- 1Discipline of Pediatrics, School of Medicine, Trinity College Dublin, The University of Dublin, Dublin, Ireland
- 2Trinity Research in Childhood Centre, Trinity College Dublin, Dublin, Ireland
- 3Children's Health Ireland (CHI) at Tallaght, Dublin, Ireland
- 4St. Michael's House, Dublin, Ireland
- 5Department of Clinical Genetics, Birmingham Women's Hospital, Birmingham, United Kingdom
- 6Royal College of Surgeons in Ireland, Dublin, Ireland
- 7Children's Health Ireland at Crumlin, Dublin, Ireland
- 8Children's Health Ireland at Temple St. Dublin, Dublin, Ireland
- 9Central Remedial Clinic, Dublin, Ireland
- 10Department of Paediatrics, Cork University Hospital, Cork, Ireland
- 11Department of Neonatology, The Coombe Women and Infants University Hospital, Dublin, Ireland
Cerebral Palsy (CP) describes a heterogenous group of non-progressive disorders of posture or movement, causing activity limitation, due to a lesion in the developing brain. CP is an umbrella term for a heterogenous condition and is, therefore, descriptive rather than a diagnosis. Each case requires detailed consideration of etiology. Our understanding of the underlying cause of CP has developed significantly, with areas such as inflammation, epigenetics and genetic susceptibility to subsequent insults providing new insights. Alongside this, there has been increasing recognition of the multi-organ dysfunction (MOD) associated with CP, in particular in children with higher levels of motor impairment. Therefore, CP should not be seen as an unchanging disorder caused by a solitary insult but rather, as a condition which evolves over time. Assessment of multi-organ function may help to prevent complications in later childhood or adulthood. It may also contribute to an improved understanding of the etiology and thus may have an implication in prevention, interventional methods and therapies. MOD in CP has not yet been quantified and a scoring system may prove useful in allowing advanced clinical planning and follow-up of children with CP. Additionally, several biomarkers hold promise in assisting with long-term monitoring. Clinicians should be aware of the multi-system complications that are associated with CP and which may present significant diagnostic challenges given that many children with CP communicate non-verbally. A step-wise, logical, multi-system approach is required to ensure that the best care is provided to these children. This review summarizes multi-organ dysfunction in children with CP whilst highlighting emerging research and gaps in our knowledge. We identify some potential organ-specific biomarkers which may prove useful in developing guidelines for follow-up and management of these children throughout their lifespan.
Introduction
Cerebral palsy (CP) describes a heterogenous group of non-progressive disorders of posture or movement, causing activity limitation, due to a lesion in the developing brain (1). The worldwide prevalence of CP is ~2 per 1,000 live births (2), although the most recent data from the Surveillance of Cerebral Palsy in Europe Network reports the prevalence as 1.64 per 1,000 live births (3).
There are many recognized risk factors for cerebral palsy, including preterm birth, multiple gestation, congenital malformation, genetic and metabolic abnormalities, intra-uterine exposure to infection or inflammation, birth asphyxia, perinatal stroke and thrombophilia (4). CP has an overall rate of 13% in survivors of term neonatal encephalopathy (NE), and 24% of term children with CP have a history of moderate to severe NE (5). Neonatal asphyxia induces global hypoxic-ischaemia resulting in multi organ injury (6). Early multi-organ dysfunction (MOD) in neonates with encephalopathy may persist in later childhood. Cardiac, renal, hepatic, hematological and neurological dysfunction are well-described in neonates with NE, but follow-up in childhood is not routine practice.
Life-expectancy is reduced in children with CP, based on retrospective data collected between 1983 and 2010 in California (7, 8). Respiratory and cardiac dysfunction are well-documented causes of increased morbidity and mortality in children with CP and dysfunction in other organs such as the renal, gastrointestinal and hematological systems are gaining recognition. Assessment of multi-organ function may be useful to ensure complete resolution of any abnormalities and avoid complications in later childhood or adulthood. It may also contribute to an improved understanding of the etiology and thus may have an implication in prevention, interventional methods and therapies.
While CP is heterogenous condition with a wide range of etiologies and subtypes it is acknowledged that functional level is a strong predictor of morbidity and mortality. Greater degrees of motor impairment are associated with a larger proportion of accompanying impairments (9, 10). Mortality also increases with increasing severity of impairment and those with the highest disability scores have a 50% mortality by 15 years of age (11). Throughout this article, although some issues are present across all functional levels, the co-morbidities described are most-likely to be relevant to those with a Gross Motor Function Classification system (GMFCS) of IV-V, unless otherwise stated. Our focus is on children with CP, but as more children survive into adulthood we believe it is important to consider that the care provided in childhood may have a significant impact on future health. Also, where little research in children with CP exists, or, where the phenotype changes between childhood and adulthood, we have evaluated and included adult studies because we believe this can provide valuable insights.
We aimed to summarize multi-organ dysfunction in children with CP whilst highlighting emerging research and gaps in our knowledge. We identify some potential organ-specific biomarkers which may prove useful for research purposes or for developing guidelines for follow-up and management of these children throughout their lifespan (Table 1).
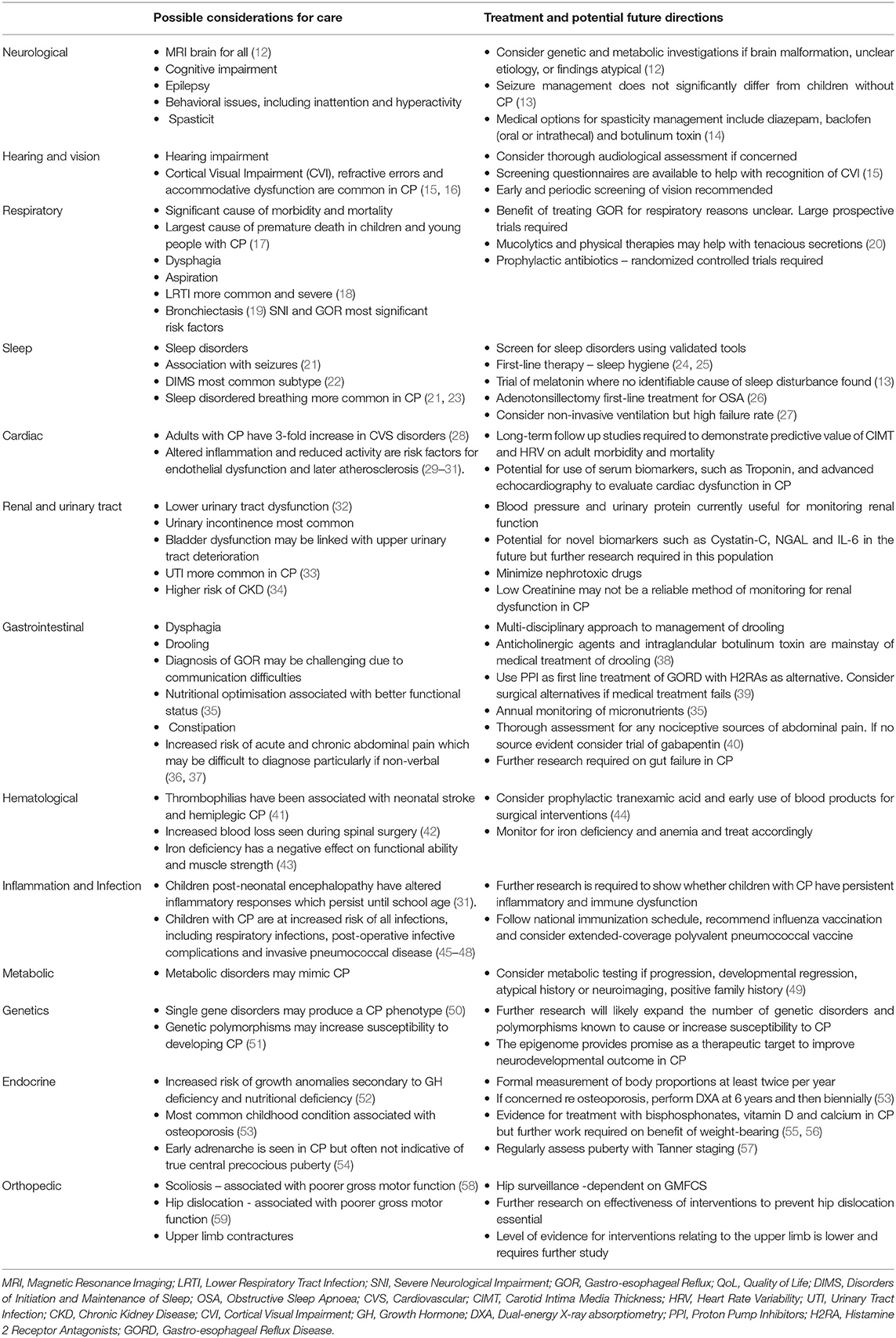
Table 1. Summary of multi-organ involvement, management and potential future directions for research in Cerebral Palsy (CP).
The Nervous System
Cerebral palsy, by definition, is attributable to a lesion in the developing brain (1). Periventricular white matter lesions are observed on magnetic resonance images (MRI) in 50% of children with CP, and cortical or subcortical gray matter lesions in 20% (60). However, in a study of children with CP born after 36 weeks' gestation, neuroimaging studies were normal in one third of children (61). In the same study, the most common abnormality on neuroimaging was focal infarction, observed in 22% of the children. Other abnormalities noted were brain malformation, including schizencephaly, hydrocephalus, polymicrogyria, lissencephaly, agenesis of the corpus callosum, septo-optic dysplasia, and cerebellar anomalies. Periventricular leukomalacia (PVL) which is associated with prematurity was seen in 12% of the children, hypoxic ischaemic brain injury in 5% and intracranial hemorrhage in a further 5% (61). Gray and white matter lesion burden has been shown to correlate with motor and cognitive function (60). The American Academy of Neurology recommend neuroimaging in all children with CP. In those where history and imaging do not determine etiology or where findings are atypical, genetic or metabolic investigations should be considered. Where a brain malformation is found, it is recommended that genetic and metabolic conditions also be considered (12).
Cerebral Palsy is the most common cause of motor disability in children (4). Studies of the epidemiology of CP traditionally group children with CP into phenotypic categories based on the type of tone abnormality and the distribution of limb weakness (61). The severity of motor impairment is assessed by the Gross Motor Function Classification System (GMFCS) and this is the most widely used standard measure of motor function (62). Aside from motor impairment, neuropsychological & cognitive function is commonly impaired in children with CP. Intellectual disability is seen in approximately one third of patients with CP, GMFCS I-II and two thirds of patients with CP, GMFCS III-V (13) and has previously been shown to be the strongest predictor of mortality in CP (5). Children with CP who have a history of neonatal encephalopthy (NE) are significantly more likely to develop cognitive impairment and have a greater burden of disability, including epilepsy, than children with CP who did not have NE (5).
The overall prevalence of epilepsy in children with cerebral palsy was found to be 38%, although this varies significantly depending on CP subtypes, etiology and cognitive function (63). Prevalence increases with increasing severity of motor impairment. There is a higher prevalence of epilepsy in children with CP secondary to CNS malformations, CNS infections, and gray matter lesions than those with CP secondary to white matter changes or CP of unknown etiology. Furthermore, the prevalence of epilepsy in CP increased with decreased cognitive function (63). Age of onset of epilepsy can vary with type of CP, and early recognition and diagnosis is essential for prompt management. Management of seizure disorders are not significantly different in children with CP compared to those without, but care should be taken not to confuse seizures with dyskinesia (13). Behavioral issues are also more common in children with CP overall and especially with coexisting epilepsy (64). Hyperactivity and inattention are significantly higher in children with CP and epilepsy than in children with CP alone (64).
Hypertonia is a significant issue for many children with CP, leading to impaired motor function, pain and difficulties with daily care. Hypertonia may manifest primarily as spasticity, dystonia or less commonly rigidity, alone or in combination (65). Spasticity management involves a multi-disciplinary approach, including individual, goal-oriented physiotherapy and occupational therapy (14). Options for medical treatment include diazepam, baclofen (oral or intrathecal) and botulinum toxin type A (14). In certain cases, Selective Dorsal Rhizotomy may be considered (14). Dystonia management requires specific medications, including trihexyphenidyl and gabapentin, but current evidence is limited and the majority of care pathways rely on expert opinion (66, 67). Choice of treatment should be tailored to the individual and based on their treatment goals.
Hearing and Vision
The incidence of hearing impairment in children with CP is reported to be between 7 to 37.5% (68) with the following distribution: 48% conductive, 4% sensorineural, 25% mixed and 23% unspecified (68). Conductive hearing loss has been associated with a high rate of chronic otitis media, eustachian tube dysfunction, abnormal anatomy and craniofacial anomalies in this cohort. Sensorineural hearing loss (SNHL) has been postulated to be associated with low birth weight, hyperbilirubinemia and neonatal hypoxemia in this population, with more severe hearing loss in those with quadriplegia, epilepsy or intellectual disability (68). Furthermore, congenital CMV infection is associated with cerebral palsy and is a leading cause of SNHL in children (69). Although there are inherent difficulties in testing this patient group for hearing loss using pure tone audiometry, it should not preclude thorough audiological assessment.
Children with cerebral palsy can be diagnosed with visual impairments that are ocular or cerebral in origin or combination of both (70). Cortical visual impairment (CVI), refractive errors and accommodative dysfunction are common in children with cerebral palsy (15). Periventricular Leucomalacia (PVL) on MRI is found to have a strong association with CVI (71). Dutton et al. provide a questionnaire inventory as an effective means to assist the clinician in recognition of CVI so that the appropriate strategies and management can be put in place to assist the child (15). Lesions involving the basal ganglia are more frequently associated with impaired visual function than those involving the visual occipital cortex (72). Furthermore, there is a correlation between the severity of basal ganglia lesions and the degree of visual impairment, with moderate and severe lesions of the basal ganglia always associated with visual impairment in one study (73). White matter lesions are not as reliable an indicator of neonates who will develop visual impairment, as only those with severe white matter changes had visual impairment and the presence or severity of visual impairment was not always associated with lesions involving the visual cortex. There was over 90% concordance between visual assessments performed in the 1st year of life and at school-going age (73), supporting the need for early and periodic screening and long-term monitoring.
Respiratory Dysfunction
Disorders of the respiratory system are a significant cause of morbidity (74) and are the largest cause of premature death in children and young people with CP (17, 75, 76). Respiratory symptoms are common, particularly in those with a GMFCS level of V and are most prevalent at meal-times (77). Respiratory diagnoses account for a high proportion of hospital admissions in this population (78, 79) and many of these admissions may be predictable and modifiable (79). Blackmore et al. demonstrated that 12.1% of children and young people with CP were admitted to hospital due to respiratory illness over the course of 1 year (80). In the same period, 19.2% had been prescribed 2 or more courses of antibiotics for respiratory issues (80).
Reasons for the high rate of respiratory morbidity are complex, multifactorial and several factors, such as seizures, oromotor dysfunction, gastro-esophageal reflux and scoliosis, may coexist within the same individual (80, 81). Impaired airway clearance, due to respiratory muscle weakness, impaired coordination and poor positioning, in the context of poorer motor function, may predispose to infection and atelectasis. Bronchopulmonary dysplasia may be an issue in children with CP who were born prematurely (74, 82).
Swallowing is inextricably linked with respiratory dysfunction. It is a complex process involving the brainstem, multiple overlapping cortical areas, sensory input from the oropharynx and a high level of coordination between the central and enteric nervous systems (83). Chewing and swallowing problems are significantly associated with poorer gross motor function and intellectual ability (84) and impact on respiratory health. Dysphagia is common, occurring in 43% of all children with CP (83) and in children with severe generalized CP and intellectual disability can be as high as 99% (85). This can result in video fluoroscopically-proven aspiration in up to 70% of children with severe CP (86). Aspiration is a significant cause of respiratory symptoms and pneumonia in CP and can result from both direct aspiration from the oropharynx or indirect aspiration of gastric contents (87). Aspiration may result in pneumonia through introduction of pathogenic bacteria, alteration of the respiratory microbiome, promotion of airway inflammation or a complex interaction of all of these factors (88–92).
Lower respiratory tract infections (LRTI) in general are more common in children with CP and they have more severe disease requiring admission to the intensive care unit (18). Thorburn et al. reported that 89% of children with moderate to severe CP (n = 53, 90% with GMFCS ≥3) ventilated in the pediatric intensive care unit for 4 or more days carried abnormal bacteria flora, with Pseudomonas and Klebsiella being the most frequent. In children without CP (n = 257) the carriage rates of abnormal bacteria were 55% with 10% resistant bacteria. Almost half of these were resistant bacteria and the mortality rate was significantly higher amongst the group with CP compared those without CP (17 vs. 10%) (93). Bronchiectasis, which predisposes to recurrent LRTI, is reported in 67% of patients with CP presenting to a dysphagia clinic (94). Similarly, a retrospective study of 100 children with chronic pulmonary aspiration found that 66% had bronchiectasis, with the significant risk factors found to be severe neurological impairment (NI) (OR 9.45, 95% CI 2.05–43.6) and parent report of previous gastroesophageal reflux (OR3.36 95% CI 1.08–10.43). In the cohort of children with severe NI (n = 30), 93% had bronchiectasis (19).
Management of respiratory morbidity often requires a multifaceted approach. Those who care for children with CP must rule out aspiration when they present with respiratory symptoms. In particular, one must be cognisant of the fact that silent aspiration is more common in children with NI (95). In the respiratory context, some authors argue that gastroesophageal reflux (GOR) should be treated (94), presumably in an effort to reduce indirect aspiration of gastric contents. However, the evidence here is not conclusive. A randomized control trial of 38 children with asthma and GOR found no difference in respiratory symptoms or lung function between those treated with a proton-pump inhibitor (PPI) and placebo (96). Conversely, a prospective study comparing PPI vs. histamine H2-receptor antagonists (H2RA) identified 35 out of 74 children with esophageal and extra-esophageal (respiratory and laryngeal) manifestations of GOR (97). Eighty-three percent had complete resolution of symptoms when treated with a PPI, compared with 35% in the H2RA group. Conflicting evidence is also seen with regards to the surgical treatment of GOR for respiratory symptoms. A prospective cohort study, comparing outcomes of Nissen fundoplication in children with NI (n = 46) vs. without NI (n = 42) showed a reduction in the proportion of children with NI who had more than 4 respiratory infections per year from 33% pre-operatively to 8, 18, and 10% at 1, 2, and 3 year postoperative, respectively (98). However, respiratory symptoms were not reported to be improved post-operatively. Another retrospective review of 34 patients following fundoplication, 15 of whom had NI, evaluated outcomes at 7 years post-operative. Pulmonary symptoms were frequent in children with NI pre-operatively (13/15) and did not significantly improve after surgery, while half of the children with NI developed new symptoms or complications during the period of follow-up (99). A retrospective analysis of discharge data for 342 pediatric patients, found no difference in hospital admissions for aspiration pneumonia, other pneumonia or respiratory distress before or after anti-reflux surgery (100). Finally, a meta-analysis by Lauriti et al. showed that gastro-jejunal feeding was no better at preventing respiratory complications than anti-reflux surgery in children with NI (101). Large prospective, high quality trials would be useful to determine if and when treatment of GOR is useful in improving respiratory morbidity.
