- 1Department of Pediatric Cardiology, Xinhua Hospital, School of Medicine, Shanghai Jiao Tong University, Shanghai, China
- 2Ministry of Education-Shanghai Key Laboratory of Childrens Environmental Health, Xinhua Hospital, School of Medicine, Shanghai Jiao Tong University, Shanghai, China
- 3Clinical Research Unit, Xinhua Hospital, School of Medicine, Shanghai Jiao Tong University, Shanghai, China
- 4Obstetrics Department, International Peace Maternity and Child Health Hospital of China, School of Medicine, Shanghai Jiao Tong University, Shanghai, China
- 5Children Heart Center, Sichuan Provincial Maternity and Child Health Care Hospital, Sichuan, China
Background: Maternal gestational weight gain (GWG) may be associated with cardiovascular diseases in the offspring from childhood to adulthood. We aimed to investigate the association between maternal GWG and the left ventricle (LV) geometry and function in the offspring, and explore the influence of the intrauterine environment on early childhood cardiac change.
Methods: Data of 981 mother-offspring pairs from the Shanghai Birth Cohort was used. Maternal pre-pregnancy weight and height, weight in the first trimester (≤12 weeks), and before delivery were measured. The echocardiography, blood pressure, and anthropometry assessment were evaluated in the offspring at 4 years of age.
Results: Interventricular septal thickness during diastole had a significantly positive correlation with total GWG [β = 0.009, (0.001, 0.017)]. In the second and third trimesters, LV mass index [β = 0.149, (0.015,0.282)], interventricular septal thickness in systole [β = 0.027, (0.011,0.043)], and in diastole [β = 0.014, (0.005,0.023)] were positively associated with GWG. The risks of eccentric [OR = 1.115, (1.232, 1.010)] and concentric hypertrophy [OR = 1.133, (1.259,1.018)] increased with the elevation of maternal GWG.
Conclusions: This study suggested that the excessive maternal GWG was associated with the thickening of the interventricular septum in the offspring, especially during the second and third trimesters. Excessive GWG in the second and third trimesters was a risk factor for LV eccentric and concentric hypertrophy in the offspring.
Introduction
Left ventricle (LV) geometry and function are important factors that influence cardiac remodeling, and are also determinants of cardiovascular events in adulthood (1, 2). LV geometry may increase morbidity and mortality even in asymptomatic conditions, which could occur before the onset of overt hypertension and heart failure (3–6).
The LV geometry and functional changes are often evaluated in adults and adolescents. Morphologic changes in the LV could progress due to cumulative exposures from an early age, which could impair LV function ultimately in later life (3). However, studies on early LV geometry and functional changes in children are limited.
Apart from early influences during childhood, the LV geometry and function could also be affected by various maternal factors, such as obesity, gestational hypertension, and diabetes (7–9). Gestational weight gain (GWG) could reflect the health condition of both the fetus and mother during pregnancy (10, 11). It may play an important role in the development of cardiovascular diseases originating from the intrauterine environment (11–13). Increased GWG and maternal obesity may increase the risk of hypertension (14, 15), ventricular myocardial hypertrophy (16, 17), myocardial fibrosis (18), and congenital heart defects (19), which have been demonstrated in previous adult and animal studies. However, the impact of GWG on early LV geometry and function in young offspring remains unclear.
Based on the Shanghai Birth Cohort (SBC), we aimed to investigate the association of maternal GWG with offspring LV geometry and function at 4 years of age to explore the influence of the intrauterine environment on early childhood cardiac geometry and function.
Materials and Methods
Participants
The SBC is an ongoing prospective cohort study conducted in six collaborating hospitals in Shanghai, China. Volunteer couples were recruited during preconception care or in early pregnancy from 2013 to 2016, and the mother-fetus pairs were followed from preconception or early pregnancy to the end of the gestation. A detailed description of the cohort has been provided elsewhere (20). In our study, only women who had a singleton live birth, with recorded weight and height during pre-pregnancy, and recorded weight at pre-delivery were included. Miscarriage, stillbirth, multiple pregnancies, lost to follow-up, and women without available medical records were excluded. For offspring, children with congenital heart disease, lost to follow-up, uncooperative, and without available records were excluded. Finally, 981 mother-offspring pairs were included in the analysis. Ethical approval was granted by the Ethical Committee of Xinhua Hospital affiliated to Shanghai Jiao Tong University School of Medicine (Protocol no. XHEC-C-2013-001-2). All parents or guardians of participants signed the written informed consent before enrollment.
Measurement of Maternal Factors
Information on demographic and sociodemographic characteristics (e.g., maternal age, race, education level), reproductive characteristics (e.g., parity, gestational week, delivery mode, birth weight and length), lifestyle factors (e.g., passive smoking or alcohol drinking status during pregnancy), and history of gestational hypertension or diabetes were collected through structured questionnaires and extraction of the inpatient history of the pregnant women from medical records. Pre-pregnancy weight and height, weight in the first trimester (≤12 weeks), and weight before delivery were measured at each clinical visit. Maternal pre-pregnancy body mass index (BMI), total GWG, GWG in the first (≤ 12 weeks) or second and third trimesters (>12 weeks) were calculated. Based on the World Health Organization (WHO) criteria, the pre-pregnancy BMI was categorized as underweight (< 18.5 kg/m2), normal weight (18.5–24.9 kg/m2), overweight (25.0–29.9 kg/m2), and obese (≥ 30.0 kg/m2) (21).
Measurement of Offspring Factors
The height and weight of the offspring were measured according to the standard protocol. BMI was calculated in the 4-year-old children. Blood pressure (BP) and heart rate (HR) of the 4-year-old children were assessed by one trained staff while they were supine using the OMRAN HBP-1300 automatic BP device (Omron Healthcare, Guangzhou, China) on the left arm at heart level and with the appropriate cuff size for arm circumference. Three measurements were taken at 5-min intervals. The mean of the measurements was used in all analyses.
Transthoracic echocardiography examinations were performed for the children according to the American and European guidelines (22) by trained operators using the Philip EPIQ7C (Philips Healthcare, Andover, USA) ultrasound that uses the X5-1 (1-5MHz) or S8-3 (8-3MHz) matrix-array transducers (Philips Healthcare, Andover, USA). Measurements of the left ventricle (LV) dimensions were acquired from two-dimensional (2D)-guided M-mode echocardiograms, including the thickness of LV interventricular septum (IVSs and IVSd), posterior wall (LVPWs and LVPWd), and the internal diameter (LVIDs and LVIDd) of the LV during systole and diastole. LV ejection fraction (LVEF) and fractional shortening (FS) were calculated to evaluate the systolic function of LV.
