefits and costs may vary according to the resources at hand for a given center (see Table 3).
Building evidence demonstrates that cEEG can be cost-effective and beneficial to short-term outcomes, such as reduced anti-seizure medication use and reduced length of NICU stay. In a cohort of neonates with HIE randomized to treatment of EEG seizures or clinical seizures, the use of cEEG to guide seizure management was associated with significantly lower seizure burden (median seizure burden of 449 vs. 2,226 s) and decreased time to treatment completion (mean time 79 vs. 170 min) (37). At another center, implementation of a cEEG-based neonatal status epilepticus protocol was followed by decreased progression of seizures to status epilepticus by 10%, and length of stay decreased by almost 10 days (49). There were also lower phenobarbital levels, indicating better targeted therapy with lower exposure to antiseizure medications (ASMs), which carry their own risks and adverse effects (49). An additional single-center study found that continuous EEG use increased electrographic seizure detection and at the same time decreased phenobarbital exposure, as well as decreased how many neonates were discharged on an ASM. This further demonstrates that even with the increased identification of seizures, cEEG allows for tailored therapy that minimizes overtreatment via accurate diagnosis (50). In a longitudinal study performed at a single center that assessed practices across three periods, when comparing cEEG to aEEG or routine EEG, there was improved seizure identification with cEEG as compared to aEEG or routine EEG. Furthermore, there was decreased risk of receiving an ASM with cEEG as compared to aEEG or routine EEG use (51). As cEEG monitoring is more widely used, mounting evidence shows that it is associated with improved short-term outcomes in the NICU.
Future Uses of CEEG
Future work is needed to reduce barriers to cEEG implementation and improve the cost effectiveness of this technology (see Table 4). There are notable barriers to widespread implementation and use of cEEG (see Table 3). Hardware costs of EEG machines and electrodes represent a significant investment for settings with limited resources. There are also high personnel costs; skilled EEG technologists are needed to apply electrodes and trained neurophysiologists are required to interpret the EEG data. To further extend this resource, a larger institution can remotely interpret EEG for multiple hospitals using a digital central server with remote access. Implementation of remote cEEG at two hospitals affiliated with the Children's Hospital of Philadelphia identified electrographic seizures in 24% and impacted clinical care in 75% (52). This was feasible, effective and clinically significant care. This creates a centralized hub for EEG interpretation and management and increases access to the valuable resource of cEEG. Centralized, remote EEG interpretation is one strategy that neonatal neurology can employ to address health disparities by providing more equitable access to cEEG.
In addition to centralized EEG interpretation, automated seizure detection is another method that might allow further expansion of cEEG use in the future. Automated seizure detection algorithms applied to neonatal cEEG have wide ranges of reported sensitivities between 43 and 81% and specificities between 56 and 90% (53–59). The nature of neonatal seizures as being focal and often brief make automated seizure detection in neonates particularly challenging. With one method of automated neonatal seizure detection, the odds of detecting seizures increased with increasing seizure amplitude, duration, rhythmicity, and number of EEG channels involved (55). These algorithms are limited in their application to neonatal EEG due to artifacts and false-positive events which are frequently related to nursing care, including patting, along with respiratory artifacts, sweat artifacts, and increased rhythmicity in sleep (53, 55, 58, 59). Computer vision algorithms can be applied to video recording using dense optical flow estimation to reduce false positives in automated seizure detection (53).
Single-use or reusable neonatal EEG caps with electrodes at set distances that can be easily applied at the bedside can also increase access to cEEG. Several EEG caps sized for neonates have become available for clinical use, with some having the ability to record using a full neonatal electrode configuration in the International 10–20 System, and some using a limited number of 6 or 10 electrodes, increasing the number of channels recorded compared to aEEG (60, 61). There is ongoing need for innovation to improve these caps, however, as one model of full montage EEG cap yielded uninterpretable recordings in 10% of neonates <35 weeks PMA and 52% of neonates ≥35 weeks PMA, with uninterpretable recordings more frequent with older, heavier infants with larger head circumferences (60). Wireless EEG devices can also be applied, and allow for wireless digital transmission of EEG recordings (60).
