- 1Department of Respiratory Medicine, Children's Hospital of Chongqing Medical University, National Clinical Research Center for Child Health and Disorders, Ministry of Education Key Laboratory of Child Development and Disorders, Chongqing Key Laboratory of Pediatrics, Chongqing, China
- 2Department of Pediatrics, Jiangjin District Central Hospital, Chongqing, China
Background: Multiple non-invasive ventilation (NIV) modalities have been identified that may improve the prognosis of pediatric patients with acute lower respiratory infection (ALRI). However, the effect of NIV in children with ALRI remains inconclusive. Hence, this study aimed to evaluate the efficacy of various NIV strategies including continuous positive airway pressure (CPAP), high flow nasal cannula (HFNC), bilevel positive airway pressure (BIPAP), and standard oxygen therapy in children with ALRI and the need for supplemental oxygen.
Methods: Embase, PubMed, Cochrane Library, and Web of Science databases were searched from inception to July 2021. Randomized controlled trials (RCTs) that compared different NIV modalities for children with ALRI and the need for supplemental oxygen were included. Data were independently extracted by two reviewers. Primary outcomes were intubation and treatment failure rates. Secondary outcome was in-hospital mortality. Pairwise and Bayesian network meta-analyses within the random-effects model were used to synthesize data. The certainty of evidence was assessed using the Grading of Recommendations Assessment, Development and Evaluation framework.
Results: A total of 21 RCTs involving 5,342 children were included. Compared with standard oxygen therapy, CPAP (OR: 0.40, 95% CrI: 0.16–0.90, moderate quality) was associated with a lower risk of intubation. Furthermore, both CPAP (OR: 0.42, 95% CrI: 0.19–0.81, low quality) and HFNC (OR: 0.51, 95% CrI: 0.29–0.81, low quality) reduced treatment failure compared with standard oxygen therapy. There were no significant differences among all interventions for in-hospital mortality. Network meta-regression showed that there were no statistically significant subgroup effects.
Conclusion: Among children with ALRI and the need for supplemental oxygen, CPAP reduced the risk of intubation when compared to standard oxygen therapy. Both CPAP and HFNC were associated with a lower risk of treatment failure than standard oxygen therapy. However, evidence is still lacking to show benefits concerning mortality between different interventions. Further large-scale, multicenter studies are needed to confirm our results.
Systematic Review Registration: https://www.crd.york.ac.uk/prospero/display_record.php?RecordID=172156, identifier: CRD42020172156.
Introduction
Acute lower respiratory infection (ALRI), such as pneumonia and bronchiolitis, constitute a major cause of respiratory failure in children (1, 2), of whom 15% may need intensive care unit (ICU) care and respiratory support (3). The global mortality annually is estimated as 6,50,000 for ALRI in children younger than 5 years (4). Standard oxygen therapy (SOT) at a flow rate of 1–4 L/min for children with ALRI and hypoxemia is recommended by the World Health Organization (WHO) (5, 6). However, despite the provision of SOT and supportive care, more effective respiratory support systems are still needed for some critically ill children because of worsening respiratory distress (7).
To avoid airway invasivity related to the intubation, various non-invasive ventilation (NIV) strategies, such as continuous positive airway pressure (CPAP), bilevel positive airway pressure (BIPAP) ventilation, and high flow nasal cannula (HFNC), have been proposed among pediatric patients (8, 9). However, the effect of NIV in children with ALRI remains inconclusive. A previous pairwise meta-analysis (10) that compared two of the three respiratory support devices (SOT, CPAP, and HFNC) revealed that in terms of reducing treatment failure, HFNC was better than SOT but inferior to CPAP. However, there were no significant differences between the therapies in terms of intubation and mortality rates. However, this conventional meta-analysis only focused on head-to-head trials of comparison of two interventions without assessing multiple interventions as a whole. In addition, since the publication of this meta-analysis, several new randomized controlled trials (RCTs) have been published (11–18). More importantly, there are no systematic reviews that have assessed the efficacy of BIPAP in pediatric patients.
A network meta-analysis (NMA) enables comparison of multiple interventions and improved precision through a combination of direct and indirect estimates of effects (19, 20). Therefore, we aimed to perform a rigorous and comprehensive Bayesian network meta-analysis to update current clinical study data and evaluate the effect of multiple NIV strategies in children with ALRI.
Methods
This systematic review was registered on PROSPERO with registration number: CRD42020172156. This report complies with the recommendations of Preferred Reporting Items for Systematic Review and Meta-Analysis (PRISMA) Extension statement for NMA (21).
Search Strategy
Pubmed, Embase, Cochrane Central Register of Controlled Trials (CENTRAL), and Web of Science were searched by one reviewer (ZW) from inception to August 1, 2020 (full search strategy is listed in Supplementary Material). The literature search was last updated on July 1, 2021.
Eligibility Criteria and Outcome Measures
Type of Studies
We included all RCTs reported in English. Observational studies, case reports, review articles, comments, letters, conference abstracts, and editorials were excluded.
