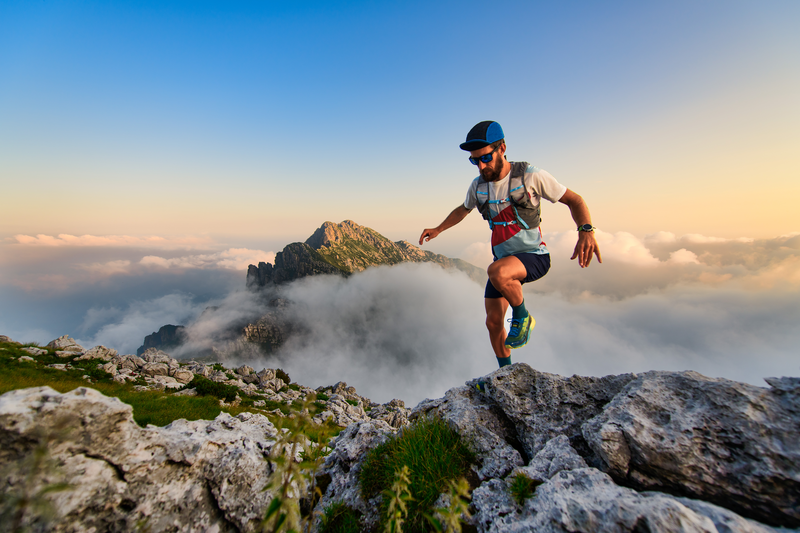
95% of researchers rate our articles as excellent or good
Learn more about the work of our research integrity team to safeguard the quality of each article we publish.
Find out more
ORIGINAL RESEARCH article
Front. Pediatr. , 23 November 2021
Sec. Neonatology
Volume 9 - 2021 | https://doi.org/10.3389/fped.2021.747509
Introduction: Maternal tobacco smoking during pregnancy is a global health problem leading to an increased risk for fetal and neonatal morbidities. So far, there are no data of the potential impact of maternal smoking during pregnancy on the most vulnerable period after birth – the immediate postnatal transition. The aim of the present study was therefore, to compare cerebral oxygenation during immediate postnatal transition in term neonates with and without prenatal tobacco exposure.
Methods: Included in this post-hoc analysis were healthy term neonates, with measurements of cerebral oxygenation (INVOS 5100C) during the first 15 min after birth, and for whom information on maternal smoking behavior during pregnancy was available. Neonates with prenatal tobacco exposure (smoking group) were matched 1:1 according to gestational age (±1 week), birth weight (±100 grams) and hematocrit (±5 %) to neonates without (non-smoking group). Cerebral regional tissue oxygen saturation (crSO2), cerebral fractional tissue oxygen extraction (cFTOE), arterial oxygen saturation (SpO2) and heart rate (HR) within the first 15 min after birth were compared between the two groups.
Results: Twelve neonates in the smoking group with a median (IQR) gestational age of 39.1 (38.8–39.3) weeks and a birth weight of 3,155 (2,970–3,472) grams were compared to 12 neonates in the non-smoking group with 39.1 (38.7–39.2) weeks and 3,134 (2,963–3,465) grams. In the smoking group, crSO2 was significantly lower and cFTOE significantly higher until min 5 after birth. HR was significantly higher in the smoking group in min 3 after birth. Beyond this period, there were no significant differences between the two groups.
Conclusion: Cerebral oxygenation within the first 5 min after birth was compromised in neonates with prenatal tobacco exposure. This observation suggests a higher risk for cerebral hypoxia immediately after birth due to fetal tobacco exposure.
Tobacco smoking during pregnancy is a global health problem with a prevalence of 1.7%, whereby the highest prevalence of 8.1% is seen in Europe. Regrettably, 30.6% of European women, who smoke daily before pregnancy, continue smoking during pregnancy (1).
Maternal tobacco smoking during pregnancy is significantly linked to fetal and neonatal morbidities and mortality. Pregnancy related complications including placental abruption or preeclampsia (2) result from impaired placental and umbilical circulation, inducing increased risk for neonatal adverse outcome (3). Prenatal tobacco exposure is further related to preterm birth (4), lower birth weight, smaller head circumference, fetal growth restriction, neurological morbidities, major congenital malformations, and sudden infant death syndrome (5–11).