Another future direction of EEG includes expanding this form of monitoring in utero. When considering processes that begin before birth that predispose neonates to seizures, such as chorioamnionitis, placental insufficiency or chronic placental abruption, brain malformations, and neonatal onset genetic epilepsies, seizures can begin prior to delivery. When evaluating a neonate with seizures, a component of the pregnancy history includes abnormal movements, including repetitive movements sometimes interpreted as hiccups, which may indicate seizures occurring before the time of birth. Though these are clinically suspected to be seizures retrospectively, there is no current system to accurately diagnose or monitor for seizures in utero. There is ongoing research in fetal EEG in both animal models and in small studies involving limited electrodes in humans (62–65). Fetal EEG may be on the horizon to enhance early detection of seizures and may allow for improved early treatment of seizures using antiseizure medications that cross the placenta, or with administration of antiseizure medications at delivery.
Conclusions
Continuous EEG is an essential tool for brain-centered care in the NICU. Assessment of the EEG background allows for improved prognostication in acute brain injury such as HIE. Continuous EEG is also the gold standard method for diagnosis and management of neonatal seizures and should be used to detect seizures in clinical conditions which portend a high risk for seizures, for differential diagnosis of paroxysmal events, and to assess response to treatment. Though there are clear benefits to cEEG, there are also significant equipment and personnel costs associated with cEEG implementation in a hospital system. Future applications of cEEG include centralized remote EEG interpretation to improve access to this resource, and fetal EEG to improve neuroprotection in utero.
Author Contributions
ASK conceived of the idea and wrote the manuscript. CW provided critical feedback and contributed to and edited the manuscript. All authors contributed to the article and approved the submitted version.
Funding
ASK is supported by a grant from the American Epilepsy Society and the Pediatric Epilepsy Research Foundation.
Conflict of Interest
The authors declare that the research was conducted in the absence of any commercial or financial relationships that could be construed as a potential conflict of interest.
Publisher's Note
All claims expressed in this article are solely those of the authors and do not necessarily represent those of their affiliated organizations, or those of the publisher, the editors and the reviewers. Any product that may be evaluated in this article, or claim that may be made by its manufacturer, is not guaranteed or endorsed by the publisher.
References
1. World Health Organization Department of Mental Health and Substance Abuse Agarwal R Department of Maternal N Child and Adolescent Health OASI Institute for Research and Prevention of Mental Retardation Troina I. Guidelines on Neonatal Seizures (2011).
2. Pressler RM, Cilio MR, Mizrahi EM, Moshé SL, Nunes ML, Plouin P, et al. The ILAE classification of seizures and the epilepsies: modification for seizures in the neonate. Position paper by the ILAE Task Force on Neonatal Seizures. Epilepsia. (2021) 62:615–28. doi: 10.1111/epi.16815
3. Shellhaas RA, Chang T, Tsuchida T, Scher MS, Riviello JJ, Abend NS, et al. The American Clinical Neurophysiology Society's guideline on continuous electroencephalography monitoring in neonates. J Clin Neurophysiol. (2011) 28:7. doi: 10.1097/WNP.0b013e31823e96d7
4. Tsuchida TN, Wusthoff CJ, Shellhaas RA, Abend NS, Sullivan JE, Nguyen S, et al. American Clinical Neurophysiology Society standardized EEG terminology and categorization for the description of continuous EEG monitoring in neonates: report of the American Clinical Neurophysiology Society critical care monitoring committee. J Clin Neurophysiol. (2013) 30:13. doi: 10.1097/WNP.0b013e3182872b24
