- 1Clinical Pharmacology and Toxicology Unit, Shamir Medical Center (Assaf Harofeh), Affiliated to Sackler Faculty of Medicine, Tel-Aviv University, Tel Aviv, Israel
- 2Division of Clinical Pharmacy, Institute for Drug Research, School of Pharmacy, Faculty of Medicine, Hebrew University of Jerusalem, Jerusalem, Israel
- 3Department of Neonatology, Shamir Medical Center (Assaf Harofeh), Affiliated to Sackler Faculty of Medicine, Tel-Aviv University, Tel Aviv, Israel
- 4Division of Obstetrics and Gynecology, Shamir Medical Center (Assaf Harofeh), Affiliated to Sackler Faculty of Medicine, Tel-Aviv University, Tel Aviv, Israel
- 5Departments of Neonatology and Pediatrics, Dana Dwek Children's Hospital, Tel Aviv Medical Center, Affiliated to Sackler Faculty of Medicine, Tel-Aviv University, Tel Aviv, Israel
- 6Department of Epidemiology, Mailman School of Public Health, Columbia University, New York, NY, United States
- 7Residues Lab, Kimron Veterinary Institute, Beit-Dagan, Israel
- 8Department of Epidemiology and Preventive Medicine, School of Public Health, Sackler Faculty of Medicine, Tel-Aviv University, Tel Aviv, Israel
- 9Department of Internal Medicine A, Shamir Medical Center (Assaf Harofeh), Affiliated to Sackler Faculty of Medicine, Tel-Aviv University, Tel Aviv, Israel
- 10Feto-Maternal Unit, Lis Hospital, Tel Aviv Medical Center, Affiliated to Sackler Faculty of Medicine, Tel-Aviv University, Tel Aviv, Israel
Background: Polychlorinated biphenyls (PCBs) are ubiquitous environmental contaminants found in human tissues. PCBs can be transferred through the placenta and may disrupt the maternal thyroid homeostasis, and affect fetal thyroid hormone production. Several studies have shown that intrauterine exposure to PCBs might be associated with abnormal levels of thyroid hormones in mothers and their offspring.
Objectives: To examine the associations between environmental exposure to PCBs and thyroid hormone levels in mothers and newborns.
Methods: The EHF-Assaf-Harofeh-Ichilov cohort includes 263 mothers-newborns dyads. A total of 157 mother-newborn dyads had both PCBs and thyroid function measures. Regression models were used to estimate associations between maternal PCB exposure and maternal and newborn thyroid function, controlling for possible confounders.
Results: Four PCBs congeners were analyzed: PCBs 118, 138, 153, and 180. ∑PCBs median (IQR) level was 14.65 (2.83–68.14) ng/g lipids. The median maternal thyroid-stimulating hormone (TSH) level was 2.66 (0.70–8.23) μIU/ml, the median maternal free thyroxine (FT4) level was 12.44 (11.27–13.53) μg/dL, the median maternal thyroid peroxidase antibodies (TPO Ab) level was 9.6 (7.36–12.51) IU/mL. Newborns' median total thyroxine (T4) level was 14.8 (7.6–24.9) μg/dL. No association was found between exposure to different congeners or to ∑PCBs and maternal TSH, FT4, thyroglobulin autoantibodies (Tg Ab), TPO Ab and newborn total T4 levels. In multivariable analysis a 1% change in ∑PCBs level was significantly associated with a 0.57% change in maternal TSH levels in women with body mass index (BMI) < 19. The same association was observed for each of the studied PCB congeners. Maternal TPO Ab levels statistically significantly increased by 0.53 and 0.46% for 1% increase in PCB 118 and 153 congeners, respectively. In women with BMI > 25, the association between the PCBs levels and maternal TSH levels was in the opposite direction. No association was found in women with normal BMI (19–24.9).
Conclusions: Background exposure to environmentally relevant concentrations of some PCBs can alter thyroid hormone homeostasis in pregnant women and might be associated with abnormal TSH levels and TPO-Ab in women with low BMI. However, these findings require further investigation.
Introduction
Endocrine-disrupting chemicals (EDCs) contribute substantially to human morbidity and are estimated to result in hundreds of billions in costs per year (1). The Endocrine Society presented evidence that EDCs have multiple effects on multiple endocrine systems, including those associated with female reproduction, thyroid function, neurodevelopment, and neuroendocrine systems especially during development (2).
Polychlorinated biphenyls (PCBs) are a large family of persistent endocrine-disrupting industrial chemicals, once widely used as non-flammable lubricants and insulators (3, 4). PCBs were banned from production in the late 1970s in the USA and from 2001 onwards worldwide by the Stockholm Convention on Persistent Organic Pollutants (5). However, their chemical nature, lipophilicity, and resistance to degradation have led to bioaccumulation in the ecosystem, with ongoing human exposure (6). Due to their lipophilic structure, PCBs are readily absorbed from the environment into the food chain, rendering human and animal exposure ubiquitous. Human exposure mainly involves consuming fatty food like fish, meat, and dairy products (7, 8). PCBs 118, 138, 153, 180 are among the most frequently detected congeners in white adipose tissue and blood samples (9–11). These PCBs are more heavily chlorinated and have a half-life of years (12). PCBs 138, 153, and 180 have the highest detection frequencies in the US population and contributed to 80% of the total PCBs in human serum (13). PCB 118 is considered a dioxin-like compound, mono-ortho PCB, where PCBs 138, 153, and 180 are non-dioxin-like congeners, di-ortho congeners (3, 4).
Some studies examined PCBs as endocrine disruptors both in animals (14, 15) and in humans, with a particular focus on thyroid hormones (16, 17) and steroid hormones related to the reproductive system (18). In particular, associations between PCB serum levels and thyroid function have been examined both in adults (19) where thyroid hormone differences were observed in relation to serum PCB concentrations, and neonates (20) showing that prenatal exposure to some PCBs impacts newborn TSH levels.
Thyroid function is considered a potential target for endocrine disruption by persistent chemicals such as PCBs (16, 17, 21). PCBs may disrupt thyroid hormone signaling and lead to thyroid disease given the structural similarities to thyroid hormones (2). In particular, PCBs can disrupt estrogen receptors, aryl hydrocarbon receptors (AhR), or thyroid hormone receptors (22, 23). Thangavelu et al. suggest that PCBs exposure may reduce the serum levels of LH, testosterone and estradiol, may cause a decrease in the Leydig cell population and may decrease activities of steroidogenic enzymes 3β- and 17β-HSD (24).
A recent study showed that serum AhR bioactivities were increased with specific PCB congeners, regardless of their dioxin-like properties (25). Miyazaki et al. posited that weak suppression of TR-mediated transcription by non-dioxin-like PCBs is caused by dissociating thyroid hormone receptor (TR) from the TR response element (TRE) (26). The combined data from human studies and experimental animal studies also suggest that PCBs negatively affect thyroid function by interference with transport and metabolism (27). Curtis et al. found that higher Polybrominated biphenyls (PBBs) were associated with higher free T3, lower free T4, and a higher FT3/FT4 ratio and higher PCBs levels were associated with higher FT4 and a higher FT3/FT4 ratio (28).
It is known that adequate levels of thyroid hormones are crucial for the proper development of the brain. Epidemiological studies found that subclinical disruptions in thyroid hormones during pregnancy are associated with decrements in cognitive functions of offspring later in life. Hence, exposure to thyroid-disrupting chemicals may have significant consequences for public health even if they cause a small change in thyroid hormone levels. This could explain some, but not all of the neurodevelopmental effects of PCBs; however, data are not consistent across the studies (2, 11, 29–34).
Pregnant women, fetuses, infants, and children are most vulnerable to low-dose environmental exposures. There is growing evidence of adverse effects of environmental exposure on reproduction, pre and postnatal development (35–37). As a lipophilic substance, PCBs can be transferred through the placenta and during breastfeeding to increase children's body burden. Therefore, children are exposed to PCBs starting from conception, and the exposure continues throughout different stages of their lives (38). Exposure to these chemicals may have adverse effects on the developing fetus, including intrauterine growth retardation (IUGR), neurocognitive deficit, and hormonal dysfunction (39–41). Organochlorines, including PCBs, have been shown to cross the placenta and have been associated with neurobehavioral effects in children (42, 43).
Some studies suggest that TSH values differ in pregnant women as pre-pregnancy body mass index (BMI) increases, and there is an association between lower FT4 levels and higher BMI (44). In addition, there are differences in body fat distribution between overweight and normal-weight women during pregnancy (45). Changes in maternal fat mass can range from −10 to +15 kg at term (46).
In this study we aimed to evaluate the possible associations between background exposure to PCBs and thyroid hormone levels within a birth cohort of Israeli mothers and newborns.
Materials and Methods
Study Population
We used data collected as part of a birth cohort performed at Shamir Medical Center (Assaf Harofe) and “Dana Dwek” Children's Hospital, Tel Aviv Medical Center.
From January 2013 through April 2015, 263 mother-father-newborn triads were recruited. The women recruited into the study were asked to participate during attendance in the delivery room. Data on social and demographic characteristics and lifestyle variables from both the father and mother were obtained through a detailed questionnaire. Data on occupation, residential history, diet, hobbies, and a detailed health history were also collected. At the delivery room blood and urine samples were collected from mothers and fathers. After delivery, cord blood samples, meconium samples, and breast milk (colostrum) samples were collected. All samples were processed according to a standard protocol, and frozen at −80°C.
Maternal height, pre-pregnancy weight, and weight at the time of delivery were extracted from medical records. As part of the routine physical examination, all infants underwent a structured examination for congenital malformations.