Type of Participants
This review included children aged from 29 days to 18 years, with ALRI (including bronchiolitis and WHO-defined pneumonia or severe pneumonia) and the need for supplemental oxygen. Following the WHO guideline, pneumonia is defined as acute presentation of either cough or tachypnea and also had either tachypnea or lower chest wall indrawing (6). WHO-defined severe pneumonia is based on cough or difficulty in breathing plus at least one of the following: central cyanosis or oxygen saturation <90% on pulse oximetry; respiratory distress (e.g., grunting, chest indrawing); and general danger signs (inability to breastfeed or drink, lethargy or unconscious, and convulsions) (6). The definitions of bronchiolitis were individualized for each study.
Studies conducted in pediatric intensive care unit (PICU), general ward, or emergency department (ED) were included. Studies that only include patients in the neonatal period (the first month of life) were excluded. We excluded treatments used at home or for chronic conditions.
Types of Interventions and Comparators
We included RCTs comparing two or more of the following four non-invasive respiratory support devices: (1) SOT: nasal cannula, nasal prong, nasal catheter, and mask with no limit on the flow rate; (2) CPAP: the ventilatory setting and interface were not limited; (3) HFNC: the flow rate and fraction of inspired oxygen (FiO2) were not limited; and (4) BIPAP: the ventilatory setting and interface were not limited.
Type of Outcomes
The primary outcomes were (1) treatment failure, defined by the individual authors in the included studies, and (2) the rate of intubation. The secondary outcome was in-hospital mortality at the end of the follow-up period (<28 days).
Study Selection and Data Extraction
Two reviewers (YH and XZ) independently screened the titles and abstracts retrieved from the search strategy to identify those meeting the pre-specified criteria. Subsequently, further screening was performed to select eligible articles by reviewing the full texts.
The two reviewers (YH and XZ) performed data extraction independently. The following data were extracted: first author, publication year, country, study characteristics (trial design, sample size, study setting, and funding source), patients' characteristics [age, diagnosis information, comorbidities, and mean pulse oximetry saturation (SpO2) on admission], details of the intervention, and outcome data for each endpoint of interest. Any disagreement was resolved by discussion.
Risk of Bias Assessment
Two researchers (YH and XZ) evaluated the methodological quality of the eligible studies according to the Cochrane collaboration's risk of bias tool for randomized trials (22). Disagreements were solved by consensus.
Statistical Analysis
Pairwise Meta-Analysis
Conventional pairwise meta-analysis was conducted using the Mantel-Haenszel method within a random-effects model. Odds ratios (ORs) and 95% confidence intervals (CIs) were calculated for all dichotomous outcomes using Review Manager software (RevMan version 5.3). Heterogeneity was assessed using the I2 statistic (low heterogeneity = 25%, moderate heterogeneity = 50%, and high heterogeneity = 75%) (23).
Network Meta-Analysis
The NMA was conducted in a Bayesian framework random-effects model (24) using the Markov Chain Monte Carlo with vague priors by “GeMTC” package in R (version 3.6.2) and WinBUGS (version 1.4.3); the results were presented in the form of ORs and 95% credibility intervals (CrIs). The analyses used generalized linear models with a logit link function and 100,000 iterated simulations discarding the initial 20,000 iterations as burn-in. I2 statistic was used to detect global heterogeneity by using the “mtc.anohe” function of the “GeMTC” package (25). The node-splitting method was used to assess inconsistency between direct and indirect comparisons (26). Surface under the cumulative ranking curve (SUCRA) was calculated to rank the probabilities for each intervention, and a larger value indicates the better rank for each treatment (27). When 10 or more studies were available for an outcome, we assessed publication bias using the comparison-adjusted funnel plots in Stata (version 15.1).
The certainty of the evidence for the each outcome was evaluated using the Grading of Recommendations Assessment, Development and Evaluation (GRADE) framework (28) with five criteria: study limitations, imprecision, indirectness, heterogeneity and inconsistency, and publication bias.
Network Meta-Regression, Subgroup Analysis, and Sensitivity Analysis
Network meta-regression and subgroup analyses [considering mean age (<1 year vs. more than 1 year), country income (high income vs. low and middle income), mean SpO2 on admission (lower than 92% vs. more than 92%), study location (ICU vs. non-ICU), and type of disease (pneumonia vs. bronchiolitis)] were performed to explain the observed between-trail heterogeneity if data were available. For the sensitivity analyses, we eliminated the study by McCollum et al. (18). from consideration as the study included participants with high-risk conditions (such as HIV infection and severe malnutrition), and it was conducted in the general ward without daily physician supervision. Therefore, the lack of physician oversight and the examined populations were significantly different from the other studies.
Results
Selection of Articles and Characteristics of Studies
The literature search yielded 12,176 records, and 146 proved potentially eligible. Of these, we excluded 125 studies (see Figure 1) and included 21 trials (11–18, 29–41) with 5,342 children aged 19 days to 16 years. The literature search process is presented in Figure 1 and the network geometry is presented in Figure 2. Descriptive data for included studies are listed in Table 1. Study sample sizes ranged from 28 to 1,472. Among the included trials, three studies (18, 29, 41) compared CPAP with SOT, nine (11–13, 15, 16, 32, 35, 36, 39) compared HFNC with SOT, seven (14, 17, 33, 34, 37, 38, 40) compared HFNC with CPAP, one (30) compared BIPAP with SOT, and one 3-arm study (31) compared CPAP with both HFNC and SOT. Ten studies (47.6%) were conducted in in high-income countries, eight (38.1%) in middle-income countries, and three (14.3%) in low-income countries (42). Twelve studies (12, 13, 16, 17, 29, 32, 33, 35, 36, 38, 40, 41) were conducted in patients with acute bronchiolitis, and the other nine trials (11, 14, 15, 18, 30, 31, 34, 37, 39) were performed in patients with WHO defined severe pneumonia. Treatment failure was determined by clinical signs such as heart rate, respiratory rate, SpO2, and a need to escalate treatment. The criteria for treatment failure varied slightly among included studies and are summarized in Supplementary Table 1.
