- 1Department of Rehabilitation Medicine, Catholic University of Daegu School of Medicine, Daegu, South Korea
- 2Department of Pediatrics, Catholic University of Daegu School of Medicine, Daegu, South Korea
Objective: To determine whether a portable microcurrent therapy device (PMTD) of the rectus abdominis muscles is effective for treating desaturation during feeding in preterm infants and to evaluate the association between initial electrical activity of respiratory muscle and long-term development delay.
Methods: Twenty preterm infants with desaturation during feeding were recruited. Respiratory muscle activity was quantified by calculating the root mean square (RMS) of the electromyography. All preterm infants received a 30 min PMTD application to the rectus abdominis and diaphragm daily for 2 weeks. RMS of diaphragm and rectus abdominis, feeding volume, frequency of desaturation during feeding at baseline (pre-PMTD) and 1, 2 week post-PMTD were measured. The number of days it took to treat desaturation after PMTD was measured. A Denver developmental screening test was performed and infants were divided into 3 groups: (1) normal; (2) caution; and (3) delayed at 3months after PMTD.
Results: The desaturation during feeding of all the preterm infants subsided after PMTD and the mean days took to treat desaturation was 25.4 ± 14.2 days. The RMS of diaphragm, rectus abdominis, and frequency of desaturation during feeding were significantly decreased and the feeding volume was significantly increased after PMTD (p < 0.01). The mean treatment duration for desaturation was negatively correlated with RMS of rectus abdominis at baseline and 1 week post-PMTD, respectively (Pearson's correlation coefficient = −0.461,−0.514, p-value = 0.047, 0.029). RMS of rectus abdominis of Group 3 is lower than that of group 1 and 2 (p < 0.01).
Conclusions: This pilot study showed that the microcurrent therapy of rectus abdominis is an efficient therapy for the treatment of preterm infants with desaturation during feeding, especially preterm infants with higher activity of the rectus abdominis. In preterm infants with lower rectus abdominis activity, longer time is required to treat desaturation by microcurrent therapy and developmental delay is observed at months post-treatment.
Introduction
In premature infants, coordination of sucking, swallowing, and breathing rhythms is critical for successful suckle feeding (1, 2). Immature coordination, gastroesophageal reflux, immature oral structures, and a combination of conditions can increase the risks of desaturation while feeding (3–9). A clinically significant oxygen desaturation phenomenon occurs when there is any reduction in oxygen saturation < 90% for 1 second or longer (10). Moreover, although preterm infants can also experience impaired lung function during gavage feeding, desaturation can occur more frequently during bottle feeding, particularly when there is an extant gavage tube (3, 11–14). Premature infants are frequently fed using these methods. Immature incoordination of suck-swallow-breathing can cause frequent desaturation, which can affect multiple organs (e.g., heart, lungs, and brain), impacting the subsequent infant growth and development (15–18).
Evidence suggests that diaphragmatic fatigue is involved in the pathogenesis of desaturation in premature infants (19–21). Paradoxical breathing is common in infants and is particularly notable in preterm infants as a result of their highly compliant chest wall (4). Reports suggest that this defect enhances volume displacement of the diaphragm during inspiration and requires substantial effort and energy, as well as promoting diaphragmatic fatigue and desaturation. Gewolb and Vice (22) demonstrated that hypoxemic episode duration and severity were related to simultaneous abdominal muscle contractions following mechanical ventilation in preterm infants. Moreover, delayed lung inflation following abdominal muscle contraction eventually results in a reduction in the lung volume below baseline.
Furthermore, a previous study reported that preterm infants with desaturation during feeding showed high electrical activity in the diaphragm and rectus abdominis muscle (23). They assumed that excessive contraction of diaphragm and rectus abdominis muscle lead to diaphragm fatigue and feeding desaturation. Therefore, they suggest that reducing the excessive activity of abdominal muscle may effectively control feeding desaturation in preterm infants.
Microcurrent electrical stimulation represents a physical modality for the delivery of current in the microampere range. No adverse effects have been associated with microcurrent stimulation as it works at the microampere level and simulates the electrical intensity of living tissue (24–28). A previous study demonstrated that microcurrent therapy markedly reduced the muscle electrical activity and increased muscle power efficiently through minimal muscle recruitment (29).
