- 1Department of Inmunobioquímica, Instituto Nacional de Perinatología (INPer), Ciudad de México, Mexico
- 2Department of Neonatología, Instituto Nacional de Perinatología (INPer), Mexico City, Mexico
- 3Department of Fisiología y Desarrollo Celular, Instituto Nacional de Perinatología (INPer), Mexico City, Mexico
- 4Instituto Nacional de Pediatría, Mexico City, Mexico
- 5Servicio Recién Nacidos, Hospital Regional Lic. Adolfo López Mateos, Instituto de Seguridad y Servicios Sociales de los Trabajadores del Estado (ISSSTE), Mexico City, Mexico
- 6Department of Pharmacology, Emory University School of Medicine, Atlanta, GA, United States
Background: Extracellular heat-shock proteins (eHsp) are highly conserved molecules that play an important role in inflammatory diseases and have been quantified in plasma from patients with infectious diseases, including sepsis. There is a constant search for dependable biochemical markers that, in combination with conventional methods, could deliver a prompt and reliable diagnosis of early-onset neonatal sepsis.
Objective: We sought to assess the level of eHsp-27, eHsp-60, eHsp-70, and tumor necrosis factor-alpha (TNFα) in plasma of healthy neonates at term and infants with early-onset neonatal sepsis.
Methods: This study included 34 newborns that were classified as healthy neonates at term (blood samples from the umbilical cord, n = 23) or infants with early-onset neonatal sepsis (blood samples obtained from umbilical artery by standard sterile procedures before starting a systemic antibiotic intervention, n = 11). All blood samples were centrifuged, and the plasma recovered to determine eHsp-27, eHsp-60, eHsp-70, and TNFα levels by ELISA.
Results: Our results indicate that the level of eHsp-27 in healthy neonates at term was 0.045 ± 0.024 pg/ml. This value decreased 2.5-fold in infants with early-onset neonate sepsis (0.019 ± 0.006 pg/ml, p = 0.004). In contrast, the levels of eHsp-60 and eHsp-70 in healthy neonates at term were 13.69 ± 5.3 and 4.03 ± 2.6 pg/ml, respectively. These protein levels increased significantly 1.8- and 1.9-fold in the plasma of infants with early-onset neonatal sepsis (p ≤ 0.001). The level of TNFα in healthy neonates at term was 2.94 ± 0.46 pg/ml, with a 3.0-fold increase in infants with early-onset neonatal sepsis (8.96 ± 0.72 pm/ml, p ≤ 0.001). The sensitivity, specificity, positive predictive value (PPV), and negative predictive value (NPV) of eHsp compared with that of C-reactive protein were 73.3, 60.0, 47.8, and 33.3%, respectively.
Conclusion: This study demonstrated a consistent increase of eHsp-60 and eHsp-70 in the plasma of infants diagnosed with early-onset neonatal sepsis. These proteins showed higher sensitivity and specificity than C-reactive protein and blood culture test.
Introduction
Extracellular heat-shock proteins (eHsp) are highly conserved molecules that regulate cellular homeostasis (1, 2), proliferation, and differentiation of the professional immune system cells and are modulated by temperature (3–5). The eHsp have been classified in high molecular weight of 60, 70, 90, and 100 kDa and low molecular weight of 20 and 27 kDa (6, 7). When released into the extracellular space, eHsp function as cell-to-cell mediators (8, 9). eHsp-60 (HSPD1; heat shock protein family D member 1) and eHsp-70 (HSPA1A; heat shock protein family A member 1A) can stimulate pro-inflammatory cytokines (10, 11), whereas eHsp-27 (HSPB1; heat shock protein family B (small) member 1) has an important anti-inflammatory function (12–14). Their presence has also been shown and their levels quantified in serum and plasma of patients with severe trauma (15, 16), chronic obstructive pulmonary disease (17, 18), inflammatory processes induced by multiple sclerosis (19), and sepsis (20, 21). Therefore, eHsp have been used as sensible indicators of the physiological status during the onset and resolution of different human pathological conditions (19, 22, 23).
Neonatal sepsis is a common and serious disease that affects a large number of newborns around the world. Although its incidence is low (one to eight cases for every 1,000 live births) (24), the risk of morbidity and mortality is high, affecting 15–50% of reported cases (24, 25). In developed countries, the estimated prevalence is 2–8% (24). The Department of Neonatal Intensive Care of the National Institute of Perinatology “Isidro Espinosa de los Reyes” (INPerIER) in Mexico City has reported an incidence of 2.3% in the total number of births attended during a 5-year period (26).
The clinical diagnosis of early-onset neonatal sepsis poses challenges due to the subtlety of signs and symptoms, which are often concealed with other transient medical conditions such as hypothermia, delayed transition from fetal to neonatal life, tachypnea, and metabolic alterations (25). The clinical identification and diagnosis of neonatal sepsis is confirmed by blood culture (27, 28), and the assessment of acute phase reactants includes C-reactive protein (CRP) (29, 30), procalcitonin (30, 31), presepsin (32, 33), and inflammatory mediators such as interleukin-6 (IL-6) and tumor necrosis factor-alpha (TNFα) (34, 35). It has been shown that, when two or more tests are combined, the accuracy of a prompt diagnosis of early-onset neonatal sepsis increases (36, 37). At INPerIER, some of these acute phase reactants or biological markers have not provided the accuracy and sensitive to support clinical data in the diagnosis of early-onset neonatal sepsis. Interestingly, several reports have shown that eHsp are reliable and practical biomarkers to identify sepsis in children (21, 38). In this study, we set out to quantify the eHsp and TNFα in plasma of healthy neonates at term and infants with early-neonatal sepsis.
Materials and Methods
Ethics Statements
This study was reviewed and approved by the National Institute of Perinatology Ethics and Research Committees (registration number 212250-3210101). All patients were informed about the purpose of the study and a maternal informed consent obtained in all cases.
Study Design and Patients
From July 2018 to June 2019, a cross-sectional study was carried out in the Neonatal Intermediate Therapy Unit for the newborn. A total of 34 newborns were included. The neonates were divided into two groups: (1) healthy neonates at term (blood samples obtained at birth from the umbilical cord, n = 23) and (2) neonates with visible signs of early-onset neonatal sepsis (blood samples obtained from umbilical artery by standard sterile procedures before starting a systemic antibiotic intervention; n = 11).