Relative wall thickness (RWT) was calculated by the sum of the thickness of the LV posterior wall in diastole (LVPWd) and interventricular septal thickness in diastole (IVSd), then divided by the internal LV diameter in diastole (LVDd) (23). LV mass (LVM) was calculated using the Devereux formula (24) and the LVM index (LVMI) was calculated using the formula: LVMI = LVM/Height2.7 (25).
Pulse wave Doppler was used to measure the mitral early diastolic flow velocity (E), late diastolic flow velocity (A) to calculate the E/A ratio. The Doppler time intervals, including the ejection time (ET), isovolumic contraction time (ICT), and isovolumic relaxation time (IRT), were obtained at the mitral inflow and LV outflow tracts to calculate the Tei index, with the formula: Tei index = (ICT + IRT)/ET. These indices were used to assess the diastolic function of the LV.
For 2D speckle-tracking analysis, 2D images of 3–5 cardiac cycles were collected and analyzed with the commercial Qlab version 10.5 software (Philips Healthcare, Andover, USA)] at a frame rate of ≥ 60/s. Peak longitudinal strain was measured in the apical four, two, and three-chamber and global peak longitudinal strain (GLS) was calculated using the strain value of each segment in LV.
The LV geometry patterns were defined using the LVMI and RWT according to Ganau and colleagues' descriptions (5). There were no recommended LVMI and RWT cutoff points (26) for 4-year-old healthy children in China. We used the sex-specific 95th percentiles of LVMI and RWT derived from the cohort as cutoff points. LVMI = 33.24 g/m2.7, RWT = 0.27 in girls and LVMI = 33.76 g/m2.7, RWT = 0.27 in boys represented the sex-specific 95th percentiles in the cohort. Four groups were constructed for LV geometry patterns: (1) LVMI <33.24 g/m2.7 for girls and <33.76 g/m2.7 for boys, and RWT < 0.27 was classified as normal left ventricular geometry; (2) normal LVMI with increased RWT (>0.27) was classified as concentric remodeling; (3) increased LVMI (girls ≥33.24 g/m2.7, boys ≥ 33.76 g/m2.7), and normal RWT (<0.27) was defined as eccentric hypertrophy; and (4) increase in both variables was identified as concentric hypertrophy.
All the examinations were performed by a single experienced operator. Both the sonographers and the observers were blinded to the participants' details.
Statistical Analyses
Linear regression models were used to investigate the associations between maternal GWG and offspring LV geometry and function changes. Five sets of models were constructed: the basic model was adjusted for none of the maternal factors or offspring factors. Model 1 was adjusted for maternal factors, including age at delivery, race, educational level, alcoholic drink intake history, exposure to passive smoke, pre-pregnancy BMI, gestational diabetes mellitus (GDM), and gestational hypertension or pre-eclampsia. Model 2 was adjusted additionally for gestational age, sex of offspring, delivery mode, and parity. Model 3 was adjusted additionally for BMI at 4 years of age. Model 4 was adjusted additionally for BP at 4 years of age. The GWG was divided into three groups according to the gestational trimesters as the total, first trimester, and second and third trimesters.
To further eliminate the effect of pre-pregnancy BMI on the LV geometry and function change in offspring, the study population was stratified into three groups (underweight, normal weight, and overweight or obese) according to the WHO criteria (21). Linear regression models were also constructed.
To test the risk of LV geometry pattern changes caused by an increase in GWG, including eccentric hypertrophy, concentric hypertrophy, and remodeling, multiple logistic regression analysis was performed in different groups, and odds ratio (OR) was calculated.
Statistical analysis was carried out using the SPSS 19.0 software program (IBM Corp., Armonk, NY, USA). All tests were two-sided with a significance level of 0.05.
Results
Basic Characteristics
The baseline characteristics of the study participants are presented in Supplementary Tables 1, 2. The mean total GWG was 14.4 ± 5.2 kg. On average, mothers gained a weight of 2.5 ± 3.2 kg in the first trimester and 11.9 ± 4.4 kg in the second and third trimesters. Most pregnant women enrolled had normal BMI (73.2%) before pregnancy. There were 14.1% who were underweight and 12.7% who were overweight or obese. In the offspring, the majority were male (52.8% male vs. 47.2% female). The average results of the LV structure and function data in the offspring were all within the reference range.
Maternal GWG and Offspring LV Geometry and Function
In the basic model (Supplementary Table 3), almost all the indicators of LV internal cavity and wall thickness had a positive correlation with total GWG. However, there was no significant association between GWG with the LV systolic and diastolic function indices at 4 years of age. After adjusting for other maternal or offspring factors (Tables 1–3) which could influence the LV geometry and function in children, only the IVSd [β = 0.009, (0.001, 0.017)] had a significant positive correlation with the total GWG. There was no significant correlation between the LV global function indices and total GWG found in any models.