Taking into account the importance of safe and effective treatment for preterm infant with desaturation during feeding, and in light of the aforementioned issues: we aimed to explore the association between initial electrical activity of respiratory muscle and long-term development delay and the efficacy of microcurrent treatment in these patients. To our knowledge, this preliminary report is the first to investigate this convenient treatment method in preterm infant with desaturation during feeding.
Materials and Methods
Participants
This was a prospective, single arm, pilot study. A total of 79 preterm infants were enrolled with desaturation during feeding who underwent treatment in neonatal intensive care unit, 59 of which the desaturation resolved spontaneously before 35 weeks of gestation. There were 20 preterm infants with respiratory distress syndrome referred from the pediatrics department to rehabilitation medicine department regarding desaturation during feeding. These infants were recruited to the present study, between May 2015 and March 2016 (Table 1).
Significant feeding desaturation was defined as an arterial oxygen saturation level using a pulse oximeter < 85% for longer than 2 s (moderate to severe desaturation) (10, 16, 23). None of the infants exhibited concurrent sepsis or craniofacial anomalies. All infants were bottle-fed during their scheduled feeding times by a skilled nurse with over 2-3 years of experience in a neonatal intensive care unit. In infants aged < 3 months, a small nipple size (Aissok, Greenmom, Anyang, Korea) was used for each feeding. Gavage-fed infants were excluded from the study. The study was approved by the Institutional Review Board and Ethics Committee of University Medical Centre (IRB number: MDCR-15-011). Written informed consent was granted by the parents or guardians of the infants.
Outcome Measurements
Surface electrodes were used to record the electromyographic (EMG) activity of the diaphragm and rectus abdominis muscle as described previously (1) using a four-channel electrophysiology unit (Medelec, Company of Oxford, Oxford, UK). Surface electrodes were placed in the eighth intercostal space between the mid-clavicular and mid-axillary line to measure the electrical activity of the diaphragm muscles. Surface electrodes were placed 2 cm laterally to the umbilicus to measure the activity of the rectus abdominis muscles. The electromyographic (EMG) activity of the muscles was recorded for 1 h at resting state after feeding. The level of respiratory muscle activity was quantified by calculating the root mean square (RMS) envelope of the EMG signal. The RMS of the diaphragm and rectus abdominis was measured. The brain imaging study (ultrasound or magnetic resonance imaging) was reviewed. Feeding volume at each feed and frequency of desaturation during feeding was measured. Treatment duration was defined as the time between the initial treatment and achieving no desaturation event during feeding. There was a loss to follow up at 3 months after treatment, for 9 infants. In 11 infants, the Denver developmental screening test II (DDST) was performed (6). The DDST includes four developmental elements: social contact, language, fine motor skills, and gross motor skills. Infants were divided into three groups: group 1 (n = 4), normal; group 2 (n = 4), caution; and group 3 (n = 3), delayed, according to guidelines (30, 31).
Treatment
All preterm infants received intensive physical therapy and microcurrent therapy in the ICU. Physical therapy consisted of oromotor stimulation, respiratory muscle stretching and relaxation, and trunk stabilization. Microcurrent therapy was applied to the front and back of body to treat diaphragm and rectus abdominus. The front patch placed to 1 cm above the umbilicus on midline. A back patch was placed at the same location on the back (Figure 1). All infants received 2 weeks of daily treatment with a small and portable microcurrent therapy device (intensity, 25μA; frequency, 8 Hz; Granthe, Cosmic Co., Seoul, Korea) for 30 min. The current intensity was significantly lower than each infant's sensation threshold. The microcurrent generator provided an alternating current using a monophasic rectangular pulse format with a reversal in polarity every 2 s.