Birth weight, length, and head circumference were measured three times using standard research procedures.
To compare the birth weights, birth weight was adjusted to gestational age at birth and infant's sex and classified according to percentile values derived from the Israeli Perinatal survey (47).
All participants signed informed consent. The study was performed according to the Declaration of Helsinki, and the Institutional Review Board approved the protocol (48).
Data Extraction
The database included 263 mother-newborn dyads. Due to financial limitations, we measured concentrations of 4 PCBs from maternal blood of 183 mothers who gave birth at Shamir Medical Center (Assaf Harofeh). On average, included and excluded dyads were similar in terms of potential confounders such as birth weight, length of gestation, and maternal age (49).
The comparison of maternal characteristics of included and excluded dyads is presented in Supplementary Table 1. There were more primiparous included in the study (31.8 vs. 18.9%, p = 0.015). Pre-pregnancy BMI was lower in the included mothers compared to the excluded (median, IQR), 21.1 (19.3–22.9) vs. 22.5 (20.5–24.3), p = 0.008. Excluded mothers had a higher percentage of Foreign-born (27 vs. 15.3%, p = 0.022).
We retrieved data on 183 dyads for which maternal serum PCBs measurements were obtained. Data on maternal demographics, exposures, lifestyle, and characteristics of labor were extracted as well as newborn anthropometrics and measurements.
Maternal laboratory test results, obtained at the time of delivery, included thyroid stimulating hormone (TSH), free thyroxine (FT4), thyroglobulin autoantibodies (TgAb), thyroid peroxidase antibody test (TPOAb), total cholesterol, and triglycerides.
Newborn total T4 was obtained through a routine national survey methodology testing for congenital hypothyroidism by heel puncture blood sample, collected between days 2–3 of life.
Cases of women with known hypothyroidism (N = 9) or hyperthyroidism (N = 2), dyads with no total T4 levels for the newborn (N = 12), twin pregnancies (N = 2), and premature delivery (<35 weeks) (N = 1) were excluded.
Sample Analysis
PCBs were measured in 200 randomly selected maternal samples. Seventeen of the 200 samples were duplicates. The correlations between the duplicates were 0.996, 0.997, 1, and 0.997 for congeners 118, 138, 153, and 180, respectively. Where duplicate samples were measured, a mean of the two results was taken.
Blood samples were collected through venipuncture into tubes containing clot activator. Samples were kept at 4°C until processing, within 4–6 h from collection. Samples were centrifuged at 1,200 g for 7 min and serum was aliquoted in 2 ml cryovials and kept frozen at −80°C until analysis.
PCBs were measured at the Centre de Toxicologie du Quebec. Congeners 118 (2,3′,4,4′,5-pentachlorobiphenyl), 138 (2,2′,3,4,4′,5′-hexachlorobiphenyl), 153 (2,2′,4,4′,5,5′-hexachlorobiphenyl), and 180 (2,2′,3,4,4′,5,5′-heptachlorobiphenyl) were measured. All measures were performed using GC/MS at INSPEQ, at the Arctic Monitoring and Assessment Programme Ring Test for Persistent Organic Pollutants in Human Serum (AMAP), organized and managed by the Centre de Toxicologie du Québec (CTQ).
The lower limit of quantification (LOQ) was 10 ng/L. For statistical analysis purposes, values below LOQ were assigned with the value of LOQ/√(2). All the samples were above the LOQ for the PCB 153. Additionally, there were 11 samples with levels below the LOQ: PCB 118—eight samples, PCB 118+138—one sample, PCB 118+180—one sample, PCB 118+138+180—one sample.
PCBs were reported as the wet weight. We normalized the concentrations using the following equation which adjusts chemical per gram of total lipids (lipid weight, ng chemical/g total lipid) (50).
Total lipids (TL) were estimated using the formula (51, 52):
TL (g/L) = 2.27 × TC (g/L) + TG (g/L) + 0.623
where TL—total lipids, TC—total cholesterol, TG—triglycerides.
Maternal TSH, FT4, thyroglobulin autoantibodies (TgAb), thyroid peroxidase antibody test (TPOAb), total cholesterol, and triglycerides were measured at the Shamir (Assaf Harofeh) biochemistry lab using standard methods.
The enzymatic method was used for the quantitative determination of cholesterol and triglycerides in human serum and plasma on Roche/Hitachi Cobas c systems. Immunoassay was used for the quantitative determination of free T4, TSH, TPOAb, and TgAb.
TPO Ab was defined positive above 34 IU/mL, and Tg Ab was defined positive above 115 IU/mL.
Statistical Analysis
Continuous variables are presented as mean and standard deviation (±SD) or median and interquartile range (IQR). Continuous variables were compared between groups using the Kruskal Wallis test or Mann-Whitney test. Categorical variables were compared using the Chi-square test, or Fisher's exact test, as appropriate.
Spearman correlation coefficients were calculated to assess the crude correlation between thyroid function of the mother and the newborn and PCBs level variables.
Potential confounders were selected based on their previously reported associations with prenatal PCB exposure or with thyroid function. Potential confounders included: gender, country of origin, date of birth, gestational age (in weeks), birth weight (in grams), prenatal tobacco smoke exposure, parity (nulliparous/multiparous), relationship status (unmarried/married or with the same partner), maternal age (in years), monthly household income (net), maternal education, and maternal employment. Our final models adjusted for maternal age and newborn sex since the association between males and females and the total T4 in newborns was different.
The maternal PCBs levels, TSH levels, FT4, TPOAb, TgAb, and newborn total T4 were skewed; therefore, we used natural logarithm transformation to achieve normality. Linear regression analysis was used to examine the associations between the exposure variables and the outcome variables, adjusting for potential confounding variables.
Since natural logarithm transformation was used for the dependent and independent variables, the regression describes the percent change in TSH levels according to a 1 percent change in the PCB levels.
Interaction variables between pre-pregnancy BMI categories and PCB levels and between newborn sex and PCB levels were included in the model (53).
All statistical tests were two-tailed, and p < 0.05 was considered statistically significant. SPSS software (IBMS SPSS Statistics for Windows, Version 25, IBM Corp, Armonk, New York, USA) was used for all statistical analyses.
Results
One hundred and fifty-seven mothers-newborn dyads were included in the final analysis. The mothers' characteristics including age, education, relationship, employment status, monthly income, alcohol and smoking habits, and country of origin are presented in Table 1. The data presented in Tables 1–3 are grouped by maternal pre-pregnancy BMI.
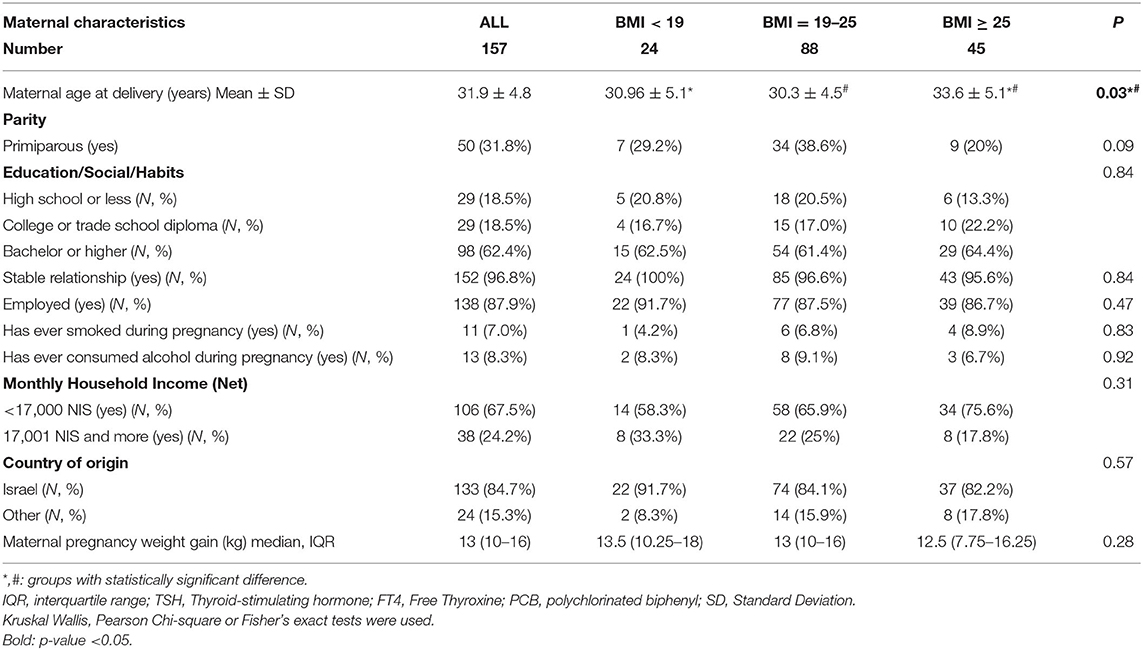
Table 1. Demographic characteristics of mothers in the EHF-Assaf-Harofeh-Ichilov birth cohort, Israel, 2013–2015, grouped by maternal pre-pregnancy BMI.
The maternal mean (±SD) age was 31.9 ± 4.8 years. Women with higher pre-pregnancy BMI were older that other 2 groups: 33.6 ± 5.1 years in BMI ≥ 25, 30.96 ± 5.1 years and 30.3 ± 4.5 years in BMI < 19 and BMI 19–24.9 respectively (p = 0.03). No other differences were observed between the 3 BMI groups. About one-third of women were primiparous (31.8%). Most women had an academic education −98 (62.4%) with a bachelor's degree or higher. Almost all women were married (96.8%) and employed (87.9%). One hundred and thirty-three mothers were born in Israel (84.7%), and most foreign-born mothers (21/24, 87.5%) were born in East Europe. Only a few women consumed alcohol or smoked during pregnancy (N = 13, 8.3% and N = 11, 7.0%, respectively).