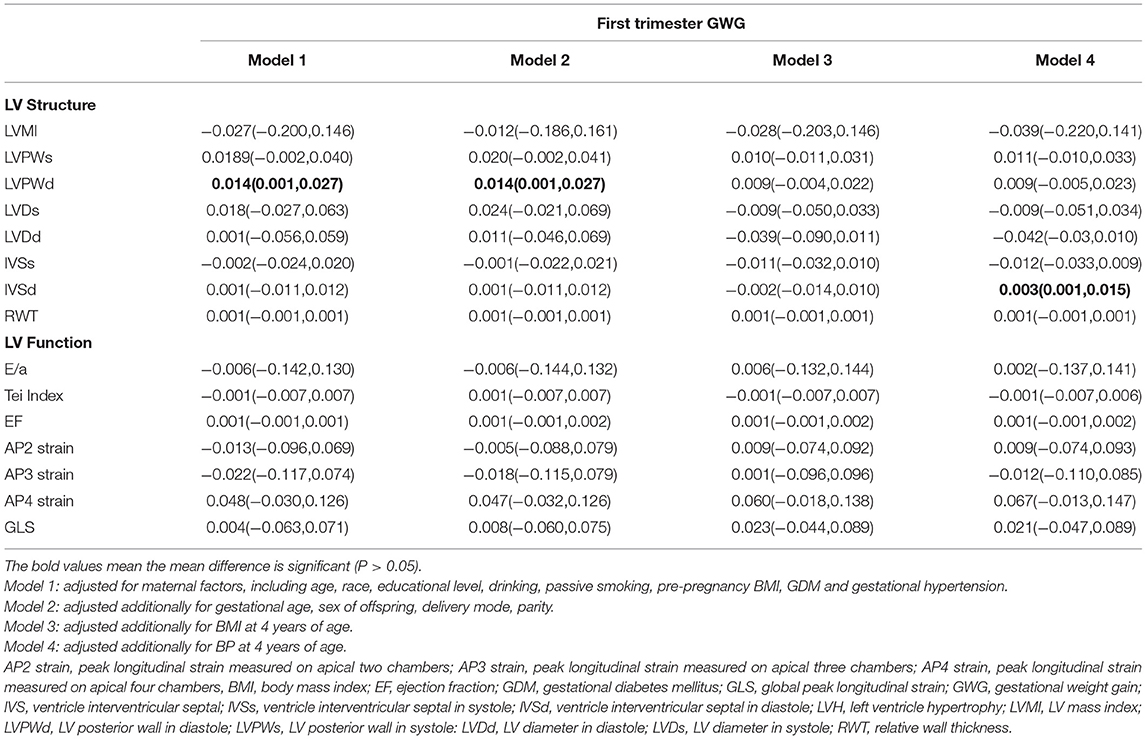
Table 2. Association between maternal GWG and offspring LV geometry and function in the first trimester.
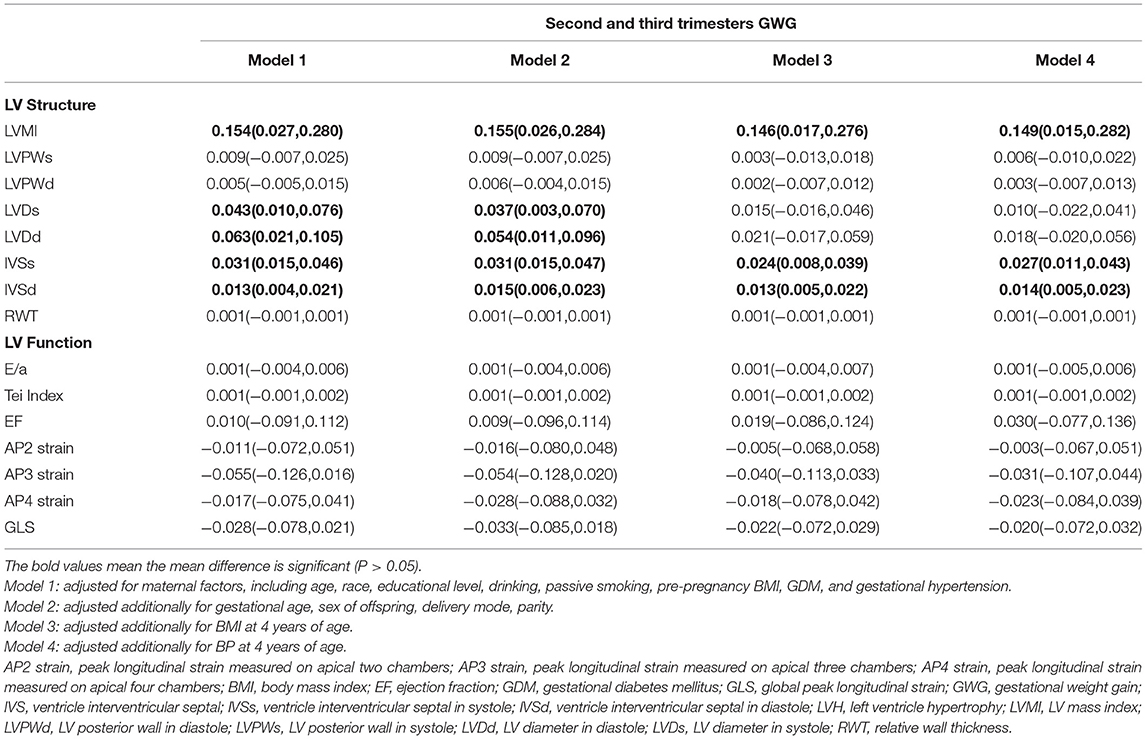
Table 3. Association between maternal GWG and offspring LV geometry and function in the second and third trimesters.
The gestational time was divided into the total, first trimester (≤ 12 weeks), and second and third trimesters (>12 weeks). In the basic model, LVMI [β = 0.147, (0.027, 0.266)], LVDd [β = 0.051, (0.011, 0.091)], IVSd [β = 0.033, (0.017, 0.047)], and IVSs [β = 0.014, (0.006, 0.022)] had a positive association with the second and third trimester GWG. GWG in the first trimester had no significant correlation with any structural and functional indices. After adjusting for maternal and offspring factors, LVMI [β = 0.149, (0.015, 0.282)], IVSs [β = 0.027, (0.011, 0.043)], and IVSd [β = 0.014, (0.005, 0.023)] continued to be positively associated with GWG in all models during the second and third trimesters. The RWT and the LV function indices had no significant correlation with GWG in any trimester.
Offspring LV Geometry and Function in Different Pre-pregnancy BMI Groups
GWG was strongly related to pre-pregnancy BMI (27). Taking the pre-pregnancy BMI into consideration, we divided the maternal-offspring pairs into three groups (underweight, normal weight, and overweight or obese) to eliminate the influence of pre-pregnancy BMI (Table 4). For underweight women, LVPWs was directly associated with the total [β = 0.046, (0.001, 0.091)] and the first trimester GWG [β = 0.068, (0.001, 0.135)]; LVPWd was positively associated with the total [β = 0.035, (0.007, 0.062)] and the second and third trimester GWG [β = 0.035, (0.002, 0.067)]; IVSs was associated with the total GWG [β = 0.049, (0.002, 0.095)]. For normal-weight women, IVSd were positively correlated with the total [β = 0.012, (0.003, 0.022)], and the second and third GWG [β = 0.015, (0.004, 0.026)]; IVSs had a positive correlation with the second and third trimester GWG [β = 0.020, (0.001, 0.039)]. But in overweight and obese women, only IVSs were correlated with the second and third trimester GWG. There was no significant association of LV function indices with GWG in any of the groups.