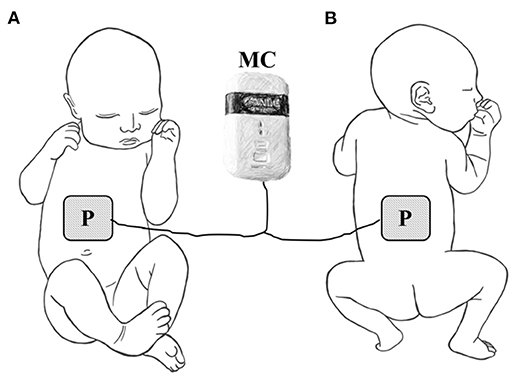
Figure 1. Placement of electrode for microcurrent therapy on (A) the front side of the infant or (B) the back of the infant. P, electrode patch, MC, microcurrent generator.
Statistical Analysis
A repeated measured one-way ANOVA was used to evaluate the change in EMG activity. A Pearson's correlation analysis and Spearman's correlation analysis were used to calculate the correlation between numeric variables. The association between DDST and other variables was evaluated using a one-way ANOVA and Kruskal-Wallis test.
Result
A total of 20 preterm infants (mean age: 37.0 ± 1.3 weeks; mean weight: 2483.0 ± 375.7 g; 11 males and 9 females) were enrolled. There were 8 out of 20 infants with brain lesions, including four with periventricular leukomalacia, two with intraventricular hemorrhage, and two with grade I germinal matrix hemorrhage. All preterm infant's desaturation during feeding had subsided after treatment. The mean duration of treatment was 25.4 ± 14.2 days.
The RMS of diaphragm and rectus abdominis muscle decreased continuously during treatment (Table 2) (p < 0.01).
The mean duration of treatment was negatively correlated with the RMS of rectus abdominis at baseline and 1 week after treatment (Pearson's correlation coefficient = −0.461,−0.514 p-value = 0.047, 0.029) (Table 3). RMS of rectus abdominis of Group 3 was lower than that of group 1 and 2 (p < 0.01) (Table 4).
There was no correlation between gestational age, weight, brain lesion, APGAR score, RMS of the diaphragm and treatment duration (Table 3). There was no association between gestational age, weight, brain lesion, APGAR score, RMS of diaphragm and developmental delay (Table 4).
Discussion
The current study explored the efficacy of microcurrent stimulation of preterm infants with desaturation during feeding. Our preliminary results show that patients had decreased RMS of diaphragm and rectus abdominis muscle and improved oxygen saturation. To the best of our knowledge, these data are the first to report the effectiveness of microcurrent in preterm infants with desaturation during feeding.
Two mechanisms of microcurrent therapy have been suggested regarding the treatment of desaturation during feeding: 1) the therapeutic effect may be related to the maintenance of intracellular Ca2+ homeostasis in muscle (24, 32). Moreover, abdominal muscle contractions in preterm infants may result in forced exhalation, which reduces the lung volume and places a substantial load on the inspiratory muscles. These muscles must then overcome the increase in workload before they can perform effective inspiration using the diaphragm. Additionally, in preterm infants, the diaphragm contains < 10% type I muscle fibers and a low amount of type IIb muscle fibers (33, 34). Together, the lack of fatigue-resistant type I muscle fibers, high proportion of fatigue-susceptible type IIc muscle fibers, and low oxidative capacity of the neonatal diaphragm indicate that the diaphragm muscle may be prone to fatigue (19, 20, 31).
Thus, enhanced diaphragmatic breathing may represent substantial energy expenditure that contributes to ventilatory failure and diaphragmatic fatigue. As a consequence, an imbalance between an imposed load and inspiratory muscle capacity in preterm infants leads to desaturation symptoms. Increased RMS indicates that work-of-breath and thoracoabdominal asynchrony are elevated in preterm infants. It has previously been shown (29) that microcurrent therapy lowers the RMS of hand grip muscle and increases of hand grip strength in the elderly. Thus, the authors suggest that an elevated concentration of intracellular calcium might alter membrane integrity, causing functionally prolonged muscle contraction.