Effects of prenatal tobacco exposure on neonatal cerebral oxygenation and perfusion after birth can be assessed by near-infrared spectroscopy (NIRS), which enables continuous and non-invasive monitoring of cerebral regional tissue oxygen saturation (crSO2) and cerebral fractional tissue oxygen extraction (cFTOE) by measuring oxygenated (HbO2) and deoxygenated hemoglobin (Hb) (12–15). When near-infrared light propagates through tissues, it is differently absorbed by HbO2 and Hb. Relative changes in HbO2 and Hb in a tissue can be calculated out of changes in the attenuation of light (16, 17). In an observational study in preterm neonates, who developed cerebral injury during the first week after birth, higher burden of cerebral hypoxia during the first 15 min after birth has already been observed (18). Measurement of cerebral oxygenation with NIRS during immediate postnatal transition in the delivery room is meanwhile well-established (19–24) and several factors influencing cerebral oxygenation within the first 15 min after birth have already been identified (25–31).
Prenatal tobacco exposure influences cerebral oxygenation during the first 2 days after birth in preterm neonates, who show lower crSO2 and higher cFTOE compared to neonates without prenatal tobacco exposure (32). A prospective cohort study has demonstrated a significant difference in peripheral (muscle) tissue oxygenation within the first 48 h after birth in healthy term neonates with prenatal tobacco exposure compared to neonates without (33). In this study, peripheral muscle tissue oxygenation was significantly lower and peripheral muscle fractional oxygen extraction was significantly higher in the smoking group within the first 24 h after birth.
However, so far no study has investigated the potential influence of maternal smoking during pregnancy on neonates during the most vulnerable period after birth—the immediate postnatal transition.
The aim of the present study was to evaluate the potential influence of prenatal tobacco exposure on cerebral oxygenation in clinically stable term neonates during the immediate neonatal transition after birth. Therefore, cerebral oxygenation of neonates from mothers who had smoked during pregnancy were compared to those from mothers who had not smoked during pregnancy. Based on existing studies, we hypothesized that cerebral oxygenation in neonates from mothers who had smoked during pregnancy would be compromised.
For the present study a post-hoc analysis of secondary outcome parameters of a prospective observational study (34), which was conducted at the Division of Neonatology, Department of Pediatrics and Adolescent Medicine, Medical University of Graz, Austria, between October 2015 and June 2018, was performed. Written parental consent had been obtained prior to birth and inclusion in the observational study. The study had been approved by the Regional Committee on Biomedical Research Ethics (EC numbers: 27–465 ex 14/15) and was carried out in accordance with The Code of Ethics of the World Medical Association (Declaration of Helsinki) (35).
Included in the present post-hoc analysis were term neonates, delivered by Cesarean section, with available maternal information on smoking behavior during pregnancy, including smoking yes/no and number of smoked cigarettes per day. We included only term neonates with NIRS measurements during the first 15 min after birth and with a blood gas analysis performed within the first 25 min after birth, to obtain the hematocrit value.
Exclusion criteria included major congenital anomalies as well as the need for any kind of medical support [medications, supplementary oxygen and/or respiratory support including continuous positive airway pressure (CPAP) or intubation], to eliminate any influence on cerebral oxygenation.
The neonates were stratified into two groups according to maternal smoking during pregnancy: neonates with maternal smoking were assigned to the “smoking group,” and neonates from mothers who had not smoked during pregnancy to the “non-smoking group.” Neonates in the smoking group were matched for gestational age ± 1 week and birth weight ± 100 g to healthy term neonates in the non-smoking group. In addition, groups were matched for hematocrit ± 5% to rule out a potential influence of hemoglobin on cerebral oxygenation.
Information on maternal smoking behavior during pregnancy including number of daily smoked cigarettes, maternal characteristics, antepartum medical history, and neonatal demographic data including gestational age, birth weight, sex, umbilical artery pH, Apgar scores and results of the first, routinely performed blood gas analysis were documented.
The neonates were delivered by planed Cesarean section, without labor and cord clamping was routinely delayed for 30 s after birth. Immediately after the cord was clamped the neonates were placed under an overhead heater in supine position dried and wrapped in warm towels.