Clinical Definitions and Inclusion Criteria
Healthy neonates consisted of neonates at term, gestational age ≥37 weeks, delivery without obstetric complications of labor and/or signs of maternal sepsis. Neonates with early-onset neonatal sepsis consisted of infants with visible signs and symptoms (feeding intolerance, lethargic or tachypnic, poor perfusion, seizures, respiratory distress, bradycardia, abdominal distension, or vomiting) normally associated with suspected sepsis as defined according to the guidelines for the management of newborns with suspected sepsis (39–41).
Some of the maternal patients presented the following conditions: preterm rupture of fetal membranes (pPROM), which was diagnosed by discharge of amniotic fluid through the vaginal canal or by a positive nitrazine test (42); clinical chorioamnionitis (CAM) was diagnosed by the presence of fever (>38°C), accompanied by two or more of the following signs: tachycardia (heart rate >100 beats per min), uterine pain or tenderness, fetid or purulent amniotic fluid, leukocytosis >15,000/mm3, CRP (>2 mg/dl), and fetal tachycardia (heart rate >160 beats per minute) (43–45).
Exclusion Criteria
Neonatal sepsis cases were excluded from the study when (1) the amount of blood collected for the quantification of eHsp and TNFα was insufficient (plasma < 1,200) and (2) antibiotic treatments started prior to blood collection.
Blood Sample Collection
Two milliliters of blood were obtained by trained medical staff. The blood samples were collected in K2-EDTA vacutainer tubes (Becton-Dickinson, NJ, USA) and centrifuged at 329 g (Beckman, GS-6R Centrifuge) for 5 min. The plasma was recovered in Eppendorf tubes and stored at −80°C until quantification of the eHsp-27, eHsp-60, eHsp-70, and TNFα by enzyme-linked immunosorbent assay (ELISA).
Biochemical Assays
Commercial ELISA kits were used to quantify the levels of eHsp-27 (DYC-1580, R&D Systems, Minneapolis, MN, USA), eHsp-60 (DYC1800-2, R&D Systems), eHsp-70 (DYC1663-2, R&D System), and TNFα (DY210, R&D System). The plasma used for the quantified was not diluted. Standard curves were calculated from 31.3 to 2,000 ng/ml, 1.25 to 80 ng/ml, 312.5 to 20,000 pg/ml, and 15.0 to 960 pg/ml, respectively, according to the manufacturer's instructions and the protocol previously reported by our research group (46). The following sensitivity values for each protein were calculated 50, 0.70, 150.0, and 5.0 pg/ml, respectively. The CRP levels were determined a few hours after the blood was obtaining. CRP was measured by nephelometry using a MININEPH PLUS System (Birmingham, UK) and with commercial kit (ZK044.L.R, Birmingham, UK) according to the manufacturer's instructions. CRP assay has a detection range of 6 to 1,232 mg/L and Inter- and Intra-Assay Coefficients of Variability <4% both at low and high concentration. CRP was processed at INPerIER core facility.
Microbiological Analysis
Bacterial culture analysis and identification test for aerobic and anaerobic microorganisms were performed at the Department of Infectología e Inmunología at the INPerIER. The procedure was performed with the Bact/Alert 3D (Dirham, BioMerieux, NC, USA) as previously reported by Thorpe et al. (47).
Statistical Analysis
Chi-square test was used to compare maternal and neonatal clinical data. eHsp and TNFα levels in healthy neonates at term and infants with early-onset neonatal sepsis were analyzed using one-way ANOVA and significant difference between groups were determined by the Tukey's test. All assays were independently replicated at least three times, and the data are presented as mean ± SEM. Statistical analyses were carried out using SigmaStat software (version 3.0). A significant difference was accepted at p ≤ 0.05.
Results
Demographic Data of the Study Population
Table 1 shows the demographic and clinical characteristics of maternal and neonatal patients included in this study. In maternal patients, no significant difference between groups was detected in age (p = 0.423), body mass index (p = 0.927), and number of neonates delivered alive during the first three pregnancies, but a significant difference during the fourth pregnancy (9.0 vs. 0.0%, p = 0.013) was found.
Maternal patients who delivered infants with early-onset neonatal sepsis developed clinical CAM in 36.0% of cases, whereas pPROM occurred in 27.0%. Only 9.0% presented CAM and pPROM simultaneously. Furthermore, 27.0% of maternal patients showed clinical preeclampsia (PE) and 18.0% of them had severe PE (Table 1) and 8.3% of newborns had intrauterine growth restriction (Sample 5, Table 2).
In neonatal patients, we found significant differences between groups. Infants with early-onset neonate sepsis showed 1.2-fold decrease in gestational age compared with healthy neonates at term (38.6 ± 1.1 weeks; p ≤ 0.001); 2.15-fold decreased in body weight at birth (2,970.5 ± 441.0 vs. 1,380 ± 804.8 kg; p = 0.006), and 82% of infants with early-onset neonatal sepsis showed APGAR < 8 at 5 min. No gender-based difference was found (Table 1).
Microbiological Analysis
Table 2 shows the bacteria detected in blood culture, maternal diagnosis, and evidence of sepsis. Blood culture was positive in 27.3% of samples taken from infants with early-onset neonatal sepsis (three of 11 cases). The bacteria identified in these samples were E. coli (1 case, S2), E. epidermidis (1 case, S6), and S. dysgalactiae (1 case, S8). Blood cultures of samples taken from healthy neonates at term were negative (Table 2).
Extracellular Heat-Shock Proteins and Inflammatory Cytokine in Plasma
To assess whether blood from different sampling sites does not affect the quantification of eHsp and TNFα, blood samples from both the umbilical cord and the umbilical artery from five different healthy neonates at term were collected. All samples were assessed for eHsp and TNFα. No differences were found (Figure 1) between sampling sites for eHsp-27 (0.051 ± 0.004 vs. 0.04 ± 0.005, p = 0.6851), eHsp-60 (14.4 ± 0.79 vs. 15.1 ± 1.02, p = 0.1958), eHsp-70 (4.08 ± 0.31 vs. 4.22 ± 0.55, p = 0.6396), and TNFα (3.3 ± 0.50 vs. 3.7 ± 0.72, p = 0.3207).