Maternal PCBs' concentration levels (lipid adjusted) are presented in Table 2. PCBs 118, 138, 153, and 180 median levels were 2.23 (1.79–3.03), 3.31 (2.51–4.76), 5.44 (3.83–7.67), and 3.69 (2.67–5.4) ng/g lipids, respectively. ∑PCBs median level was 14.65 (11.03–20.89) ng/g lipids. No statistically significant differences were observed between the 3 BMI groups. Total lipids were calculated as described in the Material and Methods section (Sample Analysis) and no statistically significant differences were observed between the three BMI groups (p = 0.06) (Table 2).
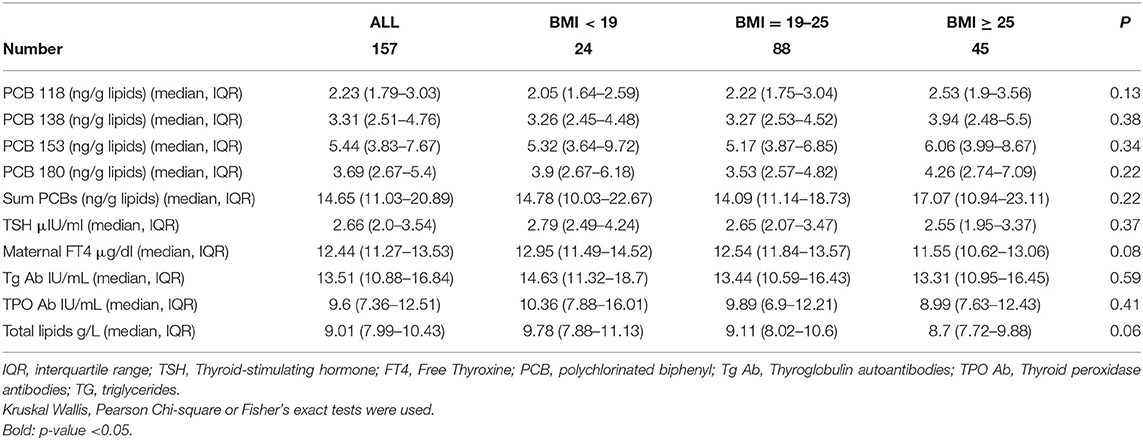
Table 2. Maternal measurements in the EHF-Assaf-Harofeh-Ichilov birth cohort Israel, 2013–2015, grouped by maternal pre-pregnancy BMI.
Maternal median FT4 and TSH levels were 12.44 (IQR 11.27–13.53, laboratory range 12–22) μg/dl and 2.66 (IQR 2.0–3.54, laboratory range 0.27–4.2) μIU/ml, respectively. Maternal Tg Ab and TPO Ab were measured 13.51 (IQR 10.88–16.84) IU/mL and 9.6 (IQR 7.36–12.51) IU/mL, respectively. Three women had thyroid peroxidase antibodies >34 IU/L and were categorized as anti-TPO positive, one in each BMI group (Table 2). No statistically significant differences were observed between the 3 BMI groups.
Newborn mean (±SD) birth week was 39.33 ± 1.28. The newborn mean (±SD) birth weight was 3,141 g (±474.25). Newborn mean (±SD) birth length was 49.28 cm (±2.08). Newborns of women with higher BMI had higher birth weight, birth length and head circumference than the other 2 BMI groups: 3,236 g (±454.87), 49.86 cm (±2.06), 34.27 cm (±1.31), (p-value: 0.02, 0.03, 0.049), respectively (Table 3).
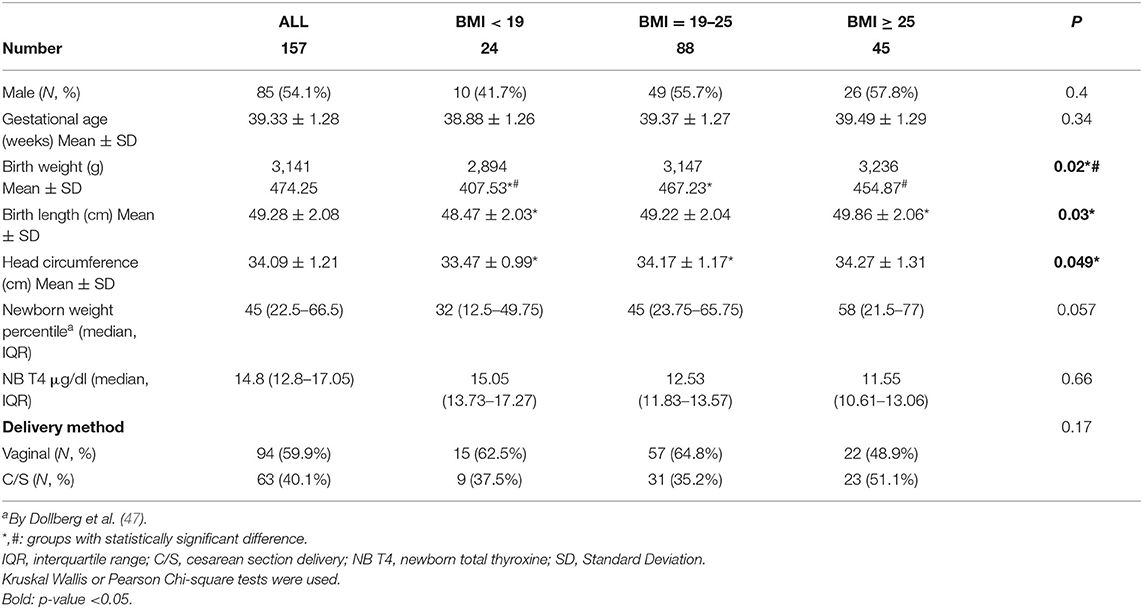
Table 3. Anthropometric measurements and characteristics of the newborns in the EHF-Assaf-Harofeh-Ichilov birth cohort, Israel, 2013–2015, grouped by maternal pre-pregnancy BMI.
Newborn weight percentile was higher in women with BMI ≥ 25, as compared with the other 2 BMI groups, median (IQR) −58 (21.5–77), vs. 32 (12.5–49.75) and 45 (23.75–65.75), p = 0.057. No other differences were observed between the 3 BMI groups.
Vaginal delivery method was recorded in 59.9% (94) of cases. Newborn median total T4 level was 14.8 μg/dL (IQR 12.8–17.05). The ranges of newborn total T4 levels were within what is considered a standard reference range (Table 3).
No correlation was found between maternal exposure to the studied PCB congeners or ∑PCBs and maternal TSH, maternal FT4, maternal TG Ab, maternal TPO Ab, and total T4 levels in the newborns in the total study population (Table 4).
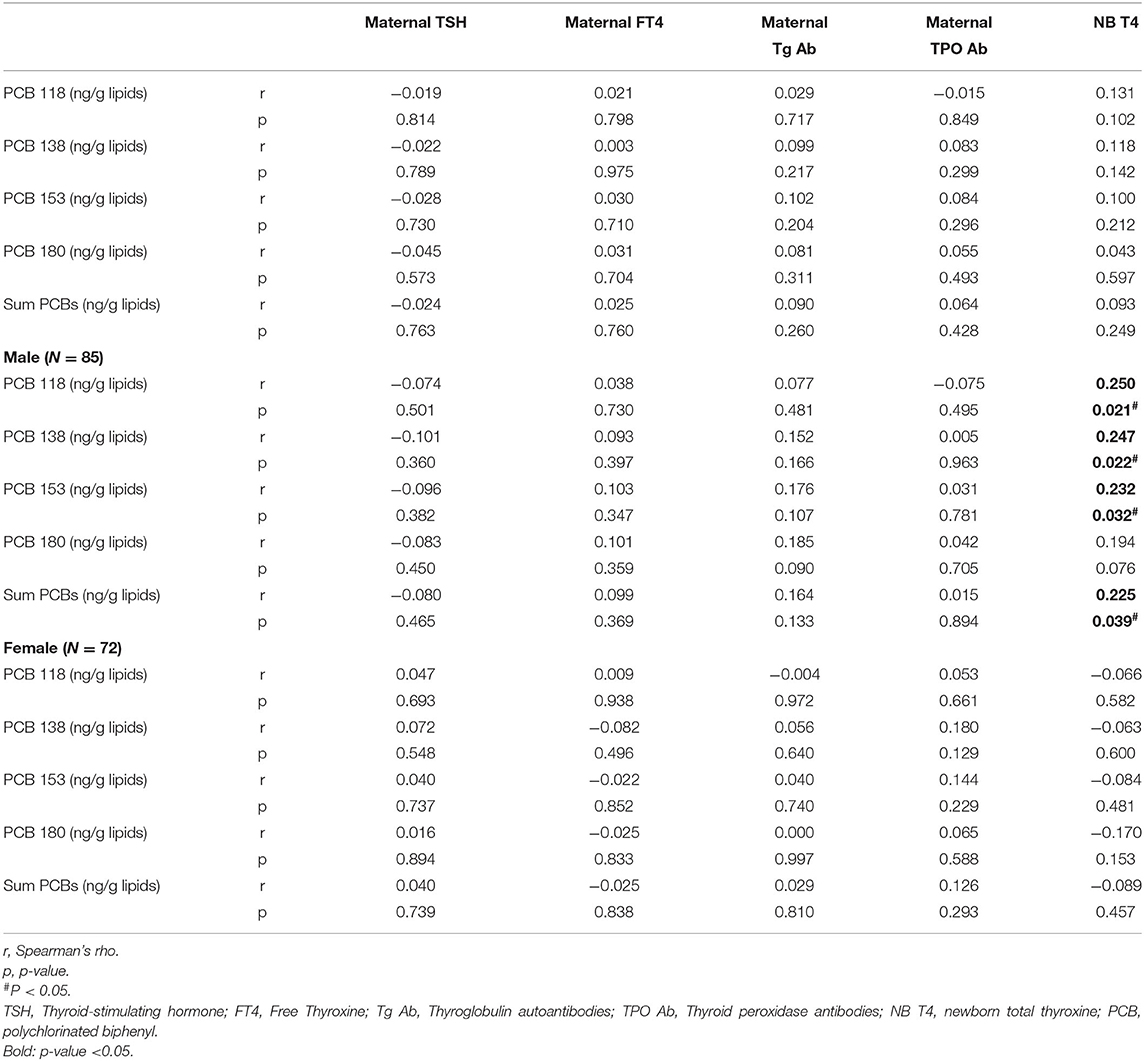
Table 4. Spearman rank correlations of PCBs (lipid adj.) and thyroid function of mothers and newborns in the EHF-Assaf-Harofeh-Ichilov birth cohort, Israel, 2013–2015 (n = 157).