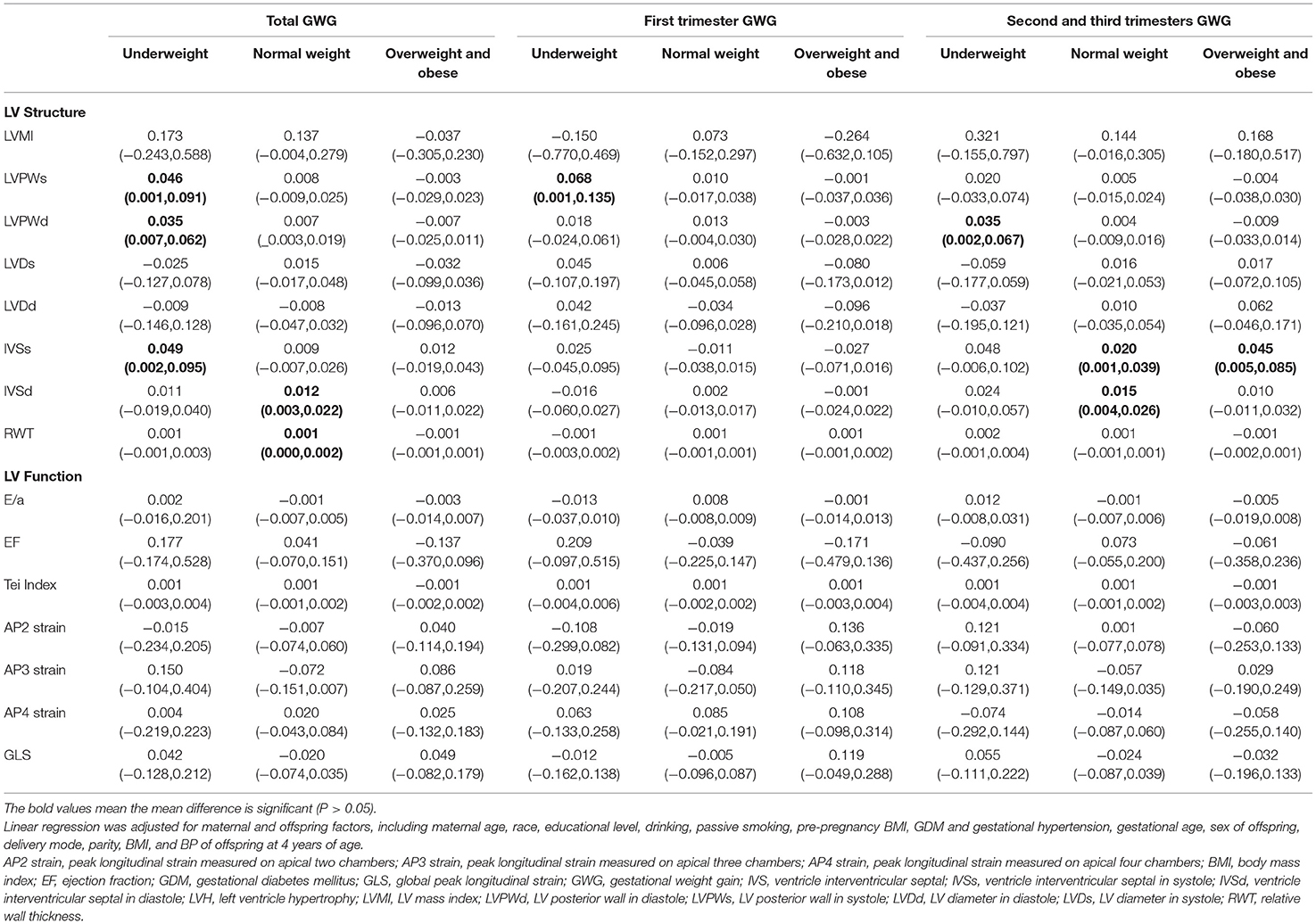
Table 4. Association between maternal GWG and LV geometry and function in offspring divided by pre-pregnant BMI.
Risk of Left Ventricle Hypertrophy in Offspring at 4 Years of Age
The risk of four types of LV geometry change patterns in offspring with maternal GWG in different trimesters is presented in Figure 1. In the second and third trimesters, the risk of eccentric [OR = 1.115, (1.232, 1.010)] and concentric hypertrophy [OR = 1.133, (1.259, 1.018)] increased with an elevation of maternal GWG after adjusting for the maternal and offspring factors. Eccentric, concentric hypertrophy, and remodeling were three types of LVH. It indicated that excessive GWG in the second and third trimesters was an independent risk factor for LVH in the offspring.
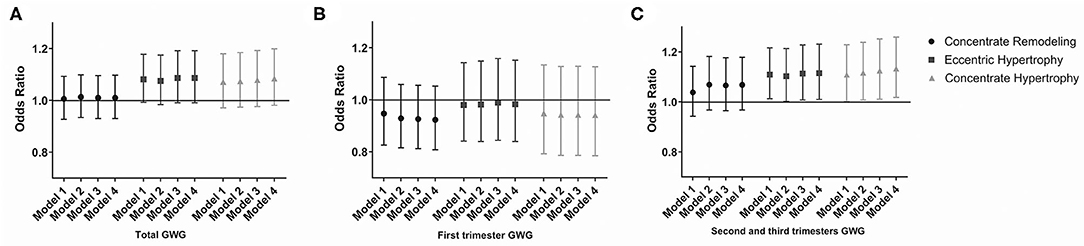
Figure 1. Odds risk (OR) of LVH in offspring with maternal GWG during different gestational trimesters. (A) OR of LVH in offspring with maternal GWG in total trimester. (B) OR of LVH in offspring with maternal GWG in first trimester. (C) OR of LVH in offspring with maternal GWG in second and third trimesters. Data are presented as OR (95%CI). Model 1: adjusted for maternal factors, including age, race, educational level, drinking, passive smoking, pre-pregnancy BMI, GDM and gestational hypertension. Model 2: adjusted additionally for gestational age, sex of offspring, delivery mode, parity. Model 3: adjusted additionally for BMI at 4 years of age. Model 4: adjusted additionally for BP at 4 years of age. BMI, body mass index; GWG, gestational weight gain; LVH, left ventricle hypertrophy.