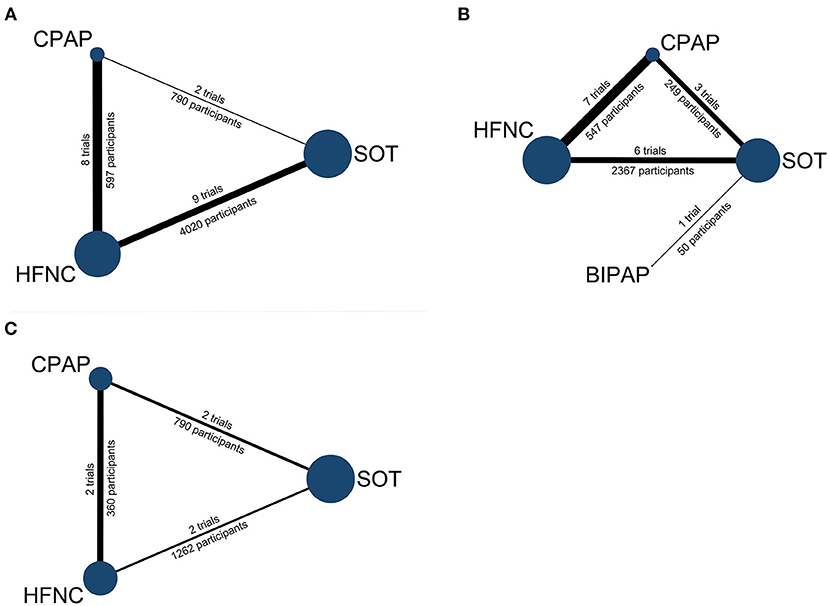
Figure 2. Network geometry: (A) treatment failure, (B) intubation, (C) in-hospital mortality. Node size represents the number of participants and the edge widths are proportional to the number of studies. BIPAP, bilevel positive airway pressure; CPAP, continuous positive airway pressure; HFNC, high-flow nasal cannula; SOT: standard oxygen therapy.
Risk of Bias Assessment
The risk of bias is summarized in Supplementary Figure 1. Due to the audible and visible differences between the device of oxygen delivery, none of the studies could be blinded for clinicians, researchers or patients. Overall, as none of the trials was at low risk of bias in all domains, we assessed all trials to be at high risk of bias.
Network Meta-Analysis
The results of pairwise comparisons are shown in Supplementary Figures 2–4.
Primary Outcomes
Treatment Failure
Seventeen trials (5,182 participants) containing three interventions (SOT, CPAP, and HFNC) provided data on treatment failure. For NMA results (Figure 3A; Supplementary Table 2), both CPAP (OR: 0.42, 95% CrI: 0.19–0.81, low quality) and HFNC (OR: 0.51, 95% CrI: 0.29–0.81, low quality) were associated with lower risk of treatment failure when compared to SOT. The SUCRA for CPAP, HFNC, and SOT were 89.0, 60.5, and 42.1%, respectively (Figure 3B; Supplementary Table 5).
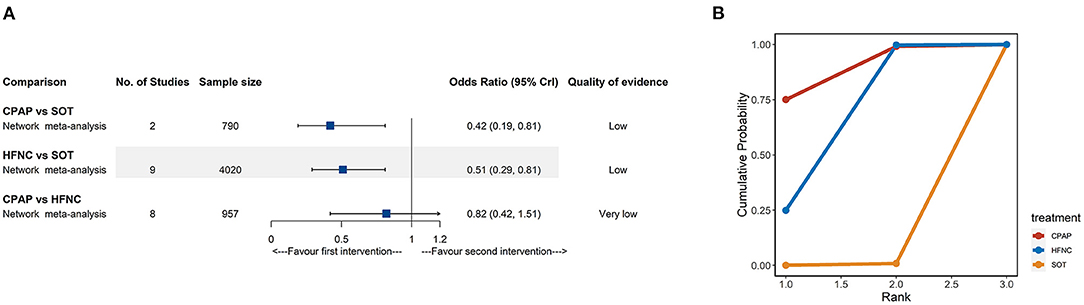
Figure 3. Network meta-analyses results (A) and cumulative probability under different rankings (B) for treatment failure. CPAP, continuous positive airway pressure; HFNC, high-flow nasal cannula; SOT, standard oxygen therapy.
Intubation Rate
Fifteen trials (2,989 participants) comparing four interventions (SOT, CPAP, HFNC, and BIPAP) reported intubation rates. According to the NMA results (Figure 4A; Supplementary Table 3), compared with SOT, CPAP (OR: 0.40, 95% CrI: 0.16–0.90, moderate quality) was associated with a lower risk of intubation. The SUCRA for BIPAP, CPAP, HFNC, and SOT were 88.1, 73.0, 28.7, and 10.2%, respectively (Figure 4B; Supplementary Table 5). The SUCRA results should be interpreted cautiously as the credible intervals were very wide for most comparisons.