A statistically significant correlation was found between maternal PCBs and total T4 in male, but not in female neonates. Spearman rank correlations for males' r (p-value): 0.250 (0.021), 0.247 (0.022), 0.232 (0.032), and 0.194 (0.076) for PCBs 118, 138, 153, and 180, respectively, and 0.225 (0.039) for ∑PCBs. Spearman rank correlations for females' r (p-value): −0.066 (0.582), −0.063 (0.6), −0.084 (0.481), −0.170 (0.153), and −0.089 (0.457) for PCBs 118, 138, 153, 180, and for ∑PCBs, respectively (Table 4).
Since a significant interaction between maternal pre-pregnancy BMI and maternal PCBs levels was found, the association between thyroid functions and PCBs was studied separately in each BMI group.
All 4 studied PCB congeners and ∑PCBs were highly correlated to maternal TSH in BMI < 19 group. Spearman rank correlations between PCB 118, 138, 153, 180, ∑PCBs and maternal TSH levels were 0.412 (p value: 0.045), 0.501 (p value: 0.013), 0.518 (p value: 0.010), 0.445 (p value: 0.03), and 0.511 (p value: 0.011), respectively (Table 5).
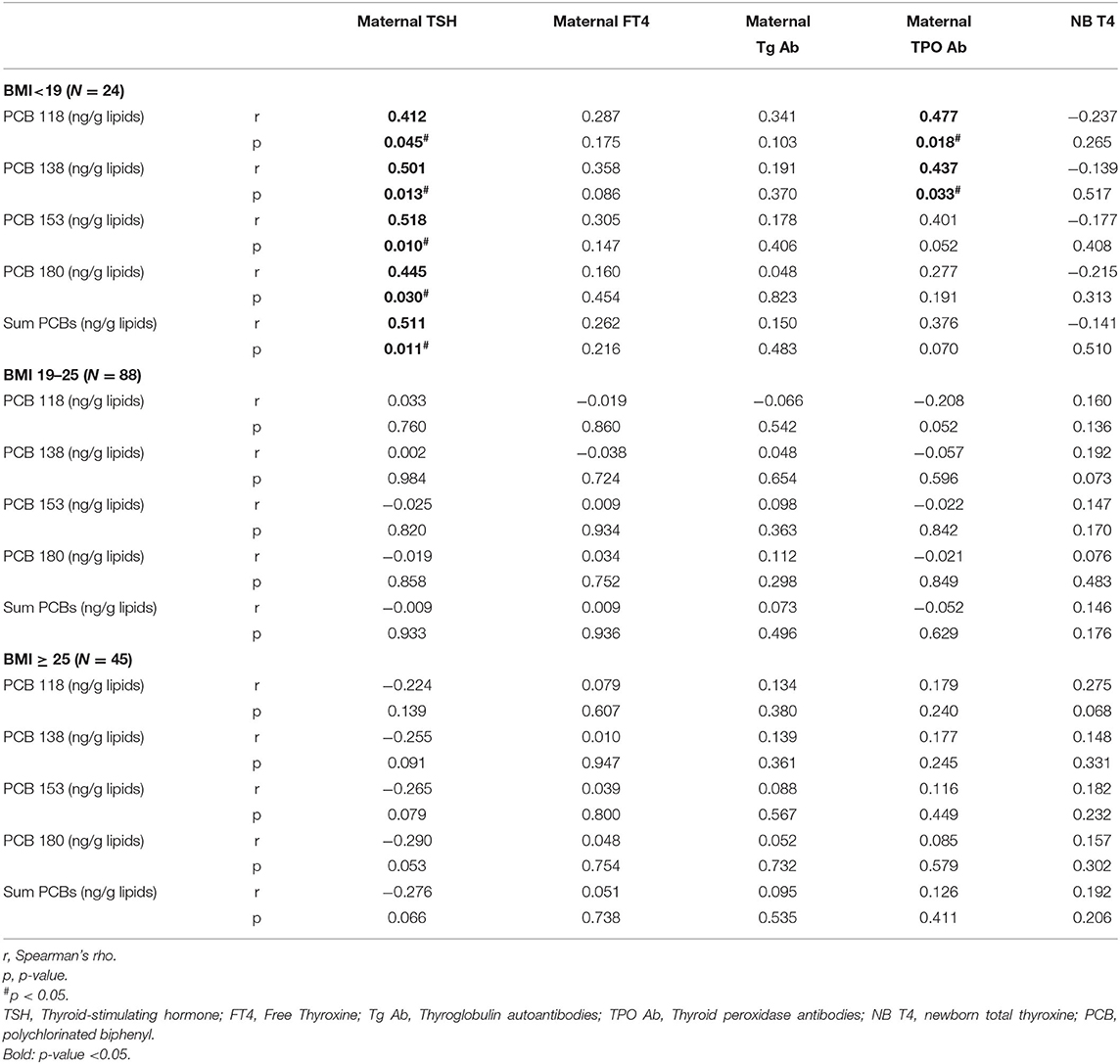
Table 5. Spearman rank correlations of PCBs (lipid adj.) and thyroid function of mothers and newborns at different BMI groups, in the EHF-Assaf-Harofeh-Ichilov birth cohort, Israel, 2013–2015, grouped by maternal pre-pregnancy BMI.
Maternal TPO Ab were highly correlated to congeners PCB 118 and 138, and approached significance for PCB 153 but not for 180 or ∑PCBs. Spearman rank correlations between PCB 118, 138, 153, 180, ∑PCBs and maternal TPO Ab were 0.477 (p value: 0.018), 0.437 (p value: 0.033), 0.401 (p value: 0.052), 0.277 (p value: 0.191), and 0.376 (p value: 0.070), respectively (Table 5).
No correlation was found between maternal FT4, maternal Tg Ab, and newborn total T4 and the studied PCB congeners or ∑PCBs within different BMI groups (Table 5).
After adjustment, in women with pre-pregnancy BMI < 19 group, a 1% increase in the ∑PCBs level was associated with a 0.57% increase in the maternal TSH levels and a 0.47, 0.59, 0.51, and 0.44% increase for a 1% increase in the PCBs 118, 138, 153, and 180, respectively. Maternal TPO Ab levels were statistically significantly increased by 0.53 and 0.46% for a 1% increase in PCB 118 and 153, respectively (Table 6).
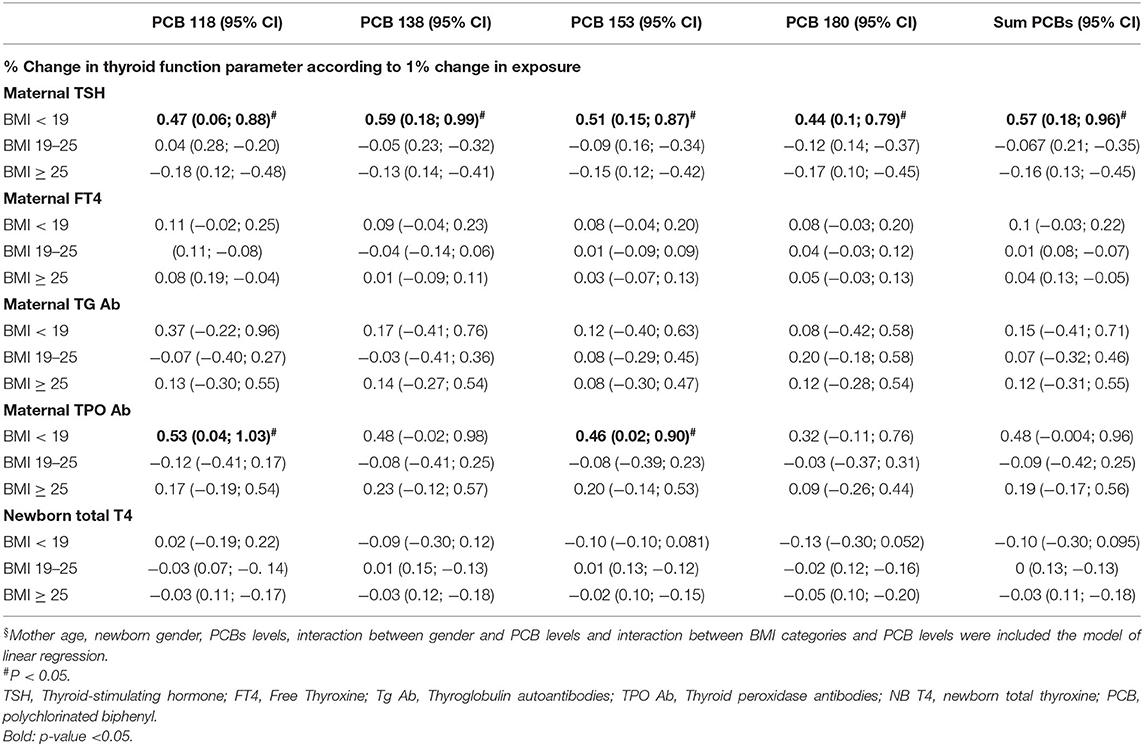
Table 6. Adjusted§ association between maternal PCB concentrations (lipid adj.) to thyroid function of mothers and newborns in the EHF-Assaf-Harofeh-Ichilov birth cohort, Israel, 2013–2015, grouped by maternal pre-pregnancy BMI (n = 157).