Second, microcurrent therapy may enhance adenosine triphosphate (ATP) synthesis of amino acid transportation, and protein synthesis, which can prevent the vicious cycle of diaphragm and rectus abdominis muscle contraction (35, 36). Prolonged actin-myosin coupling can promote muscle fiber contraction and increase the flow resistance in the microvasculature of the contracted muscle. Arteriolar and capillary constriction impairs blood flow to the tense muscle fibers and can be aggravated by local vasoconstrictor reflex. This can lead to reduced levels of oxygen and glucose, decreasing the regeneration of ATP (37–41). Decreased ATP can interfere with Ca2+ reuptake into the sarcoplasmic reticulum, a process that is partially ATP-dependent, which prolongs actin-myosin cross-bridging and initiates a vicious cycle (42). Previous studies have described the benefits of electric current on soft tissue repair as a result of supplying ATP (43).
The findings of the present study demonstrate that infants with a lower RMS of rectus abdominis take longer time to treat desaturation and developmental delay at 3 months after treatment. These findings completely correlate with the findings of previous studies and we speculate that this consistency may be attributed to the muscle hypotonia. Previous studies (44–47) have demonstrated that hypotonia has a negative impact in infants exhibiting genetic syndromes, reporting difficulties in latching and sucking during infancy and challenges in managing solid foods during childhood (45, 46). Hypotonia can interfere with the development of the motor skills required for the success of oral feeding (47), representing a challenge to the transition through the various developmental stages of feeding.
The infants with a low RMS of the rectus abdominis showed delayed development. Two mechanisms are possible for this observation. First, the infants with the low RMS of the rectus abdominis recovered slowly, therefore, they were exposed to a hypoxic environment for a longer period of time. In addition, chronic hypoxia interferes with brain development and causes developmental delay. Second, rectus abdominis is a trunk muscle essential for gross movement. Low activity of the rectus abdominis can represent an independent factor to affect development.
There are some limitations associated with this study. First, the sample size is too small. Second, our study lacks a control group for the direct comparison of microcurrent therapy to other standard dysphagia rehabilitation therapy since all parents of preterm infants want to receive microcurrent therapy. Third, we did not perform other test to evaluate the cause of desaturation (e.g., chest CT or esophageal pH monitoring). Therefore, we cannot exclude other factors influence desaturation. Therefore, future randomized controlled studies are warranted. Fourth, the duration of follow up is too short to detect developmental delay. Finally, to achieve optimal results, the effects of microcurrents of various frequencies and durations (e.g., <30 min or >3 h.) must be assessed.
Conclusion
This pilot study shows that microcurrent therapy of the rectus abdominis is an efficient treatment for preterm infants with desaturation during feeding, especially preterm infants with higher rectus abdominis activity. In preterm infants with lower rectus abdominis activity, infants take longer to respond to microcurrent therapy to treat desaturation and they show developmental delay at 3 months after treatment. We can assess the electrical activity of the rectus abdominis muscle in a clinical setting, and it can help determine the cause of desaturation and establish a therapeutic plan, as well as predict the prognosis of development.
Data Availability Statement
The original contributions presented in the study are included in the article/supplementary material, further inquiries can be directed to the corresponding author/s.
Ethics Statement
The studies involving human participants were reviewed and approved by the Institutional Review Board of Daegu Catholic University Medical Center (DCUMC) approved this study. Written informed consent to participate in this study was provided by the participants' legal guardian/next of kin.
Author Contributions
DRK contributed to the study conception and design. DRK, DGK, and JEJ contributed to the interpretation of the study results, writing, and editing of this manuscript. All authors contributed to the article and approved the submitted version.
Funding
This research was supported by the Basic Science Research Program through the National Research Foundation of Korea (NRF), which is funded by the Ministry of Education (NRF-2016R1D1A1B01014260).
Conflict of Interest
The authors declare that the research was conducted in the absence of any commercial or financial relationships that could be construed as a potential conflict of interest.
Publisher's Note
All claims expressed in this article are solely those of the authors and do not necessarily represent those of their affiliated organizations, or those of the publisher, the editors and the reviewers. Any product that may be evaluated in this article, or claim that may be made by its manufacturer, is not guaranteed or endorsed by the publisher.