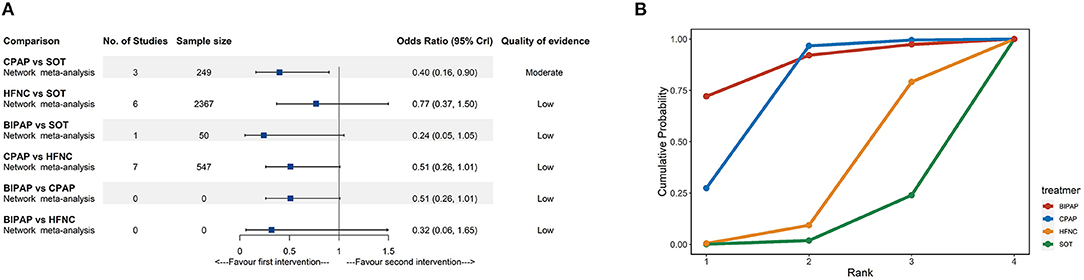
Figure 4. Network meta-analyses results (A) and cumulative probability under different rankings (B) for intubation. BIPAP, bilevel positive airway pressure; CPAP, continuous positive airway pressure; HFNC, high-flow nasal cannula; SOT, standard oxygen therapy.
Secondary Outcome
In-Hospital Mortality
Deaths were reported in only four studies (11, 18, 31, 37) involving 2,014 participants. As shown in Figure 5A, there were no significant differences for mortality rate among the different treatments from NMA. The network evidence was low or very low quality in all cases (Supplementary Table 4). Similarly, all CrIs were very wide and included the null value; the SUCRA results should be interpreted cautiously (Figure 5B; Supplementary Table 5).
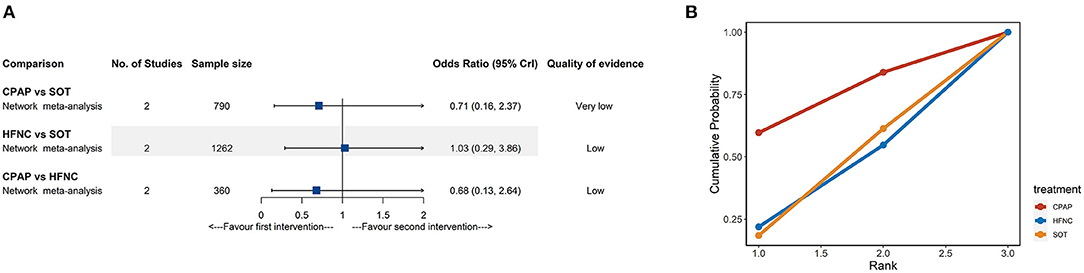
Figure 5. Network meta-analyses results (A) and cumulative probability under different rankings (B) for in-hospital mortality. CPAP, continuous positive airway pressure; HFNC, high-flow nasal cannula; SOT, standard oxygen therapy.
Network Meta-Regression and Sensitivity Analyses
For treatment failure and intubation rate, the results of network meta-regression demonstrated that there were no statistically significant subgroup effects regarding mean age, country income, mean SpO2 on admission, study location, and type of disease. The full details of the subgroup and network meta-regression analyses are reported in Supplementary File (Supplementary Figures 5, 6). We could not perform further analysis for in-hospital mortality due to the limited number of studies.
The McCollum study (18) did not report the intubation rate; therefore, sensitivity analyses were conducted for the other two outcomes (see Supplementary Tables 6, 7). After excluding the McCollum study (18), sensitivity network analysis revealed a lower rate of treatment failure for CPAP (OR: 0.54, 95% CrI: 0.32–0.90), when compared to HFNC. The remaining results were consistent with our primary analyses.
Heterogeneity and Inconsistency Analyses
Heterogeneity and inconsistency assessments are detailed in Supplementary Figures 7–9.
For treatment failure, two comparisons (CPAP vs. HFNC and HFNC vs. SOT) were found incoherent in the node-splitting assessment. The heterogeneity analysis suggested high heterogeneity in one comparison (CPAP vs. SOT). As for intubation rate, no evidence of inconsistency or heterogeneity between the studies was found. Regarding in-hospital mortality, there was no evidence for inconsistency. However, the heterogeneity test indicated high heterogeneity in one comparison (CPAP vs. SOT).
Publication Bias
There was no obvious indication of asymmetry according to the comparison-adjusted funnel plots (Supplementary Figure 10).
Discussion
In this first network meta-analysis comparing different NIV modalities in children with ALRI, we found that CPAP reduced the risk of intubation when compared to standard oxygen therapy. What is more, a lower incidence of treatment failure rate was found for both CPAP and HFNC when compared to standard oxygen therapy. However, there was no evidence of differences for all modalities concerning in-hospital mortality. Overall, the certainty of evidence was low or very low for all the outcomes, not only because of the small number of trials in each node, but also due to the variability of methodology and participants among studies.