5. Murray DM, Boylan GB, Ryan CA, Connolly S. Early EEG findings in hypoxic-ischemic encephalopathy predict outcomes at 2 years. Pediatrics. (2009) 124:e459–67. doi: 10.1542/peds.2008-2190
6. Awal MdA, Lai MM, Azemi G, Boashash B, Colditz PB. EEG background features that predict outcome in term neonates with hypoxic ischaemic encephalopathy: a structured review. Clin Neurophysiol. (2016) 127:285–96. doi: 10.1016/j.clinph.2015.05.018
7. Weeke LC, Boylan GB, Pressler RM, Hallberg B, Blennow M, Toet MC, et al. Role of EEG background activity, seizure burden and MRI in predicting neurodevelopmental outcome in full-term infants with hypoxic-ischaemic encephalopathy in the era of therapeutic hypothermia. Eur J Paediatr Neurol. (2016) 20:855–64. doi: 10.1016/j.ejpn.2016.06.003
8. Dunne JM, Wertheim D, Clarke P, Kapellou O, Chisholm P, Boardman JP, et al. Automated electroencephalographic discontinuity in cooled newborns predicts cerebral MRI and neurodevelopmental outcome. Arch Dis Child. (2017) 102:F58–64. doi: 10.1136/archdischild-2015-309697
9. Azzopardi DV, Edwards AD, Halliday HL, Kapellou O, Porter E, Whitelaw A. Moderate hypothermia to treat perinatal asphyxial encephalopathy. N Engl J Med. (2009) 361:1349–58. doi: 10.1056/NEJMoa0900854
10. Glauser TA, Clancy RR. Adequacy of routine EEG examinations in neonates with clinically suspected seizures. J Child Neurol. (1992) 7:215–20. doi: 10.1177/088307389200700216
11. Glass HC, Wusthoff CJ, Shellhaas RA, Tsuchida TN, Bonifacio SL, Cordeiro M, et al. Risk factors for EEG seizures in neonates treated with hypothermia. Neurology. (2014) 82:1239–44. doi: 10.1212/WNL.0000000000000282
12. Sansevere AJ, Kapur K, Peters JM, Fernández IS, Loddenkemper T, Soul JS. Seizure prediction models in the neonatal intensive care unit. J Clin Neurophysiol. (2019) 36:186–94. doi: 10.1097/WNP.0000000000000574
13. Pavlidis E, Lloyd RO, Mathieson S, Boylan GB. A review of important electroencephalogram features for the assessment of brain maturation in premature infants. Acta Paediatr. (2017) 106:1394–408. doi: 10.1111/apa.13956
14. Scher MS. Neurophysiological assessment of brain function and maturation: I. A measure of brain adaptation in high risk infants. Pediatr Neurol. (1997) 16:191–8. doi: 10.1016/S0887-8994(97)00008-8
15. Scher MS. Neurophysiological assessment of brain function and maturation II. A measure of brain dysmaturity in healthy preterm neonates. Pediatr Neurol. (1997) 16:287–95. doi: 10.1016/S0887-8994(96)00009-4
16. Kato T, Okumura A, Hayakawa F, Tsuji T, Natsume J, Watanabe K. Evaluation of brain maturation in pre-term infants using conventional and amplitude-integrated electroencephalograms. Clin Neurophysiol. (2011) 122:1967–72. doi: 10.1016/j.clinph.2010.12.063
17. Goto A, Ishii A, Shibata M, Ihara Y, Cooper EC, Hirose S. Characteristics of KCNQ2 variants causing either benign neonatal epilepsy or developmental and epileptic encephalopathy. Epilepsia. (2019) 60:1870–80. doi: 10.1111/epi.16314
18. Kato M, Yamagata T, Kubota M, Arai H, Yamashita S, Nakagawa T, et al. Clinical spectrum of early onset epileptic encephalopathies caused by KCNQ2 mutation. Epilepsia. (2013) 54:1282–7. doi: 10.1111/epi.12200
19. Beal JC, Cherian K, Moshe SL. Early-onset epileptic encephalopathies: ohtahara syndrome and early myoclonic encephalopathy. Pediatr Neurol. (2012) 47:317–23. doi: 10.1016/j.pediatrneurol.2012.06.002
20. Abend NS, Wusthoff CJ. Neonatal seizures and status epilepticus. J Clin Neurophysiol. (2012) 29:441–8. doi: 10.1097/WNP.0b013e31826bd90d
21. Scher MS, Alvin J, Gaus L, Minnigh B, Painter MJ. Uncoupling of EEG-clinical neonatal seizures after antiepileptic drug use. Pediatr Neurol. (2003) 28:277–80. doi: 10.1016/S0887-8994(02)00621-5