The British Thoracic Society (BTS) recommends the use of mucolytics, such as nebulised saline, to assist clearance of “tenacious secretions” in children with neuromuscular weakness (20). Physical therapies to help removal of respiratory secretions are likely to be helpful but may be a significant extra burden on families (94). Finally, there is no evidence for the use of prophylactic antibiotics in children with CP. Long-term azithromycin is recognized as beneficial in cystic fibrosis (102) likely secondary to its anti-inflammatory properties. The BTS recommend consideration of prophylactic antibiotics in non-cystic fibrosis bronchiectasis although the authors acknowledge that controlled trials are lacking (103). Prophylactic antibiotics are frequently prescribed for children with CP. Macrolide antibiotics have antibacterial, anti-inflammatory, pharmacokinetic and bioavailability characteristics that make them useful for prophylaxis, as well as being safe with few side effects (74, 94, 104). High quality randomized controlled trials are required to assess their effectiveness and indications for use in children with CP.
Sleep Problems
The prevalence of sleep disorders among children with CP is up to 36%, although this varies within subgroups, according to CP phenotype (bilateral spastic, 41%; unilateral spastic, 24%; dyskinesia, 30.8%; ataxia/non-classified, 17.4%) (105, 106) and can have a significant impact on quality of life (107). Total sleep disorders are principally associated with seizures (21). Subtypes of sleep disorders in children with CP include disorders of initiating and maintaining sleep (DIMS), sleep breathing disorders, sleep hyperhidrosis, sleep-wake transition disorders, disorders of arousal, parasomnias and non-restorative sleep (105). DIMS are the most frequently occurring subtype of sleep disorder with symptoms including difficulty falling asleep and frequent night awakening (21, 22). This may be secondary to pain, involuntary movements, associated behavioral and adaptive difficulties, underlying brainstem dysfunction or severe visual impairment (21, 108–112).
Behavioral considerations are a significant cause of sleep disturbance in CP. In a study of children with moderate to severe motor disability (n = 505, 216 had CP) which used parental questionnaires to evaluate sleep problems, 25% of children with CP had difficulties relaxing at night (113). A systematic review and meta-analysis by Horwoord et al. (105) calculated the rate of problems with “sleeping routines” as 51.3%, using the data from a retrospective observational study of 154 children with CP. Interestingly, this concern decreased with worsening motor function from 100% in children classified as GMFCS I to 34% in children classified as GMFCS V and with increasing age.
The Sleep Disturbance Scale for Children (SDSC) (114, 115), Pediatric Sleep Questionnaire (PSQ) (116) and Childhood Sleep Habit Questionnaire (CSHQ) (117, 118) are validated tools used to document sleep problems in children and are frequently used in children with CP. Sleep affects the quality of life of these children (107, 119) and their families (120, 121) thus, sleep disorders are ideally evaluated in children with CP using these questionnaires and subsequently monitored and managed appropriately. Sleep hygiene is first-line therapy for sleep disturbance in all children including those with neurodevelopmental disabilities (24, 25, 122). This includes measures such as a consistent bedtime routine, a dark and quiet bedroom, limiting technology use before bed and independence in falling asleep (25, 123). Other therapy options for managing sleep disorders in children with neurodisability include behavioral strategies such as play-based therapy (124) and medications such as melatonin.
Recent research has provided interesting insights into some of the possible pathophysiological mechanisms that underlie sleep disturbance in CP. Circadian Locomotor Output Cycles Kaput (CLOCK) genes are rhythmically expressed in the pineal gland which secretes melatonin and is crucial for regulation of the sleep-wake cycle (125). Expression of CLOCK is altered following hypoxic-ischaemic brain injury in animal models (126) and alterations in circadian rhythm have been reported in children following even mild perinatal hypoxic-ischaemia (127). Day and night variability of melatonin has been found to be lower in children with CP (128). Melatonin is widely prescribed for sleep disturbances and has shown improvements in sleep latency and night-waking in these children (121, 129, 130). The National Institute for Health and Care Excellence (NICE) have produced a guideline on assessment and management of cerebral palsy in under 25-year-olds, within which guidance on managing sleep disturbances is provided (13). Sleep hygiene is recommended in all cases and melatonin where an identifiable cause of sleep disturbance is not found (13).
Sleep-disordered breathing is more common in children with CP than in their typically-developing peers (21, 23, 131), occurring in up to 18.1% of children with CP compared with 7.4% of controls (109). Children with more severe CP as measured by the GMFCS, as well as children with comorbid epilepsy have a higher risk of obstructive sleep apnoea (OSA) (132). OSA may be contributed to by hypotonia, glossoptosis, laryngomalacia, midface and mandibular abnormalities, adenotonsillar hypertrophy, gastro-esophageal reflux, medications and brainstem dysfunction (23, 27). Children with CP may be at increased risk of central sleep apnoea (CSA) as neurological disorders are the commonest risk factor for this (133). Sleep-disordered breathing in CP may also be caused by muscular weakness. Children with CP have reduced diaphragmatic mobility, respiratory muscle strength and chest expansion (134). Sleep disorders in general have a significant impact on quality of life in CP (107, 135) and therefore, treatment should be strongly considered for sleep disordered breathing. Non-invasive ventilation is a potential treatment. However, this can prove more challenging in children with CP and in a retrospective review, 55% of patients with CP failed to establish on this treatment compared with an overall failure rate of 8.7% in the same tertiary service (27). The American Academy of Pediatrics has recognized adenotonsillectomy as first line treatment for sleep apnoea in children (136). A randomized trial (n = 464) showed benefits in reducing symptoms of OSA as well as improvements in behavioral, quality of life and polysomnographic measures (26). Surgery may be rendered more difficult in children with neurological impairment due to co-morbidities. A retrospective review of 375 children with OSA and syndromic or neurological comorbidities, 105 of whom had cerebral palsy, found that the average apnoea-hypopnoea index reduced from 12.4 to 5.7 (p = 0.002) following tonsillectomy, leading the authors to conclude that adenotonsillectomy remained the mainstay of treatment for OSA in these children.
Sleep disorders can be difficult to diagnose in this population but can have a significant effect on the lives of children with CP and their families. It is important to be mindful of sleep issues and consideration should be given to regular screening for these disorders in children with CP.
The Cardiovascular System
A cohort study which included 958 adults with CP reported that they had a 3-fold increase in disorders of the circulatory system (28). In a linkage study involving the Victorian Cerebral Palsy Register and the Australian National Death Index, out of 3,507 individuals with CP, 418 were known to have died between the years 1970 and 2010, with cardiac causes of death second only to respiratory (76).
Chronic inflammation is known to contribute to the formation of atherosclerosis, a common cardiovascular disorder (29) and children with CP are known to have altered inflammatory responses (30, 31) increasing the possibility that these children with CP may develop arterial pathology that leads to atherosclerosis in adult life. Children with CP are less physically active than their typically developing peers (137) and lower levels of physical activity are more likely to be associated with endothelial dysfunction (138–140). Early stage atherosclerosis can be demonstrated by measuring the carotid intima media thickness (CIMT) of the carotid arteries using advanced ultrasound (141). Increased CIMT is predictive of atherosclerosis and future cardiovascular events (142). Carotid ultrasound assessment of children with CP (n = 100), demonstrated increased CIMT when compared to controls (n = 35), suggesting that children with CP are at increased risk of atherosclerosis and coronary artery disease (141). Follow up studies of these children in later childhood and adulthood are required for prompt diagnosis and management.
Heart rate variability (HRV) reflects autonomic control of the sinus node. Significant reductions in HRV have been found between children with CP and controls. Within children with CP, HRV significantly decreases with poorer motor function as measured by GMFCS (143–145). This indicates a less efficient and less adaptive cardiac autonomic system in children with CP (144) and reiterates the need for cardiac surveillance in those with CP, particularly those most severely affected.
Measurement of fetal HRV has also been raised as a possible tool for predicting the development of cerebral palsy. A retrospective review of 95 children with CP, born at term, compared data from fetal monitoring and compared them with controls. Late decelerations and reduced beat-to-beat variability were associated with a sharp increase in risk of developing CP (146). Reduction in HRV can improve the prediction of neurodevelopmental outcome in preterm infants (n = 79) when combined with poor repertoire abnormal general movements (PR GMs) using Prechtl's assessment method (147, 148).
Evidence for myocardial dysfunction in children with CP is lacking. However, the possibility of myocardial involvement is raised by the fact that a proportion of neonates with NE have concurrent cardiac dysfunction (149). Martin-Ancel et al. showed that 29% of neonates with perinatal asphyxia had cardiac dysfunction consistent with myocardial ischaemia (150). The rate of cardiovascular system involvement in infants with post-asphyxial hypoxic ischaemic encephalopathy is reported to be between 50 and 78% (151–153). The variation may be due to the difference in criteria used for involvement of cardiac dysfunction in the above studies. Children with epilepsy who are seizure free have been shown to have impaired systolic and diastolic cardiac function using 2-dimensional speckle tracking echocardiography (154). In this case-control study (n = 120) there were no differences between the subgroups to explain this subclinical dysfunction and suggest that there may be unknown factors involved. Krishnamoorthy et al. prospectively assessed myocardial dysfunction in patients with moderate to severe traumatic brain injury using speckle tracking echocardiography and compared them with age and sex-matched controls (n = 30) (155). They have shown that abnormalities of myocardial strain are seen in this population and that the abnormality persists for at least 1 week. The mechanism underlying this is uncertain but may relate to neuroendocrine dysfunction and dysregulated inflammation (156).
Although it is known that there is acute myocardial dysfunction at the time of the initial insult in some children who develop CP, the long term myocardial function of these patients is not known. Traditional markers of cardiac function such as ejection fraction/shortening fraction are too crude to recognize potential subtle signs of myocardial dysfunction and are unable to detect regional wall dysfunction. With the advancement of echocardiography, newer forms of assessing myocardial function are readily available such as deformation imaging using speckle tracking, tissue doppler, tricuspid annular plane systolic excursion, mitral annular plane systolic excursion and fractional area change. These more sophisticated echocardiography techniques may be able to detect early cardiac dysfunction helping to guide management of these complex patients.
There is a paucity of research in follow-up of cardiovascular dysfunction throughout childhood in cerebral palsy. Echocardiographic assessment of myocardial strain combined with serum biomarkers may allow more accurate evaluation of cardiac dysfunction in later childhood. Troponin T and N-terminal pro b-type natriuretic peptide (NT-pro-BNP) have been shown to be useful markers of cardiac dysfunction in other populations, such as term neonates with perinatal asphyxia, children with chronic kidney disease and children with leukemia (157–159). A prospective study of infants with perinatal asphyxia (n = 54) showed that Troponin T is elevated in those with abnormal MRI findings and that Troponin T correlates with developmental outcomes at 2 years of age (160). Further research is required to establish whether initial abnormal Troponin T and BNP results correlate with subtle myocardial dysfunction parameters in later childhood.
Renal and Urinary Tract Dysfunction
Renal and urinary tract dysfunction is multifactorial in the population of children with CP. Lower urinary tract dysfunction and renal dysfunction may be linked. Lower urinary tract dysfunction is more common in those with CP, affecting, on average 55.5% of this population, urinary incontinence being the most common symptom (32). Lower urinary tract symptoms (LUTS) are known to have a significant impact on quality of life in children and their families (161, 162). Urinary incontinence is more common in children and adults with CP (163–165). Children with CP achieve continence later than their typically developing peers (165, 166) and experience more daytime incontinence, nocturnal enuresis, or a combination of both (165). Abnormal urodynamic studies have been reported in 85% of children with CP referred for urological assessment (n = 27) in the following categories: spastic diplegia (n = 10), spastic quadriplegia (n = 7), spastic hemiplegia (n = 5), dystonic quadriplegia (n = 3), dystonic diplegia (n = 1), spastic monoplegia (n = 1) and intellectual disability (n = 15) (167). The commonest presenting symptom was incontinence (74%) followed by frequency (56%) and urgency (37%) (167). Disorders of urinary storage, most frequently reduced bladder capacity, detrusor overactivity and increased post-void residual volumes, are more common than disorders of voiding (e.g., urinary hesitancy and frequency) (167–169). Greater degrees of motor impairment as evidenced by the GMFCS are associated with a greater frequency of lower urinary tract symptoms and urodynamic abnormalities (170). GMFCS and intellectual ability have both been associated with attainment of continence (165, 171) although agreement on the influence of intellectual ability on continence in CP is not universal (172). Reid et al. advocated for early investigation of urological symptoms with urodynamic studies in children with CP as a way to rationalize treatment, noting that in many cases incontinence can be improved or cured (167).
Bladder dysfunction such as interrupted voiding, hesitancy and urinary retention, but not urinary incontinence, have been linked with upper urinary tract deterioration in children with CP (173). Further urological evaluation may thus be warranted in these children. Notably, obstructive voiding complaints are more common in adults with CP than children and may represent progression over time (174). Half of adults with CP had small, high pressure bladders, putting them at risk for upper urinary tract deterioration (n = 49, mean age = 31 years, 55% male, 98% GMFCS III-V), thus emphasizing the need for ongoing monitoring of people with CP and urinary tract symptoms (174). That said, this paper describes the quarter of patients with CP who underwent urodynamic studies according to the centre's criteria for investigation, which includes recurrent UTI, progressive urinary incontinence, hydronephrosis, urinary retention and progressive LUTS. Therefore, there may be an element of selection bias which skews the results.
Urinary tract infections are more common in children with CP (33, 166) occurring in ~20% (175). Children with CP have an increased incidence of constipation and fecal incontinence (165, 176, 177), both risk factors for the development of UTI (178, 179). Recurrent febrile urinary tract infections have been linked with upper urinary tract deterioration in children with CP and should prompt further urological evaluation. Congenital urinary tract abnormalities have not been found to be more common in children with CP (180).
A significant number of children with CP post NE may develop renal dysfunction later in life. Up to 72% of infants who have a 5-min Apgar score of ≤ 6 show signs of renal compromise (181, 182). Renal involvement in the neonatal period is also associated with neurological severity and outcome (183–186). An episode of acute kidney injury (AKI) in the neonatal period is correlated with an increased risk of developing chronic kidney disease (CKD) in later life (181, 187, 188). Therefore, any children who have had AKI as a result of perinatal asphyxia, regardless of severity, require regular renal function and blood pressure monitoring.
In a large retrospective cohort study, adults with neurodevelopmental disabilities (n = 33,561) had a greater incidence rate of CKD than adults without neurodevelopmental disabilities (n = 6.5 million). This increased risk was maintained even when adjusted for demographics, diabetes, hypertension and cardiovascular disease. Adults with neurodevelopmental disabilities, throughout all subgroups, were also more likely to have advanced CKD. The incidence rate ratio of advanced CKD for those with CP compared to adults without NDDs was 1.83 (95% CI = 1.61–2.07) (34). This highlights that children with CP have a greater risk of developing CKD and renal function monitoring including minimizing nephrotoxic drugs should be considered.
One of the most widely used methods of monitoring renal function is to measure serum creatinine but it does not rise until there is significant renal dysfunction. In addition, children with non-ambulatory CP have been shown to have lower serum creatinine concentrations at baseline (189). This likely represents a reduction in muscle mass and bone mineral density which is evident in children with CP (190–192). This may present some difficulty in monitoring for CKD as these children may have falsely reassuring creatinine levels, compounded by the fact that early CKD is often silent. In the outpatient setting, markers such as blood pressure, along with urinary protein can be useful for monitoring renal function.
Novel biomarkers such as Neutrophil gelatinase-associated lipocalin (NGAL), cystatin C, Interleukin-6 (IL-6) and Fibroblast Growth Factor (FGF-23) can improve the early diagnosis of AKI and CKD (193–197), predict clinical outcome (198, 199) and help quantitation of severity in CKD (200). One or more of these or other novel biomarkers may prove useful for monitoring of renal function in children and adults with CP but this has not, as yet, been assessed in this population.
Gastrointestinal Dysfunction
There is a high prevalence of gastrointestinal disorders in children with cerebral palsy including dysphagia, gastroesophageal reflux disease (GORD) and chronic constipation (201). Dysphagia is related to inefficiencies in the oral preparation, oropharyngeal and esophageal stages of swallowing. It is a common problem in children with CP, occurring in 43% (83), and can be caused by oromotor dysfunction, oral sensory impairment, abnormal neurological development and esophageal dysmotility. GORD in these children can make dysphagia worse (85). Clinically significant oral motor dysfunction was demonstrated in a further study in 90% of children with CP (202). Complications of dysphagia in this population include recurrent lower respiratory tract infections and chronic aspiration, as discussed previously, as well as malnourishment, with an association between dysphagia and poor weight gain (85).
The issue of drooling is inextricably linked with dysphagia and GORD and is defined as “the unintentional loss of saliva and contents from the mouth” (203). It occurs in between 37.4 and 58% (204) of children with cerebral palsy and is subdivided into anterior and posterior types (205). Anterior drooling occurs when saliva leaks from the mouth and posterior drooling is where the saliva spills into the oropharynx and hypopharynx (205). Children with cerebral palsy do not have excessive salivation, but an oromotor muscle incoordination and sensory perception issues (206). Severe anterior drooling can lead to social isolation, damp clothing, irritated facial skin, interference with speech, damage to books, communication aids and computers (203, 207). Posterior drooling can lead to aspiration which may result in pneumonia.
Sialorrhoea can be exacerbated by GORD in children with CP through the oesophagogastric reflex. Treatment of children with CP, siallorhoea and gastro-esophageal reflux with antireflux medication has been shown to reduce drooling in a double-blind, placebo-controlled, crossover trial (n = 9) (208).