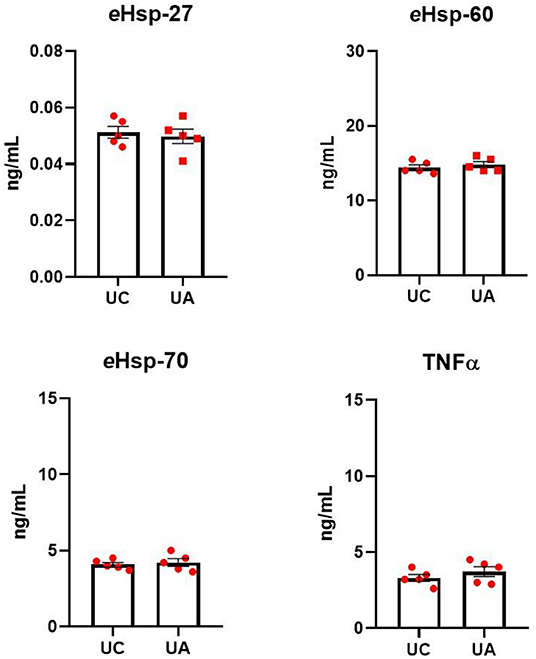
Figure 1. Quantification of eHsp-27, eHsp-60, eHsp-70, and TNFα in plasma from blood samples collected from the umbilical cord (UC) and the umbilical artery (UA) in healthy neonates at term (n = 5). Concentration was expressed as ng/ml. Data represent the mean ± SEM.
Figure 2 shows the levels of eHsp-27, eHsp-60, eHsp-70, and TNFα in the plasma of healthy neonates at term and infants with early-onset neonatal sepsis. The levels of eHsp-27 decreased 2.2-fold in infants with early-onset neonatal sepsis compared with healthy neonates at term (0.045 ± 0.02 vs. 0.019 ± 0.006 pg/ml, p = 0.008; Figure 2). In contrast, the levels of eHsp-60, eHsp-70, and TNFα increased significantly in all infants with early-onset neonatal sepsis compared with healthy neonates at term 1.6-fold (14.15 ± 5.7 vs. 24.7 ± 3.0 pg/ml, p ≤ 0.001), 2.0-fold (4.03 ± 2.6 vs. 7.9 ± 0.62 pg/ml, p ≤ 0.001), and 3.0-fold (2.94 ± 0.46 vs. 8.96 ± 0.72 pg/ml, p ≤ 0.001), respectively (Figure 2).
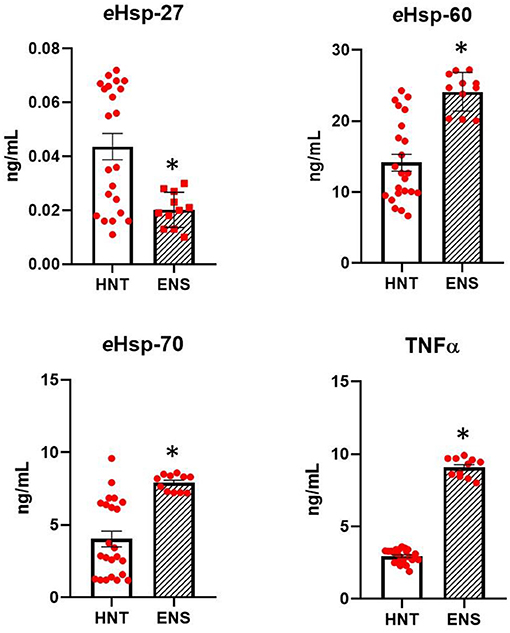
Figure 2. Comparison in the secretion of eHsp-27, eHsp-60, eHsp-70, and TNFα between healthy neonates at term (HNT, n = 23) and neonates with early-onset neonatal sepsis (ENS, n = 11). Concentration was expressed as ng/ml. Data represent the mean ± SEM. Statistical difference was observed between both groups *p ≤ 0.001.
Table 3 shows the relevance of assessing eHsp levels as part of routine clinical laboratory tests for early-onset neonatal sepsis. The sensitivity and specificity of eHsp compared with that in CRP test of 11 samples from infants with early-onset neonatal sepsis were 73.3 and 63.2%, respectively. In addition, positive predictive value (PPV) and negative predictive value (NPV) values were 47.8 and 36.4%, respectively. Finally, the sensitivity and specificity of eHsp compared with that of blood culture were 73.3 and 60.0%, whereas PPV and NPV values were 47.8 and 33.3%, respectively.
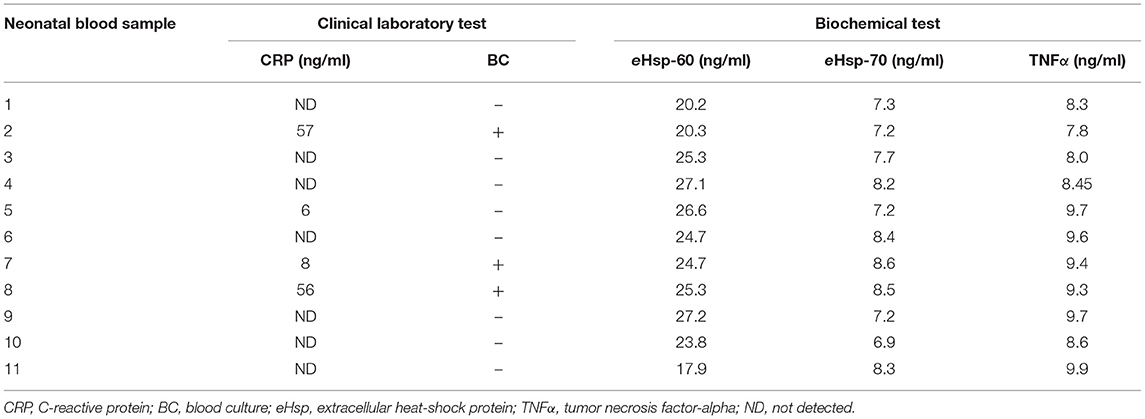
Table 3. Comparison between clinical laboratory and biochemical test in early-onset neonatal sepsis.