NIRS measurements were performed immediately after birth under standardized conditions. The forehead was cleaned to remove vernix and amniotic fluid, as these could affect probe contact. The NIRS sensor was placed on the neonates' left fronto-parietal head and was fixed with a CPAP cap or an elastic bandage. NIRS measurements were continuously performed during the first 15 min after birth with an INVOS 5100C Cerebral/Somatic Oximeter Monitor (Medtronic, Minneapolis, MN, U.S.A.) and a neonatal transducer. cFTOE was calculated out of crSO2 and arterial oxygen saturation (SpO2) using the following formula: cFTOE = (SpO2-crSO2)/SpO2 (36). According to a quality criterion for cerebral measurements, crSO2 and SpO2 values were eliminated when crSO2 was higher than SpO2 (37).
SpO2 and heart rate (HR) were measured with pulse oximetry, applied on the neonates' right hand or wrist, using the IntelliVue MP30 monitor (Koninklijke Philips, Amsterdam, The Netherlands).
All variables were recorded continuously during the first 15 min after birth and were stored in a multichannel system (alpha-trace digital MM, B.E.S.T. Medical Systems, Vienna, Austria) for subsequent analyses. Values of crSO2 SpO2 and HR were stored every second.
Using a pneumatic cuff on the neonates' right upper arm, non-invasive blood pressure (MABP) was measured once in minute 15 after birth. The IntelliVue MP50 monitor (Koninklijke Philips, Amsterdam, The Netherlands) was used for this purpose. Rectal body temperature was also measured once in minute 15 after birth.
Hematocrit, hemoglobin and fetal hemoglobin (HbF) values as well as pH, bicarbonate, base excess, partial oxygen pressure (pO2) and partial carbon dioxide pressure (pCO2) were measured by blood gas analysis from a capillary sample within 25 min after birth. Samples were obtained by a heel-stick only at the discretion of the attending neonatologist. The analysis was performed according to local standard operating procedures with a blood gas analyzer (ABL 800 Flex, Drott, Wiener Neustadt, Austria).
Data are presented as mean and standard deviation (SD) or median and interquartile range (IQR) for continuous data and absolute and relative frequency for categorical data, respectively. Baseline differences between groups were analyzed using t-test or Mann–Whitney U-test for and Chi-square test, as appropriate. A linear mixed model with fixed effects for time and nicotine use and interaction of time and nicotine use applying a first-order autoregressive covariance structure was used for calculation of overall effects and differences between groups at each minute. Course of parameters were analyzed starting with the third minute after birth until minute 15. The first 2 min of life were not analyzed due to the high number of missing values. For the visualization of the courses of analyzed parameters, estimated values according to the linear mixed model with 95% confidence intervals (95% CI) are shown. A p-value of p < 0.05 was considered statistically significant. Statistical analyses were performed using SPSS 26.0 (SPSS, Chicago, IL, USA).
Eligible were 158 term neonates, who were included in the prospective observational NIRS study. Eighteen neonates were stratified to the smoking group and 140 to the non-smoking group. Two neonates in the smoking group and 25 neonates in the non-smoking group were excluded due to the need for medical support, including supplementary oxygen and/or respiratory support. None of the neonates in the smoking group and three neonates in the non-smoking group were excluded as no NIRS measurements were available within the first 15 min after birth. Three neonates in the smoking group and 29 neonates in the non-smoking group had no blood gas analysis performed within the first 25 min after birth and were therefore excluded. Furthermore, one neonate in the smoking group and 71 neonates in the non-smoking group were excluded, as no matching according to gestational age, birth weight and hematocrit was possible. Finally, 12 neonates in the smoking group and 12 neonates in the non-smoking group fulfilled inclusion and matching criteria (Figure 1).
Figure 1. Study flow chart showing the number of included term neonates and rationales for exclusion.
There were no differences in maternal characteristics including maternal age at the time of delivery [31.0 (27.2–35.3) years in the smoking group and 31.0 (26.6–34.2) years in the non-smoking group; p = 0.743] and parturition [2.0 (1.6–2.8) in the smoking group and 1.0 (1.1–2.1) in the non-smoking group; p = 0.114]. Mothers in the smoking group had a body-mass index of 31.8 ± 8.4 and in the non-smoking group 28.3 ± 6.0; p = 0.387. In both groups, there were no severe preexisting maternal morbidities. Except for medication treating hypothyroidism (smoking group n = 2; non-smoking group n = 4), the mothers had no additional medications. The mothers in the smoking group reported to smoke median (IQR) 8 (4–10) cigarettes per day.