A multidisciplinary approach to the management of drooling is advisable (209, 210). The team can include neurologists, otolaryngologists, pediatricians, plastic surgeons, speech and language therapists, psychologists, physiotherapists, nurses and teachers. The treatment options vary from less invasive behavioral and non-medical to pharmacological interventions to more invasive surgical management usually reserved for children over 4 years of age.
The two main medical treatments are anti-cholinergic agents and intraglandular botulinum toxin injections to the submandibular and/or parotid glands. The anti-cholinergic drugs most commonly used are atropine, benztropine, glycopyrrolate bromide and scopolamine. Pharmacological interventions cannot selectively block stimulation of the salivary glands and as a result unwanted side effects can occur for example, constipation, blurred vision behavior disturbance, urinary retention and thickening of secretions (211).
Botulinum Toxin Injections into the submandibular glands and/or parotid glands under ultrasound guidance is often used if there is an inadequate response to the anticholinergic drugs. The main drawback is that they are only effective for about 6 months, need to be repeated and responsiveness can diminish over time. Parents should be advised of the potential for adverse effects such as xerostomia and dysphagia (205). There is insufficient evidence on the efficacy of many of these pharmacological treatments in siallorhoea (204), however, benztropine and Botulinum Toxin injections have been shown to reduce drooling in a recent meta-analysis (38).
Surgery is usually reserved for children that have failed all the less invasive treatments discussed. Submandibular or parotid duct ligation as well as submandibular gland excision are not without significant complications, although rare. For example, salivary stones, scarring, facial and hypoglossal nerve damage (212, 213) can occur. Submandibular gland duct relocation is useful for anterior drooling (214) but contraindicated for posterior drooling.
Symptoms suggestive of GOR are seen in 77% of children with CP (n = 58), of whom 90% were subsequently proven to have GORD by pH monitoring and endoscopic evidence of oesophagitis (201). The increased risk of GORD in children with CP is due to poor posture, scoliosis, increased intra-abdominal pressure from spasticity or medication side effects. These side effects include those of anticonvulsants which may increase nausea, vomiting and dysphagia, and, in turn, worsen the severity of GORD (215). Prospective analysis of 101 children with NI demonstrated that early onset of NI, mitochondrial disorders and EEG abnormalities were significant risk factors for GOR (216). In a cohort of children with CP and GORD (n = 18), significantly prolonged gastric emptying and abnormal esophageal motility was demonstrated on manometry (p < 0.01), suggesting that GI motility dysfunction contributes to GOR and oesophagitis in this group (201).
The diagnosis of GOR in some children with CP may be challenging because of difficulties with communication. GOR has been found in 91% of children with CP who have regurgitation, vomiting, recurrent abdominal pain, haematemesis and/or pulmonary aspiration (201). However, in many cases these symptoms may not be present. Considerable agitation and autoaggressive behavior can be a marker for pathological GOR and therefore, should be excluded when parents report these behaviors (217).
The North American and European Society for Pediatric Gastroenterology Hepatology and Nutrition (NASPGHAN, ESPGHAN) have published guidelines on the treatment of GORD, based on the available evidence (39). Lifestyle factors such as raising the head of the bed and avoiding exposure to smoke may be helpful in treating GOR. Thickening of feeds can help to reduce overt regurgitation and vomiting (39). The mainstays of pharmacological management are histamine receptor antagonists (H2RA) and proton pump inhibitors (PPI). It is recommended that PPIs are used as first line pharmacologic treatment of GORD, with use of H2RAs if PPIs are not available or contra-indicated (39). Baclofen is an alternative which may be considered prior to surgery if other medical treatments have failed (39). Surgical interventions, such as fundoplication, can be considered in children with GORD and neurological impairment if optimal medical therapy has been unsuccessful, with total oesophagogastric dissociation reserved as a rescue procedure for those in whom fundoplication has failed (39).
Children with more severe CP are at greater risk of poor nutritional status (218). It is estimated that up to 46% of children with CP can be classified as undernourished (219), however, it is only documented in 7.9% of children with CP admitted to hospital, suggesting significant under recognition (220). Dysphagia, GORD and constipation were significantly associated with malnourishment (220). ESPGHAN has published detailed recommendations for monitoring and managing nutritional status in children with NI, including biannual monitoring of anthropometric measurements and annual measurement of micronutrients. Nutritional optimisation may be beneficial in improving the functional status of children with CP (35). In a prospective study (n = 14) where children with CP and malnourishment completed a 6 month nutritional rehabilitation programme, 64% had an improvements in their “Gross Motor Function Measure” scores (221).
Constipation is also a substantial problem in children with neurodevelopmental disabilities (222) affecting almost ¾ of children with CP (201). This is mainly due to prolonged transit time, particularly in the more proximal segments of the colon (201). Treatment of constipation for children with NI is not significantly different than their typically developing peers apart from avoidance of polyethylene glycol and liquid paraffin in those with significant risk of pulmonary aspiration. Additionally, increased fluid and fiber intake can be considered in this population (223).
Children with NI are at significant risk of acute and chronic pain with the abdomen accounting for a significant proportion (11–32%) of these symptoms (36, 37). In a cross-sectional study involving children with CP aged 5–18 years (n = 288), 67.1% had acute pain and 31.4% had chronic pain with risk factors including dyskinesia, bilateral involvement and GMFCS IV-V (224) and, of those with acute pain, 42% also reported chronic pain (224). Abdominal pain may occur as a result of nociceptive pain sources such as appendicitis, pancreatitis or cholecystitis, all of which may be more difficult to diagnose in children with CP, particularly if they are non-verbal. Children with CP are also at increased risk of pain as a direct result of neurological dysfunction, including central neuropathic pain and visceral hyperalgesia (36, 225). This is particularly seen with lesions in the thalamus and spinothalamic tract. In these situations, normal or minimally noxious stimuli can result in significant pain (226). This means that routine activities, such as feeding, which inevitably produce gut distension, may provoke considerable pain. Non-pharmacological approaches to treatment such as reduction in feed volumes, venting of feeding tubes or alterations in feed timing may be useful. Management of pain, including abdominal pain, in these children should initially begin with a thorough assessment for any nociceptive sources of pain. If no obvious source can be identified a trial of empiric medical treatment with gabapentin has been suggested, with escalation to tricyclic antidepressants and methadone considered the following steps (40). In two retrospective studies (n = 22 and n = 42), gabapentinoids have been shown to reduce pain behaviors (227, 228). Prospective trials to confirm efficacy in this cohort would be useful. Above all, a systematic and thorough approach to assessing and managing pain in these children is required.
Gut and intestinal failure is increasingly being recognized in children with neurodisabling conditions (229). This is not surprising given the sizeable interface between the central and enteric nervous system. However, there is a significant paucity of research in this area which should be addressed in the future.
The Hematological System
There is evidence to suggest that cerebral infarction secondary to pre or perinatal cerebral occlusion occurs in 13-37% children with hemiplegic CP and the infarction may occur due to a thrombophilia (12, 41, 230). Gunther et al. (41) studied 91 cases of neonatal arterial-ischaemic stroke and found that 68% had at least one pro-thrombotic tendency. However it is important to note that 24% of the 182 controls also had an abnormality. Mercuri et al. noted that the factor V Leiden mutation in particular may be associated with poorer outcomes in neonatal stroke (230). Coagulopathies and thrombophilias have been reported to be associated with neonatal stroke include antiphospholipid antibodies (231), anti-cardiolipin antibodies (232), increased lipoprotein (41), protein C resistance (41) and protein C and S deficiencies (12). The American Academy of Neurology and Child Neurological Society have recommended that diagnostic testing for a coagulation disorder should be considered in children with hemiplegic CP although the level of evidence was relatively low (12). The NICE guidelines for assessment of cerebral palsy (13) make no recommendation for routine testing for coagulation or thromboembolic disorders in children with CP.
The coagulation and inflammatory systems overlap and interact. Activated coagulation factors are proinflammatory and in turn inflammation can promote coagulation (233). Elevated inflammatory markers and coagulation factors coexist in neonates who subsequently develop CP (233). In children with NE, a risk factor for CP, it is known that coagulation parameters are strong predictors of outcome (234). Antenatally, it has been shown that children who subsequently develop CP are more likely to have had 2 or more placental lesions of thrombosis or inflammation (235) and that fetal thrombotic vasculopathy is significantly associated with NE (236). It is likely, therefore, that the interplay between coagulation and inflammation contribute to white matter damage and multi-organ dysfunction (237) and are thus important in the etiology of CP (233).
Beyond the neonatal period, children with CP have prothrombin times (PT) and activated partial thromboplastin times (APTT) which are within the normal ranges but which are significantly longer than controls (42). They also have significantly reduced calcium and magnesium levels, both important cofactors for coagulation (42). Brenn et al. examined bleeding in children with severe spastic quadriplegia during posterior spinal fusion surgery compared with controls (n = 34) and found that children with CP have significantly more bleeding and that bleeding occurs earlier in the course of surgery (42). Kannan et al. also reported significantly more blood loss during spinal surgery in children with neuromuscular scoliosis compared to those with idiopathic scoliosis (n = 25) and found reduced factor VII activity (238). Children with CP are also often taking medication which can effect coagulation, as reported in several case reports, such as valproate (239, 240), carbamazepine (241), and azithromycin (242). Therefore, consideration of coagulopathy and thrombophilia in children with CP is important, particularly prior to surgery, including the administration of prophylactic tranexamic acid and early use of blood products (44).
There is a high rate of nutritional deficiency amongst children with CP (243–245), which may correlate with anemia. However, evidence regarding anemia in children with CP is sparse. In institutionalized children and young people (n = 108, age 8–29 years) with cerebral palsy, iron-deficiency was reported in 38% and anemia was found in 33.3% of participants. Both iron-deficiency and anemia were significantly increased in those who had liquid diets compared to normal diets (95.6 vs. 22.3% and 87 vs. 18.8%, respectively) (246). In another case series (n = 30) iron-deficiency anemia was found in 4 (13.3%) children with neuromotor disabilities (247). In comparison, rates of iron-deficiency anemia in both low and middle-income children in the United States of America have been reported to range between 2 and 3% (248, 249). This is significant because iron-deficiency, even without anemia, is known to affect neurodevelopment including motor, cognitive and social-emotional function (43). In children with spastic CP, it has been reported that iron deficiency anemia has a negative effect on functional ability and muscle strength (250). Further research is needed to quantify the extent of iron deficiency and anemia in children with CP to ensure adequate treatment if necessary.
The Immune System—Inflammation and Infection
NE accounts for around 24% of all cases of CP in term infants (4, 5). Infants with NE have been reported to have persistent inflammatory response over the 1st week of life, with higher neutrophil, monocyte and Toll-like receptor-4 expression, correlating with the degree of brain injury (251, 252). High concentration of Interleukin (IL)-1, 8, 9 and tumor necrosis factor (TNF)-α were demonstrated in neonatal blood from children with Cerebral Palsy in comparison with control children (253). One of the most important underlying pathophysiological mechanisms leading to CP includes intra-amniotic inflammation and infection eliciting an inflammatory response and damage to the developing brain. Fetal inflammatory response syndrome (FIRS) is a severe form of intra amniotic infection or inflammation. It stimulates the activation of innate immune system of the fetus, similar to that seen in adult inflammatory response syndrome. FIRS can lead to multi-organ dysfunction and causes neurological, renal and hematological abnormalities (254).
Perinatal inflammation is also associated with many neuropsychiatric and neuro-psychological disorders and it is suggested that inflammation has long term consequences on the brain during childhood (255). Children post-NE have been shown to have persistent inflammatory responses at school age (31). The injury processes can persist for months and years and a tertiary mechanism of damage, which includes inflammation and epigenetic changes, has been proposed (255). Dysregulated immune function is also found in school age children with CP who had brain injury in the neonatal period (30). School-age, preterm children with CP secondary to PVL had significantly higher levels of TNF-α in peripheral blood mononuclear cells (PBMC's) after lipopolysaccharide (LPS) stimulation compared to control school-age preterm children with normal neurodevelopment.
Infections are a significant contributor to childhood death, particularly in those with underlying conditions. CP was the most common underlying condition associated with death due to infection in the 1–4 year age category (45). Pneumonia in particular is an important cause of morbidity and mortality in children with CP, especially among those with severe spastic quadriplegia, epilepsy and intellectual disability (46). An observational data-linkage study of a developmental anomaly registry with a national death index revealed that, of those with available causes of death, 58.6% were attributable to respiratory causes, of whom 49% died of pneumonia at a mean age of 14.6 years (11). Non-respiratory infections accounted for a further 5% of deaths at a mean age of 16.6 years.
Children with CP also appear to be at increased risk of infective complications following surgery. In a retrospective analysis of 1,250 children with CP who underwent appendectomy had a significantly greater risk of developing sepsis/organ failure (adjusted OR = 2.47; p = 0.13), operation-related infections (adjusted OR = 2.65; p = 0.001), pneumonia (adjusted OR4.26; p < 0.001), and urinary tract infection (adjusted OR = 5.19; p < 0.001) (47). The mechanisms underlying this increased risk are unknown and likely multifactorial.
Vaccination is one of the most successful ways of preventing infections. Children with CP should follow the vaccination schedule in the country in which they live. However, additional vaccinations may be recommended to provide them with added protection given their associated co-morbidities. Children with CP have a 2-3 times higher risk of incomplete or delayed immunization (256) A retrospective review of data from children <7 years of age included in the Victorian CP register (n = 476) found that 19.2% were overdue at least one vaccine (256). It has also been reported that children with moderate to severe motor impairment are less likely to have received all of their vaccinations than those with less severe impairment (n = 98; p < 0.05) (257).
Children with neurological disorders have been acknowledged as a population which is at increased risk of serious complications of influenza (258). Despite this only 74% of pediatricians in the United States recognized CP as a high-risk condition (n = 412) (259). This survey-based study also found that 50% of children with a neurologic or neurodevelopmental disorder (n = 1143) were already vaccinated or their parents planned for vaccination against influenza (259) and programmes to improve the uptake rate are required.
A large population-based, case-control study in Denmark (n = 1,665) shows a higher risk of invasive pneumococcal disease amongst children with several chronic conditions. The adjusted risk ratio for children with a neurological disorder compared with children without such a disorder was 3.0 (95% confidence interval = 2.1–4.3) (48). There may be an argument for providing these children with extended-coverage polyvalent pneumococcal vaccines but this requires further research.
Recent research has shown that inflammatory responses in children with CP are altered. Further research may help to clarify the role of inflammation as a tertiary cause of neurological injury with the potential to develop therapeutic targets. There may also be a significant impact on immune function and further work is needed to optimize vaccination programs for children with CP and improve infection-associated morbidity and mortality.
Metabolic Considerations in Assessing MOD
Inborn errors of metabolism (IEM) can present with CP like symptoms. A systematic review by Leach et al. identified almost 70 IEMs belonging to 13 different biochemical categories, that closely mimic cerebral palsy (CP). While these only account for 0.1–0.2% of CP cases, early diagnosis is essential to prevent organ damage (260). Metabolic investigations should be considered where the clinical history is consistent with a metabolic disorder. These features may include chronic progression, neurodevelopmental regression or non-central nervous system (CNS) organ involvement (49). Other signs include absent history of perinatal injury, and pattern of disease inheritance; so called “familial CP” which can be elicited by obtaining a thorough family history and/or presence of involuntary movements, ataxia, muscle atrophy, oculomotor abnormalities or sensory loss (260–262). In children with CP and normal magnetic resonance imaging (MRI), further testing for metabolic and/or genetic conditions has been recommended (263, 264). The presence of abnormal MRI findings which are unexpected or inconsistent with the suspected etiology should also warrant metabolic investigations (263). An example of this are globus pallidus abnormalities which can be indicative of the rare neurometabolic disorder, neurodegeneration with brain iron accumulation (NIBA) with pantothenate kinase-associated neurodegeneration (PKAN) the most frequent subtype identified (265).
Individualized investigations for IEMs can be planned according to the individual clinical picture i.e., history, examination and any neuroimaging. These investigations may include plasma, urine or cerebrospinal amino acids, a plasma acylcarnitine profile and urine organic acids, mucopolysaccharides, and oligosaccharides (261, 266). Targeted biomarker analysis or single gene analysis are useful where the phenotype and investigative results highly correlate with a specific metabolic condition. For example a clinical picture of significant dystonia, macrocephaly and MRI revealing widened Sylvian fissures and basal ganglia abnormalities may be confirmed as glutaric aciduria type 1 with urine organic acid analysis and genetic study of the glutaryl CoA dehydrogenase (GCDH) gene (261, 262). However due to the overlapping and, in certain instances, unspecific phenotypes of IEMs, next generation and whole exome sequencing has proven useful in non-specific findings where no distinct etiology is suspected (260, 261). Due to the sheer number of IEMs and the generalized diagnostic approach currently used, Metabolomics has emerged as an innovative method of improving diagnostic efficiency of IEMs as there are numerous possible diagnoses and it may be potentially used as a tool in precision medicine (267, 268). It should be used in conjunction with next generation and exome sequencing to help clarify the pathogenicity of genetic variants.
Mitochondrial disorders are neurometabolic diseases which can also present with CP like symptoms (260, 262). They are characterized by dysfunctional energy production and typically manifest as multi-organ dysfunction, often with neurological impairment and can be similar to CP (261). Mitochondria are postulated to play a significant role in hypoxic-ischaemic events which may lead to CP and mitochondrial targets are now being explored as a potential future therapeutic intervention as an alternative to therapeutic hypothermia in those with perinatal brain injury. These targets include protecting from mitochondrial permeabilization, directly targeting mitochondrial downstream apoptotic pathways and indirect protection and preservation of mitochondrial function (269).
It is important to be mindful of potential metabolic diagnoses when seeing children with CP as they can have significant implications, the most significant of which is to prevent deterioration and improve clinical outcomes.
Genetic Considerations in Assessing MOD
An increasing number of studies implicate genetics in the etiology of cerebral palsy (CP). A genetic component to cerebral palsy may prompt the clinician to be particularly vigilant for specific multi-organ abnormalities. Several non-metabolic single gene disorders can present as CP. Many single gene mutations linked with CP have been identified using whole exome sequencing, including mutations in KCNC3, KANK1, AP4MI, GAD1, and ADD3 with different mutations linked to the development of different subtypes of CP (50, 270–272). For example, mutations in KCNC3 have been found in some individuals diagnosed with ataxic CP (50) and heterozygous deletions of KANK1 are associated with early motor delay and hypotonia, progressing to spastic quadriplegia and intellectual disability (273). Each of these single gene disorders are individually rare but together may account for a significant number of cases of CP. They are important to recognize as a genetic diagnosis can help with prognosis, monitoring and family counseling.
