- 1Department of Pediatric Neurology, Medical School, Comenius University and National Institute of Children's Diseases, Bratislava, Slovakia
- 2Department of Pediatrics, University Hospital Salzburg, Paracelsus Medical University Salzburg, Salzburg, Austria
- 3Department of Neonatology, Kepler University Hospital Med Campus IV, Johannes Kepler University Linz, Linz, Austria
- 4Division of Neuropathology, Department of Pathology and Molecular Pathology, Kepler University Hospital Neuromed Campus, Johannes Kepler University Linz, Linz, Austria
- 5Department of Medical Genetics, Kepler University Hospital Med Campus IV, Johannes Kepler University Linz, Linz, Austria
Mitochondriopathies represent a wide spectrum of miscellaneous disorders with multisystem involvement, which are caused by various genetic changes. The establishment of the diagnosis of mitochondriopathy is often challenging. Recently, several mutations of the VARS2 gene encoding the mitochondrial valyl-tRNA synthetase were associated with early onset encephalomyopathies or encephalocardiomyopathies with major clinical features such as hypotonia, developmental delay, brain MRI changes, epilepsy, hypertrophic cardiomyopathy, and plasma lactate elevation. However, the correlation between genotype and phenotype still remains unclear. In this paper we present a male Caucasian patient with a recurrent c.1168G>A (p.Ala390Thr) and a new missense biallelic variant c.2758T>C (p.Tyr920His) in the VARS2 gene which were detected by whole exome sequencing (WES). VARS2 protein was reduced in the patient's muscle. A resulting defect of oxidative phosphorylation (OXPHOS) was proven by enzymatic assay, western blotting and immunohistochemistry from a homogenate of skeletal muscle tissue. Clinical signs of our patient included hyperlactatemia, hypertrophic cardiomyopathy (HCM) and pulmonary hypertension, which led to early death at the age of 47 days without any other known accompanying signs. The finding of novel variants in the VARS2 gene expands the spectrum of known mutations and phenotype presentation. Based on our findings we recommend to consider possible mitochondriopathy and to include the analysis of the VARS2 gene in the genetic diagnostic algorithm in cases with early manifesting and rapidly progressing HCM with hyperlactatemia.
Introduction
Mitochondria are organelles responsible for energy production in the form of adenosine triphosphate (ATP) through the oxidative phosphorylation system (OXPHOS). The process of energy production is performed by the mitochondrial respiratory chain and ATP synthase located in the inner membrane of mitochondria (1). Mitochondrial disorders represent a wide spectrum of heterogeneous syndromes predominantly presenting with symptoms as lactic acidosis, hypotonia, developmental delay, failure to thrive, and encephalopathy due to cell energy depletion. Mitochondrial disorders are associated with many causative genes (2–4), which are encoded by mitochondrial DNA (mtDNA) or nuclear DNA (5). Valyl-tRNA synthetase (Val-tRNA) involved in mitochondrial translation (the process of protein synthesis from the information contained in a molecule of messenger RNA – mRNA) is encoded by the VARS2 gene (6) and catalyzes the amino acid valine's attachment to its tRNA (7). Dysfunction of the protein product is responsible for combined oxidative phosphorylation deficiency 20 (OMIM: # 615917) inherited in an autosomal recessive manner. Rare biallelic variants in the VARS2 gene have been associated with severe clinical features as mitochondrial encephalomyopathy or encephalocardiomyopathy in 23 affected individuals from 19 families worldwide (1, 4, 6–15). In the present paper we describe a compound heterozygous case with a recurrent c.1168G>A (p.Ala390Thr) and a novel missense variant c.2758T>C (p.Tyr920His) in the VARS2 gene causing isolated hypertrophic cardiomyopathy, hyperlactatemia, and pulmonary hypertension leading to early death. The pathogenicity of the detected variants is supported by immunohistochemistry, enzymatic analysis, and western blot in a muscle sample of the affected individual. Finally, we compare our findings with the case reports published so far.
Methods
Clinical Description
All clinical information about our patient was obtained from medical records retrospectively with the patient's parents' informed consent and was correlated with potential causative mutations detected by whole-exome sequencing (WES).
Genetic Investigation
Genetic testing was performed from the peripheral blood of the patient. Karyotyping was performed according to a standard cytogenetic protocol. SNP array was performed with Illumina Cyto 850Kv1.1 Bead Chip. In addition, DNA isolated from the patient's peripheral blood lymphocytes was analyzed by whole-exome sequencing. The library was prepared by SureSelect60Mbv6 (Agilent) and paired-end sequenced on a HiSeq 4000 platform (Illumina) with a read-length of 100 bases. In order to align reads to the human genome assembly hg19 Burrows-Wheeler Aligner (BWA, v.0.5.87.5) was applied and detection of genetic variation was performed using SAMtools (v 0.1.18), PINDEL (v 0.2.4t), and ExomeDepth (v 1.0.0). The cut-off for biallelic inheritance was set to <1% allele frequency, for monoallelic inheritance to <0.1%. The size of reference entries was >20,000 exomes in the database at the time of analysis (16). With this approach, 97.3% of the target sequences were covered >20-fold. The detected mutations were verified by Sanger sequencing, followed by examining the DNA from peripheral blood of the patient's parents.