Indications for the Cesarean sections were previous Cesarean section (n = 7), breech position (n = 3), and maternal request (n = 2) in the smoking group and previous Cesarean section (n = 6), breech position (n = 3), maternal request (n = 2), and preeclampsia (n = 1) in the non-smoking group. Anesthesia during Cesarean section was in all cases epidural anesthesia, except of one mother in the smoking group, who received general anesthesia.
Neonatal characteristics and results of capillary blood samples are presented in Table 1. Sampling time for blood gas analyses was in median (IQR) in minute 16.0 (15.0–16.5) in the smoking group and 16.5 (15.0–18.0) in the non-smoking group.
Table 1. Characteristics of term neonates from mothers who had smoked during pregnancy and in term neonates from mothers who had not smoked during pregnancy (smoking group and non-smoking group).
The courses of crSO2 and cFTOE during the first 15 min after birth are demonstrated for the two groups in Table 2; Figures 2A,B. crSO2 was significantly lower until min 5 after birth (in both groups: min 3: n = 11, min 4: n = 12, min 5: n = 11) and cFTOE was significantly higher until min 5 after birth (in both groups: min 3: n = 10, min 4: n = 11, min 5: n = 11) in the smoking group compared to the non-smoking group. SpO2 and HR during the first 15 min after birth are demonstrated in Table 3; Figures 2C,D. There were no significant differences between the two groups in SpO2. HR was significantly higher in the smoking group in min 3 after birth compared to the non-smoking group. From min 4 until 15, there were no further significant differences between the two groups.
Table 2. Cerebral regional oxygen saturation (%) and cerebral fractional tissue oxygen extraction in term neonates from mothers who had smoked during pregnancy compared to term neonates from mothers who had not smoked during pregnancy (smoking group and non-smoking group).
Figure 2. (A–D) crSO2, cFTOE, SpO2 and HR of term neonates from mothers who had smoked during pregnancy (light blue spots) compared to term neonates from mothers who had not smoked during pregnancy (dark blue spots).
Table 3. Arterial oxygen saturation (%) and heart rate (bpm) in term neonates from mothers who had smoked during pregnancy compared to term neonates from mothers who had not smoked during pregnancy (smoking group and non-smoking group).
To the best of our knowledge, this study is the first investigating a potential influence of maternal smoking during pregnancy on cerebral oxygenation during the immediate neonatal transition after birth in healthy term neonates. We found that cerebral oxygenation within the first 5 min after birth was lower in infants whose mothers had smoked during pregnancy compared to those of the non-smoking group. These results support our hypothesis that maternal smoking during pregnancy is associated with compromised cerebral oxygenation immediately after birth.
In neonates from mothers who had smoked during pregnancy, polyglobulia (38) and higher HbF values (39) have been reported. These factors may influence cerebral oxygenation, as cFTOE correlates negatively with total hemoglobin concentration. In our study, term neonates with prenatal tobacco exposure were therefore matched for hematocrit value. Moreover, there were no differences in HbF between the two groups. This suggests that other mechanisms have to be responsible for the observed differences in cerebral oxygenation during the immediate neonatal transition after birth.
Cerebral oxygenation immediately after birth in those neonates from mothers who had smoked during pregnancy may display the fetal conditions in utero. Nicotine moderates the release of catecholamines from adrenal glands and nerve cells, leading to vasoconstriction in placental and umbilical vessels (40, 41). Tobacco smoking also induces morphological changes in the placenta with infarcts and calcifications, again causing utero-placental vasoconstriction (42). This might be associated with placental insufficiency and chronic fetal hypoxia due to compromised oxygen delivery and nutrients supply to the fetus (38). However, as we did not find any differences in SpO2 and pO2 between the two groups, systemic arterial hypoxia in the smoking group can be ruled out as a cause of the impaired cerebral oxygenation in the smoking group.
HR was significantly higher in min 3 and a trend toward higher values in min 4 after birth in the smoking group was observed in the present study. This is in accordance with reports of increased maternal and fetal HR due to tobacco exposure (38, 43). An explanation for the increased HR is the nicotine induced catecholamine release (42). This effect may probably persist in the first minutes after birth and explains the higher HR in the smoking group. The increased HR in the smoking group would suggest increased cardiac output with improved cerebral perfusion and, thus, cerebral oxygenation. However, in the present study we observed lower crSO2 and higher cFTOE in the smoking group, suggesting—in the case of similar oxygen content of the blood (similar hemoglobin level and SpO2/pO2 levels in both groups)—impaired cerebral perfusion due to vasoconstriction and/or impairment of cerebral autoregulatory capacity in the first minutes after birth.