It is clear there is no single “CP gene” but there is increasing recognition of a genetic element to CP in a large number of cases. Despite improvements in obstetric, prenatal and perinatal care, there has been little change in the incidence of CP in term neonates, which may be due to an underlying genetic pathophysiology (270, 273). In most studies the prevalence of CP appears to be higher in the male population compared to the female population and it has been suggested that recessive X-linked chromosome variants may contribute to this difference (270). Higher rates of CP have also been reported in monozygotic twins and consanguineous families (271). Furthermore, the presence of coexisting congenital anomalies is significantly higher in the CP population compared to their healthy counterparts with rates of 11–32 and 1–2%, respectively (270, 271).
A significant number of candidate CP genes have also been identified alongside other genetic polymorphisms which have been proposed to contribute to the etiology of CP including copy number variants and single nucleotide polymorphisms (271). De novo and inherited genetic variants may account for up to 30% of CP cases and have been postulated to, in some cases, directly cause CP, while in others merely contribute to CP susceptibility (270, 274). For example, a large Australian case-control study of children with CP (n = 443) found that polymorphisms in Toll Like Receptor-4 (TLR-4) reduced the risk of developing CP, while polymorphisms in IL-6 and IL-8 may increase susceptibility to CP in the presence of viral exposure (51).
Finally, epigenetic modifications are gaining increasing recognition as contributing to tertiary brain damage following an initial insult (275). Epigenetics refers to any process by which gene activity is altered without changing the DNA sequence, including methylation and histone, acetylation or micro-RNA modifications (276, 277). It is thought that early life exposures can modify the epigenome and provide a link to neurodevelopmental outcome (278, 279). Epigenome-wide analysis of 15 monozygotic twins discordant for CP showed regional differences in DNA methylation associated with development of CP (280). Gene loci involved were associated with hypoxia, inflammation and cell adhesion. Further research with larger sample sizes would be useful to confirm these findings. Differences in methylation were also noted to be significantly different between adolescents with CP and controls (n = 32, p < 0.05) (281). In the future, the epigenome may have potential as a therapeutic target to improve neurodevelopmental outcomes in CP.
The vast genetic heterogeneity underlying CP emphasizes the complexity of the contribution of genetics to the etiology and development of CP and points toward a multifactorial etiology of CP with interaction between genetics and the environment (271, 282). While genetic causation should not change the clinical diagnosis of CP, inclusion as a subclassification by etiology may allow for more targeted therapy in these cases (273, 274).
The Endocrine System
All children with disability are at risk for some form of endocrine dysfunction due to the disruption of endocrine feedback secondary to abnormalities in muscle, bone or brain mechanisms. Regular assessment from an endocrine perspective should be considered with the emphasis on growth, pubertal status and bone health. Knowing the underlying etiology of cerebral palsy can focus endocrine evaluation but is not essential.
All children with cerebral palsy should have formal measurement of body proportions at least twice per year to guide nutrition and to identify growth anomalies. Assessing length or height in proportion to weight is important to ascertain whether short stature is due to nutritional deficiency or a hormone imbalance. Nutritional deficiency is more likely to result in delayed puberty. Exogenous obesity can drive growth in childhood but following puberty can result in obesity in an immobile child causing long term implications for respiratory function and sleep apnoea. Children with midline defects will have hypothalamic pituitary axis dysfunction which can result in growth hormone deficiency impacting on tone, mood and glycaemic control as well as stature. Studies have indicated that circulating GH secretion profiles are lower in children with cerebral palsy compared to controls (52, 283). Hypoglycaemia can occur as a consequence of metabolic stress in the newborn period, growth or cortisol hormone deficiency. Deranged glucose metabolism may only become apparent at times of stress and illness and in children with spasticity and seizure disorders could be missed.
CP is the most common childhood condition associated with osteoporosis, and children with CP frequently sustain fractures with minor trauma (53). Any child or adolescent with immobilization, nutritional deficiency (especially impacting vitamin D or calcium metabolism) or delays in pubertal onset will be impacted by a reduction in bone mass accrual. Apparent reduction in bone density may also be artifactual if not adjusted for height. Growth hormone has been shown to be positively associated with surrogate markers of bone turnover in puberty (284) suggesting that this could be a factor in the attainment of bone density due to the lower circulating levels of growth hormone (283). In a systematic review of children with severe cerebral palsy, significant determinants of low BMD were limited ambulation, feeding difficulties, previous fracture, anticonvulsant use, and lower fat mass (285). The International Society of Clinical Densitometry has identified the importance of correct bone mineral density assessment in cerebral palsy and has issued official positions on both the definition of osteoporosis in this condition (286) and the appropriate site of BMD assessment using bone densitometry (287). If a child with CP is considered at risk of osteoporosis, bone densitometry (DXA) scans should be performed to assess baseline at 6 years of age, and repeated every 1–2 years depending on individual risk factors (53). In treating children with CP and osteoporosis there is probable evidence for bisphosphonates, possible evidence for vitamin D and calcium supplementation and insufficient evidence for weight bearing activities as effective interventions to improve BMD (55, 56). Bisphosphonates have been shown to improve pain on manipulation in children with CP and osteoporosis (288).
Puberty also plays a role in the development of bone health. Clinical assessment of puberty with Tanner staging is therefore recommended (57). Mean age of breast development in girls with CP is similar but with wider range of onset, while Menarche occurs later in Caucasian girls with CP (289). Early adrenarche is seen in cerebral palsy but more commonly in those with hydrocephalus or associated epilepsy. This is not indicative of central precocious puberty and can progress slowly over many years before true pubertal onset (54). Many children and adolescents with cerebral palsy can experience normal pubertal progression and menses (290).
The Musculoskeletal System
Scoliosis and hip dislocation are common problems in children with CP. The overall incidence of scoliosis in CP of 20–25% (291), with a risk of up to 90% for spinal deformities in patients with GMFCS level V (58). Scoliosis is related to poor truncal tone and muscle weakness (292) and predictors for scoliosis include GMCFS IV and V, epilepsy and limited knee extension (293). The scoliosis pattern in children with GMFCS IV is usually a single long C- shaped curve that is most often kyphotic, but sometimes lordotic. In ambulatory patients with less motor involvement the pattern of deformity may be similar to that in idiopathic scoliosis with both thoracic and lumbar components (292, 294). Progression of scoliosis is usually gradual, but onset of puberty, deterioration in neurological function or prolonged time spent in a wheelchair can lead to a more rapid progression (292). Progressive scoliosis causes difficulties with daily care and mobilization, can lead to pain, cardiac and pulmonary complications, altered seizure thresholds and skin compromise (295).
Hip dislocation develops in 15–20% of cases (59). There is almost a direct link between higher levels of GMFCS and risk of hip subluxation. Risk of developing a hip migration index (MI) >30% is ~30, 50, and 80% at GMFCS III, IV, and V, respectively (296). Rate of progression of the MI increases from almost 2% at GMFCS III to 12% at GMFCS V (296). Hip dislocation develops because of contractures and spasticity of adductors, hamstrings and hip flexors (297). The combination of this spasticity and reduction in weight bearing lead to acetabular dysplasia (298). Hip surveillance is considered essential in reducing hip dislocation and need for surgery (299). Hippotherapy may lead to improved symmetry and stability although grade of evidence is low (299). Splints may reduce rate of dislocation but are ineffective at preventing hip dislocation (299). There is insufficient evidence as to whether therapy with botulinum toxin can prevent hip dislocation (300).
Both of the aforementioned orthopedic complications of CP may be linked. Hip dislocation or windswept deformity of the hip many cause pelvic obliquity and trigger scoliosis, while scoliosis itself can lead to pelvic obliquity and thus increase the risk of hip dislocation especially on the high side (59). Early identification of these issues of scoliosis and hip dislocation are important for improved surgical outcomes, although there are multiple prerequisites before consideration of surgery, such as Cobb angle >40–50 degrees which is progressive and interfering with sitting, age and medical comorbidities (292).
The upper limb may also develop significant complications in CP. Upper limb contractures developed in 34% of a population-based sample of children with CP (n = 771). These contractures began at preschool age and the best predictor was high scores on the Manual Ability Classification System (301). The level of evidence for interventions relating to the upper limb is much lower than that for the lower limbs. Further high quality studies in this area are required.
Discussion
Multi-organ dysfunction in cerebral palsy to date has not been quantified. Better insight into multi organ dysfunction in children with CP may be gained by using novel biomarkers and new diagnostic tools. In this review, we focused on various long-term issues associated with each organ system and have identified parameters and novel biomarkers for monitoring MOD. In the future, with further study, biomarkers including serum and urinary NGAL, cystatin C and IL-6 show good ability to predict AKI and may be useful in long-term follow up. Cardiology assessment including measurement of serum troponin, pro-NT-BNP, echocardiography using tissue doppler and speckle-tracking may be used to monitor cardiovascular function in children with CP. The use of these biomarkers may initially prove most useful to the research community in providing new insights in to subtle organ dysfunction. However, this may lead to discovery of new therapeutic targets and improved outcomes. There is also the possibility that these biomarkers will become the norm in clinical care if there are clinically-relevant long-term consequences noted in those areas.
Throughout this article, where possible, we have attempted to comment on the impact of dysfunction in each of the organ systems involved. For example, pain is one of the most significant consequences of dysfunction in the gastrointestinal system. It is also an example of the complex interactions between the neurological and non-neurological systems which may receive further attention by considering CP as a multi-system disorder with multi-organ dysfunction. However, in many cases, the answer to that question has not been adequately addressed in the literature. We hope that this article highlights some of those gaps and provokes further research into outcomes in the less well-studied organ systems of children with CP.
An example of an area where further research and exploration of consequences is required is the immune system. Children with CP have altered immune function compared to age-matched controls. Understanding the immune response in these children with CP and exploring systemic inflammation holds promise for future development of immunomodulatory adjunctive therapies. Further research in this area can contribute to better prediction of outcome and improved prognosis.
We believe that a MOD scoring system would prove useful, perhaps especially in the research context as a way to correlate with other health related outcomes. It may also prove useful in allowing advanced clinical planning, discussions around prognosis and follow-up of children with CP throughout the lifespan. CP registries are now in existence throughout the world. The information provided by these registries, as well as the expert collaboration that they promote, in conjunction with a MOD scoring system, might allow for the production of tailored guidelines for follow-up and management of MOD in children with CP. We suggest that follow up of multi-organ function is important to identify and pre-emptively manage potential long-term complications in CP.
Another issue which may be raised, if one considers CP as a multi-system disorder, is the definition of CP. The definition developed by Rosenbaum et al. (1) which was published in 2007 is probably the most widely accepted. However, recently questions have arisen regarding whether this definition needs to be revisited in light of increasing knowledge in several areas, for example, the genetic causes and predispositions to development of CP (270, 272–274). The Rosenbaum definition is limited in its reference to co-morbidities stating that “The motor disorders of cerebral palsy are often accompanied by disturbances of sensation, perception, cognition, communication, and behavior, by epilepsy, and by secondary musculoskeletal problems” (1). If the definition of CP is revisited, consideration may be given to including a reference to the multi-system nature of the disorder.
This review highlights the need for an awareness amongst healthcare professionals of the multi-systemic complications that are associated with CP, particularly in those with greater levels of impairment. That many of these children communicate non-verbally compounds the difficulties that the physician faces in understanding the source of many of their symptoms. The cause of symptoms such as pain, behavioral or sleep disturbance may be multi-factorial and present substantial diagnostic challenges. A step-wise, logical, multi-system approach, with support from a multi-disciplinary team, is required to ensure that the best care is provided to these children and their families.
Author Contributions
JA contributed to the conception and design, acquisition, analysis and interpretation of data, drafted the first and subsequent drafts of the work, revised it critically, and approved the final version of the manuscript. ZZ, SD, LW, and ZA contributed to the conception and design, acquisition, analysis and interpretation of data, drafting the work and revising it critically, and approved the final version of the manuscript. MS, OF, AG, AJ, TL, SQ, BE, JR, SP, PK, ER, CM, LB, OH, LG, and SK contributed to the analysis and interpretation of data, revising the work critically, and approved the final version of the manuscript. DM and EM contributed to the conception and design, analysis and interpretation of data, drafting the work and revising it critically, and approved the final version of the manuscript. All authors contributed to the article and approved the submitted version.
Funding
National Children's Hospital Foundation, Tallaght, Dublin & National Children's Research Centre (NCRC), Dublin, Ireland (Award no. D/19/2).
Conflict of Interest
The authors declare that the research was conducted in the absence of any commercial or financial relationships that could be construed as a potential conflict of interest.
Publisher's Note
All claims expressed in this article are solely those of the authors and do not necessarily represent those of their affiliated organizations, or those of the publisher, the editors and the reviewers. Any product that may be evaluated in this article, or claim that may be made by its manufacturer, is not guaranteed or endorsed by the publisher.
References
1. Rosenbaum P, Paneth N, Leviton A, Goldstein M, Bax M, Damiano D, et al. A report: the definition and classification of cerebral palsy April 2006. Dev Med Child Neurol Suppl. (2007) 109:8–14. doi: 10.1017/s001216220500112x
2. Stavsky M, Mor O, Mastrolia SA, Greenbaum S, Than NG, Erez O. Cerebral palsy-trends in epidemiology and recent development in prenatal mechanisms of disease, treatment, and prevention. Front Pediatr. (2017) 5:21. doi: 10.3389/fped.2017.00021
3. Perra O, Rankin J, Platt MJ, Sellier E, Arnaud C, De La Cruz J, et al. Decreasing cerebral palsy prevalence in multiple births in the modern era: a population cohort study of European data. Arch Dis Childhood. (2020) 2020:fetalneonatal-2020-318950. doi: 10.1136/archdischild-2020-318950
4. Keogh JM, Badawi N. The origins of cerebral palsy. Curr Opin Neurol. (2006) 19:129–34. doi: 10.1097/01.wco.0000218227.35560.0d
5. Badawi N, Felix JF, Kurinczuk JJ, Dixon G, Watson L, Keogh JM, et al. Cerebral palsy following term newborn encephalopathy: a population-based study. Dev Med Child Neurol. (2005) 47:293–8. doi: 10.1017/S0012162205000575
6. Jacobs SE, Berg M, Hunt R, Tarnow-Mordi WO, Inder TE, Davis PG. Cooling for newborns with hypoxic ischaemic encephalopathy. Cochrane Datab Syst Rev. (2013) 2013:Cd003311. doi: 10.1002/14651858.CD003311.pub3
7. Brooks JC, Strauss DJ, Shavelle RM, Tran LM, Rosenbloom L, Wu YW. Recent trends in cerebral palsy survival. Part I: period and cohort effects. Dev Med Child Neurol. (2014) 56:1059–64. doi: 10.1111/dmcn.12520
8. Brooks JC, Strauss DJ, Shavelle RM, Tran LM, Rosenbloom L, Wu YW. Recent trends in cerebral palsy survival. Part II: individual survival prognosis. Dev Med Child Neurol. (2014) 56:1065–71. doi: 10.1111/dmcn.12519
9. Himmelmann K, Beckung E, Hagberg G, Uvebrant P. Gross and fine motor function and accompanying impairments in cerebral palsy. Dev Med Child Neurol. (2006) 48:417–23. doi: 10.1017/S0012162206000922
10. Shevell MI, Dagenais L, Hall N. Comorbidities in cerebral palsy and their relationship to neurologic subtype and GMFCS level. Neurology. (2009) 72:2090–6. doi: 10.1212/WNL.0b013e3181aa537b
11. Blair E, Langdon K, McIntyre S, Lawrence D, Watson L. Survival and mortality in cerebral palsy: observations to the sixth decade from a data linkage study of a total population register and National Death Index. BMC Neurol. (2019) 19:111. doi: 10.1186/s12883-019-1343-1
12. Ashwal S, Russman BS, Blasco PA, Miller G, Sandler A, Shevell M, et al. Practice parameter: diagnostic assessment of the child with cerebral palsy: report of the Quality Standards Subcommittee of the American Academy of Neurology and the Practice Committee of the Child Neurology Society. Neurology. (2004) 62:851–63. doi: 10.1212/01.WNL.0000117981.35364.1B
13. Shaunak M, Kelly VB. Cerebral palsy in under 25 s: assessment and management (NICE Guideline NG62). Arch Dis Childhood-Educ Pract. (2018) 103:189–93. doi: 10.1136/archdischild-2017-312970
14. National Institute for Health and Care Excellence. Spasticity in under 19s: Management: National Institute for Health and Care Excellence (NICE) (London), (2012).
15. Dutton GN, Calvert J, Cockburn D, Ibrahim H, Macintyre-Beon C. Visual disorders in children with cerebral palsy: the implications for rehabilitation programs and school work. Eastern J Med. (2012) 17:178.