Discusion
eHsp have traditionally been considered as intracellular molecules involved in cellular protections (48, 49). However, in recent years, they have been reported as molecules related to different components of the immune response (5, 50). It has been shown that eHsp-60 and eHsp-70 proteins are associated with the inflammatory response (21, 38, 51) and are increased in the plasma of children with septic shock (52, 53). Notwithstanding, the role of eHsp in the plasma of infants with clinical evidence of early-onset neonatal sepsis is poorly unknown.
The main findings of this study are as follows: (1) there is an upregulation of eHsp-60 and eHsp-70 in plasma of patients with early-onset neonatal sepsis, in parallel with an increment of TNFα level that has been previously reported as an early-onset neonatal sepsis biomarker; (2) the downregulation of eHsp-27 in plasma of patients with early-onset neonatal sepsis indicates an inverse relationship with the levels of eHsp-60 and eHsp-70; (3) the high levels of eHsp-60 and eHsp-70 in plasma were consistently detected in neonates with visible signs and symptoms of sepsis even in cases with an undetectable level of CRP and bacteria in blood cultures (Table 3); (4) eHsp-60 and eHsp-70 tests showed higher sensitivity and specificity compared with CRP and blood culture tests.
Studies by Wheeler et al. (54) and He et al. (55) in children with severe sepsis have shown a significant increase in the levels of eHsp-70, TNFα, IL-1β, IL-6, IL-8, IL-13, IL-27, macrophage inflammatory protein-1α, and matrix metalloproteinase-8 (MMP-8) in blood and plasma (54, 56). Studying the systemic inflammatory response syndrome in children, Fitrolaki et al. (51) demonstrated increased levels Hsp-72, Hsp-90, IL-8, IL-6, and TNFα in patients diagnosed with sepsis and considered these as biomarkers associated with fatal outcome in these patients (51, 57). In this investigation, we showed that infants with early-onset neonatal sepsis presented increased levels of eHsp-60 and eHsp-70 that are correlated with an increment in TNFα (Table 3), supporting previous evidence reported by our group and replicating previous reports (46, 51).
A positive blood culture is considered the gold standard for the diagnosis and identification of many clinical infections (27, 58). However, it has a low sensitivity and specificity when used to diagnose neonatal sepsis (59). Recently, it has been shown that neonatal sepsis produced by Group B Streptococcus, Escherichia coli, Enterococcus faecalis, Staphylococcus epidermidis, Streptococcus pneumonia, Acinetobacter baumannii, and Neisseria meningitidis is associated with increased levels of eHsp-70, eHsp-90, and TNFα in blood and plasma (51, 55). Our findings provide new evidence and support previous results showing that infants with early-onset neonatal sepsis with positive blood culture for E. coli, S. epidermidis, and S. dysgalactiae also display marked increase levels of eHsp-60, eHsp-70, and TNFα in plasma.
Using experimental models of infection Campisi et al. (60) showed that E. coli induces a dose-dependent expression of eHsp-72, which is mediated by Toll-like receptor (TLR) by recognizing different structural components of bacteria (60–62). The secretion of eHsp-72 has been associated with increased levels of other biomarkers, such as nitric oxide, TNFα, IL-1β, and IL-6 (60).
Figure 3 shows a proposed model for the differential actions of the anti-inflammatory (eHsp-27) and proinflammatory (eHsp-60 and eHsp-70) response (5, 9). In healthy patients, eHsp-27 is the mainly expressed eHsp, and it is related to protein inhibitory β, a negative regulator of the classical nuclear transcription factor-kappa β (NFkβ) pathway (5, 50), which reduce the production of molecules associated with oxidative stress (63), apoptosis (64, 65), IL-1β, TNFα (12, 21), and collagenolytic action of MMP-9 (66, 67) (Figure 3A). During infection, the inflammatory response is activated, reducing the expression of eHsp-27, increasing the activity of NFkβ and enhancing the levels of eHsp-60 and eHsp-70. This chain of events upregulates IL-1β, TNFα, and MMP-9 (68–70). In sepsis, this activation is mediated by TLR-4 (61, 62) (Figure 3B). Interestingly, our data demonstrate a significant imbalance between a decrease of anti-inflammatory eHsp-27 and an increase of pro-inflammatory eHsp-60 and eHsp-70 in infants with early-onset neonatal sepsis.
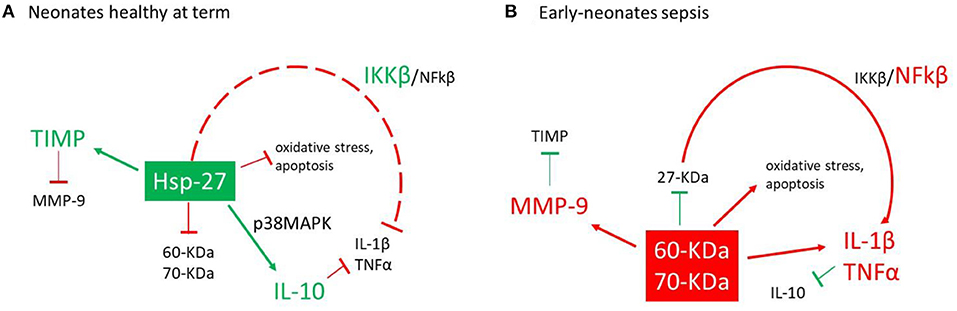
Figure 3. Action model for the activation of eHsp and proinflammatory cytokines. (A) In healthy neonates at term, eHsp-27 modulates the expression of the protein inhibitor (Ikβ) reducing the activity of the nuclear factor-kappa β (NFkβ) pathway (17, 31), modulating the inflammatory response (17, 47), oxidative stress (39), and action of matrix metalloproteinases-9 (MMP-9) (49, 52). (B) During the infections process, the inflammatory response is activated which reduces the expression of eHsp-27, increased the expression of eHsp-60, eHsp-70, and NFkβ and finally increases the secretion of IL-1β, TNFα, and MMP-9 (48, 51, 53).