An increase in cerebral blood flow velocity between 20 and 42 h after birth has already been described in newborn infants from mothers who had smoked during pregnancy (32, 44). This caused an impairment in cerebral perfusion due to changes in cerebrovascular resistance, leading to higher cFTOE (32, 44). This increase of cFTOE and decrease of crSO2 was observed by Verhagen et al. (32) in preterm neonates with prenatal tobacco exposure during the first two days as well as on day eight after birth. On the first day after birth, the median crSO2 was 73 vs. 81% and cFTOE was 0.24 vs. 0.15 when comparing a smoking group to a non-smoking group, respectively (32). We can add to this data, as in the present study crSO2 was significantly lower in the first 5 min after birth in the smoking group, with a trend toward a lower cerebral oxygenation up to minute ten. When comparing the maximum differences of crSO2 and cFTOE of the smoking vs. the non-smoking group from Verhagen et al. (32) on the first day after birth and our results at min 3 after birth (crSO2 41.4% smoking vs. 54.7% non-smoking group; cFTOE 0.42 vs. 0.30), our study revealed an even more pronounced difference in cerebral oxygenation.
We have some limitations to acknowledge. First, the number of cigarettes smoked during pregnancy may be underreported, since they were self-reported by the mothers using a questionnaire; moreover, some mothers in the non-smoking group could have neglected smoking during pregnancy. We did not perform measurements of nicotine levels in hair or urine, which would have informed us on the actual tobacco consumption. Second, background factors that might be associated with smoking were not collected/documented and may have had an impact on the reported results. Third, the number of included neonates in this study is small, therefore interpretation of these results has to be performed with caution. However, the main reason for the small sample size was the strict matching criteria to obtain comparable groups and even in this small cohort the results were not only significantly different, but also differences between groups were of clinical relevance. Matching according to birth weight was performed to rule out any potential influence of fetal growth restriction due to smoking on cerebral oxygenation. Therefore, the matching allowed us to achieve homogeneity in birth weight between the two groups.
Immediately after birth, cerebral oxygenation was compromised with lower crSO2 and higher cFTOE values in stable term neonates delivered by Cesarean section from mothers who had smoked during pregnancy, compared to neonates without prenatal tobacco exposure. We assume that in the included stable term neonates, with an inconspicuous postnatal transition period, the observed lower cerebral oxygenation in the smoking group might be of questionable clinical relevance. However, our results suggest that neonates with prenatal tobacco exposure are at a higher risk of cerebral hypoxia immediately after birth. This could be even more pronounced in compromised term neonates and/or preterm neonates potentially causing cerebral injury. This observation should be implemented in the education about the harmful effects of tobacco smoking especially for women of childbearing age and those being pregnant.
The original contributions presented in the study are included in the article/supplementary material, further inquiries can be directed to the corresponding author/s.
The studies involving human participants were reviewed and approved by Regional Committee on Biomedical Research Ethics, Medical University of Graz. Written informed consent to participate in this study was provided by the participants' legal guardian/next of kin.
CW and GP conceived the research idea and finalized the methods. CW, GP, and AA analyzed the data and CW wrote the first draft. CW, MB, BS, LM, EP, NH, BU, and GP contributed to data collection, interpretation of the results, and drafting and finalizing the manuscript. All authors contributed to the article and approved the submitted version.
The authors declare that the research was conducted in the absence of any commercial or financial relationships that could be construed as a potential conflict of interest.
All claims expressed in this article are solely those of the authors and do not necessarily represent those of their affiliated organizations, or those of the publisher, the editors and the reviewers. Any product that may be evaluated in this article, or claim that may be made by its manufacturer, is not guaranteed or endorsed by the publisher.
We would like to express our gratitude to the parents for allowing us to investigate their infants, as well as to all the nurses, physicians and midwives involved in the treatment of these infants. We also want to thank Evelyn Ziehenberger for her technical assistance to perform this study.
1. Lange S, Probst C, Rehm J, Popova S. National, regional, and global prevalence of smoking during pregnancy in the general population: a systematic review and meta-analysis. Lancet Glob Health. (2018) 6:e769–76. doi: 10.1016/S2214-109X(18)30223-7
2. DiFranza JR, Lew RA. Effect of maternal cigarette smoking on pregnancy complications and sudden infant death syndrome. J Fam Pract. (1995) 40:385–94.