16. Philip SS, Dutton GN. Identifying and characterising cerebral visual impairment in children: a review. Clin Exp Optom. (2014) 97:196–208. doi: 10.1111/cxo.12155
17. Himmelmann K, Sundh V. Survival with cerebral palsy over five decades in western Sweden. Dev Med Child Neurol. (2015) 57:762–7. doi: 10.1111/dmcn.12718
18. Millman AJ, Finelli L, Bramley AM, Peacock G, Williams DJ, Arnold SR, et al. Community-acquired pneumonia hospitalization among children with neurologic disorders. J Pediatrics. (2016) 173:188–95.e4. doi: 10.1016/j.jpeds.2016.02.049
19. Piccione JC, McPhail GL, Fenchel MC, Brody AS, Boesch RP. Bronchiectasis in chronic pulmonary aspiration: risk factors and clinical implications. Pediatr Pulmonol. (2012) 47:447–52. doi: 10.1002/ppul.21587
20. Hull J, Aniapravan R, Chan E, Chatwin M, Forton J, Gallagher J, et al. British Thoracic Society guideline for respiratory management of children with neuromuscular weakness. Thorax. (2012) 67(Suppl. 1):i1–40. doi: 10.1136/thoraxjnl-2012-201964
21. Newman CJ, O'Regan M, Hensey O. Sleep disorders in children with cerebral palsy. Dev Med Child Neurol. (2006) 48:564–8. doi: 10.1017/S0012162206001198
22. Horwood L, Mok E, Li P, Oskoui M, Shevell M, Constantin E. Prevalence of sleep problems and sleep-related characteristics in preschool- and school-aged children with cerebral palsy. Sleep Med. (2018) 50:1–6. doi: 10.1016/j.sleep.2018.05.008
23. Koyuncu E, Türkkani MH, Sarikaya FG, Özgirgin N. Sleep disordered breathing in children with cerebral palsy. Sleep Med. (2017) 30:146–50. doi: 10.1016/j.sleep.2016.01.020
24. Grigg-Damberger M, Ralls F. Treatment strategies for complex behavioral insomnia in children with neurodevelopmental disorders. Curr Opin Pulm Med. (2013) 19:616–25. doi: 10.1097/MCP.0b013e328365ab89
25. Hall WA, Nethery E. What does sleep hygiene have to offer children's sleep problems? Paediatr Respir Rev. (2019) 31:64–74. doi: 10.1016/j.prrv.2018.10.005
26. Marcus CL, Moore RH, Rosen CL, Giordani B, Garetz SL, Taylor HG, et al. A randomized trial of adenotonsillectomy for childhood sleep apnea. N Engl J Med. (2013) 368:2366–76. doi: 10.1056/NEJMoa1215881
27. Grychtol R, Chan EY. Use of non-invasive ventilation in cerebral palsy. Arch Dis Child. (2018) 103:1170–7. doi: 10.1136/archdischild-2017-313959
28. Ryan JM, Peterson MD, Ryan N, Smith KJ., O'connell NE, Liverani S, et al. Mortality due to cardiovascular disease, respiratory disease, and cancer in adults with cerebral palsy. Dev Med Child Neurol. (2019) 61:924–8. doi: 10.1111/dmcn.14176
29. Wong BW, Meredith A, Lin D, McManus BM. The biological role of inflammation in atherosclerosis. Canad J Cardiol. (2012) 28:631–41. doi: 10.1016/j.cjca.2012.06.023
30. Lin C-Y, Chang Y-C, Wang S-T, Lee T-Y, Lin C-F, Huang C-C. Altered inflammatory responses in preterm children with cerebral palsy. Ann Neurol. (2010) 68:204–12. doi: 10.1002/ana.22049
31. Zareen Z, Strickland T, Eneaney VM, Kelly LA, McDonald D, Sweetman D, et al. Cytokine dysregulation persists in childhood post Neonatal Encephalopathy. BMC Neurol. (2020) 20:115. doi: 10.1186/s12883-020-01656-w
32. Samijn B, Van Laecke E, Renson C, Hoebeke P, Plasschaert F, Vande Walle J, et al. Lower urinary tract symptoms and urodynamic findings in children and adults with cerebral palsy: A systematic review. Neurourol Urodyn. (2017) 36:541–9. doi: 10.1002/nau.22982
33. Anígilájé EA, Bitto TT. Prevalence and Predictors of Urinary Tract Infections among Children with Cerebral Palsy in Makurdi, Nigeria. Int J Nephrol. (2013) 2013:937268. doi: 10.1155/2013/937268
34. Whitney DG, Schmidt M, Bell S, Morgenstern H, Hirth RA. Incidence rate of advanced chronic kidney disease among privately insured adults with neurodevelopmental disabilities. Clin Epidemiol. (2020) 12:235–43. doi: 10.2147/CLEP.S242264
35. Herrera-Anaya E, Angarita-Fonseca A, Herrera-Galindo VM, Martínez-Marín RD, Rodríguez-Bayona CN. Association between gross motor function and nutritional status in children with cerebral palsy: a cross-sectional study from Colombia. Dev Med Child Neurol. (2016) 58:936–41. doi: 10.1111/dmcn.13108
36. Hauer J, Houtrow AJ. Pain assessment and treatment in children with significant impairment of the central nervous system. Pediatrics. (2017) 139:1002. doi: 10.1542/peds.2017-1002
37. McKinnon CT, Meehan EM, Harvey AR, Antolovich GC, Morgan PE. Prevalence and characteristics of pain in children and young adults with cerebral palsy: a systematic review. Dev Med Child Neurol. (2019) 61:305–14. doi: 10.1111/dmcn.14111
38. Sridharan K, Sivaramakrishnan G. Pharmacological interventions for treating sialorrhea associated with neurological disorders: A mixed treatment network meta-analysis of randomized controlled trials. J Clin Neurosci. (2018) 51:12–7. doi: 10.1016/j.jocn.2018.02.011
39. Rosen R, Vandenplas Y, Singendonk M, Cabana M, Di Lorenzo C, Gottrand F, et al. Pediatric gastroesophageal reflux clinical practice guidelines: joint recommendations of the North American Society for Pediatric Gastroenterology, Hepatology, and Nutrition (NASPGHAN) and the European Society for Pediatric Gastroenterology, Hepatology, and Nutrition (ESPGHAN). J Pediatr Gastroenterol Nutr. (2018) 66:516. doi: 10.1097/MPG.0000000000001889
40. Hauer J. Improving comfort in children with severe neurological impairment. Prog Palliat Care. (2012) 20:349–56. doi: 10.1179/1743291X12Y.0000000032
41. Günther G, Junker R, Sträter R, Schobess R, Kurnik K, Kosch A, et al. Symptomatic ischemic stroke in full-term neonates: role of acquired and genetic prothrombotic risk factors. Stroke. (2000) 31:2437–41. doi: 10.1161/01.STR.31.10.2437
42. Brenn BR, Theroux MC, Dabney KW, Miller F. Clotting parameters and thromboelastography in children with neuromuscular and idiopathic scoliosis undergoing posterior spinal fusion. Spine. (2004) 29:E310–4. doi: 10.1097/01.BRS.0000132513.88038.64
43. Lozoff B, Beard J, Connor J, Barbara F, Georgieff M, Schallert T. Long-lasting neural and behavioral effects of iron deficiency in infancy. Nutr Rev. (2006) 64:S34–91. doi: 10.1301/nr.2006.may.S34-S43
44. Theroux MC, DiCindio S. Major surgical procedures in children with cerebral palsy. Anesthesiol Clin. (2014) 32:63–81. doi: 10.1016/j.anclin.2013.10.014
45. Ladhani S, Pebody RG, Ramsay ME, Lamagni TL, Johnson AP, Sharland M. Continuing impact of infectious diseases on childhood deaths in England and Wales, 2003-2005. Pediatr Infect Dis J. (2010) 29:310–3. doi: 10.1097/INF.0b013e3181d73322
46. Reddihough D, Baikie G, Walstab J. Cerebral palsy in Victoria, Australia: mortality and causes of death. J Paediatr Child Health. (2001) 37:183–6. doi: 10.1046/j.1440-1754.2001.00644.x
47. Dhiman N, Chi A, Pawlik TM, Efron DT, Haut ER, Schneider EB, et al. Increased complications after appendectomy in patients with cerebral palsy: are special needs patients at risk for disparities in outcomes? Surgery. (2013) 154:479–85. doi: 10.1016/j.surg.2013.05.038
48. Hjuler T, Wohlfahrt J, Staum Kaltoft M, Koch A, Biggar RJ, Melbye M. Risks of invasive pneumococcal disease in children with underlying chronic diseases. Pediatrics. (2008) 122:e26–32. doi: 10.1542/peds.2007-1510
49. Heinen F. Metabolic testing in children with cerebral palsy: less doing and more thinking? Dev Med Child Neurol. (2011) 53:198–9. doi: 10.1111/j.1469-8749.2010.03885.x
50. Parolin Schnekenberg R, Perkins EM, Miller JW, Davies WI, D'Adamo MC, Pessia M, et al. De novo point mutations in patients diagnosed with ataxic cerebral palsy. Brain. (2015) 138:1817–32. doi: 10.1093/brain/awv117
51. Djukic M, Gibson CS, MacLennan AH, Goldwater PN, Haan EA, McMichael G, et al. Genetic susceptibility to viral exposure may increase the risk of cerebral palsy. Austr New Zealand J Obstetr Gynaecol. (2009) 49:247–53. doi: 10.1111/j.1479-828X.2009.00999.x
52. Hegazi MA, Soliman OE, Hasaneen BM, El-Arman M, El-Galel NA, El-Deek BS. Growth hormone/insulin-like growth factor-1 axis: a possible non-nutritional factor for growth retardation in children with cerebral palsy. J Pediatr. (2012) 88:267–74. doi: 10.2223/JPED.2197
53. Houlihan CM, Stevenson RD. Bone density in cerebral palsy. Phys Med Rehabil Clin. (2009) 20:493–508. doi: 10.1016/j.pmr.2009.04.004
54. Zacharin M. Endocrine problems in children and adolescents who have disabilities. Horm Res Paediatr. (2013) 80:221–8. doi: 10.1159/000354305
55. Ozel S, Switzer L, Macintosh A, Fehlings D. Informing evidence-based clinical practice guidelines for children with cerebral palsy at risk of osteoporosis: an update. Dev Med Child Neurol. (2016) 58:918–23. doi: 10.1111/dmcn.13196
56. Simm PJ, Biggin A, Zacharin MR, Rodda CP, Tham E, Siafarikas A, et al. Consensus guidelines on the use of bisphosphonate therapy in children and adolescents. J Paediatr Child Health. (2018) 54:223–33. doi: 10.1111/jpc.13768
57. Trinh A, Fahey MC, Brown J, Fuller PJ, Milat F. Optimizing bone health in cerebral palsy across the lifespan. Dev Med Child Neurol. (2017) 59:232–3. doi: 10.1111/dmcn.13355
58. Hasler C, Brunner R, Grundshtein A, Ovadia D. Spine deformities in patients with cerebral palsy; the role of the pelvis. J Child Orthopaedics. (2020) 14:9–16. doi: 10.1302/1863-2548.14.190141
59. Helenius IJ, Viehweger E, Castelein RM. Cerebral palsy with dislocated hip and scoliosis: what to deal with first? J Child Orthopaedics. (2020) 14:24–9. doi: 10.1302/1863-2548.14.190099
60. Pagnozzi AM, Dowson N, Doecke J, Fiori S, Bradley AP, Boyd RN, et al. Automated, quantitative measures of grey and white matter lesion burden correlates with motor and cognitive function in children with unilateral cerebral palsy. NeuroImage: Clin. (2016) 11:751–9. doi: 10.1016/j.nicl.2016.05.018
61. Wu YW, Croen LA, Shah SJ, Newman TB, Najjar DV. Cerebral Palsy in a Term Population: Risk Factors and Neuroimaging Findings. Pediatrics. (2006) 118:690. doi: 10.1542/peds.2006-0278
62. Rosenbaum PL, Walter SD, Hanna SE, Palisano RJ, Russell DJ, Raina P, et al. Prognosis for gross motor function in cerebral palsy: creation of motor development curves. JAMA. (2002) 288:1357–63. doi: 10.1001/jama.288.11.1357
63. Carlsson M, Hagberg G, Olsson I. Clinical and aetiological aspects of epilepsy in children with cerebral palsy. Dev Med Child Neurol. (2003) 45:371–6. doi: 10.1111/j.1469-8749.2003.tb00415.x
64. Carlsson M, Olsson I, Hagberg G, Beckung E. Behaviour in children with cerebral palsy with and without epilepsy. Dev Med Child Neurol. (2008) 50:784–9. doi: 10.1111/j.1469-8749.2008.03090.x
65. Jethwa A, Mink J, Macarthur C, Knights S, Fehlings T, Fehlings D. Development of the Hypertonia Assessment Tool (HAT): a discriminative tool for hypertonia in children. Dev Med Child Neurol. (2010) 52:e83–7. doi: 10.1111/j.1469-8749.2009.03483.x
66. Fehlings D, Brown L, Harvey A, Himmelmann K, Lin J-P, Macintosh A, et al. Pharmacological and neurosurgical interventions for managing dystonia in cerebral palsy: a systematic review. Dev Med Child Neurol. (2018) 60:356–66. doi: 10.1111/dmcn.13652
67. Lumsden DE, Crowe B, Basu A, Amin S, Devlin A, DeAlwis Y, et al. Pharmacological management of abnormal tone and movement in cerebral palsy. Arch Dis Child. (2019) 104:775. doi: 10.1136/archdischild-2018-316309
68. Weir FW, Hatch JL, McRackan TR, Wallace SA, Meyer TA. Hearing loss in pediatric patients with cerebral palsy. Otol Neurotol. (2018) 39:59–64. doi: 10.1097/MAO.0000000000001610
69. Fowler KB, McCollister FP, Sabo DL, Shoup AG, Owen KE, Woodruff JL, et al. A targeted approach for congenital cytomegalovirus screening within newborn hearing screening. Pediatrics. (2017) 139:2128. doi: 10.1542/peds.2016-2128
70. Deramore Denver B, Froude E, Rosenbaum P, Wilkes-Gillan S, Imms C. Measurement of visual ability in children with cerebral palsy: a systematic review. Dev Med Child Neurol. (2016) 58:1016–29. doi: 10.1111/dmcn.13139
71. Philip SS, Guzzetta A, Chorna O, Gole G, Boyd RN. Relationship between brain structure and Cerebral Visual Impairment in children with Cerebral Palsy: A systematic review. Res Dev Disabil. (2020) 99:103580. doi: 10.1016/j.ridd.2020.103580
72. Mercuri E, Atkinson J, Braddick O, Anker S, Cowan F, Rutherford M, et al. Basal ganglia damage and impaired visual function in the newborn infant. Arch Dis Childhood-Fetal Neonatal Edition. (1997) 77:F111–4. doi: 10.1136/fn.77.2.F111
73. Mercuri E, Anker S, Guzzetta A, Barnett A, Haataja L, Rutherford M, et al. Visual function at school age in children with neonatal encephalopathy and low Apgar scores. Arch Dis Childhood-Fetal Neonatal Edition. (2004) 89:F258–62. doi: 10.1136/adc.2002.025387
74. Boel L, Pernet K, Toussaint M, Ides K, Leemans G, Haan J, et al. Respiratory morbidity in children with cerebral palsy: an overview. Dev Med Child Neurol. (2019) 61:646–53. doi: 10.1111/dmcn.14060
75. Durufle-Tapin A, Colin A, Nicolas B, Lebreton C, Dauvergne F, Gallien P. Analysis of the medical causes of death in cerebral palsy. Ann Phys Rehabil Med. (2014) 57:24–37. doi: 10.1016/j.rehab.2013.11.002
76. Reid SM, Carlin JB, Reddihough DS. Survival of individuals with cerebral palsy born in Victoria, Australia, between 1970 and 2004. Dev Med Child Neurol. (2012) 54:353–60. doi: 10.1111/j.1469-8749.2012.04218.x
77. Blackmore AM, Bear N, Blair E, Gibson N, Jalla C, Langdon K, et al. Prevalence of symptoms associated with respiratory illness in children and young people with cerebral palsy. Dev Med Child Neurol. (2016) 58:780–1. doi: 10.1111/dmcn.13016
78. Meehan EM, Reid SM, Williams KJ, Freed GL, Sewell JR, Reddihough DS. Medical service use in children with cerebral palsy: The role of child and family factors characteristics. J Paediatr Child Health. (2016) 52:621–7. doi: 10.1111/jpc.13163
79. Blackmore AM, Bear N, Blair E, Langdon K, Moshovis L, Steer K, et al. Predicting respiratory hospital admissions in young people with cerebral palsy. Arch Dis Child. (2018) 103:1119–24. doi: 10.1136/archdischild-2017-314346
80. Blackmore AM, Bear N, Blair E, Gibson N, Jalla C, Langdon K, et al. Factors associated with respiratory illness in children and young adults with cerebral palsy. J Pediatrics. (2016) 168:151–7.e1. doi: 10.1016/j.jpeds.2015.09.064
81. Himmelmann K. Putting prevention into practice for the benefit of children and young people with cerebral palsy. Arch Dis Child. (2018) 103:1100. doi: 10.1136/archdischild-2018-315134
82. Seddon PC, Khan Y. Respiratory problems in children with neurological impairment. Arch Dis Child. (2003) 88:75–8. doi: 10.1136/adc.88.1.75
83. Erasmus CE, van Hulst K, Rotteveel JJ, Willemsen MAAP, Jongerius PH. Clinical practice: swallowing problems in cerebral palsy. Eur J Pediatr. (2012) 171:409–14. doi: 10.1007/s00431-011-1570-y
84. Parkes J, Hill N, Platt MJ, Donnelly C. Oromotor dysfunction and communication impairments in children with cerebral palsy: a register study. Dev Med Child Neurol. (2010) 52:1113–9. doi: 10.1111/j.1469-8749.2010.03765.x
85. Calis EA, Veugelers R, Sheppard JJ, Tibboel D, Evenhuis HM, Penning C. Dysphagia in children with severe generalized cerebral palsy and intellectual disability. Dev Med Child Neurol. (2008) 50:625–30. doi: 10.1111/j.1469-8749.2008.03047.x
86. Mirrett PL, Riski JE, Glascott J, Johnson V. Videofluoroscopic assessment of dysphagia in children with severe spastic cerebral palsy. Dysphagia. (1994) 9:174–9. doi: 10.1007/BF00341262
87. Serel Arslan S, Demir N, Karaduman AA. Both pharyngeal and esophageal phases of swallowing are associated with recurrent pneumonia in pediatric patients. Clin Respir J. (2018) 12:767–71. doi: 10.1111/crj.12592
88. Mandell LA, Niederman MS. Aspiration pneumonia. N Engl J Med. (2019) 380:651–63. doi: 10.1056/NEJMra1714562
89. Duvallet C, Larson K, Snapper S, Iosim S, Lee A, Freer K, et al. Aerodigestive sampling reveals altered microbial exchange between lung, oropharyngeal, and gastric microbiomes in children with impaired swallow function. PLoS ONE. (2019) 14:e0216453. doi: 10.1371/journal.pone.0216453
90. Sacco O, Silvestri M, Sabatini F, Sale R, Moscato G, Pignatti P, et al. IL-8 and airway neutrophilia in children with gastroesophageal reflux and asthma-like symptoms. Respir Med. (2006) 100:307–15. doi: 10.1016/j.rmed.2005.05.011
91. Theodoropoulos DS, Pecoraro DL, Efstratiadis SE. The association of gastroesophageal reflux disease with asthma and chronic cough in the adult. Am J Respir Med. (2002) 1:133–46. doi: 10.1007/BF03256602
92. Hunt EB, Sullivan A, Galvin J, MacSharry J, Murphy DM. Gastric aspiration and its role in airway inflammation. Open Respir Med J. (2018) 12:1–10. doi: 10.2174/1874306401812010001
93. Thorburn K, Jardine M, Taylor N, Reilly N, Sarginson RE, van Saene HKF. Antibiotic-resistant bacteria and infection in children with cerebral palsy requiring mechanical ventilation. Pediatr Crit Care Med. (2009) 10:2. doi: 10.1097/PCC.0b013e31819368ac
94. Fitzgerald DA, Follett J, Van Asperen PP. Assessing and managing lung disease and sleep disordered breathing in children with cerebral palsy. Paediatr Respir Rev. (2009) 10:18–24. doi: 10.1016/j.prrv.2008.10.003
95. Weir KA, McMahon S, Taylor S, Chang AB. Oropharyngeal aspiration and silent aspiration in children. Chest. (2011) 140:589–97. doi: 10.1378/chest.10-1618
96. Størdal K, Johannesdottir G, Bentsen B, Knudsen P, Carlsen K, Closs O, et al. Acid suppression does not change respiratory symptoms in children with asthma and gastro-oesophageal reflux disease. Arch Dis Child. (2005) 90:956–60. doi: 10.1136/adc.2004.068890
97. Ummarino D, Miele E, Masi P, Tramontano A, Staiano A, Vandenplas Y. Impact of antisecretory treatment on respiratory symptoms of gastroesophageal reflux disease in children. Dis Esophagus. (2012) 25:671–7. doi: 10.1111/j.1442-2050.2011.01301.x
98. Knatten CK, Kvello M, Fyhn TJ, Edwin B, Schistad O, Aabakken L, et al. Nissen fundoplication in children with and without neurological impairment: A prospective cohort study. J Pediatr Surg. (2016) 51:1115–21. doi: 10.1016/j.jpedsurg.2015.12.007
99. Heinrich M, Kain A, Bergmann F, von Schweinitz D. Parents reported reduced symptoms and improved satisfaction after fundoplication and their perceptions were an important outcome measure. Acta Paediatr. (2017) 106:168–73. doi: 10.1111/apa.13621
100. Lee SL, Shabatian H, Hsu J-W, Applebaum H, Haigh PI. Hospital admissions for respiratory symptoms and failure to thrive before and after Nissen fundoplication. J Pediatr Surg. (2008) 43:59–65. doi: 10.1016/j.jpedsurg.2007.09.020
101. Lauriti G, Lisi G, Lelli Chiesa P, Zani A, Pierro A. Gastroesophageal reflux in children with neurological impairment: a systematic review and meta-analysis. Pediatr Surg Int. (2018) 34:1139–49. doi: 10.1007/s00383-018-4335-0
102. Equi A, Balfour-Lynn I, Bush A, Rosenthal M. Long term azithromycin in children with cystic fibrosis: a randomised, placebo-controlled crossover trial. Lancet. (2002) 360:978–84. doi: 10.1016/S0140-6736(02)11081-6
103. Pasteur MC, Bilton D, Hill AT. British Thoracic Society guideline for non-CFbronchiectasis. Thorax. (2010) 65:i1. doi: 10.1136/thx.2010.136119
104. Abusamra R, Ross Russell R. Management of respiratory disease in children with muscular weakness. Paediatr Child Health. (2015) 25:515–21. doi: 10.1016/j.paed.2015.06.011
105. Horwood L, Li P, Mok E, Shevell M, Constantin E A. systematic review and meta-analysis of the prevalence of sleep problems in children with cerebral palsy: how do children with cerebral palsy differ from each other and from typically developing children? Sleep Health. (2019) 5:555–71. doi: 10.1016/j.sleh.2019.08.006
106. Munyumu K, Idro R, Abbo C, Kaddumukasa M, Katabira E, Mupere E, et al. Prevalence and factors associated with sleep disorders among children with cerebral palsy in Uganda; a cross-sectional study. BMC Pediatr. (2018) 18:26. doi: 10.1186/s12887-018-0996-z
107. Sandella DE, O'Brien LM, Shank LK, Warschausky SA. Sleep and quality of life in children with cerebral palsy. Sleep Med. (2011) 12:252–6. doi: 10.1016/j.sleep.2010.07.019
108. Hayashi M, Inoue Y, Iwakawa Y, Sasaki H, REM. sleep abnormalities in severe athetoid cerebral palsy. Brain Dev. (1990) 12:494–7. doi: 10.1016/S0387-7604(12)80214-2
109. Leger D, Prevot E, Philip P, Yence C, Labaye N, Paillard M, et al. Sleep disorders in children with blindness. Ann Neurol. (1999) 46:648–51. doi: 10.1002/1531-8249(199910)46:4<648::AID-ANA14>3.0.CO;2-X
110. Mindell JA, De Marco CM. Sleep problems of young blind children. J Visual Impairment Blindness. (1997) 91:106. doi: 10.1177/0145482X9709100106
111. Quine L. Sleep problems in children with mental handicap. J Ment Defic Res. (1991) 35:269–90. doi: 10.1111/j.1365-2788.1991.tb00402.x
112. Tröster H, Brambring M. Sleep disorders in visually handicapped children in infancy and preschool age. Prax Kinderpsychol Kinderpsychiatr. (1995) 44:36–44.