A pairwise meta-analysis (10) of different NIV modalities in children with ALRI by Luo and colleagues showed that HFNC reduced treatment failure than SOT, despite no reduction in intubation rate. They also reported that HFNC had a higher risk of treatment failure when compared with CPAP. Our primary and sensitivity analyses are consistent with these previous findings. However, we found that CPAP use was associated with lower risks of treatment failure and intubation compared to SOT. This contrasts with the previous study by Luo et al. (10) where no significant differences between these two interventions were observed. The differences between their results and ours may be explained by the following reasons. First, this NMA had included more recently published studies. Second, this was an NMA where apart from the direct synthesis, the indirect evidence also contributed to the overall effect estimate.
Compared with low-flow oxygen therapy, CPAP works by delivering continuous distending pressure (43). Application of CPAP prevents collapse of alveoli and small airways during expiration, thus increases functional residual capacity (43, 44). HFNC has been shown to improve alveolar ventilation and carbon dioxide elimination (45) by decreasing the dead space through establishing washout in the nasopharyngeal space (46). High flow rates can also provide variable end-expiratory distending pressure based on flow rate (47, 48). Such mechanisms may explain the greater benefit of CPAP and HFNC over standard oxygen therapy in some patients. However, the airway pressure generated by HFNC is determined not only by the HFNC flow, but also by the degree of air leak from both mouth and nose (44). This may partially explain why HFNC displayed a less favorable clinical response than CPAP.
In comparison to CPAP, BIPAP delivers positive airway pressure at two different levels during inspiration and expiration, and could more efficiently decrease inspiratory work of breathing than CPAP (49). However, our pooled results suggested that when compared with SOT, BIPAP did not decrease risk of intubation. It should be noted that most of the evidence that contributed to this comparison was indirect. There was only one trial included in this meta-analysis that directly assessed the effect of BIPAP by comparing it with SOT, and this study did not report treatment failure or mortality. Therefore, conclusions regarding the relative effectiveness of BIPAP are limited, and further research is required.
In our study, we were unable to show benefits among different interventions on in-hospital mortality. Several possible explanations may account for these results. First, among the enrolled studies, 12 (57%) were focused on patients with acute bronchiolitis. Given that very low incidence of mortality has been reported since the introduction of NIV for acute bronchiolitis (33), this would make it difficult to detect differences among various methods of respiratory support. Second, in several trials (32, 33, 36–38), once treatment failure or deterioration occurred, crossover to an alternative respiratory support as rescue therapy was allowed, and the crossover may have avoided exacerbation of respiratory distress. The dilution effect of crossover may partially explain the absence of differences between any of the interventions on mortality.
Among the studies we included, 10 (47.6%) were conducted in high-income countries, where 60%−81% of children improved with standard oxygen therapy alone. Considering that non-invasive oxygen approaches are more costly and complex than standard oxygen therapy, these strategies might not be needed as preferred forms of treatment for mild cases. In resource-rich regions, more research is needed to identify children who are likely to deteriorate on standard oxygen therapy, and therefore who would benefit from the early initiation of non-invasive ventilation. However, at the other end of the spectrum of clinical severity, several included studies (11, 18, 31) from low- and middle-income countries (LMICs) showed case fatality rates of 7–14%. Meta-regression and subgroup analyses of country income (LMICs vs. high-income) found that there were no significant interactions between subgroups with regard to treatment failure and intubation rates. However, due to the low number of studies that reported mortality, subgroup analysis for in-hospital mortality was not conducted. Existing data are scarce on the effect of non-invasive ventilation on mortality of children in LMICs, and the results of previous studies remain controversial (11, 18, 31, 50). More studies will be necessary to define the role of various non-invasive ventilation modalities in resource-limited settings.
Some limitations of this review should be noted. First, there was limited statistical power for some comparisons, such as intubation rate and in-hospital mortality, because of the small numbers of trials forming several of the nodes in this network of the meta-analysis. Notably, only one of the included studies examined the efficacy of BIPAP, indicating that further studies are necessary to obtain more precise effects estimates. Second, this study involved a heterogeneous population with a wide age range (0–18 years) and disease spectrum (bronchiolitis and pneumonia). However, the heterogeneity of the study population may enhance the generalizability of the results. Moreover, to address this problem, multiple subgroup analyses were performed. Multiple subgroup results remained consistent with the overall findings. Hence, we believed that the results of our study were credible. Third, the included trials used variable definitions of treatment failure, which might produce heterogeneity. Finally, when eliminating the McCollum study (18) in the sensitivity analysis, some non-significant findings became significant, which encourages further studies to validate these results.
Conclusions
Compared to standard oxygen therapy, the use of CPAP reduced intubation rate in children with ALRI and the need for supplemental oxygen. Additionally, both CPAP and HFNC decreased the risk of treatment failure when compared with standard oxygen therapy. However, evidence is still lacking to show benefits concerning mortality between different interventions. Further large-scale, multicenter studies are needed to confirm our results.
Data Availability Statement
The original contributions presented in the study are included in the article/Supplementary Material, further inquiries can be directed to the corresponding author.
Author Contributions
ZW is responsible for the conception, data search, data analysis, statistical analyses, data interpretation, writing, and revising the manuscript. YH is responsible for inclusion and exclusion of studies, assessment of methodological quality, data extraction, writing, and revising the manuscript. XZ is responsible for inclusion and exclusion of studies, assessment of methodological quality, data extraction, and statistical analyses. ZL is responsible for the supervision and reviewed the manuscript for important intellectual content. All authors approved the manuscript.