Discussion
In this prospective cohort study, we found that greater GWG was associated with LV morphologic changes in the offspring as early as 4 years of age, especially the thickening of IVS. Greater GWG during the second and third trimesters was an independent risk factor for LV eccentric and concentric hypertrophy in the offspring.
Cardiac structural changes usually occur before global functional alteration, which is often evaluated during adulthood and adolescence. However, morphologic changes in the LV could progress due to accumulated exposures during childhood, which could impair the LV function ultimately in later life (3). In our study, regional cardiac structural changes occurred as early as 4 years of age during follow-up, whereas there were no changes in the global function. This result provided evidence for early screening of the cardiovascular structure changes in young children, which may be useful for preventing the generation and progression of cardiovascular disease in adulthood.
LV wall thickening is the manifestation of myocardial hypertrophy (28). The thickness of the IVS is one of the reliable markers for evaluating the adverse outcomes in cardiovascular diseases, including coronary artery disease (29), atrial fibrillation (30), and valve replacement (31). In patients with hypertension, the thickening of IVS has been observed before LVMI and functional changes (32); conversely, isolated hypertrophy of the IVS with normal LVM and function has been demonstrated to increase the risk of the development of hypertension in the future (33–35). The thickness of IVS is a multifactorial index influenced by the growth and BP of children. In our study, the thickness of the IVS was found to be significantly associated with greater GWG, independent of offspring's BMI, BP, and other factors.
We focused on the LV geometry and functional patterns in a birth cohort comprising randomly selected children. These LV structural changes, including thickening of the IVS, may be physiologic during early childhood since the pathological changes usually occur in adolescents (26, 36) and adults (9), or in high-risk groups of children, such as those with obesity (37), obstructive sleep apnea (38), or hypertension (39). The transition to pathologic remodeling could be heralded by progressive ventricular dilatation, distortion of the shape of the cavity, and disruption of the normal cardiac geometry and function (40). The LV geometry patterns described the four different LV remodeling features. Eccentric hypertrophy, concentric hypertrophy, and cardiac remodeling were classified as different types and states of LVH. LVH indicated a pathological hyperdynamic state, which may alter LV structure and function that may predispose to the development of heart failure or other adverse cardiovascular prognosis (41). The pressure overload pattern of concentric hypertrophy was associated with high systolic blood pressure and high peripheral resistance. Eccentric LV hypertrophy was associated with normal peripheral resistance, but high cardiac indexes consistent with excess circulating blood volume. Concentric remodeling was characterized by high peripheral resistance, low cardiac index, and increased arterial stiffness (5, 40, 42). In Cuspidi's meta-analysis (43), the risk of LVH was 4.2-fold greater in obese than in non-obese participants and eccentric hypertrophy was the most common type of LVH in obesity. Consistently, we found that the maternal GWG during the second and third trimesters was an independent risk factor for hyperdynamic status in children at 4 years of age. It indicated that the maternal intrauterine environment may have a long-term influence on their offspring's hemodynamic status. However, this hypothesis needs further validation in future studies with larger sample sizes.
Greater GWG was associated with many adverse maternal and offspring outcomes from birth to adulthood. Greater maternal pre-pregnancy weight and GWG were associated with higher systolic blood pressure (36), adverse accumulation of lipid and inflammatory profiles (9, 44), increased risk of congenital heart disease (19, 45), myocardial hypertrophy (16), hypertension (15), and premature death in later life in the offspring (46, 47). The GWG in the second and third trimesters accounted for a majority of the total GWG and played an important role in offspring growth, adiposity, and metabolic state (48). It was a key period for organ development, including the heart, muscles, bones, and liver (48–50). In our study, greater GWG during the second and third trimesters was significantly associated with thickening of the LV wall and was a risk factor for LVH in the offspring, independent of the influence from offspring growth or BP. Therefore, judicious monitoring of GWG during the second and third trimesters should be recommended, which may help promote the health of both the mother and her child.
There are several possible explanations for the mechanisms responsible for a greater GWG increasing the risk of LVH. Greater maternal GWG is an abnormal metabolic state, which modifies the intrauterine environment, thereby influencing placental function and fetal programming of the cardiovascular system, which, in turn, affects fetal heart development. A mother with excessive weight gain or obesity has a suboptimal uterine nutritional climate, which may disrupt the inflammatory and hormone metabolic homeostasis of the mother, including insulin resistance, increased level of leptin, lipid, and pro-inflammatory cytokines. These metabolic disorders may change the placental hemodynamics causing placental vascular insufficiency, increased lipogenesis, infarction, hypoxia, and inflammatory activation (12). These may cause an abnormal accumulation of glycogen and glucose uptake in the myocytes, thus, increasing the load on the fetal heart, ultimately resulting in myocyte hypertrophy (28, 51). Furthermore, the abnormal intrauterine environment may influence the epigenetic modifications of genes associated with cardiovascular function and development (13). The effect of epigenetics is inborn and lifelong. It may have a cumulative effect on the vascular and myocyte function in the offspring from the fetal stage to adulthood. These may increase the risk of the early development of hypertension, ventricular hypertrophy, atherosclerosis, and premature cardiac failure, consequently influencing the prognosis of cardiovascular health in later life (12).
Early screening of LV geometry and function by echocardiography at 4 years of age without the administration of any sedative agents was feasible. Thus, this age may be a suitable time for early screening. Furthermore, early screening of LV structural changes should be recommended in the offspring of mothers who had excessive weight gain during pregnancy, and emphasize the need for additional monitoring and weight management during the second and third trimesters.
Strengths and Limitations
To our knowledge, this study was the largest prospective birth cohort study that assessed LV geometry and function using detailed echocardiography during early childhood in China. Furthermore, previous research on the association between maternal and offspring factors associated with LV geometry usually focused on maternal obesity (46) and were performed in adolescents, adults, or high-risk groups of offspring with obesity (43), abnormal blood pressure (52), or other metabolic syndromes (26). This study provided the first evidence of maternal GWG as an independent risk factor for LV hypertrophy at an early age in the general population.
Our study has several limitations. First, cardiac magnetic resonance imaging is the gold standard to evaluate LV geometry. However, this procedure requires the administration of sedatives in young children. Therefore, in our study, we opted for echocardiography as a more suitable modality for children aged 4 years. Second, data on maternal weight during the second trimester were not available in the medical records, which may need a more detailed investigation in future studies. Third, there was an unequal representation of the populations enrolled in our study regarding the different degrees of GWG and pre-pregnancy BMI, which may have influenced the results of the subgroup analysis.