113. Hemmingsson H, Stenhammar A-M, Paulsson K. Sleep problems and the need for parental night-time attention in children with physical disabilities. Child Care Health Dev. (2009) 35:89–95. doi: 10.1111/j.1365-2214.2008.00907.x
114. Bruni O, Ottaviano S, Guidetti V, Romoli M, Innocenzi M, Cortesi F, et al. The Sleep Disturbance Scale for Children (SDSC) construction and validation of an instrument to evaluate sleep disturbances in childhood and adolescence. J Sleep Res. (1996) 5:251–61. doi: 10.1111/j.1365-2869.1996.00251.x
115. Romeo DM, Bruni O, Brogna C, Ferri R, Galluccio C, De Clemente V, et al. Application of the Sleep Disturbance Scale for Children (SDSC) in preschool age. Eur J Paediatr Neurol. (2013) 17:374–82. doi: 10.1016/j.ejpn.2012.12.009
116. Chervin RD, Hedger K, Dillon JE, Pituch KJ. Pediatric sleep questionnaire (PSQ): validity and reliability of scales for sleep-disordered breathing, snoring, sleepiness, and behavioral problems. Sleep Med. (2000) 1:21–32. doi: 10.1016/S1389-9457(99)00009-X
117. Goodlin-Jones BL, Sitnick SL, Tang K, Liu J, Anders TF. The Children's sleep habits questionnaire in toddlers and preschool children. J Dev Behav Pediatr. (2008) 29:82–8. doi: 10.1097/DBP.0b013e318163c39a
118. Owens JA, Spirito A, McGuinn M. The Children's Sleep Habits Questionnaire (CSHQ): psychometric properties of a survey instrument for school-aged children. Sleep-NY. (2000) 23:1043–52. doi: 10.1093/sleep/23.8.1d
119. Zuculo GM, Knap CCF, Pinato L. Correlation between sleep and quality of life in cerebral palsy. CoDAS. (2014) 26:447–56. doi: 10.1590/2317-1782/20140201435
120. Davis E, Shelly A, Waters E, Boyd R, Cook K, Davern M. The impact of caring for a child with cerebral palsy: quality of life for mothers and fathers. Child Care Health Dev. (2010) 36:63–73. doi: 10.1111/j.1365-2214.2009.00989.x
121. Wayte S, McCaughey E, Holley S, Annaz D, Hill CM. Sleep problems in children with cerebral palsy and their relationship with maternal sleep and depression. Acta Paediatr. (2012) 101:618–23. doi: 10.1111/j.1651-2227.2012.02603.x
122. Jan JE, Owens JA, Weiss MD, Johnson KP, Wasdell MB, Freeman RD, et al. Sleep hygiene for children with neurodevelopmental disabilities. Pediatrics. (2008) 122:1343–50. doi: 10.1542/peds.2007-3308
123. Allen SL, Howlett MD, Coulombe JA, Corkum PV. ABCs of SLEEPING: A review of the evidence behind pediatric sleep practice recommendations. Sleep Med Rev. (2016) 29:1–14. doi: 10.1016/j.smrv.2015.08.006
124. Fehr KK, Russ SW, Ievers-Landis CE. Treatment of sleep problems in young children: A case series report of a cognitive-behavioral play intervention. Clin Pract Pediatr Psychol. (2016) 4:306. doi: 10.1037/cpp0000153
125. Macchi MM, Bruce JN. Human pineal physiology and functional significance of melatonin. Front Neuroendocrinol. (2004) 25:177–95. doi: 10.1016/j.yfrne.2004.08.001
126. Ding X, Sun B, Huang J, Xu L, Pan J, Fang C, et al. The role of miR-182 in regulating pineal CLOCK expression after hypoxia-ischemia brain injury in neonatal rats. Neurosci Lett. (2015) 591:75–80. doi: 10.1016/j.neulet.2015.02.026
127. Ding X, Cheng Z, Sun B, Huang J, Wang L, Han X, et al. Distinctive sleep problems in children with perinatal moderate or mild hypoxic-ischemia. Neurosci Lett. (2016) 614:60–4. doi: 10.1016/j.neulet.2015.12.061
128. Santos JS, Giacheti CM, Dornelas LS, Silva NC, Souza ALDM, Guissoni Campos LM, et al. Day/night melatonin content in cerebral palsy. Neurosci Lett. (2018) 686:23–7. doi: 10.1016/j.neulet.2018.08.045
129. Dodge NN, Wilson GA. Melatonin for treatment of sleep disorders in children with developmental disabilities. J Child Neurol. (2001) 16:581–4. doi: 10.1177/088307380101600808
130. Ross C, Davies P, Whitehouse W. Melatonin treatment for sleep disorders in children with neurodevelopmental disorders: an observational study. Dev Med Child Neurol. (2002) 44:339–44. doi: 10.1111/j.1469-8749.2002.tb00821.x
131. Kotagal S, Gibbons VP, Stith JA. Sleep abnormalities in patients with severe cerebral palsy. Dev Med Child Neurol. (1994) 36:304–11. doi: 10.1111/j.1469-8749.1994.tb11850.x
132. Garcia J, Wical B, Wical W, Schaffer L, Wical T, Wendorf H, et al. Obstructive sleep apnea in children with cerebral palsy and epilepsy. Dev Med Child Neurol. (2016) 58:1057–62. doi: 10.1111/dmcn.13091
133. Kritzinger FE, Al-Saleh S, Narang I. Descriptive analysis of central sleep apnea in childhood at a single center. Pediatr Pulmonol. (2011) 46:1023–30. doi: 10.1002/ppul.21469
134. Bennett S, Siritaratiwat W, Tanrangka N, Bennett MJ, Kanpittaya J. Diaphragmatic mobility in children with spastic cerebral palsy and differing motor performance levels. Respir Physiol Neurobiol. (2019) 266:163–70. doi: 10.1016/j.resp.2019.05.010
135. Angriman M, Caravale B, Novelli L, Ferri R, Bruni O. Sleep in children with neurodevelopmental disabilities. Neuropediatrics. (2015) 46:199–210. doi: 10.1055/s-0035-1550151
136. Marcus CL, Brooks LJ, Ward SD, Draper KA, Gozal D, Halbower AC, et al. Diagnosis and management of childhood obstructive sleep apnea syndrome. Pediatrics. (2012) 130:e714–e55. doi: 10.1542/peds.2012-1672
137. Okur EO, Inal-Ince D, Saglam M, Vardar-Yagli N, Arikan H. Physical activity patterns in children with cerebral palsy and typically developing peers. Physiother Theory Pract. (2019) 2019:1–9. doi: 10.1080/09593985.2019.1641863
138. Mueller UM, Walther C, Adam J, Fikenzer K, Erbs S, Mende M, et al. Endothelial function in children and adolescents is mainly influenced by age, sex and physical activity-an analysis of reactive hyperemic peripheral artery tonometry. Circ J. (2017) 81:717–25. doi: 10.1253/circj.CJ-16-0994
139. Pahkala K, Heinonen OJ, Simell O, Viikari JS, Rönnemaa T, Niinikoski H, et al. Association of physical activity with vascular endothelial function and intima-media thickness. Circulation. (2011) 124:1956–63. doi: 10.1161/CIRCULATIONAHA.111.043851
140. Trigona B, Aggoun Y, Maggio A, Martin XE, Marchand LM, Beghetti M, et al. Preclinical noninvasive markers of atherosclerosis in children and adolescents with type 1 diabetes are influenced by physical activity. J Pediatr. (2010) 157:533–9. doi: 10.1016/j.jpeds.2010.04.023
141. Cece H, Yetisgin A, Abuhandan M, Yildiz S, Calik M, Karakas O, et al. Evaluation of carotid intima-media thickness, a marker of subclinical atherosclerosis, in children with cerebral palsy. Pediatr Radiol. (2012) 42:679–84. doi: 10.1007/s00247-012-2361-y
142. Gazolla FM, Bordallo MAN, Madeira IR, de Miranda Carvalho CN, Monteiro AMV, Rodrigues NCP, et al. Association between cardiovascular risk factors and carotid intima-media thickness in prepubertal Brazilian children. J Pediatr Endocrinol Metab. (2015) 28:579–87. doi: 10.1515/jpem-2014-0116
143. Katz-Leurer M, Amichai T. Heart rate variability in children with cerebral palsy. Dev Med Child Neurol. (2019) 61:730–1. doi: 10.1111/dmcn.14095
144. Kholod H, Jamil A, Katz-Leurer M. The associations between motor ability, walking activity and heart rate and heart rate variability parameters among children with cerebral palsy and typically developed controls. NeuroRehabilitation. (2013) 33:113–9. doi: 10.3233/NRE-130934
145. Zamunér AR, Cunha AB, da Silva E, Negri AP, Tudella E, Moreno MA. The influence of motor impairment on autonomic heart rate modulation among children with cerebral palsy. Res Dev Disabil. (2011) 32:217–21. doi: 10.1016/j.ridd.2010.09.020
146. Nelson KB, Dambrosia JM, Ting TY. Grether JK. Uncertain value of electronic fetal monitoring in predicting cerebral palsy. N Engl J Med. (1996) 334:613. doi: 10.1056/NEJM199603073341001
147. Einspieler C, Prechtl HF. Prechtl's assessment of general movements: a diagnostic tool for the functional assessment of the young nervous system. Ment Retard Dev Disabil Res Rev. (2005) 11:61–7. doi: 10.1002/mrdd.20051
148. Dimitrijević L, Bjelaković B, Colović H, Mikov A, Živković V, Kocić M, et al. Assessment of general movements and heart rate variability in prediction of neurodevelopmental outcome in preterm infants. Early Hum Dev. (2016) 99:7–12. doi: 10.1016/j.earlhumdev.2016.05.014
149. O'Dea M, Sweetman D, Bonifacio SL, El-Dib M, Austin T, Molloy EJ. Management of multi organ dysfunction in neonatal encephalopathy. Front Pediatr. (2020) 8:239. doi: 10.3389/fped.2020.00239
150. Martín-Ancel A, García-Alix A, Gayá F, Cabañas F, Burgueros M, Quero J. Multiple organ involvement in perinatal asphyxia. J Pediatr. (1995) 127:786–93. doi: 10.1016/S0022-3476(95)70174-5
151. Hankins GD, Koen S, Gei AF, Lopez SM, Van Hook JW, Anderson GD. Neonatal organ system injury in acute birth asphyxia sufficient to result in neonatal encephalopathy. Obstet Gynecol. (2002) 99:688–91. doi: 10.1097/00006250-200205000-00004
152. Shah P, Riphagen S, Beyene J, Perlman M. Multiorgan dysfunction in infants with post-asphyxial hypoxic-ischaemic encephalopathy. Arch Dis Childhood-Fetal Neonatal Edition. (2004) 89:F152–5. doi: 10.1136/adc.2002.023093
153. Shankaran S, Woldt E, Koepke T, Bedard MP, Nandyal R. Acute neonatal morbidity and long-term central nervous system sequelae of perinatal asphyxia in term infants. Early Hum Dev. (1991) 25:135–48. doi: 10.1016/0378-3782(91)90191-5
154. Çelik SF, Baratali E, Güven AS, Torun YA. Left ventricular myocardial deformation abnormalities in seizure-free children with epilepsy. Seizure. (2018) 61:153–7. doi: 10.1016/j.seizure.2018.08.017
155. Krishnamoorthy V, Chaikittisilpa N, Lee J, Mackensen GB, Gibbons EF, Laskowitz D, et al. Speckle tracking analysis of left ventricular systolic function following traumatic brain injury: a pilot prospective observational cohort study. J Neurosurg Anesthesiol. (2020) 32:156–61. doi: 10.1097/ANA.0000000000000578
156. Krishnamoorthy V, Mackensen GB, Gibbons EF, Vavilala MS. Cardiac dysfunction after neurologic injury: what do we know and where are we going? Chest. (2016) 149:1325–31. doi: 10.1016/j.chest.2015.12.014
157. Corella Aznar EG, Ayerza Casas A, Jiménez Montañés L, Calvo Escribano MÁ C, Labarta Aizpún JI, Samper Villagrasa P. Use of speckle tracking in the evaluation of late subclinical myocardial damage in survivors of childhood acute leukaemia. Int J Cardiovasc Imaging. (2018) 34:1373–81. doi: 10.1007/s10554-018-1346-9
158. Joseph S, Kumar S, Ahamed MZ, Lakshmi S. Cardiac Troponin-T as a marker of myocardial dysfunction in term neonates with perinatal asphyxia. Indian J Pediatr. (2018) 85:877–84. doi: 10.1007/s12098-018-2667-3
159. Rinat C, Becker-Cohen R, Nir A, Feinstein S, Algur N, Ben-Shalom E, et al. B-type natriuretic peptides are reliable markers of cardiac strain in CKD pediatric patients. Pediatr Nephrol. (2012) 27:617–25. doi: 10.1007/s00467-011-2025-4
160. Sweetman DU, Kelly L, Hurley T, Onwuneme C, Watson RWG, Murphy JFA, et al. Troponin T correlates with MRI results in neonatal encephalopathy. Acta Paediatr. (2020) 109:2266–70. doi: 10.1111/apa.15255
161. Beaufils J, Olivari-Philiponnet C, Honoré T, Heyman R, Bonan I, Rauscent H. Impact of lower urinary tract dysfunction and anorectal disorders on quality of life in children with cerebral palsy. Ann Phys Rehabil Med. (2018) 61:e321–e2. doi: 10.1016/j.rehab.2018.05.750
162. Schast AP, Zderic SA, Richter M, Berry A, Carr MC. Quantifying demographic, urological and behavioral characteristics of children with lower urinary tract symptoms. J Pediatr Urol. (2008) 4:127–33. doi: 10.1016/j.jpurol.2007.10.007
163. Marciniak C, O'Shea SA, Lee J, Jesselson M, Dudas-Sheehan D, Beltran E, et al. Urinary incontinence in adults with cerebral palsy: prevalence, type, and effects on participation. PMR. (2014) 6:110–20. doi: 10.1016/j.pmrj.2013.07.012
164. Singh BK, Masey H, Morton R. Levels of continence in children with cerebral palsy. Paediatr Nurs. (2006) 18:23–6. doi: 10.7748/paed.18.4.23.s17
165. Wright AJ, Fletcher O, Scrutton D, Baird G. Bladder and bowel continence in bilateral cerebral palsy: A population study. J Pediatr Urol. (2016). 12:383.e1–8. doi: 10.1016/j.jpurol.2016.05.027
166. Ozturk M, Oktem F, Kisioglu N, Demirci M, Altuntas I, Kutluhan S, et al. Bladder and bowel control in children with cerebral palsy: case-control study. Croat Med J. (2006) 47:264–70.