Muscle Biopsy
The biopsy of the vastus lateralis muscle was performed under general anesthesia according to standard procedures. Muscle tissue was cut into several pieces for formalin fixation, glutaraldehyde fixation, and rapid freezing in isopentane cooled in liquid nitrogen for subsequent analyses.
Histopathology
The histopathology and the enzyme histochemical stain analysis encompassed the following stains: Haematoxylin and Eosin (H&E), Elastica van Gieson (EvG), Congored, ATPase at pH 9,4, pH 4,3 and 4,2, reduced nicotinamide adenine dinucleotide-tetrazolium reductase (NADH), Gomori trichrome, cytochrome c oxidase (COX), succinate dehydrogenase (SDH), acid phosphatase, periodic acid-Schiff (PAS), Sudan black, myoadenylate deaminase (MAD), phosphofructokinase (PFK), phosphorylase, and acetylcholinesterase (ACE). The immunohistochemical analyses included the following antibodies: CD3, CD20, CD8, CD4, CD45, CD79a, CD68, HLA-DRII, beta-spectrin, alpha-sarcoglycan, beta-sarcoglycan, gamma-sarcoglycan, delta-sarcoglycan, dystrophin (N-terminus), dystrophin (C-terminus), dystrophin (rod domain), dysferlin, titin, emerin, telethonin, POMT1, myotilin, lamin A/C, caveolin-3, actinin, laminin2, collagen VI, desmin, myosin fast, myosin slow, myosin neonatal, membrane attack complex, vimentin, and utrophin at appropriate dilutions.
Enzymatic Analysis
Muscle 600 × g homogenates were used for determination of enzymatic activities of the OXPHOS complexes. Enzyme activities of the OXPHOS complexes were determined as previously described (17). The rotenone-sensitive complex I activity was measured spectrophotometrically as NADH/decylubiquinoneoxidoreductase at 340 nm. The enzyme activities of citrate synthase, complex IV (ferrocytochrome c/oxygenoxidoreductase), and oligomycin-sensitive ATPase activity of the F1FO ATP synthase (complex V) were determined as previously described (18). The whole reaction mixture for the ATPase activity measurement was treated for 10 s with an ultra-sonifier (Bio cell disruptor 250, Branson, Vienna, Austria). The reaction mixture for the measurement of the complex III activity contained 50 mM potassium phosphate buffer pH 7.8, 2 mM EDTA, 0.3 mM KCN, 100 μM cytochrome c, 200 μM reduced decyl-ubiquinol. The reaction was started by addition of the 600 g homogenate. After 3–4 min the reaction was inhibited with 1 μM antimycin A. All spectrophotometric measurements (Uvicon 922, Kontron, Milan, Italy) were performed at 37°C.
Substrate Oxidation Analysis
Muscle 600 × g homogenate was incubated with different 14C-labeled substrates (Table 1) for 20 min at 37°C according to Bookelman et al. (19) in a reaction volume of 50 μl. The reactions were stopped by the addition of 20 μl of 15% HClO4 and the 14CO2 was collected in 1M NaOH-wetted filter paper snippets (Whatman) placed in the screw cap of 2 ml reaction tubes (Sarstedt). After incubation for 15 min on ice, the filter papers were transferred to new 2 ml reaction tubes containing 1 ml of scintillation buffer Ultima Gold (Perkin Elmer) and counted in a scintillation counter (Packard 1600 TR). The scintillation counts of the different substrate combinations were related to the total activities per reaction and calculated as nmol/h/mg protein activity oxidation.
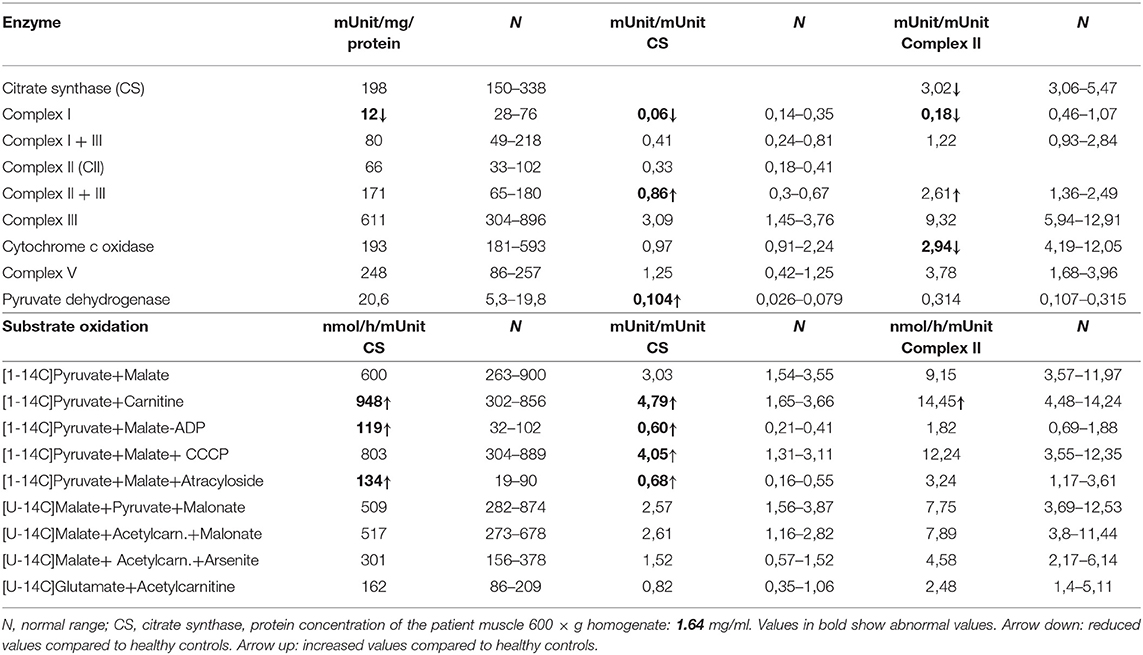
Table 1. OXPHOS enzymes activity measurements and substrate oxidation in muscle of the reported patient with VARS2 deficiency.