Conclusion
Excessive GWG, especially during the second and third trimesters, was associated with increased thickening of the IVS. Thus, excessive GWG during the second and third trimesters is a risk factor for LV eccentric and concentric hypertrophy at 4 years of age in the offspring. Our results provide evidence supporting the early screening of LV geometry and function during early childhood, and emphasize the need for additional monitoring and weight management of pregnant mothers during the second and third trimesters.
Data Availability Statement
The raw data supporting the conclusions of this article will be made available by the authors, without undue reservation.
Ethics Statement
The studies involving human participants were reviewed and approved by the research ethics boards of Shanghai Xinhua Hospital (the coordination center, approved on August 23, 2013, ref no. M2013-010). Written informed consent to participate in this study was provided by the participants' legal guardian/next of kin.
Author Contributions
JW, BD, and YW drafted and revised the manuscript. JW, BD, YW, ZL, YW, SC, and KS contributed to the conception and design of the work. JW, BD, YW, ZL, and YY contributed to the acquisition of data. JW, BD, YW, QC, XZ, and ZL contributed to the analysis or interpretation of the data. YW, SC, and KS critically revised the manuscript. All authors gave their final approval and agreed to be accountable for all aspects of this work ensuring its integrity and accuracy.
Funding
This study was partly funded by the National Key R and D Program of China (Grant number: 2018YFC1002400 to 403), the Collaborative Innovation Program of Shanghai Municipal Health Commission (Grant number: 2020CXJQ01), Hospital Funded Clinical Research, Clinical Research Unit, Xinhua Hospital Affiliated to Shanghai Jiaotong University School of Medicine (Grant number: 19XHCR06B).
Conflict of Interest
The authors declare that the research was conducted in the absence of any commercial or financial relationships that could be construed as a potential conflict of interest.
Publisher's Note
All claims expressed in this article are solely those of the authors and do not necessarily represent those of their affiliated organizations, or those of the publisher, the editors and the reviewers. Any product that may be evaluated in this article, or claim that may be made by its manufacturer, is not guaranteed or endorsed by the publisher.
Acknowledgments
We gratefully acknowledge all the children and their parents for their participation and the research staff who have contributed to patient recruitment and data collection in the SBC.
Supplementary Material
The Supplementary Material for this article can be found online at: https://www.frontiersin.org/articles/10.3389/fped.2021.722385/full#supplementary-material
References
1. Lai CC, Sun D, Cen R, Wang J, Li S, Fernandez-Alonso C, et al. Impact of long-term burden of excessive adiposity and elevated blood pressure from childhood on adulthood left ventricular remodeling patterns: the Bogalusa Heart Study. J Am Coll Cardiol. (2014) 64:1580–7. doi: 10.1016/j.jacc.2014.05.072
2. Van daele CM, De Meyer T, De Buyzere ML, Gillebert TC, Denil SL, Bekaert S, et al. Family history of cardiovascular disease and offspring echocardiographic left ventricular structure and function: the Asklepios Study. J Am Soc Echocardiogr. (2013) 26:1290–7.e2. doi: 10.1016/j.echo.2013.07.024
3. Cheng S, Vasan RS. Advances in the epidemiology of heart failure and left ventricular remodeling. Circulation. (2011) 124:e516–9. doi: 10.1161/CIRCULATIONAHA.111.070235
4. Gaasch WH, Zile MR. Left ventricular structural remodeling in health and disease: with special emphasis on volume, mass, and geometry. J Am Coll Cardiol. (2011) 58:1733–40. doi: 10.1016/j.jacc.2011.07.022
5. Ganau A, Devereux RB, Roman MJ, de Simone G, Pickering TG, Saba PS, et al. Patterns of left ventricular hypertrophy and geometric remodeling in essential hypertension. J Am Coll Cardiol. (1992) 19:1550–8. doi: 10.1016/0735-1097(92)90617-V
6. Kim SH, Cho GY, Baik I, Lim SY, Choi CU, Lim HE, et al. Early abnormalities of cardiovascular structure and function in middle-aged Korean adults with prehypertension: the Korean genome epidemiology study. Am J Hypertens. (2011) 24:218–24. doi: 10.1038/ajh.2010.213
7. Appiah D, Schreiner PJ, Gunderson EP, Konety SH, Jacobs DR Jr., Nwabuo CC, et al. Association of gestational diabetes mellitus with left ventricular structure and function: the CARDIA study. Diabetes Care. (2016) 39:400–7. doi: 10.2337/dc15-1759
8. Himmelmann A. Blood pressure and left ventricular mass in children and adolescents: the hypertension in pregnancy offspring study. Blood Press Suppl. (1994) 3:1–46. doi: 10.1097/00004872-199303000-00006
9. Hochner H, Friedlander Y, Calderon-Margalit R, Meiner V, Sagy Y, Avgil-Tsadok M, et al. Associations of maternal prepregnancy body mass index and gestational weight gain with adult offspring cardiometabolic risk factors: the Jerusalem Perinatal Family Follow-up Study. Circulation. (2012) 125:1381–9. doi: 10.1161/CIRCULATIONAHA.111.070060
10. Gaillard R, Steegers EA, Tiemeier H, Hofman A, Jaddoe VW. Placental vascular dysfunction, fetal and childhood growth, and cardiovascular development: the generation R study. Circulation. (2013) 128:2202–10. doi: 10.1161/CIRCULATIONAHA.113.003881
11. Institute of M National Research Council Committee to Reexamine IOMPWG. The National Academies Collection: Reports funded by National Institutes of Health. In: Rasmussen KM, Yaktine AL, editors. Weight Gain During Pregnancy: Reexamining the Guidelines. Washington, DC: National Academies Press (US) (2009).