References
2. Lau C, Smith EO, Schanler RJ. Coordination of suck-swallow and swallow respiration in preterm infants. Acta Paediatr. (2003) 92:721–7. doi: 10.1111/j.1651-2227.2003.tb00607.x
3. Shivpuri CR, Martin RJ, Carlo WA, Fanaroff AA. Decreased ventilation in preterm infants during oral feeding. J Pediatr. (1983) 103:285–9. doi: 10.1016/S0022-3476(83)80368-0
4. Heldt GP. Development of stability of the respiratory system in preterm infants. J Appl Physiol. (1985) 65:441–4. doi: 10.1152/jappl.1988.65.1.441
5. Mathew OP. Respiratory control during nipple feeding in preterm infants. Pediatr Pulmonol. (1988) 5:220–4. doi: 10.1002/ppul.1950050408
6. Rosen CL, Glaze DG, Frost JD. Hypoxemia associated with feeding in the preterm infant and full-term neonate. Am J Dis Child. (1984) 138:623–8. doi: 10.1001/archpedi.1984.02140450005002
7. Nugent ST, Finley JP. Spectral analysis of the EMG and diaphragmatic muscle fatigue during periodic breathing in infants. J Appl Physiol. (1985) 58:830–3. doi: 10.1152/jappl.1985.58.3.830
8. Choi HW, Park HW, Kim HY, Lim G, Koo SE, Lee BS, et al. Feeding desaturation and effects of orocutaneous stimulation in extremely low birth weight infants. J Korean Soc Neonatol. (2010) 17:193–200. doi: 10.5385/jksn.2010.17.2.193
9. Poets CF, Langner MU, Bohnhorst B. Effects of bottle feeding and two different methods of gavage feeding on oxygenation and breathing patterns in preterm infants. Acta Paediatr. (1997) 86:419–23. doi: 10.1111/j.1651-2227.1997.tb09034.x
10. Thoyre SM, Carlson J. Occurrence of oxygen desaturation events during preterm infant bottle feeding near discharge. Early Hum Dev. (2003) 72:25–36. doi: 10.1016/S0378-3782(03)00008-2
11. Blondheim O, Abbasi S, Fox WW, Bhutani VK. Effect of enteral gavage feeding rate on pulmonary functions of very low birth weight infants. J Pediatr. (1993) 122:751–5. doi: 10.1016/S0022-3476(06)80021-1
12. Shiao SY. Comparison of continuous versus intermittent sucking in very-low-birth-weight infants. J Obstet Gynecol Neonatal Nurs. (1997) 26:313–9. doi: 10.1111/j.1552-6909.1997.tb02147.x
13. Shiao SY, Youngblut JM, Anderson GC, DiFiore JM, Martin RJ. Nasogastric tube placement: effects on breathing and sucking in very-low-birth-weight infants. Nurs Res. (1995) 44:82–8. doi: 10.1097/00006199-199503000-00004
14. Chen CH, Wang TM, Chang HM, Chi CS. The effect of breast- and bottle-feeding on oxygen saturation and body temperature in preterm infants. J Hum Lact. (2000) 16:21–7. doi: 10.1177/089033440001600105
15. Farahani R, Kanaan A, Gavrialov O, Brunnert S, Douglas RM, Morcillo P, et al. Differential effects of chronic intermittent and chronic constant hypoxia on postnatal growth and development. Pediatr Pulmonol. (2008) 43:20–8. doi: 10.1002/ppul.20729
16. Garg M, Kurzner SI, Bautista DB, Keens TG. Clinically unsuspected hypoxia during sleep and feeding in infants with bronchopulmonary dysplasia. Pediatrics. (1988) 81:635–42.