In multivariable analysis a 1% change in maternal ∑PCBs level was associated with a small, but statistically significant change in newborn total T4 levels of 0.13% (95% CI 0.04; 0.22) for males, but not females. For each specific PCB congener, the results showed the same trend (Table 7).

Table 7. Adjusted§ association between maternal PCB concentrations (lipid adj.) to total T4 in male newborns in the EHF-Assaf-Harofeh-Ichilov birth cohort, Israel, 2013–2015 (n = 85).
After controlling for maternal age, PCB levels and BMI groups, the association between newborn sex and newborn total T4 was not statistically significant 0.001 95% CI (−0.07; 0.07). The interaction between maternal PCBs and maternal thyroid parameters was not statistically significantly different between males and females. In addition, there was no significant difference in all studied maternal and newborn thyroid parameters between males and females (data not shown).
Discussion
In our birth cohort study of 157 mothers-newborns dyads, we aimed to evaluate the possible associations between background exposure to PCB congeners (118, 138, 153, and 180) at environmentally relevant levels and maternal and newborn thyroid hormones.
A significant positive correlation was found between maternal ∑PCBs and maternal TSH levels in women with pre-pregnancy BMI < 19. Maternal TPO Ab were highly correlated to congeners PCB 118 and 138, approaching significance to PCB153, but not to PCB180 or ∑PCBs in women with pre-pregnancy BMI < 19 (Table 4). No correlation was found in women with normal BMI (19–24.9). In women with BMI ≥ 25, the correlation tends to be negatively associated. In multivariable analysis the same trends were observed for maternal TSH and maternal TPO Ab. After adjustment, in women with pre-pregnancy BMI < 19 group, a 1% increase in the levels of ∑PCBs or either of the studied congeners was associated with a 0.44–0.57% increase in the maternal TSH levels and a 0.46–0.53% increase in maternal TPO Ab. The clinical relevance of this increase is ambiguous since the change is within the normal range, however, the public health relevance on a population level is much greater. Environmentally relevant maternal levels of PCBs are associated with an increase in maternal TPO Ab implying that clinical thyroid disease later in life in a significant proportion of women might be attributable to PCBs. In small observational studies, elevated TPOAb levels have been positively associated with exposure to PCBs (54, 55). PCBs might affect the development of autoimmune thyroid disease (56).
A possible explanation for these results is related to the rate of turnover of adipose tissue. Since PCBs are lipophilic, they have a higher affinity to fat tissue. Theoretically, women with low BMI have a more rapid turnover of PCBs, and a more significant effect is observed compared to women with high BMI (more fat tissue that acts as a “buffer,” slower turnover) (57). Body fat distribution is an important factor underlying metabolic abnormalities. Visceral adipose tissue differs from subcutaneous adipose tissue in its endocrine function, lipolytic activity, and immunologic function (58, 59). Straughen et al. found that visceral adipose tissue increased across pregnancy significantly more rapidly for normal weight compared with overweight/obese women (45).
In our cohort, women with higher BMI were older, but it was the only difference between the BMI groups. No other differences were observed between the groups (Table 1). There were no statistically significant differences between the 3 BMI groups regarding individual PCB levels or ∑PCBs level, maternal TSH, FT4, TPO Ab, and Tg Ab. However, the median TSH levels in women with BMI < 19 were higher −2.79 μIU/ml vs. 2.55 μIU/ml compared to women with BMI ≥ 25, with range (2.49–4.24) vs. (1.95–3.37), respectively. A study from Finland aimed to calculate gestational age-specific thyroid function and the associations between body mass index (BMI) and thyroid hormones, reported that the upper limits for TSH in women with BMI > 30 was higher than in women with BMI 20–25 (60). A study among Thai pregnant women found that BMI was a negative predictor of free T4, although there was no correlation between anti-TPO Abs and BMI (61). In our study, the association was found to be reversed, probably due to exposure to the studied PCBs.
We found no correlation between different PCB congeners or ∑PCBs concentrations in maternal serum and newborn's total T4 levels. Statistically significant correlation was found between PCBs and total T4 in male, but not in female newborns. However, after controlling for maternal age, PCB levels and BMI groups, the association between newborn sex and newborn total T4 was not statistically significant 0.001 95%CI (−0.07; 0.07).
Berg et al. similar to our study, have suggested that background exposure to POPs can alter pregnant women's thyroid function, but in opposite direction (62). They reported that among 19 POPs, eight different PCBs were detected in more than 80% of the samples, including the 4 PCBs tested in our cohort. Several individual PCBs were inversely associated with maternal T3, T4 and FT4, but not with infant TSH. A Canadian cohort study found that PCBs were negatively correlated with total maternal T3 in early pregnancy and with free T3 at delivery, but were not correlated with thyroxin levels (63). Polybrominated diphenyl ethers (PBDEs) and 3 PCBs were tested in this study, the same PCB congeners as in our cohort. A study performed in Michigan residents found a positive association between serum levels of the PCB 118, 138, 153, and 180 congeners and greater total and free T4 and total T3, which were stronger among women (19).
De Cock et al. found no association between cord plasma PCB 153 levels and total T4 in either male or female newborns (64). The Hokkaido birth cohort study (65) reported no significant association between maternal or neonatal TH and total PCBs or non-dioxin-like PCBs. However, they found associations between dioxin-like compounds and elevated neonatal FT4 levels, especially in males. Baba et al. measured multiple octachlorodibenzo-p-dioxin and dibenzofurans (OCDD/Fs) and PCBs, two of these PCBs were measured in our study. Later, the same group published another study examining PCB metabolites—hydroxylated PCBs (OH-PCBs) and maternal and neonatal thyroid hormones (17). They found that maternal exposure to OH-PCBs may increase maternal and neonatal FT4 levels, and a significant positive association was found between maternal ΣOH-PCB and male's FT4 levels, whereas no significant association was observed in female neonates.
While there is substantial evidence for the associations between exposure to PCBs and thyroid function in pregnant women, associations in neonates are more inconsistent. The heterogeneity observed in the studies could be related to differences in design, exposure levels, maternal age, the analytical method used, the period of pregnancy when thyroid hormones were analyzed, differences in the EDC compounds assessed, and the genetic variations. Llop et al. explored the role of genetic polymorphisms in DIO-encoding genes in the association between maternal organochlorine compounds (including PCBs 138, 153, and 180) and thyroid hormone levels (66), suggesting that the magnitude of the association between maternal organochlorine compounds exposure and thyroid parameters, depended on the genotype for the DIO1 gene (encoding iodothyronine deiodinases, important enzymes for the synthesis and determination of TH concentration).
In the last decades, epidemiological studies have reported endocrine and reproductive disorders with persistent organic pollutants (POPs) exposure during early life. The intrauterine period is exceptionally susceptible to chemical exposures and their effects on gene expression with epigenetic alteration such as DNA methylation and chromatin remodeling (67, 68). Mechanisms of PCB neurotoxicity are not fully understood and most probably involve effects on neurotransmitters, hormonal balance, and intracellular signaling (30, 69, 70). Some PCBs have structural similarity to thyroxine (T4), and these compounds can interfere with thyroid hormone production, receptor binding, or transport, resulting in altered hormone levels (71–73). Because thyroid hormones play a significant role in brain development (74), this may explain some of PCBs' neurodevelopmental effects (29, 75). Studies on animals have found allelic differences in the aryl hydrocarbon receptor, which affect sensitivity to PCB exposure, resulting in cognitive deficits and motor dysfunction. This data suggest that the AhR pathway plays a role in PCB neurotoxicity (22).
Maternal PCB serum levels measured in our cohort are consistent with the reduction in PCB levels found in the blood of Israeli donors over the last decades since 1975 (76). The same trend was proven for PCB levels and other contaminants in Israeli mothers' breast milk, with a significant reduction over the years (77, 78). This trend of reduction in contaminations in the environment is explained due to restrictions on Israeli agriculture and industry. PCB levels measured in our cohort are consistent with levels reported in other studies. The data from the MIREC study, a prospective birth cohort study of women from ten Canadian cities between 2008 and 2011, reported mean ∑PCBs (118, 138, 153, 170, 180, 187) concentration of 34.9 ng/g lipids (79).