22. Shellhaas RA. Continuous long-term electroencephalography: the gold standard for neonatal seizure diagnosis. Semin Fetal Neonatal Med. (2015) 20:149–53. doi: 10.1016/j.siny.2015.01.005
23. Murray DM, Boylan GB, Ali I, Ryan CA, Murphy BP, Connolly S. Defining the gap between electrographic seizure burden, clinical expression and staff recognition of neonatal seizures. Arch Dis Child. (2008) 93:F187–91. doi: 10.1136/adc.2005.086314
24. Malone A, Ryan CA, Fitzgerald A, Burgoyne L, Connolly S, Boylan GB. Interobserver agreement in neonatal seizure identification. Epilepsia. (2009) 50:2097–101. doi: 10.1111/j.1528-1167.2009.02132.x
25. Wusthoff CJ, Sundaram V, Abend NS, Massey SL, Lemmon ME, Thomas C, et al. Seizure control in neonates undergoing screening vs confirmatory EEG monitoring. Neurology. (2021) 97:e587–96. doi: 10.1212/WNL.0000000000012293
26. Newborn C on FA. Hypothermia and neonatal encephalopathy. Pediatrics. (2014) 133:1146–50. doi: 10.1542/peds.2014-0899
27. Shah DK, Wusthoff CJ, Clarke P, Wyatt JS, Ramaiah SM, Dias RJ, et al. Electrographic seizures are associated with brain injury in newborns undergoing therapeutic hypothermia. Arch Dis Child. (2014) 99:F219–24. doi: 10.1136/archdischild-2013-305206
28. Ronen GM, Buckley D, Penney S, Streiner DL. Long-term prognosis in children with neonatal seizures: A population-based study. Neurology. (2007) 69:1816–22. doi: 10.1212/01.wnl.0000279335.85797.2c
29. McBride MC, Laroia N, Guillet R. Electrographic seizures in neonates correlate with poor neurodevelopmental outcome. Neurology. (2000) 55:506–13. doi: 10.1212/WNL.55.4.506
30. Glass HC, Glidden D, Jeremy RJ, Barkovich AJ, Ferriero DM, Miller SP. Clinical neonatal seizures are independently associated with outcome in infants at risk for hypoxic-ischemic brain injury. J Pediatr. (2009) 155:318–23. doi: 10.1016/j.jpeds.2009.03.040
31. Zhou C, Lippman Bell JJ, Sun H, Jensen FE. Hypoxia-induced neonatal seizures diminish silent synapses and long-term potentiation in hippocampal CA1 neurons. J Neurosci. (2011) 31:18211–22. doi: 10.1523/JNEUROSCI.4838-11.2011
32. Younkin DP, Delivoria-Papadopoulos M, Maris J, Donlon E, Clancy R, Chance B. Cerebral metabolic effects of neonatal seizures measured with in vivo 31P NMR spectroscopy. Ann Neurol. (1986) 20:513–9. doi: 10.1002/ana.410200412
33. Miller SP, Weiss J, Barnwell A, Ferriero DM, Latal-Hajnal B, Ferrer-Rogers A, et al. Seizure-associated brain injury in term newborns with perinatal asphyxia. Neurology. (2002) 58:542–8. doi: 10.1212/WNL.58.4.542
34. Miller SM, Goasdoue K, Björkman ST. Neonatal seizures and disruption to neurotransmitter systems. Neural Regen Res. (2017) 12:216–7. doi: 10.4103/1673-5374.200803
35. Kharoshankaya L, Stevenson NJ, Livingstone V, Murray DM, Murphy BP, Ahearne CE, et al. Seizure burden and neurodevelopmental outcome in neonates with hypoxic–ischemic encephalopathy. Dev Med Child Neurol. (2016) 58:1242–8. doi: 10.1111/dmcn.13215
36. Fitzgerald MP, Massey SL, Fung FW, Kessler SK, Abend NS. High electroencephalographic seizure exposure is associated with unfavorable outcomes in neonates with hypoxic-ischemic encephalopathy. Seizure. (2018) 61:221–6. doi: 10.1016/j.seizure.2018.09.003
37. Srinivasakumar P, Zempel J, Trivedi S, Wallendorf M, Rao R, Smith B, et al. Treating EEG seizures in hypoxic ischemic encephalopathy: a randomized controlled trial. Pediatrics. (2015) 136:e1302–9. doi: 10.1542/peds.2014-3777
38. Glass HC, Wusthoff CJ, Shellhaas RA. Amplitude integrated EEG: the child neurologist's perspective. J Child Neurol. (2013) 28:1342–50. doi: 10.1177/0883073813488663
39. Wusthoff CJ, Shellhaas RA, Clancy RR. Limitations of single-channel EEG on the forehead for neonatal seizure detection. J Perinatol. (2009) 29:237–42. doi: 10.1038/jp.2008.195
40. Sandoval Karamian AG, Wusthoff CJ. How helpful is aEEG? Context and user experience matter. Am J Perinatol. (2020). doi: 10.1055/s-0040-1721711 [Epub ahead of print].