Conflict of Interest
The authors declare that the research was conducted in the absence of any commercial or financial relationships that could be construed as a potential conflict of interest.
Publisher's Note
All claims expressed in this article are solely those of the authors and do not necessarily represent those of their affiliated organizations, or those of the publisher, the editors and the reviewers. Any product that may be evaluated in this article, or claim that may be made by its manufacturer, is not guaranteed or endorsed by the publisher.
Supplementary Material
The Supplementary Material for this article can be found online at: https://www.frontiersin.org/articles/10.3389/fped.2021.749975/full#supplementary-material
References
1. Shah PS, Ohlsson A, Shah JP. Continuous negative extrathoracic pressure or continuous positive airway pressure compared to conventional ventilation for acute hypoxaemic respiratory failure in children. Cochrane Database Syst Rev. (2013) 2013:CD003699. doi: 10.1002/14651858.CD003699.pub4
2. Meissner HC. Viral bronchiolitis in children. N Engl J Med. (2016) 374:62–72. doi: 10.1056/NEJMra1413456
3. Pierce HC, Mansbach JM, Fisher ES, Macias CG, Pate BM, Piedra PA, et al. Variability of intensive care management for children with bronchiolitis. Hosp Pediatr. (2015) 5:175–84. doi: 10.1542/hpeds.2014-0125
4. Troeger C, Blacker B, Khalil IA, Rao PC, Cao J, Zimsen SRM, et al. Estimates of the global, regional, and national morbidity, mortality, and aetiologies of lower respiratory infections in 195 countries, 1990–2016: a systematic analysis for the Global Burden of Disease Study 2016. Lancet Infect Dis. (2018) 18:1191–210. doi: 10.1016/S1473-3099(18)30310-4
5. World Health Organization. Oxygen Therapy for Children: A Manual for Health Workers. Geneva: WHO (2016).
6. World Health Organization. Pocket Book of Hospital Care for Children: Guidelines for the Management of Common Childhood Illnesses, 2nd Edn. Geneva: World Health Organization (2013).
7. Harris M, Clark J, Coote N, Fletcher P, Harnden A, McKean M, et al. British Thoracic Society guidelines for the management of community acquired pneumonia in children: update 2011. Thorax. 2011 66(Suppl. 2):ii1–23. doi: 10.1136/thoraxjnl-2011-200598
8. Morley SL. Non-invasive ventilation in paediatric critical care. Paediatr Resp Rev. (2016) 20:24–31. doi: 10.1016/j.prrv.2016.03.001
9. Viscusi CD, Pacheco GS. Pediatric emergency noninvasive ventilation. Emerg Med Clin North Am. (2018) 36:387–400. doi: 10.1016/j.emc.2017.12.007
10. Luo J, Duke T, Chisti MJ, Kepreotes E, Kalinowski V, Li J. Efficacy of high-flow nasal cannula vs standard oxygen therapy or nasal continuous positive airway pressure in children with respiratory distress: a meta-analysis. J Pediatrics. (2019) 215:199–208 e8. doi: 10.1016/j.jpeds.2019.07.059
11. Maitland K, Kiguli S, Olupot-Olupot P, Hamaluba M, Thomas K, Alaroker F, et al. Randomised controlled trial of oxygen therapy and high-flow nasal therapy in African children with pneumonia. Intensive Care Med. (2021) 47:566–76. doi: 10.1007/s00134-021-06385-3
12. Türe E, Yazar A, Akn F, Pekcan S. High-flow nasal cannula is superior to standard face-mask oxygen therapy in viral bronchiolitis. signa vitae. (2020) 16:47–53. doi: 10.22514/sv.2020.16.0007
13. Murphy S, Bruckmann E, Doedens LG, Khan AB, Salloo A, Omar S. High-flow oxygen therapy v. standard care in infants with viral bronchiolitis. South Afr J Critic Care. (2020) 36:109. doi: 10.7196/SAJCC.2020.v36i2.438
14. Liu C, Cheng WY Li JS, Tang T, Tan PL, Yang L. High-Flow Nasal Cannula vs. Continuous Positive Airway Pressure Therapy for the Treatment of Children <2 Years With Mild to Moderate Respiratory Failure Due to Pneumonia. Front Pediatr. (2020) 8:590906. doi: 10.3389/fped.2020.590906
15. Franklin D, Shellshear D, Babl FE, Hendrickson R, Williams A, Gibbons K, et al. High flow in children with respiratory failure: A randomised controlled pilot trial – A paediatric acute respiratory intervention study. J Paediatr Child Health. (2020) 57:273–81. doi: 10.1111/jpc.15259
16. Durand P, Guiddir T, Kyheng C, Blanc F, Vignaud O, Epaud R, et al. A randomised trial of high-flow nasal cannula in infants with moderate bronchiolitis. Eur Resp J. (2020) 56:1901926. doi: 10.1183/13993003.01926-2019
17. Cesar RG, Bispo BRP, Felix PHCA, Modolo MCC, Souza AAF, Horigoshi NK, et al. High-flow nasal cannula versus continuous positive airway pressure in critical bronchiolitis: a randomized controlled pilot. J Pediatric Intensive Care. (2020) 09:248–55. doi: 10.1055/s-0040-1709656
18. McCollum ED, Mvalo T, Eckerle M, Smith AG, Kondowe D, Makonokaya D, et al. Bubble continuous positive airway pressure for children with high-risk conditions and severe pneumonia in Malawi: an open label, randomised, controlled trial. Lancet Resp Med. (2019) 7:964–74. doi: 10.1016/S2213-2600(19)30243-7
19. Brignardello-Petersen R, Rochwerg B, Guyatt GH. What is a network meta-analysis and how can we use it to inform clinical practice? Pol Arch Med Wewn. (2014) 124:659–60. doi: 10.20452/pamw.2546
20. Greco T, Biondi-Zoccai G, Saleh O, Pasin L, Cabrini L, Zangrillo A, et al. The attractiveness of network meta-analysis: a comprehensive systematic and narrative review. Heart Lung vessels. (2015) 7:133–42.