17. Bass JL, Corwin M, Gozal D, Moore C, Nishida H, Parker S, et al. The effect of chronic or intermittent hypoxia on cognition in childhood: a review of the evidence. Pediatrics. (2004) 114:805–16. doi: 10.1542/peds.2004-0227
18. Samuels MP, Poets CF, Southall DP. Abnormal hypoxemia after life-threatening events in infants born before term. J Pediatr. (1994) 125:441–6. doi: 10.1016/S0022-3476(05)83292-5
19. Le Souëf PN, England SJ, Stogryn HA, Bryan AC. Comparison of diaphragmatic fatigue in newborn and older rabbits. J Appl Physiol. (1985) 65:1040–4. doi: 10.1152/jappl.1988.65.3.1040
20. Lopes JM, Muller NL, Bryan MH, Bryan AC. Synergistic behavior of inspiratory muscles after diaphragmatic fatigue in the newborn. J Appl Physiol Respir Environ Exerc Physiol. (1981) 51:547–51. doi: 10.1152/jappl.1981.51.3.547
21. Muller N, Gulston G, Cade D, Whitton J, Froese AB, Bryan MH, et al. Diaphragmatic muscle fatigue in the newborn. J Appl Physiol Respir Environ Exerc Physiol. (1979) 46:688–95. doi: 10.1152/jappl.1979.46.4.688
22. Gewolb IH, Vice FL. Abnormalities in the coordination of respiration and swallow in preterm infants with bronchopulmonary dysplasia. Dev Med Child Neurol. (2006) 48:595–9. doi: 10.1111/j.1469-8749.2006.tb01321.x
23. Kwon DR, Park GY, Jeong JE, Kim WT, Lee EJ. Augmentation of respiratory muscle activities in preterm infants with feeding desaturation. Korean J Pediatr. (2018) 61:78–83. doi: 10.3345/kjp.2018.61.3.78
24. Kim MY, Kwon DR, Lee HI. Therapeutic effect of microcurrent therapy in infants with congenital muscular torticollis. PM R. (2009) 1:736–9. doi: 10.1016/j.pmrj.2009.06.008
25. Kwon DR, Park GY. Efficacy of microcurrent therapy in infants with congenital muscular torticollis involving the entire sternocleidomastoid muscle: a randomized placebo-controlled trial. Clin Rehabil. (2014) 28:983–91. doi: 10.1177/0269215513511341
26. Mäenpää H, Jaakkola R, Sandström M, Von Wendt L. Does microcurrent stimulation increase the range of movement of ankle dorsiflexion in children with cerebral palsy? Disabil Rehabil. (2004) 26:669–77. doi: 10.1080/09638280410001684046
27. Byl NN, McKenzie AL, West JM, Whitney JD, Hunt TK, Hopf HW, et al. Pulsed microamperage stimulation: a controlled study of healing of surgically induced wounds in Yucatan pigs. Phys Ther. (1994) 74:201–13. doi: 10.1093/ptj/74.3.201
28. Wessberg GA, Carroll WL, Dinham R, Wolford LM. Evaluation of microcurrent electrical nerve stimulation (MENS) effectiveness on muscle pain in temporomandibular disorders patients. J Appl Oral Sci. (2006) 14:61–6. doi: 10.1590/S1678-77572006000100012
29. Kwon DR, Kim J, Kim Y, An S, Kwak J, Lee S, et al. Short-term microcurrent electrical neuromuscular stimulation to improve muscle function in the elderly: A randomized, double-blinded, sham-controlled clinical trial. Medicine. (2017) 96:e7407. doi: 10.1097/MD.0000000000007407
30. Frankenburg WK, Dodds JB. The denver developmental screening test. J Pediatr. (1967) 71:181–91. doi: 10.1016/S0022-3476(67)80070-2
31. Frankenburg WK, Dodds J, Archer P, Shapiro H, Bresnick B. The Denver II: a major revision and restandardization of the Denver Developmental Screening Test. Pediatrics. (1992) 89:91–7.