12. Roberts VH, Frias AE, Grove KL. Impact of maternal obesity on fetal programming of cardiovascular disease. Physiology. (2015) 30:224–31. doi: 10.1152/physiol.00021.2014
13. Van De Maele K, Devlieger R, Gies I. In utero programming and early detection of cardiovascular disease in the offspring of mothers with obesity. Atherosclerosis. (2018) 275:182–95. doi: 10.1016/j.atherosclerosis.2018.06.016
14. Lawlor DA, Najman JM, Sterne J, Williams GM, Ebrahim S, Davey Smith G. Associations of parental, birth, and early life characteristics with systolic blood pressure at 5 years of age: findings from the Mater-University study of pregnancy and its outcomes. Circulation. (2004) 110:2417–23. doi: 10.1161/01.CIR.0000145165.80130.B5
15. Mamun AA, O'Callaghan M, Callaway L, Williams G, Najman J, Lawlor DA. Associations of gestational weight gain with offspring body mass index and blood pressure at 21 years of age: evidence from a birth cohort study. Circulation. (2009) 119:1720–7. doi: 10.1161/CIRCULATIONAHA.108.813436
16. Zielinsky P, Piccoli AL Jr. Myocardial hypertrophy and dysfunction in maternal diabetes. Early Hum Dev. (2012) 88:273–8. doi: 10.1016/j.earlhumdev.2012.02.006
17. Fernandez-Twinn DS, Blackmore HL, Siggens L, Giussani DA, Cross CM, Foo R, et al. The programming of cardiac hypertrophy in the offspring by maternal obesity is associated with hyperinsulinemia, AKT, ERK, and mTOR activation. Endocrinology. (2012) 153:5961–71. doi: 10.1210/en.2012-1508
18. Huang Y, Yan X, Zhao JX, Zhu MJ, McCormick RJ, Ford SP, et al. Maternal obesity induces fibrosis in fetal myocardium of sheep. Am J Physiol Endocrinol Metab. (2010) 299:E968–75. doi: 10.1152/ajpendo.00434.2010
19. Mills JL, Troendle J, Conley MR, Carter T, Druschel CM. Maternal obesity and congenital heart defects: a population-based study. Am J Clin Nutr. (2010) 91:1543–9. doi: 10.3945/ajcn.2009.28865
20. Zhang J, Tian Y, Wang W, Ouyang F, Xu J, Yu X, et al. Cohort profile: the Shanghai Birth Cohort. Int J Epidemiol. (2019) 48:21–g. doi: 10.1093/ije/dyy277
21. World Health Organization. Global Database on Body Mass Index: BMI Classification. Available online at: http://www.assessmentpsychology.com/icbmi.htm (accessed August 19, 2015).
22. Lai WW, Geva T, Shirali GS, Frommelt PC, Humes RA, Brook MM, et al. Guidelines and standards for performance of a pediatric echocardiogram: a report from the Task Force of the Pediatric Council of the American Society of Echocardiography. J Am Soc Echocardiogr. (2006) 19:1413–30. doi: 10.1016/j.echo.2006.09.001
23. Gidding SS, Carnethon MR, Daniels S, Liu K, Jacobs DR Jr., Sidney S, et al. Low cardiovascular risk is associated with favorable left ventricular mass, left ventricular relative wall thickness, and left atrial size: the CARDIA study. J Am Soc Echocardiogr. (2010) 23:816–22. doi: 10.1016/j.echo.2010.05.023
24. Devereux RB, Alonso DR, Lutas EM, Gottlieb GJ, Campo E, Sachs I, et al. Echocardiographic assessment of left ventricular hypertrophy: comparison to necropsy findings. Am J Cardiol. (1986) 57:450–8. doi: 10.1016/0002-9149(86)90771-X
25. Yan Y, Li S, Guo Y, Fernandez C, Bazzano L, He J, et al. Life-course cumulative burden of body mass index and blood pressure on progression of left ventricular mass and geometry in midlife: the bogalusa heart study. Circ Res. (2020) 126:633–43. doi: 10.1161/CIRCRESAHA.119.316045
26. Hietalampi H, Pahkala K, Jokinen E, Rönnemaa T, Viikari JS, Niinikoski H, et al. Left ventricular mass and geometry in adolescence: early childhood determinants. Hypertension. (2012) 60:1266–72. doi: 10.1161/HYPERTENSIONAHA.112.194290
27. Santos S, Eekhout I, Voerman E, Gaillard R, Barros H, Charles MA, et al. Gestational weight gain charts for different body mass index groups for women in Europe, North America, and Oceania. BMC Med. (2018) 16:201. doi: 10.1186/s12916-018-1189-1
28. Guzzardi MA, Liistro T, Gargani L, Ait Ali L, D'Angelo G, Rocchiccioli S, et al. Maternal obesity and cardiac development in the offspring: study in human neonates and minipigs. JACC Cardiovasc Imaging. (2018) 11:1750–5. doi: 10.1016/j.jcmg.2017.08.024
29. Huang BT, Peng Y, Liu W, Zhang C, Huang FY, Wang PJ, et al. Increased interventricular septum wall thickness predicts all-cause death in patients with coronary artery disease. Intern Med J. (2015) 45:275–83. doi: 10.1111/imj.12667
30. Apostolakis S, Sullivan RM, Olshansky B, Lip GY. Left ventricular geometry and outcomes in patients with atrial fibrillation: the AFFIRM Trial. Int J Cardiol. (2014) 170:303–8. doi: 10.1016/j.ijcard.2013.11.002
31. van Straten AH, Soliman Hamad MA, Peels KC, van den Broek KC, ter Woorst JF, Elenbaas TW, et al. Increased septum wall thickness in patients undergoing aortic valve replacement predicts worse late survival. Ann Thorac Surg. (2012) 94:66–71. doi: 10.1016/j.athoracsur.2012.03.027
32. Iurenev AP, Smolenskii AV, Bakhshaliev AB. [Long-term observations of hypertension patients with asymmetrical hypertrophy of the interventricular septum]. Biull Vsesoiuznogo Kardiol Nauchn Tsentra AMN SSSR. (1982) 5:51–3.