Our study has several strengths and limitations. Assessment of potential thyroid impairment is difficult due to the thyroid system's complexity, especially during pregnancy. Therefore, we included all significant components in the maternal thyroid homeostasis. As for newborn assessment—we had only the total T4 results without TSH levels from the National survey screening. Total T4 screening may be enough for hypothyroidism screening but might be insufficient to assess the effect of PCBs exposure. A recent study combined three European mother–child cohorts and found an association between exposure to PCB-153, p,p′-DDE and newborn TSH levels (20).
Since pregnancy-specific TSH reference range is not available in our medical center, we used an upper reference limit of ~4.0 μIU/ml, based on the recent recommendation of the American Thyroid Association (ATA). About 20% of all participating women had values above this pregnancy-specific upper limit. For these pregnant women at high risk for thyroid disease the ATA recommends TSH levels be measured with a reflex anti-TPO antibody if TSH is 2.5–10 μIU/ml (80). In our cohort, only three women were TPO Ab positive, two of them had TSH levels above 2.5 μIU/ml, and only one was Tg Ab positive.
PCBs are a large family of 209 congeners, our study tested only 4 congeners. However, PCBs 118, 138, 153, 180, are among the most frequently detected congeners in white adipose tissue and blood samples (9, 10). These PCBs have the highest detection frequencies in the US population and have a half-life of years.
It has been recommended that regression modeling be carried out both with and without lipid correction for lipophilic compounds in the blood (50), but it has rarely been followed, and lipid-based models are commonly used. PCBs are typically associated with the lipid matrix in tissues because of their non-polar and lipophilic character, and the results may be expressed per weight of lipid rather than on a whole weight or a volume basis. If equilibrium is reached, information regarding serum PCB levels and serum lipid levels may be predictive of PCB body burden (81). Total lipids were calculated for the mothers and no statistically significant differences were observed between the three BMI groups (p = 0.06). Moreover, we performed the correlation analysis between the wet-weighted PCBs (not lipid adjusted) to the thyroid parameters of mother and newborn. The same trends as in lipid-adjusted correlations were observed (Supplementary Tables 2, 3). There are physiological variations in lipid metabolism during pregnancy, with a marked increase of lipids levels during the third trimester of pregnancy (82). Considering the changes in lipid metabolism during pregnancy, the use of a lipid-adjusted model is appropriate in our setup.
Conclusions
The present study may suggest that background low-dose exposure to some PCBs can alter thyroid hormone homeostasis in pregnant women, and might be associated with abnormal TSH levels and TPO-Ab in women with low BMI. However, in women with high BMI (>25), this trend is inverse.
Forthcoming, the remaining samples of recruited mother-father-infant triads will be studied including blood, urine, cord blood, breast milk and meconium, for concentrations of PCBs and other contaminants. Additionally, our cohort of children is being followed prospectively for future studies to evaluate developmental outcomes.
Data Availability Statement
The datasets presented in this study can be found in online repositories. The names of the repository/repositories and accession number(s) can be found below: https://ipchem.jrc.ec.europa.eu/#discovery.
Ethics Statement
The studies involving human participants were reviewed and approved by Helsinki committee, Shamir Medical Center (Assaf Harofeh). Written informed consent to participate in this study was provided by the participants' legal guardian/next of kin. Written informed consent was obtained from the individual(s), and minor(s)' legal guardian/next of kin, for the publication of any potentially identifiable images or data included in this article.
Author Contributions
MBer, DB, AB, EK, AL, RKe, JT, MBet, MM, AO, PF-L, MBr, TZ-B, MBerk, IM, and RM: substantial contributions to the conception or design of the work, or the acquisition, analysis, or interpretation of data for the work. MBer, DB, DM, RL, PF-L, TZ-B, RKo, CK, MBerk, IM, and RM: drafting the work or revising it critically for important intellectual content. MBer, MBerk, and RM: agree to be accountable for all aspects of the work in ensuring that questions related to the accuracy or integrity of any part of the work are appropriately investigated and resolved. All authors contributed to the article and approved the submitted version.
Funding
This work was supported by the Environment and Health Fund (EHF)-Grant No. RGA1202.
Conflict of Interest
The authors declare that the research was conducted in the absence of any commercial or financial relationships that could be construed as a potential conflict of interest.
Publisher's Note
All claims expressed in this article are solely those of the authors and do not necessarily represent those of their affiliated organizations, or those of the publisher, the editors and the reviewers. Any product that may be evaluated in this article, or claim that may be made by its manufacturer, is not guaranteed or endorsed by the publisher.
Supplementary Material
The Supplementary Material for this article can be found online at: https://www.frontiersin.org/articles/10.3389/fped.2021.705395/full#supplementary-material
References
1. Trasande L, Zoeller RT, Hass U, Kortenkamp A, Grandjean P, Myers JP, et al. Estimating burden and disease costs of exposure to endocrine-disrupting chemicals in the European Union. J Clin Endocrinol Metab. (2015) 100:1245–55. doi: 10.1210/jc.2014-4324
2. Gore AC, Chappell VA, Fenton SE, Flaws JA, Nadal A, Prins GS, et al. EDC-2: the endocrine society's second scientific statement on endocrine-disrupting chemicals. Endocr Rev. (2015) 36:E1–150. doi: 10.1210/er.2015-1010
3. Agency for Toxic Substances and Disease Registry. Toxicological Profile for Polychlorinated Biphenyls (PCBs)-Update. Atlanta (2000).
4. World Health Organization United Nations Environment Programme Inter-Organization Programme for the Sound Management of Chemicals, Bergman, Åke, Heindel Jerrold J, et al. State of the Science of Endocrine Disrupting Chemicals 2012: Summary for Decision-Makers. World Health Organization. (2013). Available online at: https://apps.who.int/iris/handle/10665/78102
5. United Nations Environment Programme (UNEP). Stockholm Convention on Persistent Organic Pollutants (POPs). Secr Stock Conv. (2018)
6. Domingo JL. Polybrominated diphenyl ethers in food and human dietary exposure: a review of the recent scientific literature. Food Chem Toxicol. (2012) 50:238–49. doi: 10.1016/j.fct.2011.11.004
7. Freels S, Chary LK, Turyk M, Piorkowski J, Mallin K, Dimos J, et al. Congener profiles of occupational PCB exposure versus PCB exposure from fish consumption. Chemosphere. (2007) 69:435–43. doi: 10.1016/j.chemosphere.2007.04.087
8. Fernández-González R, Yebra-Pimentel I, Martínez-Carballo E, Simal-Gándara J. A critical review about human exposure to Polychlorinated Dibenzo-p-Dioxins (PCDDs), Polychlorinated Dibenzofurans (PCDFs) and Polychlorinated Biphenyls (PCBs) through Foods. Crit Rev Food Sci Nutr. (2015) 55:1590–617. doi: 10.1080/10408398.2012.710279
9. Müllerová D, Kopecký J. White adipose tissue: storage and effector site for environmental pollutants. Physiol Res. (2007) 56:375–81.