Clinical guidelines in cases of possible early-onset neonatal sepsis require both CRP assessment and positive blood culture. CRP is produced by the liver and is increased in response to early-onset neonatal sepsis (71). However, several studies have often show inconsistency in the assessment of CRP, possibly due to the gestational age and volume required for testing (30). Nevertheless, the sensibility of these tests increases when additional markers are assessed (72).
The most remarkable finding of our study is that the levels of eHsp-60 and eHsp-70 were consistently increased in all infants with early-onset neonate sepsis (Table 3), whereas blood culture and CRP tests when combined were able to detect 27.27% of the positive cases. The sensitivity, specificity, PPV, and NPV for eHsp with regard to the CRP test was 73.3, 63.2, 47.8, and 36.4%, respectively.
Conclusion
Our study highlights that eHsp-60 and eHsp-70 measured in the plasma of infants could be used as a reliable biomarker of early-onset neonatal sepsis, because the levels of these proteins are consistently elevated and show high sensitivity, specificity, PPV, and NPV. These results provide a strong indication that the assessment of these proteins, together with conventional tests such as CRP and blood culture, can provide a highly sensitive and accurate diagnostic tool to confirm diagnosis of early-onset neonatal sepsis.
Data Availability Statement
The raw data supporting the conclusions of this article will be made available by the authors, without undue reservation.
Ethics Statement
The studies involving human participants were reviewed and approved by Comiteé de Ética en Investigación. Instituto Nacional de Perinatología. The patients/participants provided their written informed consent to participate in this study.
Author Contributions
AC-E, GZ-G, and RM-C obtained blood samples from neonates with and without evidence of early-neonatal sepsis. AC-E and GZ-G provided clinical data on neonates. AC-E, GZ-G, JP-L, and HF-H performed the quantification of eHsp. JP-L performed the quantification of TNFα. PS-T, PG-M, and HF-H conceived and designed the study. AC-E, GZ-G, ND, PS-T, OD-R, and HF-H analyzed the data and interpreted the results. PS-T, PG-M, OD-R, and HF-H wrote the manuscript. All other authors gave approval for the final version of manuscript.
Funding
This research was supported by a grant (number 212250-3210101 assigned to HF-H) from the National Institute of Perinatology, Mexico City. This paper is part of the experimental work of AC-E required for obtaining the Master in Science degree (number 516222901) from Programa de Ciencias Medicas, Odontológicas y de la Salud, Universidad Nacional Autónoma de México (UNAM). We thank CONACyT for supporting his studies.
Conflict of Interest
The authors declare that the research was conducted in the absence of any commercial or financial relationships that could be construed as a potential conflict of interest.
Publisher's Note
All claims expressed in this article are solely those of the authors and do not necessarily represent those of their affiliated organizations, or those of the publisher, the editors and the reviewers. Any product that may be evaluated in this article, or claim that may be made by its manufacturer, is not guaranteed or endorsed by the publisher.
References
1. Rylander MN, Feng Y, Bass J, Diller KR. Thermally induced injury and heat-shock protein expression in cells and tissues. Ann N Y Acad Sci. (2005) 1066:222–42. doi: 10.1196/annals.1363.009
2. Moloney TC, Hoban DB, Barry FP, Howard L, Dowd E. Kinetics of thermally induced heat shock protein 27 and 70 expression by bone marrow-derived mesenchymal stem cells. Protein Sci. (2012) 21:904–9. doi: 10.1002/pro.2077
3. Wallin RP, Lundqvist A, More SH, von Bonin A, Kiessling R, Ljunggren HG. Heat-shock proteins as activators of the innate immune system. Trends Immunol. (2002) 23:130–5. doi: 10.1016/S1471-4906(01)02168-8
4. Ferat-Osorio E, Sanchez-Anaya A, Gutierrez-Mendoza M, Bosco-Garate I, Wong-Baeza I, Pastelin-Palacios R, et al. Heat shock protein 70 down-regulates the production of toll-like receptor-induced pro-inflammatory cytokines by a heat shock factor-1/constitutive heat shock element-binding factor-dependent mechanism. J Inflamm. (2014) 11:19. doi: 10.1186/1476-9255-11-19
5. Zininga T, Ramatsui L, Shonhai A. Heat shock proteins as immunomodulants. Molecules. (2018) 23:2846. doi: 10.3390/molecules23112846
6. Gusev NB, Bukach OV, Marston SB. Structure, properties, and probable physiological role of small heat shock protein with molecular mass 20 kD (Hsp20, HspB6). Biochemistry. (2005) 70:629–37. doi: 10.1007/s10541-005-0162-8
7. Daugaard M, Rohde M, Jaattela M. The heat shock protein 70 family: highly homologous proteins with overlapping and distinct functions. FEBS Lett. (2007) 581:3702–10. doi: 10.1016/j.febslet.2007.05.039
8. Ahmed KA, Xiang J. Mechanisms of cellular communication through intercellular protein transfer. J Cell Mol Med. (2011) 15:1458–73. doi: 10.1111/j.1582-4934.2010.01008.x