21. Hutton B, Salanti G, Caldwell DM, Chaimani A, Schmid CH, Cameron C, et al. The PRISMA extension statement for reporting of systematic reviews incorporating network meta-analyses of health care interventions: checklist and explanations. Ann Intern Med. (2015) 162:777–84. doi: 10.7326/M14-2385
22. Higgins JPT, Green S. Cochrane Handbook for Systematic Reviews of Interventions Version 5.1.0. Oxford: The Cochrane Collaboration 2011. Available online at: https://training.cochrane.org/handbook
23. Higgins JP, Thompson SG, Deeks JJ, Altman DG. Measuring inconsistency in meta-analyses. BMJ. (2003) 327:557–60. doi: 10.1136/bmj.327.7414.557
24. Sutton AJ, Abrams KR. Bayesian methods in meta-analysis and evidence synthesis. Stat Methods Med Res. (2001) 10:277–303. doi: 10.1177/096228020101000404
25. van Valkenhoef G, Lu G, de Brock B, Hillege H, Ades AE, Welton NJ. Automating network meta-analysis. Res Synth Methods. (2012) 3:285–99. doi: 10.1002/jrsm.1054
26. Veroniki AA, Vasiliadis HS, Higgins JP, Salanti G. Evaluation of inconsistency in networks of interventions. Int J Epidemiol. (2013) 42:332–45. doi: 10.1093/ije/dys222
27. Salanti G, Ades AE, Ioannidis JP. Graphical methods and numerical summaries for presenting results from multiple-treatment meta-analysis: an overview and tutorial. J Clin Epidemiol. (2011) 64:163–71. doi: 10.1016/j.jclinepi.2010.03.016
28. Salanti G, Del Giovane C, Chaimani A, Caldwell DM, Higgins JP. Evaluating the quality of evidence from a network meta-analysis. PLoS ONE. (2014) 9:e99682. doi: 10.1371/journal.pone.0099682
29. Thia LP, McKenzie SA, Blyth TP, Minasian CC, Kozlowska WJ, Carr SB. Randomised controlled trial of nasal continuous positive airways pressure (CPAP) in bronchiolitis. Arch Dis Childhood. (2008) 93:45–7. doi: 10.1136/adc.2005.091231
30. Yañez LJ, Yunge M, Emilfork M, Lapadula M, Alcántara A, Fernández C, et al. A prospective, randomized, controlled trial of noninvasive ventilation in pediatric acute respiratory failure. Pediatric Critic Care Med. (2008) 9:484–9. doi: 10.1097/PCC.0b013e318184989f
31. Chisti MJ, Salam MA, Smith JH, Ahmed T, Pietroni MAC, Shahunja KM, et al. Bubble continuous positive airway pressure for children with severe pneumonia and hypoxaemia in Bangladesh: an open, randomised controlled trial. Lancet. (2015) 386:1057–65. doi: 10.1016/S0140-6736(15)60249-5
32. Kepreotes E, Whitehead B, Attia J, Oldmeadow C, Collison A, Searles A, et al. High-flow warm humidified oxygen versus standard low-flow nasal cannula oxygen for moderate bronchiolitis (HFWHO RCT): an open, phase 4, randomised controlled trial. Lancet. (2017) 389:930–9. doi: 10.1016/S0140-6736(17)30061-2
33. Milési C, Essouri S, Pouyau R, Liet JM, Afanetti M, Portefaix A, et al. High flow nasal cannula (HFNC) versus nasal continuous positive airway pressure (nCPAP) for the initial respiratory management of acute viral bronchiolitis in young infants: a multicenter randomized controlled trial (TRAMONTANE study). Intensive Care Med. (2017) 43:209–16. doi: 10.1007/s00134-016-4617-8
34. Vitaliti G, Vitaliti MC, Finocchiaro MC, Di Stefano VA, Pavone P, Matin N, et al. Randomized comparison of helmet CPAP versus high-flow nasal cannula oxygen in pediatric respiratory distress. Respir Care. (2017) 62:1036–42. doi: 10.4187/respcare.05384
35. Ergul AB, Caliskan E, Samsa H, Gokcek I, Kaya A, Zararsiz GE, et al. Using a high-flow nasal cannula provides superior results to OxyMask delivery in moderate to severe bronchiolitis: a randomized controlled study. Eur J Pediatr. (2018) 177:1299–307. doi: 10.1007/s00431-018-3191-1
36. Franklin D, Babl FE, Schlapbach LJ, Oakley E, Craig S, Neutze J, et al. A randomized trial of high-flow oxygen therapy in infants with bronchiolitis. N Engl J Med. (2018) 378:1121–31. doi: 10.1056/NEJMoa1714855
37. Ramnarayan P, Lister P, Dominguez T, Habibi P, Edmonds N, Canter RR, et al. FIRST-line support for Assistance in Breathing in Children (FIRST-ABC): a multicentre pilot randomised controlled trial of high-flow nasal cannula therapy versus continuous positive airway pressure in paediatric critical care. Critical Care. (2018) 22:144. doi: 10.1186/s13054-018-2080-3