3. Morrow RJ, Ritchie JW, Bull SB. Maternal cigarette smoking: the effects on umbilical and uterine blood flow velocity. Am J Obstet Gynecol. (1988) 159:1069–71. doi: 10.1016/0002-9378(88)90415-2
4. Walsh RA. Effects of maternal smoking on adverse pregnancy outcomes: examination of the criteria of causation. Hum Biol. (1994) 66:1059–62.
5. Higgins S. Smoking in pregnancy. Curr Opin Obstet Gynecol. (2002) 14:145–51. doi: 10.1097/00001703-200204000-00007
6. Mitchell EA, Thompson JM, Robinson E, Wild CJ, Becroft DMO, Clark PM, et al. Smoking, nicotine and tar and risk of small for gestational age babies. Acta Paediatrc. (2002) 91:323–8. doi: 10.1111/j.1651-2227.2002.tb01723.x
7. Horta BL, Victoria CG, Menezes AM, Halpern R, Barros FC. Low birthweight, preterm births and intrauterine growth retardation in relation to maternal smoking. Paediatr Perinat Epidemiol. (1997) 11:140–51. doi: 10.1046/j.1365-3016.1997.d01-17.x
8. Dempsey DA, Benowitz NL. Risks and benefits of nicotine to aid smoking cessation in pregnancy. Drug Saf. (2001) 24:277–322. doi: 10.2165/00002018-200124040-00005
9. Rogers JM. Tobacco and pregnancy: overview of exposure and effects. Birth Defects Res C Embryo Today. (2008) 84:1–15. doi: 10.1002/bdrc.20119
10. Herrmann M, King K, Weitzman M. Prenatal tobacco smoke and postnatal secondhand smoke exposure and child neurodevelopment. Curr Opin Pediatr. (2008) 20:184–90. doi: 10.1097/MOP.0b013e3282f56165
11. Werler MM, Sheehan JE, Mitchell AA. Association of vasoconstrictive exposures with risks of gastroschisis and small intestinal atresia. Epidemiology. (2003) 14:349–54. doi: 10.1097/01.EDE.0000059226.36219.DE
12. Jobsis FF. Noninvasive, infrared monitoring of cerebral and myocardial oxygen sufficiency and circulatory parameters. Science. (1977) 198:1264–7. doi: 10.1126/science.929199
13. Ferrari M, Mottola L, Quaresima V. Principles, techniques, and limitations of near infrared spectroscopy. Can J Appl Physiol. (2004) 29:463–87. doi: 10.1139/h04-031
14. Dullenkopf A, Kolarova A, Schulz G, Frey B, Baenziger O, Weiss M. Reproducibility of cerebral oxygenation measurement in neonates and infants in the clinical setting using the NIRO 300 oximeter. Pediatr Crit Care Med. (2005) 6:344–7. doi: 10.1097/01.PCC.0000161282.69283.75
15. Urlesberger B, Pichler G, Gradnitzer E, Reiterer F, Zobel G, Müller W. Changes in cerebral blood volume and cerebral oxygenation during periodic breathing in term infants. Neuropediatrics. (2000) 31:75–81. doi: 10.1055/s-2000-7477
16. Wolfberg AJ, du Plessis AJ. Near-infrared spectroscopy in the fetus and neonate. Clin Perinatol. (2006) 33:707–28, viii. doi: 10.1016/j.clp.2006.06.010
17. Wolf M, Greisen G. Advances in near-infrared spectroscopy to study the brain of the preterm and term neonate. Clin Perinatol. (2009) 36:807–34, vi. doi: 10.1016/j.clp.2009.07.007
18. Baik N, Urlesberger B, Schwaberger B, Schmölzer GM, Avian A, Pichler G. Cerebral haemorrhage in preterm neonates: does cerebral regional oxygen saturation during the immediate transition matter? Arch Dis Child Fetal Neonatal Ed. (2015) 100:F422–7. doi: 10.1136/archdischild-2014-307590
19. Urlesberger B, Grossauer K, Pocivalnik M, Avian A, Müller W, Pichler G. Regional oxygen saturation of the brain and peripheral tissue during birth transition of term infants. J Pediatr. (2010) 157:740–4. doi: 10.1016/j.jpeds.2010.05.013
20. Kratky E, Pichler G, Rehak T, Avian A, Pocivalnik M, Müller W, et al. Regional cerebral oxygen saturation in newborn infants in the first 15 min of life after vaginal delivery. Physiol Meas. (2012) 33:95–102. doi: 10.1088/0967-3334/33/1/95