9. De Maio A. Extracellular heat shock proteins, cellular export vesicles, and the Stress Observation System: a form of communication during injury, infection, and cell damage. It is never known how far a controversial finding will go! Dedicated to Ferruccio Ritossa. Cell Stress Chaperones. (2011) 16:235–49. doi: 10.1007/s12192-010-0236-4
10. Asea A, Kraeft SK, Kurt-Jones EA, Stevenson MA, Chen LB, Finberg RW, et al. HSP70 stimulates cytokine production through a CD14-dependant pathway, demonstrating its dual role as a chaperone and cytokine. Nat Med. (2000) 6:435–42. doi: 10.1038/74697
11. Srivastava P. Interaction of heat shock proteins with peptides and antigen presenting cells: chaperoning of the innate and adaptive immune responses. Annu Rev Immunol. (2002) 20:395–425. doi: 10.1146/annurev.immunol.20.100301.064801
12. De AK, Kodys KM, Yeh BS, Miller-Graziano C. Exaggerated human monocyte IL-10 concomitant to minimal TNF-alpha induction by heat-shock protein 27 (Hsp27) suggests Hsp27 is primarily an antiinflammatory stimulus. J Immunol. (2000) 165:3951–8. doi: 10.4049/jimmunol.165.7.3951
13. Kampinga HH, Hageman J, Vos MJ, Kubota H, Tanguay RM, Bruford EA, et al. Guidelines for the nomenclature of the human heat shock proteins. Cell Stress Chaperones. (2009) 14:105–11. doi: 10.1007/s12192-008-0068-7
14. Salari S, Seibert T, Chen YX, Hu T, Shi C, Zhao X, et al. Extracellular HSP27 acts as a signaling molecule to activate NF-kappaB in macrophages. Cell Stress Chaperones. (2013) 18:53–63. doi: 10.1007/s12192-012-0356-0
15. Hashiguchi N, Ogura H, Tanaka H, Koh T, Aoki M, Shiozaki T, et al. Enhanced expression of heat shock proteins in leukocytes from trauma patients. J Trauma. (2001) 50:102–7. doi: 10.1097/00005373-200101000-00018
16. Haider T, Simader E, Gluck O, Ankersmit HJ, Heinz T, Hajdu S, et al. Systemic release of heat-shock protein 27 and 70 following severe trauma. Sci Rep. (2019) 9:9595. doi: 10.1038/s41598-019-46034-w
17. Cui X, Xing J, Liu Y, Zhou Y, Luo X, Zhang Z, et al. COPD and levels of Hsp70 (HSPA1A) and Hsp27 (HSPB1) in plasma and lymphocytes among coal workers: a case-control study. Cell Stress Chaperones. (2015) 20:473–81. doi: 10.1007/s12192-015-0572-5
18. Unver R, Deveci F, Kirkil G, Telo S, Kaman D, Kuluozturk M. Serum heat shock protein levels and the relationship of heat shock proteins with various parameters in chronic obstructive pulmonary disease patients. Turk Thorac J. (2016) 17:153–9. doi: 10.5578/ttj.30518
19. Lechner P, Buck D, Sick L, Hemmer B, Multhoff G. Serum heat shock protein 70 levels as a biomarker for inflammatory processes in multiple sclerosis. Mult Scler J Exp Transl Clin. (2018) 4:2055217318767192. doi: 10.1177/2055217318767192
20. Wynn JL, Wong HR. Pathophysiology and treatment of septic shock in neonates. Clin Perinatol. (2010) 37:439–79. doi: 10.1016/j.clp.2010.04.002
21. Giuliano JSJr, Lahni PM, Wong HR, Wheeler DS. Pediatric sepsis - part V: extracellular heat shock proteins: alarmins for the host immune system. Open Inflamm J. (2011) 4:49–60. doi: 10.2174/1875041901104010049
22. Vaara ST, Lakkisto P, Immonen K, Tikkanen I, Ala-Kokko T, Pettila V, et al. Urinary biomarkers indicative of apoptosis and acute kidney injury in the critically ill. PLoS ONE. (2016) 11:e0149956. doi: 10.1371/journal.pone.0149956
23. Chebotareva N, Bobkova I, Shilov E. Heat shock proteins and kidney disease: perspectives of HSP therapy. Cell Stress Chaperones. (2017) 22:319–43. doi: 10.1007/s12192-017-0790-0
24. Albright CM, Ali TN, Lopes V, Rouse DJ, Anderson BL. The Sepsis in Obstetrics Score: a model to identify risk of morbidity from sepsis in pregnancy. Am J Obstet Gynecol. (2014) 211:39.e1–8. doi: 10.1016/j.ajog.2014.03.010
25. Brown DR, Kutler D, Rai B, Chan T, Cohen M. Bacterial concentration and blood volume required for a positive blood culture. J Perinatol. (1995) 15:157–9.
26. Garcia-Gudino I, Yllescas-Medrano E, Maida-Claros R, Soriano-Becerril D, Diaz NF, Garcia-Lopez G, et al. Microbiological comparison of blood culture and amplification of 16S rDNA methods in combination with DGGE for detection of neonatal sepsis in blood samples. Eur J Pediatr. (2018) 177:85–93. doi: 10.1007/s00431-017-3036-3
27. Dien Bard J, McElvania TeKippe E. Diagnosis of bloodstream infections in children. J Clin Microbiol. (2016) 54:1418–24. doi: 10.1128/JCM.02919-15
28. Pan F, Zhao W, Zhang H. Value of time to positivity of blood culture in children with bloodstream infections. Can J Infect Dis Med Microbiol. (2019) 2019:5975837. doi: 10.1155/2019/5975837
29. Dillenseger L, Langlet C, Iacobelli S, Lavaux T, Ratomponirina C, Labenne M, et al. Early inflammatory markers for the diagnosis of late-onset sepsis in neonates: the nosodiag study. Front Pediatr. (2018) 6:346. doi: 10.3389/fped.2018.00346
30. Brown JVE, Meader N, Cleminson J, McGuire W. C-reactive protein for diagnosing late-onset infection in newborn infants. Cochrane Database Syst Rev. (2019) 1:CD012126. doi: 10.1002/14651858.CD012126.pub2
31. Park IH, Lee SH, Yu ST, Oh YK. Serum procalcitonin as a diagnostic marker of neonatal sepsis. Korean J Pediatr. (2014) 57:451–6. doi: 10.3345/kjp.2014.57.10.451