167. Reid CJ, Borzyskowski M. Lower urinary tract dysfunction in cerebral palsy. Arch Dis Child. (1993) 68:739–42. doi: 10.1136/adc.68.6.739
168. Karaman MI, Kaya C, Caskurlu T, Guney S, Ergenekon E. Urodynamic findings in children with cerebral palsy. Int J Urol. (2005) 12:717–20. doi: 10.1111/j.1442-2042.2005.01120.x
169. Silva JA, Alvares RA, Barboza AL, Monteiro RT. Lower urinary tract dysfunction in children with cerebral palsy. Neurourol Urodyn. (2009) 28:959–63. doi: 10.1002/nau.20714
170. Bross S, Honeck P, Kwon ST, Badawi JK, Trojan L, Alken P. Correlation between motor function and lower urinary tract dysfunction in patients with infantile cerebral palsy. Neurourol Urodyn. (2007) 26:222–7. doi: 10.1002/nau.20329
171. Roijen LE, Postema K, Limbeek VJ, Kuppevelt VH. Development of bladder control in children and adolescents with cerebral palsy. Dev Med Child Neurol. (2001) 43:103–7. doi: 10.1017/S0012162201000172
172. Van Laecke E, Golinveaux LUC, Goossens LUC, Raes ANN, Hoebeke P, Vande Walle J, et al. Children. J Urol. (2001) 166:2404–6. doi: 10.1016/S0022-5347(05)65602-9
173. Gündogdu G, Kömür M, Avlan D, Sari FB, Delibaş A, Taşdelen B, et al. Relationship of bladder dysfunction with upper urinary tract deterioration in cerebral palsy. J Pediatr Urol. (2013) 9:659–64. doi: 10.1016/j.jpurol.2012.07.020
174. Cotter KJ, Levy ME, Goldfarb RA, Liberman D, Katorski J, Myers JB, et al. Urodynamic findings in adults with moderate to severe cerebral palsy. Urology. (2016) 95:216–21. doi: 10.1016/j.urology.2016.05.024
175. Decter RM, Bauer SB, Khoshbin S, Dyro FM, Krarup C, Colodny AH, et al. Urodynamic assessment of children with cerebral palsy. J Urol. (1987) 138:1110–2. doi: 10.1016/S0022-5347(17)43518-X
176. Veugelers R, Benninga MA, Calis EA, Willemsen SP, Evenhuis H, Tibboel D, et al. Prevalence and clinical presentation of constipation in children with severe generalized cerebral palsy. Dev Med Child Neurol. (2010) 52:e216–21. doi: 10.1111/j.1469-8749.2010.03701.x
177. Krogh K, Christensen P. Laurberg S. Colorectal symptoms in patients with neurological diseases. Acta Neurol Scand. (2001) 103:335–43. doi: 10.1034/j.1600-0404.2001.103006335.x
178. Shaikh N, Hoberman A, Keren R, Gotman N, Docimo SG, Mathews R, et al. Recurrent urinary tract infections in children with bladder and bowel dysfunction. Pediatrics. (2016) 137:7. doi: 10.1542/peds.2015-2982
179. Sureshkumar P, Jones M, Cumming RG, Craig JC. Risk factors for urinary tract infection in children: a population-based study of 2856 children. J Paediatr Child Health. (2009) 45:87–97. doi: 10.1111/j.1440-1754.2008.01435.x
180. Garne E, Dolk H, Krägeloh-Mann I, Holst Ravn S, Cans C. Cerebral palsy and congenital malformations. Eur J Paediatr Neurol. (2008) 12:82–8. doi: 10.1016/j.ejpn.2007.07.001
181. LaRosa DA, Ellery SJ, Walker DW, Dickinson H. Understanding the full spectrum of organ injury following intrapartum asphyxia. Front Pediatr. (2017) 5:16. doi: 10.3389/fped.2017.00016
182. Aggarwal A, Kumar P, Chowdhary G, Majumdar S, Narang A. Evaluation of renal functions in asphyxiated newborns. J Trop Pediatr. (2005) 51:295–9. doi: 10.1093/tropej/fmi017
183. Nouri S, Mahdhaoui N, Beizig S, Zakhama R, Salem N, Ben Dhafer S, et al. [Acute renal failure in full term neonates with perinatal asphyxia. Prospective study of 87 cases]. Arch Pediatr. (2008) 15:229–35. doi: 10.1016/j.arcped.2008.01.011
184. Perlman JM. Systemic abnormalities in term infants following perinatal asphyxia: relevance to long-term neurologic outcome. Clin Perinatol. (1989) 16:475–84. doi: 10.1016/S0095-5108(18)30642-0
185. Sweetman DU, Onwuneme C, Watson WR, O'Neill A, Murphy JFA, Molloy EJ. Renal function and novel urinary biomarkers in infants with neonatal encephalopathy. Acta Paediatr. (2016) 105:e513–9. doi: 10.1111/apa.13555
186. Sweetman DU, Riordan M, Molloy EJ. Management of renal dysfunction following term perinatal hypoxia-ischaemia. Acta Paediatr. (2013) 102:233–41. doi: 10.1111/apa.12116
187. Carmody JB, Charlton JR. Short-term gestation, long-term risk: prematurity and chronic kidney disease. Pediatrics. (2013) 131:1168–79. doi: 10.1542/peds.2013-0009
188. Mammen C, Al Abbas A, Skippen P, Nadel H, Levine D, Collet JP, et al. Long-term risk of CKD in children surviving episodes of acute kidney injury in the intensive care unit: a prospective cohort study. Am J Kidney Dis. (2012) 59:523–30. doi: 10.1053/j.ajkd.2011.10.048
189. Shin YK, Yoon YK, Chung KB, Rhee Y, Cho SR. Patients with non-ambulatory cerebral palsy have higher sclerostin levels and lower bone mineral density than patients with ambulatory cerebral palsy. Bone. (2017) 103:302–7. doi: 10.1016/j.bone.2017.07.015
190. Henderson RC, Lin PP, Greene WB. Bone-mineral density in children and adolescents who have spastic cerebral palsy. J Bone Joint Surg Am. (1995) 77:1671–81. doi: 10.2106/00004623-199511000-00005
191. Huh JH, Choi SI, Lim JS, Chung CH, Shin JY, Lee MY. Lower serum creatinine is associated with low bone mineral density in subjects without overt nephropathy. PLoS ONE. (2015) 10:133062. doi: 10.1371/journal.pone.0133062
192. Ohata K, Tsuboyama T, Haruta T, Ichihashi N, Kato T, Nakamura T. Relation between muscle thickness, spasticity, and activity limitations in children and adolescents with cerebral palsy. Dev Med Child Neurol. (2008) 50:152–6. doi: 10.1111/j.1469-8749.2007.02018.x
193. Dennen P, Altmann C, Kaufman J, Klein CL, Andres-Hernando A, Ahuja NH, et al. Urine interleukin-6 is an early biomarker of acute kidney injury in children undergoing cardiac surgery. Criti Care. (2010) 14:R181. doi: 10.1186/cc9289
194. Lagos-Arevalo P, Palijan A, Vertullo L, Devarajan P, Bennett MR, Sabbisetti V, et al. Cystatin C in acute kidney injury diagnosis: early biomarker or alternative to serum creatinine? Pediatr Nephrol. (2015) 30:665–76. doi: 10.1007/s00467-014-2987-0
195. Nguyen MT, Devarajan P. Biomarkers for the early detection of acute kidney injury. Pediatr Nephrol. (2008) 23:2151. doi: 10.1007/s00467-007-0470-x
196. Seitz S, Rauh M, Gloeckler M, Cesnjevar R, Dittrich S, Koch AM. Cystatin C and neutrophil gelatinase-associated lipocalin: biomarkers for acute kidney injury after congenital heart surgery. Swiss Med Weekly. (2013) 143:304. doi: 10.4414/smw.2013.13744
197. Russo D, Battaglia Y. Clinical Significance of FGF-23 in Patients with CKD. Int J Nephrol. (2011) 2011:364890. doi: 10.4061/2011/364890
198. Bennett M, Dent CL, Ma Q, Dastrala S, Grenier F, Workman R, et al. Urine NGAL predicts severity of acute kidney injury after cardiac surgery: a prospective study. Clin J Am Soc Nephrol. (2008) 3:665–73. doi: 10.2215/CJN.04010907
199. Krawczeski CD, Vandevoorde RG, Kathman T, Bennett MR, Woo JG, Wang Y, et al. Serum cystatin C is an early predictive biomarker of acute kidney injury after pediatric cardiopulmonary bypass. Clin J Am Soc Nephrol. (2010) 5:1552–7. doi: 10.2215/CJN.02040310
200. Mitsnefes MM, Kathman TS, Mishra J, Kartal J, Khoury PR, Nickolas TL, et al. Serum neutrophil gelatinase-associated lipocalin as a marker of renal function in children with chronic kidney disease. Pediatr Nephrol. (2007) 22:101–8. doi: 10.1007/s00467-006-0244-x
201. Del Giudice E, Staiano A, Capano G, Romano A, Florimonte L, Miele E, et al. Gastrointestinal manifestations in children with cerebral palsy. Brain Dev. (1999) 21:307–11. doi: 10.1016/S0387-7604(99)00025-X
202. Reilly S, Skuse D, Poblete X. Prevalence of feeding problems and oral motor dysfunction in children with cerebral palsy: a community survey. J Pediatr. (1996) 129:877–82. doi: 10.1016/S0022-3476(96)70032-X
203. Blasco PA, Allaire JH, Drooling in the developmental disabled. Management practices and recommendations. Dev Med Child Neurol. (1992) 34:849–62. doi: 10.1111/j.1469-8749.1992.tb11382.x
204. Walshe M, Smith M, Pennington L. Interventions for drooling in children with cerebral palsy. Cochrane Datab Syst Rev. (2012) 2012:CD008624. doi: 10.1002/14651858.CD008624.pub3
205. Reddihough D, Erasmus CE, Johnson H, McKellar GM, Jongerius PH. Botulinum toxin assessment, intervention and aftercare for paediatric and adult drooling: international consensus statement. Eur J Neurol. (2010) 17(Suppl. 2):109–21. doi: 10.1111/j.1468-1331.2010.03131.x
206. Tahmassebi JF, Curzon ME. The cause of drooling in children with cerebral palsy – hypersalivation or swallowing defect? Int J Paediatr Dent. (2003) 13:106–11. doi: 10.1046/j.1365-263X.2003.00439.x
207. van der Burg JJ, Jongerius PH, van Limbeek J, van Hulst K, Rotteveel JJ. Social interaction and self-esteem of children with cerebral palsy after treatment for severe drooling. Eur J Pediatr. (2006) 165:37–41. doi: 10.1007/s00431-005-1759-z
208. Heine RG, Catto-Smith AG, Reddihough DS. Effect of antireflux medication on salivary drooling in children with cerebral palsy. Dev Med Child Neurol. (1996) 38:1030–6. doi: 10.1111/j.1469-8749.1996.tb15063.x
209. McAloney N, Kerawala CJ, Stassen LF. Management of drooling by transposition of the submandibular ducts and excision of the sublingual glands. J Ir Dent Assoc. (2005) 51:126–31.
210. Crysdale WS, McCann C, Roske L, Joseph M, Semenuk D, Chait P. Saliva control issues in the neurologically challenged. A 30 year experience in team management. Int J Pediatr Otorhinolaryngol. (2006) 70:519–27. doi: 10.1016/j.ijporl.2005.07.021
211. Jongerius PH, van Tiel P, van Limbeek J, Gabreëls FJ, Rotteveel JJ A. systematic review for evidence of efficacy of anticholinergic drugs to treat drooling. Arch Dis Child. (2003) 88:911–4. doi: 10.1136/adc.88.10.911
212. Andretta M, Tregnaghi A, Prosenikliev V, Staffieri A. Current opinions in sialolithiasis diagnosis and treatment. Acta Otorhinolaryngol Ital. (2005) 25:145–9.
213. Burton MJ. The surgical management of drooling. Dev Med Child Neurol. (1991) 33:1110–6. doi: 10.1111/j.1469-8749.1991.tb14835.x
214. Sagar P, Handa KK, Gulati S, Kumar R. Submandibular Duct Re-routing for Drooling in Neurologically Impaired Children. Indian J Otolaryngol Head Neck Surg. (2016) 68:75–9. doi: 10.1007/s12070-015-0926-4
215. Fernando T, Goldman RD. Management of gastroesophageal reflux disease in pediatric patients with cerebral palsy. Can Fam Physician. (2019) 65:796–8.
216. Kim S, Koh H, Lee JS. Gastroesophageal reflux in neurologically impaired children: what are the risk factors? Gut Liver. (2017) 11:232. doi: 10.5009/gnl16150
217. Gössler A, Schalamon J, Huber-Zeyringer A, Höllwarth ME. Gastroesophageal reflux and behavior in neurologically impaired children. J Pediatr Surg. (2007) 42:1486–90. doi: 10.1016/j.jpedsurg.2007.04.009
218. Dahl M, Thommessen M, Rasmussen M, Selberg T. Feeding and nutritional characteristics in children with moderate or severe cerebral palsy. Acta Paediatr. (1996) 85:697–701. doi: 10.1111/j.1651-2227.1996.tb14129.x
219. Troughton KE, Hill AE. Relation between objectively measured feeding competence and nutrition in children with cerebral palsy. Dev Med Child Neurol. (2001) 43:187–90. doi: 10.1111/j.1469-8749.2001.tb00185.x
220. Reyes FI, Salemi JL, Dongarwar D, Magazine CB, Salihu HM. Prevalence, trends, and correlates of malnutrition among hospitalized children with cerebral palsy. Dev Med Child Neurol. (2019) 61:1432–8. doi: 10.1111/dmcn.14329
221. Campanozzi A, Capano G, Miele E, Romano A, Scuccimarra G, Del Giudice E, et al. Impact of malnutrition on gastrointestinal disorders and gross motor abilities in children with cerebral palsy. Brain Dev. (2007) 29:25–9. doi: 10.1016/j.braindev.2006.05.008
222. Tse P, Leung S, Chan T, Sien A, Chan A. Dietary fibre intake and constipation in children with severe developmental disabilities. J Paediatr Child Health. (2000) 36:236–9. doi: 10.1046/j.1440-1754.2000.00498.x
223. Romano C, van Wynckel M, Hulst J, Broekaert I, Bronsky J, Dall'Oglio L, et al. European Society for Paediatric Gastroenterology, Hepatology and Nutrition Guidelines for the evaluation and treatment of gastrointestinal and nutritional complications in children with neurological impairment. J Pediatric Gastroenterol Nutr. (2017) 65:1646. doi: 10.1097/MPG.0000000000001646
224. Ostojic K, Paget S, Kyriagis M, Morrow A. Acute and Chronic Pain in Children and Adolescents With Cerebral Palsy: Prevalence, Interference, and Management. Arch Phys Med Rehabil. (2020) 101:213–9. doi: 10.1016/j.apmr.2019.08.475
225. Blankenburg M, Junker J, Hirschfeld G, Michel E, Aksu F, Wager J, et al. Quantitative sensory testing profiles in children, adolescents and young adults (6-20 years) with cerebral palsy: Hints for a neuropathic genesis of pain syndromes. Eur J Paediatr Neurol. (2018) 22:470–81. doi: 10.1016/j.ejpn.2017.12.015
226. Jensen TS, Finnerup NB. Allodynia and hyperalgesia in neuropathic pain: clinical manifestations and mechanisms. Lancet Neurol. (2014) 13:924–35. doi: 10.1016/S1474-4422(14)70102-4
227. Collins A, Mannion R, Broderick A, Hussey S, Devins M, Bourke B. Gabapentin for the treatment of pain manifestations in children with severe neurological impairment: a single-centre retrospective review. BMJ Paediatr Open. (2019) 3:e000467. doi: 10.1136/bmjpo-2019-000467
228. Hauer JM, Solodiuk JC. Gabapentin for management of recurrent pain in 22 nonverbal children with severe neurological impairment: a retrospective analysis. J Palliat Med. (2015) 18:453–6. doi: 10.1089/jpm.2014.0359
229. Wahid AM, Powell CV, Davies IH, Evans JA, Jenkins HR. Intestinal failure in children and young people with neurodisabling conditions. Arch Dis Child. (2017) 102:475. doi: 10.1136/archdischild-2016-311387
230. Mercuri E, Cowan F, Gupte G, Manning R, Laffan M, Rutherford M, et al. Prothrombotic Disorders and Abnormal Neurodevelopmental Outcome in Infants With Neonatal Cerebral Infarction. Pediatrics. (2001) 107:1400. doi: 10.1542/peds.107.6.1400
231. Kenet G, Sadetzki S, Murad H, Martinowitz U, Rosenberg N, Gitel S, et al. Factor V Leiden and antiphospholipid antibodies are significant risk factors for ischemic stroke in children. Stroke. (2000) 31:1283–8. doi: 10.1161/01.STR.31.6.1283
232. Golomb MR, MacGregor DL, Domi T, Armstrong DC, McCrindle BW, Mayank S, et al. Presumed pre-or perinatal arterial ischemic stroke: risk factors and outcomes. Ann Neurol. (2001) 50:163–8. doi: 10.1002/ana.1078
233. Nelson KB, Lynch JK. Stroke in newborn infants. Lancet Neurol. (2004) 3:150–8. doi: 10.1016/S1474-4422(04)00679-9
234. Sweetman D, Kelly LA, Zareen Z, Nolan B, Murphy J, Boylan G, et al. Coagulation profiles are associated with early clinical outcomes in neonatal encephalopathy. Front Pediatr. (2019) 7:399. doi: 10.3389/fped.2019.00399
235. Redline RW, O'Riordan MA. Placental lesions associated with cerebral palsy and neurologic impairment following term birth. Arch Pathol Lab Med. (2000) 124:1785–91. doi: 10.5858/2000-124-1785-PLAWCP
236. McDonald DG, Kelehan P, McMenamin JB, Gorman WA, Madden D, Tobbia IN, et al. Placental fetal thrombotic vasculopathy is associated with neonatal encephalopathy. Hum Pathol. (2004) 35:875–80. doi: 10.1016/j.humpath.2004.02.014
237. Leviton A, Dammann O. Coagulation, inflammation, and the risk of neonatal white matter damage. Pediatr Res. (2004) 55:541–5. doi: 10.1203/01.PDR.0000121197.24154.82
238. Kannan S, Meert KL, Mooney JF, Hillman-Wiseman C, Warrier I. Bleeding and coagulation changes during spinal fusion surgery: a comparison of neuromuscular and idiopathic scoliosis patients. Pediatr Crit Care Med. (2002) 3:364–9. doi: 10.1097/00130478-200210000-00007
239. Gesundheit B, Kirby M, Lau W, Koren G, Abdelhaleem M. Thrombocytopenia and megakaryocyte dysplasia: an adverse effect of valproic acid treatment. J Pediatr Hematol Oncol. (2002) 24:589–90. doi: 10.1097/00043426-200210000-00022
240. Chambers HG, Weinstein CH, Mubarak SJ, Wenger DR, Silva PD. The effect of valproic acid on blood loss in patients with cerebral palsy. J Pediatric Orthopaedics. (1999) 19:792. doi: 10.1097/01241398-199911000-00018
241. Casasin T, Allende A, Macià M, Güell R. Two episodes of carbamazepine-induced severe thrombocytopenia in the same child. Ann Pharmacother. (1992) 26:715–6. doi: 10.1177/106002809202600523
242. Stork CM, Marraffa JM, Ragosta K, Wojcik SM, Angelino KL. Elevated International Normalized Ratio associated with long-term azithromycin therapy in a child with cerebral palsy. Am J Health Syst Pharm. (2011) 68:1012–4. doi: 10.2146/ajhp100603
243. Almuneef AR, Almajwal A, Alam I, Abulmeaty M, Bader BA, Badr MF, et al. Malnutrition is common in children with cerebral palsy in Saudi Arabia - a cross-sectional clinical observational study. BMC Neurol. (2019) 19:317. doi: 10.1186/s12883-019-1553-6
244. Caramico-Favero DCO, Guedes ZCF, Morais MB. Food intake, nutritional status and gastrointestinal symptoms in children with cerebral. Palsy. Arq Gastroenterol. (2018) 55:352–7. doi: 10.1590/s0004-2803.201800000-78
245. Perenc L, Przysada G, Trzeciak J. Cerebral palsy in children as a risk factor for malnutrition. Ann Nutr Metab. (2015) 66:224–32. doi: 10.1159/000431330
246. Papadopoulos A, Ntaios G, Kaiafa G, Girtovitis F, Saouli Z, Kontoninas Z, et al. Increased incidence of iron deficiency anemia secondary to inadequate iron intake in institutionalized, young patients with cerebral palsy. Int J Hematol. (2008) 88:495–7. doi: 10.1007/s12185-008-0191-3
247. Sangermano M, D'Aniello R, Massa G, Albano R, Pisano P, Budetta M, et al. Nutritional problems in children with neuromotor disabilities: an Italian case series. Ital J Pediatr. (2014) 40:61. doi: 10.1186/1824-7288-40-61
248. Yip R, Binkin NJ, Fleshood L, Trowbridge FL. Declining prevalence of anemia among low-income children in the United States. JAMA. (1987) 258:1619–23. doi: 10.1001/jama.1987.03400120069027
249. Yip R, Walsh KM, Goldfarb MG, Binkin NJ. Declining prevalence of anemia in childhood in a middle-class setting: a pediatric success story? Pediatrics. (1987) 80:330–4.