21. Noori S, Wlodaver A, Gottipati V, McCoy M, Schultz D, Escobedo M. Transitional changes in cardiac and cerebral hemodynamics in term neonates at birth. J Pediatr. (2012) 160:943–8. doi: 10.1016/j.jpeds.2011.12.008
22. Binder C, Urlesberger B, Avian A, Pocivalnik M, Müller W, Pichler G. Cerebral and peripheral regional oxygen saturation during postnatal transition in preterm neonates. J Pediatr. (2013) 163:394–9. doi: 10.1016/j.jpeds.2013.01.026
23. Pichler G, Binder C, Avian A, Beckenbach E, Schmölzer GM, Urlesberger B. Reference ranges for regional cerebral tissue oxygen saturation and fractional oxygen extraction in neonates during immediate transition after birth. J Pediatr. (2013) 163:1558–63. doi: 10.1016/j.jpeds.2013.07.007
24. Bruckner M, Pichler G, Urlesberger B. NIRS in the fetal to neonatal transition and immediate postnatal period. Semin Fetal Neonatal Med. (2020) 25:101079. doi: 10.1016/j.siny.2020.101079
25. Wolfsberger CH, Bruckner M, Baik-Schneditz N, Schwaberger B, Mileder LP, Avian A, et al. Fetal inflammatory response syndrome and cerebral oxygenation during immediate postnatal transition in preterm neonates. Front Pediatr. (2020) 8:401. doi: 10.3389/fped.2020.00401
26. Matterberger C, Baik-Schneditz N, Schwaberger B, Schmölzer GM, Mileder L, Pichler-Stachl E, et al. Blood glucose and cerebral tissue oxygenation immediately after birth – an observational study. J Pediatr. (2018) 200:19–23. doi: 10.1016/j.jpeds.2018.05.008
27. Willfurth I, Baik-Schneditz N, Schwaberger B, Mileder L, Schober L, Urlesberger B, et al. Cerebral oxygenation in neonates immediately after cesarean section and mode of maternal anesthesia. Neonatology. (2019) 116:132–9. doi: 10.1159/000499046
28. Bruckner M, Mileder LP, Richter A, Baik-Schneditz N, Schwaberger B, Binder-Heschl C, et al. Association between regional tissue oxygenation and body temperature in term and preterm infants born by caesarean section. Children. (2020) 7:205. doi: 10.3390/children7110205
29. Baik-Schneditz N, Pichler G, Schwaberger B, Binder-Heschl C, Mileder L, Reiss IKH, et al. Effect of intrauterine growth restriction on cerebral regional oxygen saturation in preterm and term neonates during immediate postnatal transition. Neonatology. (2020) 117:324–330. doi: 10.1159/000507583
30. Baik-Schneditz N, Urlesberger B, Schwaberger B, Mileder L, Schmölzer G, Avian A, et al. Tactile stimulation during neonatal transition and its effect on vital parameters in neonates during neonatal transition. Acta Pediatr. (2018) 107:952–7. doi: 10.1111/apa.14239
31. Urlesberger B, Brandner A, Pocivalnik M, Koestenberger M, Morris N, Pichler G. A left-to-right shunt via the ductus arteriosus is associated with increased regional cerebral oxygen saturation during neonatal transition. Neonatology. (2013) 103:259–63. doi: 10.1159/000346384
32. Verhagen EA, Ter Horst HJ, Kooi EM, Keating P, van den Berg PP, Bos AF. Prenatal tobacco exposure influences cerebral oxygenation in preterm infants. Early Hum Dev. (2011) 87:401–6. doi: 10.1016/j.earlhumdev.2011.03.002
33. Pichler G, Heinzinger J, Klaritsch P, Zotter H, Muller W, Urlesberger B. Impact of smoking during pregnancy on peripheral tissue oxygenation in term neonates. Neonatology. (2008) 93:132–7. doi: 10.1159/000108408
34. Bresesti I, Bruckner M, Mattersberger C, Baik-Schneditz N, Schwaberger B, Mileder L, et al. Feasibility of transcutaneous pCO2 monitoring during immediate transition after birth – a prospective observational study. Front Pediatr. (2020) 8:11. doi: 10.3389/fped.2020.00011
35. World Medical Association. World medical association declaration of Helsinki: ethical principles for medical research involving human subjects. JAMA. (2013) 310:2191–4. doi: 10.1001/jama.2013.281053