10. Bonefeld-Jorgensen EC, Andersen HR, Rasmussen TH, Vinggaard AM. Effect of highly bioaccumulated polychlorinated biphenyl congeners on estrogen and androgen receptor activity. Toxicology. (2001) 158:141–53. doi: 10.1016/S0300-483X(00)00368-1
11. Longnecker MP, Wolff MS, Gladen BC, Brock JW, Grandjean P, Jacobson JL, et al. Comparison of polychlorinated biphenyl levels across studies of human neurodevelopment. Environ Health Perspect. (2003) 111:65–70. doi: 10.1289/ehp.5463
12. Seegal RF, Fitzgerald EF, Hills EA, Wolff MS, Haase RF, Todd AC, et al. Estimating the half-lives of PCB congeners in former capacitor workers measured over a 28-year interval. J Expo Sci Environ Epidemiol. (2011) 21:234–46. doi: 10.1038/jes.2010.3
13. Faroon O, Ruiz P. Polychlorinated biphenyls: new evidence from the last decade. Toxicol Ind Health. (2015) 32:1825–47. doi: 10.1177/0748233715587849
14. Steinberg RM, Walker DM, Juenger TE, Woller MJ, Gore AC, Steinberg RM, et al. Effects of perinatal polychlorinated biphenyls on adult female rat reproduction: development, reproductive physiology, and second generational effects. Biol Reprod. (2008) 78:1091–101. doi: 10.1095/biolreprod.107.067249
15. Dickerson SM, Cunningham SL, Gore AC, Dickerson SM, Cunningham SL, Gore AC. Prenatal PCBs disrupt early neuroendocrine development of the rat hypothalamus. Toxicol Appl Pharmacol. (2011) 252:36–46. doi: 10.1016/j.taap.2011.01.012
16. Dirinck E, Dirtu AC, Malarvannan G, Covaci A, Jorens PG, Van Gaal LF. A preliminary link between hydroxylated metabolites of polychlorinated biphenyls and free thyroxin in humans. Int J Environ Res Public Health. (2016) 13:421. doi: 10.3390/ijerph13040421
17. Itoh S, Baba T, Yuasa M, Miyashita C, Kobayashi S, Araki A, et al. Association of maternal serum concentration of hydroxylated polychlorinated biphenyls with maternal and neonatal thyroid hormones: the Hokkaido birth cohort study. Environ Res. (2018) 167:583–90. doi: 10.1016/j.envres.2018.08.027
18. Hosie S, Loff S, Witt K, Niessen K, Waag KL. Is there a correlation between organochlorine compounds and undescended testes? Eur J Pediatr Surg. (2000) 10:304–9. doi: 10.1055/s-2008-1072381
19. Jacobson MH, Darrow LA, Barr DB, Howards PP, Lyles RH, Terrell ML, et al. Serum polybrominated biphenyls (PBBs) and polychlorinated biphenyls (PCBs) and thyroid function among michigan adults several decades after the 1973–1974 pbb contamination of livestock feed. Environ Health Perspect. (2017) 125:097020. doi: 10.1289/EHP1302
20. De Cock M, De Boer MR, Govarts E, Iszatt N, Palkovicova L, Lamoree MH, et al. Thyroid-stimulating hormone levels in newborns and early life exposure to endocrine-disrupting chemicals: analysis of three European mother-child cohorts. Pediatric Res. (2017) 82:429–37. doi: 10.1038/pr.2017.50
21. Li Z-M, Hernandez-Moreno D, Main KM, Skakkebæk NE, Kiviranta H, Toppari J, et al. Association of In Utero persistent organic pollutant exposure with placental thyroid hormones. Endocrinology. (2018) 159:3473–81. doi: 10.1210/en.2018-00542
22. Klinefelter K, Hooven MK, Bates C, Colter BT, Dailey A, Infante SK, et al. Genetic differences in the aryl hydrocarbon receptor and CYP1A2 affect sensitivity to developmental polychlorinated biphenyl exposure in mice: relevance to studies of human neurological disorders. Mamm Genome. (2018) 29:112–27. doi: 10.1007/s00335-017-9728-1
23. Kahn LG, Philippat C, Nakayama SF, Slama R, Trasande L. Endocrine-disrupting chemicals: implications for human health. Lancet Diabetes Endocrinol. (2020) 8:703–18. doi: 10.1016/S2213-8587(20)30129-7
24. Thangavelu SK, Elaiyapillai SP, Ramachandran I, Bhaskaran RS, Jagadeesan A. Lactational exposure of polychlorinated biphenyls impair Leydig cellular steroidogenesis in F1 progeny rats. Reprod Toxicol. (2018) 75:73–85. doi: 10.1016/j.reprotox.2017.11.009
25. Park WH, Kang S, Lee HK, Salihovic S, Bavel B van, Lind PM, et al. Relationships between serum-induced AhR bioactivity or mitochondrial inhibition and circulating polychlorinated biphenyls (PCBs). Sci Rep. (2017) 7:9383. doi: 10.1038/s41598-017-09774-1
26. Miyazaki W, Iwasaki T, Takeshita A, Tohyama C, Koibuchi N. Identification of the functional domain of thyroid hormone receptor responsible for polychlorinated biphenyl-mediated suppression of its action in vitro. Environ Health Perspect. (2008) 116:1231–6. doi: 10.1289/ehp.11176
27. Boas M, Feldt-Rasmussen U, Main K. Thyroid effects of endocrine disrupting chemicals. Mol Cell Endocrinol. (2012) 355:240–8. doi: 10.1016/j.mce.2011.09.005
28. Curtis SW, Terrell ML, Jacobson MH, Cobb DO, Jiang VS, Neblett MF, et al. Thyroid hormone levels associate with exposure to polychlorinated biphenyls and polybrominated biphenyls in adults exposed as children. Environ Health. (2019) 18:75. doi: 10.1186/s12940-019-0509-z
29. Pinson A, Bourguignon JP, Parent AS. Exposure to endocrine disrupting chemicals and neurodevelopmental alterations. Andrology. (2016) 4:706–22. doi: 10.1111/andr.12211
30. Pessah IN, Lein PJ, Seegal RF, Sagiv SK. Neurotoxicity of polychlorinated biphenyls and related organohalogens. Acta Neuropathol. (2019) 138:363–87. doi: 10.1007/s00401-019-01978-1
31. Panesar HK, Kennedy CL, Stietz KPK, Lein PJ. Polychlorinated Biphenyls (PCBs): risk factors for autism spectrum disorder? Toxics. (2020) 8:70. doi: 10.3390/toxics8030070
32. Manoj K, Kumar SD, Swasti S, Manoj K, Vinod V, Anil P, et al. Environmental endocrine-disrupting chemical exposure: role in non-communicable diseases. Front Public Health. (2020) 8:553850. doi: 10.3389/fpubh.2020.553850
33. Pearce EN, Lazarus JH, Moreno-Reyes R, Zimmermann MB. Consequences of iodine deficiency and excess in pregnant women: an overview of current knowns and unknowns. Am J Clin Nutr. (2016) 104:918S−23S. doi: 10.3945/ajcn.115.110429
34. Haddow J, Palomaki G, Allan W, Williams J, Knight G, Gagnon J, et al. Maternal thyroid deficiency during pregnancy and subsequent neuropsychological development of the child. N Engl J Med. (1999) 341:549–55. doi: 10.1056/NEJM199908193410801
35. Teysseire R, Brochard P, Sentilhes L, Delva F. Identification and prioritization of environmental reproductive hazards: a first step in establishing environmental perinatal care. Int J Environ Res Public Health. (2019) 16:366. doi: 10.3390/ijerph16030366
36. Di Renzo GC, Conry JA, Blake J, Defrancesco MS, Denicola N, Martin JN, et al. International federation of gynecology and obstetrics opinion on reproductive health impacts of exposure to toxic environmental chemicals. Int J Gynecol Obstet. (2015) 131:219–25. doi: 10.1016/j.ijgo.2015.09.002
37. Sharpe RM, Irvine DS. How strong is the evidence of a link between environmental chemicals and adverse effects on human reproductive health? Br Med J. (2004) 328:447–51. doi: 10.1136/bmj.328.7437.447
38. Sly PD, Flack F. Susceptibility of children to environmental pollutants. Ann N Y Acad Sci. (2008) 1140:163–83. doi: 10.1196/annals.1454.017
39. Sagiv SK, Tolbert PE, Altshul LM, Korrick SA. Organochlorine exposures during pregnancy and infant size at birth. Epidemiology. (2007) 18:120–9. doi: 10.1097/01.ede.0000249769.15001.7c
40. Gilbert ME, Rovet J, Chen Z, Koibuchi N. Developmental thyroid hormone disruption: prevalence, environmental contaminants and neurodevelopmental consequences. Neurotoxicology. (2012) 33:842–52. doi: 10.1016/j.neuro.2011.11.005
41. Den Hond E, Roels HA, Hoppenbrouwers K, Nawrot T, Thijs L, Vandermeulen C, et al. Sexual maturation in relation to polychlorinated aromatic hydrocarbons: sharpe and Skakkebaek's hypothesis revisited. Environ Health Perspect. (2002) 110:771–6. doi: 10.1289/ehp.02110771
42. Hertz-Picciotto I, Park HY, Dostal M, Kocan A, Trnovec T, Sram R. Prenatal exposures to persistent and non-persistent organic compounds and effects on immune system development. Basic Clin Pharmacol Toxicol. (2008) 102:146–54. doi: 10.1111/j.1742-7843.2007.00190.x
43. Gladen BC, Rogan WJ, Hardy P, Thullen J, Tingelstad J, Tully M. Development after exposure to polychlorinated biphenyls and dichlorodiphenyl dichloroethene transplacentally and through human milk. J Pediatr. (1988) 113:991–5. doi: 10.1016/S0022-3476(88)80569-9
44. Veltri F, Poppe K. Variables contributing to thyroid (Dys)function in pregnant women: more than thyroid antibodies? Eur Thyroid J. (2018) 7:120–8. doi: 10.1159/000488279
45. Straughen JK, Trudeau S, Misra VK. Changes in adipose tissue distribution during pregnancy in overweight and obese compared with normal weight women. Nutr Diabetes. (2013) 3:e84. doi: 10.1038/nutd.2013.25
46. Lederman SA, Paxton A, Heymsfield SB, Wang J, Thornton J, Pierson J. Maternal body fat and water during pregnancy: do they raise infant birth weight? Am J Obstet Gynecol. (1999) 180:235–40. doi: 10.1016/S0002-9378(99)70181-X
47. Dollberg S, Haklai Z, Mimouni FB, Gorfein I, Gordon ES. Birthweight standards in the live-born population in Israel. Isr Med Assoc J. (2005) 7:311–4.