32. Lambert MI, Marcus P, Burgess T, Noakes TD. Electromembrane microcurrent therapy reduces signs and symptoms of muscle damage. Med Sci Sports Exerc. (2002) 34:602–7. doi: 10.1097/00005768-200204000-00007
33. Keens TG, Bryan AC, Levison H, Ianuzzo CD. Developmental pattern of muscle fiber types in human ventilatory muscles. J Appl Physiol Respir Environ Exerc Physiol. (1978) 44:909–13. doi: 10.1152/jappl.1978.44.6.909
34. Sieck GC, Fournier M, Blanco CE. Diaphragm muscle fatigue resistance during postnatal development. J Appl Physiol. (1985) 71:458–64. doi: 10.1152/jappl.1991.71.2.458
35. Lennox AJ, Shafer JP, Hatcher M, Beil J, Funder SJ. Pilot study of impedance-controlled microcurrent therapy for managing radiation-induced fibrosis in head-and-neck cancer patients. Int J Radiat Oncol Biol Phys. (2002) 54:23–34. doi: 10.1016/S0360-3016(02)02898-5
36. Cheng N, Van Hoof H, Bockx E, Hoogmartens MJ, Mulier JC, De Dijcker FJ, et al. The effects of electric currents on ATP generation, protein synthesis, and membrane transport of rat skin. Clin Orthop Relat Res. (1982) 171:264–72. doi: 10.1097/00003086-198211000-00045
37. Baker JS, McCormick MC, Robergs RA. Interaction among skeletal muscle metabolic energy systems during intense exercise. J Nutr Metab. (2010) 2010:905612. doi: 10.1155/2010/905612
38. Bønnelykke Sørensen V, Wroblewski H, Galatius S, Haunsø S, Kastrup J. Assessment of continuous skeletal muscle blood flow during exercise in humans. Microvasc Res. (2000) 9:301–9. doi: 10.1006/mvre.1999.2219
39. Degens H, Salmons S, Jarvis JC. Intramuscular pressure, force and blood flow in rabbit tibialis ante-rior muscles during single and repetitive contrac-tions. Eur J Appl Physiol. (1998) 78:13–9. doi: 10.1007/s004210050381
40. Hussain SN, Magder S. Diaphragmatic intramuscular pressure in relation to tension, shortening, and blood flow. J Appl Physiol. (1985) 71:159–67. doi: 10.1152/jappl.1991.71.1.159
41. Wisnes A, Kirkebo A. Regional distribution of blood flow in calf muscles of rat during passive stretch and sustained contraction. Acta Physiol Scand. (1976) 96:256–66. doi: 10.1111/j.1748-1716.1976.tb10194.x
42. Simons DG, Travell J. Myofascial trigger points, a possible explanation. Pain. (1981) 10:106–9. doi: 10.1300/J094v10n03_08
43. Fleischli JG, Laughlin TJ. Electrical stimulation in wound healing. J Foot Ankle Surg. (1997) 36:457–61. doi: 10.1016/S1067-2516(97)80099-3
44. Crapnell TL, Rogers CE, Neil JJ, Inder TE, Woodward LJ, Pineda RG. Factors associated with feeding difficulties in the very preterm infant. Acta Paediatr. (2013) 102:e539–45. doi: 10.1111/apa.12393
45. Mathisen B, Reilly S, Skuse D. Oral-motor dysfunction and feeding disorders of infants with turner syndrome. Dev Med Child Neurol. (1992) 34:141–9. doi: 10.1111/j.1469-8749.1992.tb14980.x
46. Cassidy SB, Schwartz S, Miller JL, Driscoll DJ. Prader-willi syndrome. Genet Med. (2012) 14:10–26. doi: 10.1038/gim.0b013e31822bead0
Keywords: desaturation, feeding, preterm, infant, rectus abdominis, microcurrent
Citation: Kwon DR, Kwon DG and Jeong JE (2021) Effects of Microcurrent on Oxygen Saturation by Controlling Rectus Abdominis Activity in Preterm Infant With Desaturation During Feeding: A Pilot Study. Front. Pediatr. 9:694432. doi: 10.3389/fped.2021.694432
Received: 13 April 2021; Accepted: 03 November 2021;
Published: 22 November 2021.
Edited by:
Fernando Cabañas, Quironsalud Madrid University Hospital, SpainReviewed by:
Sam Ebenezer Athikarisamy, University of Western Australia, AustraliaWen-Shiang Chen, National Taiwan University, Taiwan
Copyright © 2021 Kwon, Kwon and Jeong. This is an open-access article distributed under the terms of the Creative Commons Attribution License (CC BY). The use, distribution or reproduction in other forums is permitted, provided the original author(s) and the copyright owner(s) are credited and that the original publication in this journal is cited, in accordance with accepted academic practice. No use, distribution or reproduction is permitted which does not comply with these terms.
*Correspondence: Dong Rak Kwon, ZG9uZ3Jha2t3b24mI3gwMDA0MDtob3RtYWlsLmNvbQ==; Y29vbGt3b24mI3gwMDA0MDtjdS5hYy5rcg==