36. Naulaers G, Meyns B, Miserez M, Leunens V, Van Huffel S, Casaer P, et al. Use of tissue oxygenation index and fractional oxygen extraction as non-invasive parameters for cerebral oxygenation. A validation study in piglets. Neonatology. (2007) 92:120–6. doi: 10.1159/000101063
37. Pocivalnik M, Pichler G, Zotter H, Tax N, Müller W, Urlesberger B. Regional tissue oxygen saturation: comparability and reproducibility of different devices. J Biomed Opt. (2011) 16:057004. doi: 10.1117/1.3575647
38. Habek D, Habek JC, Ivanisevic M, Djelmis J. Fetal tobacco syndrome and perinatal outcome. Fetal Diagn Ther. (2002) 17:367–71. doi: 10.1159/000065387
39. Cochran-Black DL, Cowan LD, Neas BR. The relation between newborn hemoglobin F fractions and risk factors for sudden infant death syndrome. Arch Pathol Lab Med. (2001) 125:211–7. doi: 10.5858/2001-125-0211-TRBNHF
40. Atluri P, Fleck MW, Shen Q, Mah SJ, Stadfelt D, Barnes W, et al. Functional nicotine acetylcholine receptor expression in stem and progenitor cells of the early embryonic mouse cerebral cortex. Dev Biol. (2001) 240:143–56. doi: 10.1006/dbio.2001.0453
41. Wickström R. Effects of nicotine during pregnancy: human and experimental evidence. Curr Neuropharmacol. (2007) 5:213–22. doi: 10.2174/157015907781695955
42. Jauniaux E, Burton GJ. Morphological and biological effects of maternal exposure to tobacco smoke on the feto-placental unit. Early Hum Dev. (2007) 83:699–706. doi: 10.1016/j.earlhumdev.2007.07.016
43. Tulzer G, Bsteh M, Arzt W, Tews G, Schmitt K, Huhta JC. Acute effects of cigarette smoking on fetal cardiovascular and uterine Doppler parameters. Geburtshilfe Frauenheilkd. (1993) 53:689–92. doi: 10.1055/s-2007-1023610
Keywords: term neonates, immediate neonatal resuscitation, near infrared spectroscopy, NIRS, cerebral oxygenation, maternal smoking, prenatal tobacco exposure, nicotine
Citation: Wolfsberger CH, Bruckner M, Schwaberger B, Mileder LP, Pritisanac E, Hoeller N, Avian A, Urlesberger B and Pichler G (2021) Increased Risk for Cerebral Hypoxia During Immediate Neonatal Transition After Birth in Term Neonates Delivered by Caesarean Section With Prenatal Tobacco Exposure. Front. Pediatr. 9:747509. doi: 10.3389/fped.2021.747509
Received: 26 July 2021; Accepted: 02 November 2021;
Published: 23 November 2021.
Edited by:
Helmut Dietmar Hummler, University of Tübingen, GermanyReviewed by:
Hans Fuchs, University of Freiburg Medical Center, GermanyCopyright © 2021 Wolfsberger, Bruckner, Schwaberger, Mileder, Pritisanac, Hoeller, Avian, Urlesberger and Pichler. This is an open-access article distributed under the terms of the Creative Commons Attribution License (CC BY). The use, distribution or reproduction in other forums is permitted, provided the original author(s) and the copyright owner(s) are credited and that the original publication in this journal is cited, in accordance with accepted academic practice. No use, distribution or reproduction is permitted which does not comply with these terms.
*Correspondence: Gerhard Pichler, Z2VyaGFyZC5waWNobGVyQG1lZHVuaWdyYXouYXQ=
Disclaimer: All claims expressed in this article are solely those of the authors and do not necessarily represent those of their affiliated organizations, or those of the publisher, the editors and the reviewers. Any product that may be evaluated in this article or claim that may be made by its manufacturer is not guaranteed or endorsed by the publisher.
Research integrity at Frontiers
Learn more about the work of our research integrity team to safeguard the quality of each article we publish.