48. World Medical Association. World Medical Association Declaration of Helsinki: ethical principles for medical research involving human subjects. JAMA. (2013) 310:2191–4. doi: 10.1001/jama.2013.281053
49. Sheinberg R, Siegel EL, Keidar R, Mandel D, Lubetzky R, Kohn E, et al. Associations between intrauterine exposure to polychlorinated biphenyls on neonatal ano-genital distance. Reprod Toxicol. (2020) 96:67–75. doi: 10.1016/j.reprotox.2020.06.005
50. Schisterman EF, Whitcomb BW, Buck Louis GM, Louis TA. Lipid adjustment in the analysis of environmental contaminants and human health risks. Environ Health Perspect. (2005) 113:853–7. doi: 10.1289/ehp.7640
51. Bernert JT, Turner WE, Patterson DG, Needham LL. Calculation of serum “total lipid” concentrations for the adjustment of persistent organohalogen toxicant measurements in human samples. Chemosphere. (2007) 68:824–31. doi: 10.1016/j.chemosphere.2007.02.043
52. Bergonzi R, De Palma G, Tomasi C, Ricossa MC, Apostoli P. Evaluation of different methods to determine total serum lipids for normalization of circulating organochlorine compounds. Int Arch Occup Env Health. (2009) 82:1241–7. doi: 10.1007/s00420-009-0426-5
53. Figueiras A, Domenech-Massons JM, Cadarso C. Regression models: calculating the confidence interval of effects in the presence of interactions. Stat Med. (1998) 17:2099–105. doi: 10.1002/(sici)1097-0258(19980930)17:18<2099::aid-sim905>3.0.co;2-6
54. Langer P, Kocan A, Tajtaková M, Petrík J, Chovancová J, Drobná B, et al. Fish from industrially polluted freshwater as the main source of organochlorinated pollutants and increased frequency of thyroid disorders and dysglycemia. Chemosphere. (2007) 67:S379–85. doi: 10.1016/j.chemosphere.2006.05.132
55. Schell LM, Gallo MV, Ravenscroft J, DeCaprio AP. Persistent organic pollutants and anti-thyroid peroxidase levels in Akwesasne Mohawk young adults. Environ Res. (2009) 109:86–92. doi: 10.1016/j.envres.2008.08.015
56. Benvenga S, Antonelli A, Vita R. Thyroid nodules and thyroid autoimmunity in the context of environmental pollution. Rev Endocr Metab Disord. (2015) 16:319–40. doi: 10.1007/s11154-016-9327-6
57. Frayn K. Adipose tissue as a buffer for daily lipid flux. Diabetologia. (2002) 45:1201–10. doi: 10.1007/s00125-002-0873-y
58. Jensen MD. Role of body fat distribution and the metabolic complications of obesity. J Clin Endocrinol Metab. (2008) 93:S57–63. doi: 10.1210/jc.2008-1585
59. Ibrahim MM. Subcutaneous and visceral adipose tissue: structural and functional differences. Obes Rev. (2010) 11:11–8. doi: 10.1111/j.1467-789X.2009.00623.x
60. Männistö T, Surcel HM, Ruokonen A, Vääräsmäki M, Pouta A, Bloigu A, et al. Early pregnancy reference intervals of thyroid hormone concentrations in a thyroid antibody-negative pregnant population. Thyroid. (2011) 21:291–8. doi: 10.1089/thy.2010.0337
61. Gowachirapant S, Melse-Boonstra A, Winichagoon P, Zimmermann MB. Overweight increases risk of first trimester hypothyroxinaemia in iodine-deficient pregnant women. Matern Child Nutr. (2014) 10:61–71. doi: 10.1111/mcn.12040
62. Berg V, Nøst TH, Pettersen RD, Hansen S, Veyhe AS, Jorde R, et al. Persistent organic pollutants and the association with maternal and infant thyroid homeostasis: a multipollutant assessment. Environ Health Perspect. (2017) 125:127–33. doi: 10.1289/EHP152
63. Abdelouahab N, Langlois M-F, Lavoie L, Corbin F, Pasquier J-C, Takser L. Maternal and cord-blood thyroid hormone levels and exposure to polybrominated diphenyl ethers and polychlorinated biphenyls during early pregnancy. Am J Epidemiol. (2013) 178:701–13. doi: 10.1093/aje/kwt141
64. De Cock M, De Boer MR, Lamoree M, Legler J, Van De Bor M. Prenatal exposure to endocrine disrupting chemicals in relation to thyroid hormone levels in infants-a Dutch prospective cohort study. Environ Health. (2014) 13:106. doi: 10.1186/1476-069X-13-106
65. Baba T, Ito S, Yuasa M, Yoshioka E, Miyashita C, Araki A, et al. Association of prenatal exposure to PCDD_Fs and PCBs with maternal and infant thyroid hormones the Hokkaido study on environment and children's health. Sci Total Environ. (2018) 615:1239–46. doi: 10.1016/j.scitotenv.2017.09.038
66. Llop S, Murcia M, Alvarez-Pedrerol M, Grimalt JO, Santa-Marina L, Julvez J, et al. Association between exposure to organochlorine compounds and maternal thyroid status: role of the iodothyronine deiodinase 1 gene. Environ Int. (2017) 104:83–90. doi: 10.1016/j.envint.2016.12.013
67. Grandjean P, Bellinger D, Bergman Å, Cordier S, Davey-Smith G, Eskenazi B, et al. The faroes statement: human health effects of developmental exposure to chemicals in our environment. Basic Clin Pharmacol Toxicol. (2008) 102:73–5. doi: 10.1111/j.1742-7843.2007.00114.x
68. Toraño EG, García MG, Luis Fernández-Morera J, Niño-García P, Fernández AF. The impact of external factors on the epigenome: in utero and over Lifetime. Biomed Res Int. (2016) 2016:2568635. doi: 10.1155/2016/2568635
69. Westerink RHS. Modulation of cell viability, oxidative stress, calcium homeostasis, and voltage- and ligand-gated ion channels as common mechanisms of action of (mixtures of) non-dioxin-like polychlorinated biphenyls and polybrominated diphenyl ethers. Environ Sci Pollut Res. (2014) 21:6373–83. doi: 10.1007/s11356-013-1759-x
70. Klocke C, Lein PJ. Evidence implicating non-dioxin-like congeners as the key mediators of polychlorinated biphenyl (Pcb) developmental neurotoxicity. Int J Mol Sci. (2020) 21:1013. doi: 10.3390/ijms21031013
71. Cheek AAO, Kow K, Chen J, McLachlan JA. Potential mechanisms of thyroid disruption in humans, interaction of organochlorine compounds with thyroid receptor, transthyretin, and thyroid-binding globlin. Environ Health Perspect. (1999) 107:273–8. doi: 10.1289/ehp.99107273
72. Brouwer A, Morse DC, Lans MC, Gerlienke Schuur A, Murk AJ, Klasson-Wehler E, et al. Interactions of persistent environmental organohalogens with the thyroid hormone system: mechanisms and possible consequences for animal and human health. Toxicol Ind Health. (1998) 14:59–84. doi: 10.1177/074823379801400107
73. Zoeller RT, Dowling ALS, Vas AA. Developmental exposure to polychlorinated biphenyls exerts thyroid hormone-like effects on the expression of RC3/neurogranin and myelin basic protein messenger ribonucleic acids in the developing rat brain. Endocrinology. (2000) 141:181–9. doi: 10.1210/endo.141.1.7273
74. Williams GR. Neurodevelopmental and neurophysiological actions of thyroid hormone. J Neuroendocrinol. (2008) 20:784–94. doi: 10.1111/j.1365-2826.2008.01733.x
75. Winneke G, Walkowiak J, Lilienthal H. PCB-induced neurodevelopmental toxicity in human infants and its potential mediation by endocrine dysfunction. Toxicology. (2002) 181–182:161–5. doi: 10.1016/S0300-483X(02)00274-3
76. Pines A, Cucos S, Ever-Hadani P, Ron M, Lemesch C. Changes in pattern of organochlorine residues in blood of general Israeli population, 1975–1986. Sci Total Environ. (1987) 66:115–25. doi: 10.1016/0048-9697(87)90081-7
77. Polishuk ZW, Ron M, Wassermann M, Cucos S, Wassermann D, Lemesch C. Organochlorine compounds in mother and fetus during labor. Environ Res. (1977) 13:278–84. doi: 10.1016/0013-9351(77)90104-9
78. Slorach SA, Vaz R. PCB levels in breast milk: data from the UNEP/WHO pilot project on biological monitoring and some other recent studies. Environ Health Perspect. (1985) 60:121–6. doi: 10.1289/ehp.8560121
79. Bernardo B, Lanphear B, Venners S, Arbuckle T, Braun J, Muckle G, et al. Assessing the relation between plasma PCB concentrations and elevated autistic behaviours using bayesian predictive odds ratios. Int J Environ Res Public Health. (2019) 16:457. doi: 10.3390/ijerph16030457
80. Alexander EK, Pearce EN, Brent GA, Brown RS, Chen H, Dosiou C, et al. 2017 Guidelines of the American Thyroid Association for the diagnosis and management of thyroid disease during pregnancy and the postpartum. Thyroid. (2017) 27:315–89. doi: 10.1089/thy.2016.0457
81. Brown JF, Lawton RW. Polychlorinated biphenyl (PCB) partitioning between adipose tissue and serum. Bull Environ Contam Toxicol. (1984) 33:277–80. doi: 10.1007/BF01625543
Keywords: polychlorinated biphenyls (PCBs), endocrine-disrupting chemicals (EDCs), thyroid hormones, pregnancy, intrauterine exposure
Citation: Berlin M, Barchel D, Brik A, Kohn E, Livne A, Keidar R, Tovbin J, Betser M, Moskovich M, Mandel D, Lubetzky R, Ovental A, Factor-Litvak P, Britzi M, Ziv-Baran T, Koren R, Klieger C, Berkovitch M, Matok I and Marom R (2021) Maternal and Newborn Thyroid Hormone, and the Association With Polychlorinated Biphenyls (PCBs) Burden: The EHF (Environmental Health Fund) Birth Cohort. Front. Pediatr. 9:705395. doi: 10.3389/fped.2021.705395
Received: 09 May 2021; Accepted: 20 August 2021;
Published: 13 September 2021.
Edited by:
Ronald Cohen, University of Chicago, United StatesReviewed by:
Robert Sargis, University of Illinois at Chicago, United StatesMichelle Lemelman, University of Chicago, United States
Copyright © 2021 Berlin, Barchel, Brik, Kohn, Livne, Keidar, Tovbin, Betser, Moskovich, Mandel, Lubetzky, Ovental, Factor-Litvak, Britzi, Ziv-Baran, Koren, Klieger, Berkovitch, Matok and Marom. This is an open-access article distributed under the terms of the Creative Commons Attribution License (CC BY). The use, distribution or reproduction in other forums is permitted, provided the original author(s) and the copyright owner(s) are credited and that the original publication in this journal is cited, in accordance with accepted academic practice. No use, distribution or reproduction is permitted which does not comply with these terms.
*Correspondence: Matitiahu Berkovitch, mberkovitch@shamir.gov.il