41. Frenkel N, Friger M, Meledin I, Berger I, Marks K, Bassan H, et al. Neonatal seizure recognition – comparative study of continuous-amplitude integrated EEG versus short conventional EEG recordings. Clin Neurophysiol. (2011) 122:1091–7. doi: 10.1016/j.clinph.2010.09.028
42. Lawrence R, Mathur A, Tich SN, Zempel J, Inder T. A pilot study of continuous limited-channel aEEG in term infants with encephalopathy. J Pediatr. (2009) 154:835–41.e1. doi: 10.1016/j.jpeds.2009.01.002
43. Shah DK, Mackay MT, Lavery S, Watson S, Harvey AS, Zempel J, et al. Accuracy of bedside electroencephalographic monitoring in comparison with simultaneous continuous conventional electroencephalography for seizure detection in term infants. Pediatrics. (2008) 121:1146–54. doi: 10.1542/peds.2007-1839
44. Zhang L, Zhou Y-X, Chang L-W, Luo X-P. Diagnostic value of amplitude-integrated electroencephalogram in neonatal seizures. Neurosci Bull. (2011) 27:251. doi: 10.1007/s12264-011-1413-x
45. Rennie J, Chorley G, Boylan G, Pressler R, Nguyen Y, Hooper R. Non-expert use of the cerebral function monitor for neonatal seizure detection. Arch Dis Child Fetal Neonatal Ed. (2004) 89:F37–40. doi: 10.1136/fn.89.1.F37
46. Shellhaas RA, Soaita AI, Clancy RR. Sensitivity of amplitude-integrated electroencephalography for neonatal seizure detection. Pediatrics. (2007) 120:770–7. doi: 10.1542/peds.2007-0514
47. Rakshasbhuvankar AA, Wagh D, Athikarisamy SE, Davis J, Nathan EA, Palumbo L, et al. Inter-rater reliability of amplitude-integrated EEG for the detection of neonatal seizures. Early Hum Dev. (2020) 143:105011. doi: 10.1016/j.earlhumdev.2020.105011
48. Bustamante-Hervás C, Valverde E, Vega-Del-Val C, Schuffelmann S, Arnaez J. Inter-observer reliability for amplitude-integrated EEG in the newborn with perinatal asphyxia. An Pediatr. (2021). doi: 10.1016/j.anpedi.2021.01.014 [Epub ahead of print].
49. Harris ML, Malloy KM, Lawson SN, Rose RS, Buss WF, Mietzsch U. Standardized treatment of neonatal status epilepticus improves outcome. J Child Neurol. (2016) 31:1546–54. doi: 10.1177/0883073816664670
50. Bashir RA, Espinoza L, Vayalthrikkovil S, Buchhalter J, Irvine L, Bello-Espinosa L, et al. Implementation of a neurocritical care program: improved seizure detection and decreased antiseizure medication at discharge in neonates with hypoxic-ischemic encephalopathy. Pediatr Neurol. (2016) 64:38–43. doi: 10.1016/j.pediatrneurol.2016.07.007
51. Jan S, Northington FJ, Parkinson CM, Stafstrom CE. EEG monitoring technique influences the management of hypoxic-ischemic seizures in neonates undergoing therapeutic hypothermia. Dev Neurosci. (2017) 39:82–8. doi: 10.1159/000454855
52. Fitzgerald MP, Massey SL, Fung FW, Puopolo KM, Posencheg M, Allen-Napoli L, et al. Expanding access to continuous EEG monitoring in neonatal intensive care units. J Clin Neurophysiol. (2020). doi: 10.1097/WNP.0000000000000730 [Epub ahead of print].
53. Martin JR, Gabriel P, Gold J, Haas R, Davis S, Gonda D, et al. Optical flow estimation improves automated seizure detection in neonatal EEG. J Clin Neurophysiol. (2021). doi: 10.1097/WNP.0000000000000767 [Epub ahead of print].