250. El Shemy SA, Amer FE, Madani HA. Impact of iron deficiency anemia on functional abilities and muscle strength in children with spastic cerebral palsy. Pak J Biol Sci. (2019) 22:214–9. doi: 10.3923/pjbs.2019.214.219
251. Sweetman DU, Onwuneme C, Watson WR, Murphy JF, Molloy EJ. Perinatal asphyxia and erythropoietin and VEGF: serial serum and cerebrospinal fluid responses. Neonatology. (2017) 111:253–9. doi: 10.1159/000448702
252. O'Hare FM, Watson R, O'Neill A, Blanco A, Donoghue V, Molloy EJ. Persistent systemic monocyte and neutrophil activation in neonatal encephalopathy. J Maternal-Fetal Neonatal Med. (2016) 29:309–16. doi: 10.3109/14767058.2014.1000294
253. Nelson KB, Grether JK, Dambrosia JM, Walsh E, Kohler S, Satyanarayana G, et al. Neonatal cytokines and cerebral palsy in very preterm infants. Pediatr Res. (2003) 53:600–7. doi: 10.1203/01.PDR.0000056802.22454.AB
254. Gomez R, Romero R, Ghezzi F, Yoon BH, Mazor M, Berry SM. The fetal inflammatory response syndrome. Am J Obstet Gynecol. (1998) 179:194–202. doi: 10.1016/S0002-9378(98)70272-8
255. Hagberg H, Gressens P, Mallard C. Inflammation during fetal and neonatal life: implications for neurologic and neuropsychiatric disease in children and adults. Ann Neurol. (2012) 71:444–57. doi: 10.1002/ana.22620
256. Greenwood VJ, Crawford NW, Walstab JE, Reddihough DS. Immunisation coverage in children with cerebral palsy compared with the general population. J Paediatr Child Health. (2013) 49:E137–41. doi: 10.1111/jpc.12097
257. Tervo RC, Taylor B. Vaccinations and the physically handicapped child. Can Med Assoc J. (1982) 127:475–7.
258. Grohskopf LA, Alyanak E, Broder KR, Blanton LH, Fry AM, Jernigan DB, et al. Prevention and Control of Seasonal Influenza with Vaccines: Recommendations of the Advisory Committee on Immunization Practices - United States, 2020-21 Influenza Season. MMWR Recomm Rep. (2020) 69:1–24. doi: 10.15585/mmwr.rr6908a1
259. Smith M, Peacock G, Uyeki TM, Moore C. Influenza vaccination in children with neurologic or neurodevelopmental disorders. Vaccine. (2015) 33:2322–7. doi: 10.1016/j.vaccine.2015.03.050
260. Leach EL, Shevell M, Bowden K, Stockler-Ipsiroglu S, van Karnebeek CD. Treatable inborn errors of metabolism presenting as cerebral palsy mimics: systematic literature review. Orphanet J Rare Dis. (2014) 9:197. doi: 10.1186/s13023-014-0197-2
261. Gupta R, Appleton RE. Cerebral palsy: not always what it seems. Arch Dis Child. (2001) 85:356–60. doi: 10.1136/adc.85.5.356
262. Hakami WS, Hundallah KJ, Tabarki BM. Metabolic and genetic disorders mimicking cerebral palsy. Neurosciences. (2019) 24:155–63. doi: 10.17712/nsj.2019.3.20190045
263. Whitehouse WP. Metabolic testing in children with cerebral palsy: Yield could be up to 20%. Dev Med Child Neurol. (2011) 53:1160. doi: 10.1111/j.1469-8749.2011.04104.x
264. Leonard JM, Cozens AL, Reid SM, Fahey MC, Ditchfield MR, Reddihough DS. Should children with cerebral palsy and normal imaging undergo testing for inherited metabolic disorders? Dev Med Child Neurol. (2011) 53:226–32. doi: 10.1111/j.1469-8749.2010.03810.x
265. Kruer MC, Boddaert N, Schneider SA, Houlden H, Bhatia KP, Gregory A, et al. Neuroimaging features of neurodegeneration with brain iron accumulation. Am J Neuroradiol. (2012) 33:407–14. doi: 10.3174/ajnr.A2677
266. Bower A, Imbard A, Benoist JF, Pichard S, Rigal O, Baud O, et al. Diagnostic contribution of metabolic workup for neonatal inherited metabolic disorders in the absence of expanded newborn screening. Sci Rep. (2019) 9:14098. doi: 10.1038/s41598-019-50518-0
267. Mordaunt D, Cox D, Fuller M. Metabolomics to improve the diagnostic efficiency of inborn errors of metabolism. Int J Mol Sci. (2020) 21:41195. doi: 10.3390/ijms21041195
268. Clish CB. Metabolomics: an emerging but powerful tool for precision medicine. Cold Spring Harb Mol Case Stud. (2015) 1:a000588-a. doi: 10.1101/mcs.a000588
269. Leaw B, Nair S, Lim R, Thornton C, Mallard C, Hagberg H. Mitochondria, bioenergetics and excitotoxicity: New therapeutic targets in perinatal brain injury. Front Cell Neurosci. (2017) 11:199. doi: 10.3389/fncel.2017.00199
270. MacLennan AH, Thompson SC, Gecz J. Cerebral palsy: causes, pathways, and the role of genetic variants. Am J Obstet Gynecol. (2015) 213:779–88. doi: 10.1016/j.ajog.2015.05.034
271. Michael-Asalu A, Taylor G, Campbell H, Lelea L-L, Kirby RS. Cerebral Palsy: Diagnosis, Epidemiology, Genetics, and Clinical Update. Adv Pediatr. (2019) 66:189–208. doi: 10.1016/j.yapd.2019.04.002
272. van Eyk CL, Corbett MA, Maclennan AH. The emerging genetic landscape of cerebral palsy. Handb Clin Neurol. (2018) 147:331–42. doi: 10.1016/B978-0-444-63233-3.00022-1
273. Fahey MC, Maclennan AH, Kretzschmar D, Gecz J, Kruer MC. The genetic basis of cerebral palsy. Dev Med Child Neurol. (2017) 59:462–9. doi: 10.1111/dmcn.13363
274. MacLennan AH, Lewis S, Moreno-De-Luca A, Fahey M, Leventer RJ, McIntyre S, et al. Genetic or Other Causation Should Not Change the Clinical Diagnosis of Cerebral Palsy. J Child Neurol. (2019) 34:472–6. doi: 10.1177/0883073819840449
275. Fleiss B, Gressens P. Tertiary mechanisms of brain damage: a new hope for treatment of cerebral palsy? Lancet Neurol. (2012) 11:556–66. doi: 10.1016/S1474-4422(12)70058-3
276. Weinhold B. Epigenetics: the science of change. Natl Inst Environ Health Sci. (2006) 114:A160–7. doi: 10.1289/ehp.114-a160
277. Fardi M, Solali S, Hagh MF. Epigenetic mechanisms as a new approach in cancer treatment: An updated review. Genes Dis. (2018) 5:304–11. doi: 10.1016/j.gendis.2018.06.003
278. Gartstein MA, Skinner MK. Prenatal influences on temperament development: The role of environmental epigenetics. Dev Psychopathol. (2018) 30:1269–303. doi: 10.1017/S0954579417001730
279. Fitzgerald E, Boardman JP, Drake AJ. Preterm birth and the risk of neurodevelopmental disorders-is there a role for epigenetic dysregulation? Curr Genomics. (2018) 19:507–21. doi: 10.2174/1389202919666171229144807
280. Mohandas N, Bass-Stringer S, Maksimovic J, Crompton K, Loke YJ, Walstab J, et al. Epigenome-wide analysis in newborn blood spots from monozygotic twins discordant for cerebral palsy reveals consistent regional differences in DNA methylation. Clin Epigenetics. (2018) 10:25. doi: 10.1186/s13148-018-0457-4
281. Crowgey EL, Marsh AG, Robinson KG, Yeager SK, Akins RE. Epigenetic machine learning: utilizing DNA methylation patterns to predict spastic cerebral palsy. BMC Bioinform. (2018) 19:225. doi: 10.1186/s12859-018-2224-0
282. Schaefer GB. Genetics considerations in cerebral palsy. Semin Pediatr Neurol. (2008) 15:21–6. doi: 10.1016/j.spen.2008.01.004
283. Kuperminc MN, Gurka MJ, Houlihan CM, Henderson RC, Roemmich JN, Rogol AD, et al. Puberty, statural growth, and growth hormone release in children with cerebral palsy. J Pediatr Rehabil Med. (2009) 2:131–41. doi: 10.3233/PRM-2009-0072
284. Russell M, Breggia A, Mendes N, Klibanski A, Misra M. Growth hormone is positively associated with surrogate markers of bone turnover during puberty. Clin Endocrinol (Oxf). (2011) 75:482–8. doi: 10.1111/j.1365-2265.2011.04088.x
285. Mergler S, Evenhuis HM, Boot AM, De Man SA, Bindels-De Heus KG, Huijbers WA, et al. Epidemiology of low bone mineral density and fractures in children with severe cerebral palsy: a systematic review. Dev Med Child Neurol. (2009) 51:773–8. doi: 10.1111/j.1469-8749.2009.03384.x
286. Bishop N, Arundel P, Clark E, Dimitri P, Farr J, Jones G, et al. Fracture prediction and the definition of osteoporosis in children and adolescents: the ISCD (2013). Pediatric Official Positions J Clin Densitom. (2014) 17:275–80. doi: 10.1016/j.jocd.2014.01.004
287. Bianchi ML, Leonard MB, Bechtold S, Högler W, Mughal MZ, Schönau E, et al. Bone health in children and adolescents with chronic diseases that may affect the skeleton: the 2013 ISCD Pediatric Official Positions. J Clin Densitom. (2014) 17:281–94. doi: 10.1016/j.jocd.2014.01.005
288. Allington N, Vivegnis D, Gerard P. Cyclic administration of pamidronate to treat osteoporosis in children with cerebral palsy or a neuromuscular disorder: a clinical study. Acta Orthop Belg. (2005) 71:91–7.
289. Worley G, Houlihan CM, Herman-Giddens ME, O'Donnell ME, Conaway M, Stallings VA, et al. Secondary sexual characteristics in children with cerebral palsy and moderate to severe motor impairment: a cross-sectional survey. Pediatrics. (2002) 110:897–902. doi: 10.1542/peds.110.5.897
290. Zacharin M, Savasi I, Grover S. The impact of menstruation in adolescents with disabilities related to cerebral palsy. Arch Dis Child. (2010) 95:526–30. doi: 10.1136/adc.2009.174680
291. Rutz E, Brunner R. Management of spinal deformity in cerebral palsy: conservative treatment. J Child Orthopaedics. (2013) 7:415–8. doi: 10.1007/s11832-013-0516-5
292. McCarthy JJ, D'Andrea LP, Betz RR, Clements DH. Scoliosis in the child with cerebral palsy. JAAOS-J Am Acad Orthopaedic Surg. (2006) 14:367–75. doi: 10.5435/00124635-200606000-00006
293. Pettersson K, Wagner P, Rodby-Bousquet E. Development of a risk score for scoliosis in children with cerebral palsy. Acta Orthop. (2020) 91:203–8. doi: 10.1080/17453674.2020.1711621
294. Cloake T, Gardner A. The management of scoliosis in children with cerebral palsy: a review. J Spine Surg. (2016) 2:299. doi: 10.21037/jss.2016.09.05
295. Roberts SB, Tsirikos AI. Factors influencing the evaluation and management of neuromuscular scoliosis: a review of the literature. J Back Musculoskelet Rehabil. (2016) 29:613–23. doi: 10.3233/BMR-160675
296. Pruszczynski B, Sees J, Miller F. Risk factors for hip displacement in children with cerebral palsy: systematic review. J Pediatr Orthop. (2016) 36:829–33. doi: 10.1097/BPO.0000000000000577
297. Miller F, Slomczykowski M, Cope R, Lipton GE. Computer modeling of the pathomechanics of spastic hip dislocation in children. J Pediatr Orthopaedics. (1999) 19:486–92. doi: 10.1097/01241398-199907000-00012
298. Chung MK, Zulkarnain A, Lee JB, Cho BC, Chung CY, Lee KM, et al. Functional status and amount of hip displacement independently affect acetabular dysplasia in cerebral palsy. Dev Med Child Neurol. (2017) 59:743–9. doi: 10.1111/dmcn.13437
299. Novak I, McIntyre S, Morgan C, Campbell L, Dark L, Morton N, et al. A systematic review of interventions for children with cerebral palsy: state of the evidence. Dev Med Child Neurol. (2013) 55:885–910. doi: 10.1111/dmcn.12246
300. Miller SD, Juricic M, Hesketh K, McLean L, Magnuson S, Gasior S, et al. Prevention of hip displacement in children with cerebral palsy: a systematic review. Dev Med Child Neurol. (2017) 59:1130–8. doi: 10.1111/dmcn.13480
Keywords: cerebral palsy—complications, multi-organ dysfunction, cerebral palsy, multi-system involvement, review (article), neurological impairment, neurodevelopmental disorders, neurodisability
Citation: Allen J, Zareen Z, Doyle S, Whitla L, Afzal Z, Stack M, Franklin O, Green A, James A, Leahy TR, Quinn S, Elnazir B, Russell J, Paran S, Kiely P, Roche EF, McDonnell C, Baker L, Hensey O, Gibson L, Kelly S, McDonald D and Molloy EJ (2021) Multi-Organ Dysfunction in Cerebral Palsy. Front. Pediatr. 9:668544. doi: 10.3389/fped.2021.668544
Received: 16 February 2021; Accepted: 15 July 2021;
Published: 09 August 2021.
Edited by:
Sergiusz Jozwiak, Warszawski Uniwersytet Medyczny, PolandReviewed by:
Bernard Dan, Université libre de Bruxelles, BelgiumMarek Józwiak, Poznan University of Medical Sciences, Poland
Copyright © 2021 Allen, Zareen, Doyle, Whitla, Afzal, Stack, Franklin, Green, James, Leahy, Quinn, Elnazir, Russell, Paran, Kiely, Roche, McDonnell, Baker, Hensey, Gibson, Kelly, McDonald and Molloy. This is an open-access article distributed under the terms of the Creative Commons Attribution License (CC BY). The use, distribution or reproduction in other forums is permitted, provided the original author(s) and the copyright owner(s) are credited and that the original publication in this journal is cited, in accordance with accepted academic practice. No use, distribution or reproduction is permitted which does not comply with these terms.
*Correspondence: Eleanor J. Molloy, eleanor.molloy@tcd.ie