33. Grossman C, Grossman A, Koren-Morag N, Azaria B, Goldstein L, Grossman E. Interventricular septum thickness predicts future systolic hypertension in young healthy pilots. Hypertens Res. (2008) 31:15–20. doi: 10.1291/hypres.31.15
34. Harpaz D, Rosenthal T, Peleg E, Shamiss A. The correlation between isolated interventricular septal hypertrophy and 24-h ambulatory blood pressure monitoring in apparently healthy air crew. Blood Press Monit. (2002) 7:225–9. doi: 10.1097/00126097-200208000-00004
35. Eliakim-Raz N, Prokupetz A, Gordon B, Shochat T, Grossman A. Interventricular septum and posterior wall thickness are associated with higher systolic blood pressure. J Clinical Hypertens. (2016) 18:703–6. doi: 10.1111/jch.12738
36. Fraser A, Tilling K, Macdonald-Wallis C, Sattar N, Brion MJ, Benfield L, et al. Association of maternal weight gain in pregnancy with offspring obesity and metabolic and vascular traits in childhood. Circulation. (2010) 121:2557–64. doi: 10.1161/CIRCULATIONAHA.109.906081
37. Mangner N, Scheuermann K, Winzer E, Wagner I, Hoellriegel R, Sandri M, et al. Childhood obesity: impact on cardiac geometry and function. JACC Cardiovasc Imaging. (2014) 7:1198–205. doi: 10.1016/j.jcmg.2014.08.006
38. Amin RS, Kimball TR, Bean JA, Jeffries JL, Willging JP, Cotton RT, et al. Left ventricular hypertrophy and abnormal ventricular geometry in children and adolescents with obstructive sleep apnea. Am J Respir Crit Care Med. (2002) 165:1395–9. doi: 10.1164/rccm.2105118
39. Hanevold C, Waller J, Daniels S, Portman R, Sorof J. The effects of obesity, gender, and ethnic group on left ventricular hypertrophy and geometry in hypertensive children: a collaborative study of the International Pediatric Hypertension Association. Pediatrics. (2004) 113:328–33. doi: 10.1542/peds.113.2.328
40. Lang RM, Bierig M, Devereux RB, Flachskampf FA, Foster E, Pellikka PA, et al. Recommendations for chamber quantification: a report from the American Society of Echocardiography's Guidelines and Standards Committee and the Chamber Quantification Writing Group, developed in conjunction with the European Association of Echocardiography, a branch of the European Society of Cardiology. J Am Soc Echocardiogr. (2005) 18:1440–63. doi: 10.1016/j.echo.2005.10.005
41. Lyon RC, Zanella F, Omens JH, Sheikh F. Mechanotransduction in cardiac hypertrophy and failure. Circ Res. (2015) 116:1462–76. doi: 10.1161/CIRCRESAHA.116.304937
42. Roman MJ, Ganau A, Saba PS, Pini R, Pickering TG, Devereux RB. Impact of arterial stiffening on left ventricular structure. Hypertension. (2000) 36:489–94. doi: 10.1161/01.HYP.36.4.489
43. Cuspidi C, Rescaldani M, Sala C, Grassi G. Left-ventricular hypertrophy and obesity: a systematic review and meta-analysis of echocardiographic studies. J Hypertens. (2014) 32:16–25. doi: 10.1097/HJH.0b013e328364fb58
44. Singhal A, Cole TJ, Fewtrell M, Kennedy K, Stephenson T, Elias-Jones A, et al. Promotion of faster weight gain in infants born small for gestational age: is there an adverse effect on later blood pressure? Circulation. (2007) 115:213–20. doi: 10.1161/CIRCULATIONAHA.106.617811
45. Persson M, Razaz N, Edstedt Bonamy AK, Villamor E, Cnattingius S. Maternal overweight and obesity and risk of congenital heart defects. J Am Coll Cardiol. (2019) 73:44–53. doi: 10.1016/j.jacc.2018.10.050
46. Reynolds RM, Allan KM, Raja EA, Bhattacharya S, McNeill G, Hannaford PC, et al. Maternal obesity during pregnancy andpremature mortality from cardiovascular event in adult offspring: follow-up of 1 323 275 person years. BMJ. (2013) 347:f4539. doi: 10.1136/bmj.f4539
47. Eriksson JG, Sandboge S, Salonen MK, Kajantie E, Osmond C. Long-term consequences of maternal overweight in pregnancy on offspring later health: findings from the Helsinki Birth Cohort Study. Ann Med. (2014) 46:434–8. doi: 10.3109/07853890.2014.919728
48. Deierlein AL, Messito MJ, Katzow M, Berube LT, Dolin CD, Gross RS. Total and trimester-specific gestational weight gain and infant anthropometric outcomes at birth and 6 months in low-income Hispanic families. Pediatr Obes. (2020) 15:e12589. doi: 10.1111/ijpo.12589
49. Rifas-Shiman SL, Fleisch A, Hivert MF, Mantzoros C, Gillman MW, Oken E. First and second trimester gestational weight gains are most strongly associated with cord blood levels of hormones at delivery important for glycemic control and somatic growth. Metab Clin Exp. (2017) 69:112–9. doi: 10.1016/j.metabol.2017.01.019
50. Poissonnet CM, Burdi AR, Bookstein FL. Growth and development of human adipose tissue during early gestation. Early Hum Dev. (1983) 8:1–11. doi: 10.1016/0378-3782(83)90028-2
51. Mäkikallio K, Vuolteenaho O, Jouppila P, Räsänen J. Ultrasonographic and biochemical markers of human fetal cardiac dysfunction in placental insufficiency. Circulation. (2002) 105:2058–63. doi: 10.1161/01.CIR.0000015505.24187.FA
Keywords: gestational weight gain, left ventricle geometry, left ventricle hypertrophy, birth cohort, cardiovascular risk
Citation: Wang J, Du B, Wu Y, Li Z, Chen Q, Zhang X, Zhang L, Ye Y, Wu Y, Chen S and Sun K (2021) Association of Maternal Gestational Weight Gain With Left Ventricle Geometry and Function in Offspring at 4 Years of Age: A Prospective Birth Cohort Study. Front. Pediatr. 9:722385. doi: 10.3389/fped.2021.722385
Received: 08 June 2021; Accepted: 29 July 2021;
Published: 27 August 2021.
Edited by:
Ruan Kruger, North-West University, South AfricaReviewed by:
Michal Odermarsky, Skåne University Hospital, SwedenTanja Lakic, University of Novi Sad, Serbia
Copyright © 2021 Wang, Du, Wu, Li, Chen, Zhang, Zhang, Ye, Wu, Chen and Sun. This is an open-access article distributed under the terms of the Creative Commons Attribution License (CC BY). The use, distribution or reproduction in other forums is permitted, provided the original author(s) and the copyright owner(s) are credited and that the original publication in this journal is cited, in accordance with accepted academic practice. No use, distribution or reproduction is permitted which does not comply with these terms.
*Correspondence: Yurong Wu, wuyurong@xinhuamed.com.cn; Sun Chen, chengsun@hotmail.com; Kun Sun, sunkun@xinhuamed.com.cn
†These authors have contributed equally to this work and share first authorship