38. Sarkar M, Sinha R, Roychowdhoury S, Mukhopadhyay S, Ghosh P, Dutta K, et al. comparative study between noninvasive continuous positive airway pressure and hot humidified high-flow nasal cannulae as a mode of respiratory support in infants with acute bronchiolitis in pediatric intensive care unit of a tertiary care hospital. Indian J Critic Care Med. (2018) 22:85–90. doi: 10.4103/ijccm.IJCCM_274_17
39. Sitthikarnkha P, Samransamruajkit R, Prapphal N, Deerojanawong J, Sritippayawan S. High-flow nasal cannula versus conventional oxygen therapy in children with respiratory distress. Indian J Critic Care Med. (2018) 22:321–5. doi: 10.4103/ijccm.IJCCM_181_17
40. Vahlkvist S, Jurgensen L. la Cour A, Markoew S, Petersen TH, Kofoed PE. High flow nasal cannula and continuous positive airway pressure therapy in treatment of viral bronchiolitis: a randomized clinical trial. Eur J Pediatr. (2020) 179:513–8. doi: 10.1007/s00431-019-03533-2
41. Lal SN, Kaur J, Anthwal P, Goyal K, Bahl P, Puliyel JM. Nasal continuous positive airway pressure in bronchiolitis: a randomized controlled trial. Indian Pediatr. (2018) 55:27–30. doi: 10.1007/s13312-018-1222-7
42. World Bank. World Bank List of Economies. Washington: World Bank (2016). Available online at: https://iccmoot.com/wp-content/uploads/2016/08/World-Bank-list-of-economies_July2016.pdf
43. Gupta S, Donn SM. Continuous positive airway pressure: physiology and comparison of devices. Semin Fetal Neonatal Med. (2016) 21:204–11. doi: 10.1016/j.siny.2016.02.009
44. Alexiou S, Panitch HB. Physiology of non-invasive respiratory support. Semin Fetal Neonatal Med. (2016) 21:174–80. doi: 10.1016/j.siny.2016.02.007
45. Tiep B, Barnett M. High flow nasal versus high flow mask oxygen delivery: tracheal gas concentrations through a head extension airway model (abstract). Respir Care. (2002) 47:1079.
46. Roca O, Riera J, Torres F, Masclans JR. High-flow oxygen therapy in acute respiratory failure. Respir Care. (2010) 55:408. doi: 10.1097/CPM.0b013e3182514f29
47. Kubicka ZJ, Limauro J, Darnall RA. Heated, humidified high-flow nasal cannula therapy: yet another way to deliver continuous positive airway pressure? Pediatrics. (2008) 121:82–8. doi: 10.1542/peds.2007-0957
48. Spence KL, Murphy D, Kilian C, McGonigle R, Kilani RA. High-flow nasal cannula as a device to provide continuous positive airway pressure in infants. J Perinatol. (2007) 27:772–5. doi: 10.1038/sj.jp.7211828
49. Zhan Q, Sun B, Liang L, Yan X, Zhang L, Yang J, et al. Early use of noninvasive positive pressure ventilation for acute lung injury: a multicenter randomized controlled trial. Critic Care Med. (2012) 40:455–60. doi: 10.1097/CCM.0b013e318232d75e
Keywords: acute lower respiratory infection, BIPAP, children, CPAP, HFNC, meta-analysis, non-invasive ventilation
Citation: Wang Z, He Y, Zhang X and Luo Z (2021) Non-Invasive Ventilation Strategies in Children With Acute Lower Respiratory Infection: A Systematic Review and Bayesian Network Meta-Analysis. Front. Pediatr. 9:749975. doi: 10.3389/fped.2021.749975
Received: 30 July 2021; Accepted: 13 October 2021;
Published: 02 December 2021.
Edited by:
Kerry-Ann F. O'Grady, Queensland University of Technology, AustraliaReviewed by:
Zorica Momcilo Zivkovic, University Hospital Center Dr Dragiša Mišović, SerbiaGary Martin Doherty, Royal Belfast Hospital for Sick Children, United Kingdom
Copyright © 2021 Wang, He, Zhang and Luo. This is an open-access article distributed under the terms of the Creative Commons Attribution License (CC BY). The use, distribution or reproduction in other forums is permitted, provided the original author(s) and the copyright owner(s) are credited and that the original publication in this journal is cited, in accordance with accepted academic practice. No use, distribution or reproduction is permitted which does not comply with these terms.
*Correspondence: Zhengxiu Luo, bHVvemhlbmd4aXU4MTYmI3gwMDA0MDsxNjMuY29t
†These authors have contributed equally to this work