54. Ansari AH, Cherian PJ, Caicedo A, Naulaers G, De Vos M, Van Huffel S. Neonatal seizure detection using deep convolutional neural networks. Int J Neural Syst. (2019) 29:1850011. doi: 10.1142/S0129065718500119
55. Mathieson S, Rennie J, Livingstone V, Temko A, Low E, Pressler RM, et al. In-depth performance analysis of an EEG based neonatal seizure detection algorithm. Clin Neurophysiol. (2016) 127:2246–56. doi: 10.1016/j.clinph.2016.01.026
56. Cherian PJ, Deburchgraeve W, Swarte RM, De Vos M, Govaert P, Van Huffel S, et al. Validation of a new automated neonatal seizure detection system: a clinician's perspective. Clin Neurophysiol. (2011) 122:1490–9. doi: 10.1016/j.clinph.2011.01.043
57. Pavel AM, Rennie JM, de Vries LS, Blennow M, Foran A, Shah DK, et al. A machine-learning algorithm for neonatal seizure recognition: a multicentre, randomised, controlled trial. Lancet Child Adolesc Health. (2020) 4:740–9. doi: 10.1016/S2352-4642(20)30239-X
58. Ulate-Campos A, Coughlin F, Gaínza-Lein M, Fernández IS, Pearl PL, Loddenkemper T. Automated seizure detection systems and their effectiveness for each type of seizure. Seizure. (2016) 40:88–101. doi: 10.1016/j.seizure.2016.06.008
59. Sharpe C, Davis SL, Reiner GE, Lee LI, Gold JJ, Nespeca M, et al. Assessing the feasibility of providing a real-time response to seizures detected with continuous long-term neonatal electroencephalography monitoring. J Clin Neurophysiol. (2019) 36:9–13. doi: 10.1097/WNP.0000000000000525
60. Ibrahim ZH, Chari G, Abdel Baki S, Bronshtein V, Kim MR, Weedon J, et al. Wireless multichannel electroencephalography in the newborn. J Neonatal-Perinat Med. (2016) 9:341–8. doi: 10.3233/NPM-161643
61. U S. Food and Drug Administration. 510(k) Premarket Notification. (2021). Available from: https://www.accessdata.fda.gov/scripts/cdrh/cfdocs/cfpmn/pmn.cfm?ID=K151576 (accessed October 11, 2021).
62. Qureshi AI, Miran MS Li S, Jiang M. Ultrasound-guided second trimester fetal electroencephalography in two pregnant volunteers: a technical note. J Vasc Interv Neurol. (2016) 9:60–5.
63. Lacan L, Hamoud Y, Nguyen S, De Jonckheere J, Storme L, Houfflin-Debarge V, et al. Fetal sheep cerebral electrical activity: a new technique to record EEG. J Neurosci Methods. (2020) 345:108888. doi: 10.1016/j.jneumeth.2020.108888
64. Abbasi H, Gunn AJ, Unsworth CP, Bennet L. Deep convolutional neural networks for the accurate identification of high-amplitude stereotypic epileptiform seizures in the post-hypoxic-ischemic EEG of preterm fetal sheep. Annu Int Conf IEEE Eng Med Biol Soc. (2020) 2020:1–4. doi: 10.1109/EMBC44109.2020.9237753
Keywords: continuous electroencephalography, neonatal seizures, neonatal epilepsy, critically ill neonates, neurodevelopmental outcomes
Citation: Sandoval Karamian AG and Wusthoff CJ (2021) Current and Future Uses of Continuous EEG in the NICU. Front. Pediatr. 9:768670. doi: 10.3389/fped.2021.768670
Received: 01 September 2021; Accepted: 12 October 2021;
Published: 03 November 2021.
Edited by:
Eugene Dempsey, University College Cork, IrelandReviewed by:
Janette Mailo, University of Alberta, CanadaAlison J. Carey, Drexel University, United States
Brian Henry Walsh, Cork University Maternity Hospital, Ireland
Copyright © 2021 Sandoval Karamian and Wusthoff. This is an open-access article distributed under the terms of the Creative Commons Attribution License (CC BY). The use, distribution or reproduction in other forums is permitted, provided the original author(s) and the copyright owner(s) are credited and that the original publication in this journal is cited, in accordance with accepted academic practice. No use, distribution or reproduction is permitted which does not comply with these terms.
*Correspondence: Amanda G. Sandoval Karamian, c2FuZG92YWxrYSYjeDAwMDQwO2Nob3AuZWR1; orcid.org/0000-0002-0813-1334; Courtney J. Wusthoff, d3VzdGhvZmYmI3gwMDA0MDtzdGFuZm9yZC5lZHU=; orcid.org/0000-0002-1882-5567