32. Mussap M, Noto A, Fravega M, Fanos V. Soluble CD14 subtype presepsin (sCD14-ST) and lipopolysaccharide binding protein (LBP) in neonatal sepsis: new clinical and analytical perspectives for two old biomarkers. J Matern Fetal Neonatal Med. (2011) 24(Suppl. 2):12–4. doi: 10.3109/14767058.2011.601923
33. van Maldeghem I, Nusman CM, Visser DH. Soluble CD14 subtype (sCD14-ST) as biomarker in neonatal early-onset sepsis and late-onset sepsis: a systematic review and meta-analysis. BMC Immunol. (2019) 20:17. doi: 10.1186/s12865-019-0298-8
34. Fattah MA, Omer AF, Asaif S, Manlulu R, Karar T, Ahmed A, et al. Utility of cytokine, adhesion molecule and acute phase proteins in early diagnosis of neonatal sepsis. J Nat Sci Biol Med. (2017) 8:32–9. doi: 10.4103/0976-9668.198362
35. Jimenez-Sousa MA, Medrano LM, Liu P, Fernandez-Rodriguez A, Almansa R, Gomez-Sanchez E, et al. IL-6 rs1800795 polymorphism is associated with septic shock-related death in patients who underwent major surgery: a preliminary retrospective study. Ann Intensive Care. (2017) 7:22. doi: 10.1186/s13613-017-0247-8
36. Ruan L, Chen GY, Liu Z, Zhao Y, Xu GY, Li SF, et al. The combination of procalcitonin and C-reactive protein or presepsin alone improves the accuracy of diagnosis of neonatal sepsis: a meta-analysis and systematic review. Crit Care. (2018) 22:316. doi: 10.1186/s13054-018-2236-1
37. Eschborn S, Weitkamp JH. Procalcitonin versus C-reactive protein: review of kinetics and performance for diagnosis of neonatal sepsis. J Perinatol. (2019) 39:893–903. doi: 10.1038/s41372-019-0363-4
38. Wheeler DS, Lahni P, Odoms K, Jacobs BR, Carcillo JA, Doughty LA, et al. Extracellular heat shock protein 60 (Hsp60) levels in children with septic shock. Inflamm Res. (2007) 56:216–9. doi: 10.1007/s00011-007-6108-4
39. Mukhopadhyay S, Puopolo KM. Risk assessment in neonatal early onset sepsis. Semin Perinatol. (2012) 36:408–15. doi: 10.1053/j.semperi.2012.06.002
40. Cortese F, Scicchitano P, Gesualdo M, Filaninno A, De Giorgi E, Schettini F, et al. Early and late infections in newborns: where do we stand? A review. Pediatr Neonatol. (2016) 57:265–73. doi: 10.1016/j.pedneo.2015.09.007
41. Mathias B, Mira JC, Larson SD. Pediatric sepsis. Curr Opin Pediatr. (2016) 28:380–7. doi: 10.1097/MOP.0000000000000337
42. Committee on Practice Bulletins-Obstetrics. ACOG practice bulletin no. 188: prelabor rupture of membranes. Obstet Gynecol. (2018) 131:e1–14. doi: 10.1097/AOG.0000000000002663
43. Newton ER. Chorioamnionitis and intraamniotic infection. Clin Obstet Gynecol. (1993) 36:795–808. doi: 10.1097/00003081-199312000-00004
44. Tita AT, Andrews WW. Diagnosis and management of clinical chorioamnionitis. Clin Perinatol. (2010) 37:339–54. doi: 10.1016/j.clp.2010.02.003
45. Higgins RD, Saade G, Polin RA, Grobman WA, Buhimschi IA, Watterberg K, et al. Evaluation and management of women and newborns with a maternal diagnosis of chorioamnionitis: summary of a workshop. Obstet Gynecol. (2016) 127:426–36. doi: 10.1097/AOG.0000000000001246
46. Alvarez-Cabrera MC, Barrientos-Galeana E, Barrera-Garcia A, Osorio-Caballero M, Acevedo JF, Flores-Herrera O, et al. Secretion of heat shock−60,−70 kD protein, IL-1beta and TNFalpha levels in serum of a term normal pregnancy and patients with pre-eclampsia development. J Cell Mol Med. (2018) 22:5748–52. doi: 10.1111/jcmm.13824
47. Thorpe TC, Wilson ML, Turner JE, DiGuiseppi JL, Willert M, Mirrett S, et al. BacT/Alert: an automated colorimetric microbial detection system. J Clin Microbiol. (1990) 28:1608–12. doi: 10.1128/jcm.28.7.1608-1612.1990
48. Young JC. Mechanisms of the Hsp70 chaperone system. Biochem Cell Biol. (2010) 88:291–300. doi: 10.1139/O09-175
49. Saibil H. Chaperone machines for protein folding, unfolding and disaggregation. Nat Rev Mol Cell Biol. (2013) 14:630–42. doi: 10.1038/nrm3658
50. Martine P, Rebe C. Heat shock proteins and inflammasomes. Int J Mol Sci. (2019) 20. doi: 10.3390/ijms20184508
51. Fitrolaki MD, Dimitriou H, Venihaki M, Katrinaki M, Ilia S, Briassoulis G. Increased extracellular heat shock protein 90alpha in severe sepsis and SIRS associated with multiple organ failure and related to acute inflammatory-metabolic stress response in children. Medicine (Baltimore). (2016) 95:e4651. doi: 10.1097/MD.0000000000004651
52. Alder MN, Lindsell CJ, Wong HR. The pediatric sepsis biomarker risk model: potential implications for sepsis therapy and biology. Expert Rev Anti Infect Ther. (2014) 12:809–16. doi: 10.1586/14787210.2014.912131
53. Yilmaz A, Yildirim ZY, Emre S, Gedikbasi A, Yildirim T, Dirican A, et al. Urine heat shock protein 70 levels as a marker of urinary tract infection in children. Pediatr Nephrol. (2016) 31:1469–76. doi: 10.1007/s00467-016-3361-1
54. Wheeler DS, Fisher LEJr, Catravas JD, Jacobs BR, Carcillo JA, Wong HR. Extracellular hsp70 levels in children with septic shock. Pediatr Crit Care Med. (2005) 6:308–11. doi: 10.1097/01.PCC.0000161075.97355.2E
55. He Y, Du WX, Jiang HY, Ai Q, Feng J, Liu Z, et al. Multiplex cytokine profiling identifies interleukin-27 as a novel biomarker for neonatal early onset sepsis. Shock. (2017) 47:140–7. doi: 10.1097/SHK.0000000000000753
56. McConnell KW, Fox AC, Clark AT, Chang NY, Dominguez JA, Farris AB, et al. The role of heat shock protein 70 in mediating age-dependent mortality in sepsis. J Immunol. (2011) 186:3718–25. doi: 10.4049/jimmunol.1003652
57. Gelain DP, de Bittencourt Pasquali MA, Comim CM, Grunwald MS, Ritter C, Tomasi CD, et al. Serum heat shock protein 70 levels, oxidant status, and mortality in sepsis. Shock. (2011) 35:466–70. doi: 10.1097/SHK.0b013e31820fe704
58. Zea-Vera A, Ochoa TJ. Challenges in the diagnosis and management of neonatal sepsis. J Trop Pediatr. (2015) 61:1–13. doi: 10.1093/tropej/fmu079
59. Kellogg JA, Ferrentino FL, Goodstein MH, Liss J, Shapiro SL, Bankert DA. Frequency of low level bacteremia in infants from birth to two months of age. Pediatr Infect Dis J. (1997) 16:381–5. doi: 10.1097/00006454-199704000-00009
60. Campisi J, Leem TH, Fleshner M. Cell Stress Chaperones. (2003) 8:272–86. doi: 10.1379/1466-1268(2003)008<0272:sehiaf>2.0.co;2
61. Gupta A, Cooper ZA, Tulapurkar ME, Potla R, Maity T, Hasday JD, et al. Toll-like receptor agonists and febrile range hyperthermia synergize to induce heat shock protein 70 expression and extracellular release. J Biol Chem. (2013) 288:2756–66. doi: 10.1074/jbc.M112.427336
62. Tulapurkar ME, Ramarathnam A, Hasday JD, Singh IS. Bacterial lipopolysaccharide augments febrile-range hyperthermia-induced heat shock protein 70 expression and extracellular release in human THP1 cells. PLoS ONE. (2015) 10:e0118010. doi: 10.1371/journal.pone.0118010
63. Alvarez-Olmedo DG, Biaggio VS, Koumbadinga GA, Gomez NN, Shi C, Ciocca DR, et al. Recombinant heat shock protein 27 (HSP27/HSPB1) protects against cadmium-induced oxidative stress and toxicity in human cervical cancer cells. Cell Stress Chaperones. (2017) 22:357–69. doi: 10.1007/s12192-017-0768-y
64. Liu C, Huang X, Wang P, Pan Y, Cao D, Liu YY, et al. Interference of Hsp27 results in apoptosis induced by photodamage via regulation of subcellular localization of p21 in immortalized human keratinocytes. Med Sci Monit. (2019) 25:7557–66. doi: 10.12659/MSM.917164
65. Zhou ZB, Huang GX, Lu JJ, Ma J, Yuan QJ, Cao Y, et al. Up-regulation of heat shock protein 27 inhibits apoptosis in lumbosacral nerve root avulsion-induced neurons. Sci Rep. (2019) 9:11468. doi: 10.1038/s41598-019-48003-9
66. Hansen RK, Parra I, Hilsenbeck SG, Himelstein B, Fuqua SA. Hsp27-induced MMP-9 expression is influenced by the Src tyrosine protein kinase yes. Biochem Biophys Res Commun. (2001) 282:186–93. doi: 10.1006/bbrc.2001.4548
67. Rajesh Y, Banerjee A, Pal I, Biswas A, Das S, Dey KK, et al. Delineation of crosstalk between HSP27 and MMP-2/MMP-9: a synergistic therapeutic avenue for glioblastoma management. Biochim Biophys Acta Gen Subj. (2019) 1863:1196–209. doi: 10.1016/j.bbagen.2019.04.015
68. Dunsmore KE, Denenberg AG, Page K, Wong HR. Mechanism and function of heat shock-dependent IkappaBalpha expression. Inflamm Res. (2006) 55:254–9. doi: 10.1007/s00011-006-0080-2
69. Abdulsid A, Hanretty K, Lyall F. Heat shock protein 70 expression is spatially distributed in human placenta and selectively upregulated during labor and preeclampsia. PLoS ONE. (2013) 8:e54540. doi: 10.1371/journal.pone.0054540
70. Peracoli JC, Bannwart-Castro CF, Romao M, Weel IC, Ribeiro VR, Borges VT, et al. High levels of heat shock protein 70 are associated with pro-inflammatory cytokines and may differentiate early- from late-onset preeclampsia. J Reprod Immunol. (2013) 100:129–34. doi: 10.1016/j.jri.2013.08.003
71. Steel DM, Whitehead AS. The major acute phase reactants: C-reactive protein, serum amyloid P component and serum amyloid A protein. Immunol Today. (1994) 15:81–8. doi: 10.1016/0167-5699(94)90138-4
Keywords: early-neonatal sepsis, extracellular heat-shock protein, neonatal intensive care unit, tumor necrosis factor alpha, neonatal infection
Citation: Canul-Euan AA, Zúñiga-González G, Palacios-Luna JE, Maida-Claros R, Díaz NF, Saltigeral-Tigeral P, Karina García-May P, Díaz-Ruiz O and Flores-Herrera H (2021) Increased Levels of Plasma Extracellular Heat-Shock Proteins 60 and 70 kDa Characterized Early-Onset Neonatal Sepsis. Front. Pediatr. 9:740274. doi: 10.3389/fped.2021.740274
Received: 12 July 2021; Accepted: 01 November 2021;
Published: 25 November 2021.
Edited by:
Jesus Acevedo, University of Texas Southwestern Medical Center, United StatesReviewed by:
Rinawati Rohsiswatmo, RSUPN Dr. Cipto Mangunkusumo, IndonesiaMarina Alexandrovna Shevchenko, Institute of Bioorganic Chemistry (RAS), Russia
Copyright © 2021 Canul-Euan, Zúñiga-González, Palacios-Luna, Maida-Claros, Díaz, Saltigeral-Tigeral, Karina García-May, Díaz-Ruiz and Flores-Herrera. This is an open-access article distributed under the terms of the Creative Commons Attribution License (CC BY). The use, distribution or reproduction in other forums is permitted, provided the original author(s) and the copyright owner(s) are credited and that the original publication in this journal is cited, in accordance with accepted academic practice. No use, distribution or reproduction is permitted which does not comply with these terms.
*Correspondence: Héctor Flores-Herrera, aC5mbG9yZXNAaW5wZXIuZ29iLm14