Western Blot
Muscle tissue homogenates from the patient and controls (centrifuged at 600 × g) were analyzed on 10% acrylamide/bisacrylamide gels and transferred to nitrocellulose membranes. The membranes were washed in Tris-buffered saline (TBS) for 5 min, air-dried for 30 min, washed 10 min in TBS, and blocked 1 h at room temperature in 1x western blocking solution in TBS-T (Roche, Mannheim, Germany). After washing with TBS-Tween 20 (0.5%; TBS-T), the membranes were incubated with the primary antibody diluted in 1x western blocking solution in TBS-T. The indicated primary antibody dilutions and incubation times were used for western blot analysis: polyclonal rabbit VARS2 (1:1,000, o/n, 4°C; Proteintech), monoclonal mouse NDUFS4 (1:1,000, 1 h, room temperature; Abcam), SDHA (1:2,000, 1 h, room temperature; Abcam), UQCRC2 (1:1,500, 1 h, room temperature; Abcam); MT-CO2 (1:1,000, 1 h, room temperature; Abcam), ATP5F1A (1:2,000, 1 h, room temperature; Abcam), VDAC1 (1:2,000, 1 h, room temperature; Abcam), CS (1:3,000, 1 h, room temperature; THP), GPI (1:800, 1 h, room temperature; Santa Cruz). Membranes were incubated with secondary antibodies labeled polymer horseradish peroxidase-(HRP) 1:100 (EnVisionkit, Dako) at room temperature. Detection was carried out with Lumi-Light PLUSPOD substrate (Roche).
Immunohistochemistry
Muscle tissue was stained as previously described (20–23). For IHC, the following antibodies were used: complex I subunit NDUFB8 (rabbit polyclonal, 1:500; Abcam, Cambridge, UK), complex II subunit SDHA (mouse monoclonal, 1:2,000; Abcam, Cambridge, UK), complex III subunit UQCRC2 (mouse monoclonal, 1:1,500; Abcam, Cambridge, UK), complex IV subunit MT-CO1 (mouse monoclonal, 1:1,000; Abcam, Cambridge, UK), complex V subunit ATP5F1A (mouse monoclonal, 1:2,000; Abcam, Cambridge, UK), and VDAC1 (mouse monoclonal, 1:3,000; Abcam, Cambridge, UK). All antibodies were diluted in Dako antibody diluent with background-reducing components (Dako, Glostrup, Denmark). For antigen retrieval, the sections were immersed for 45 min in 1 mM EDTA, 0.05% Tween-20, pH 8, at 95°C. Muscle tissue sections were incubated for 60 min with the above-mentioned primary antibodies. Staining was done with the DAKO envision kit.
Results
Case Report – Clinical Features of the Patient
The male patient was born at term (at 39+3 weeks of gestation) by vaginal delivery using vacuum extraction, as the first child of non-consanguineous Caucasian parents. Due to the prenatally suspected aortic stenosis, the patient was immediately transferred to the Neonatal Intensive Care Unit. Birth weight (3140 g; 21st percentile), birth length (51 cm; 48th percentile), head circumference (33.5 cm; 17th percentile), Apgar scores 9/10/10, and pH from the umbilical artery were normal (pH: 7.27). The newborn had marks after vacuum extraction over the parietal region (cephalohematoma); muscle tone was normal, and no dysmorphic features were present. Postnatal echocardiography showed borderline width of the isthmus and aortic arch (but still within normal range), bicuspid aortic valve, and an open ductus arteriosus Botalli. No surgery or prostaglandin treatment was necessary, but the patient developed a need for oxygen therapy. On the third day after birth blood gas analysis showed an uncompensated metabolic acidosis with hyperlactatemia: plasma lactate: 6.7 mmol/l (normal range <2.1 mmol/l), pH: 7.28 (N: 7.35–7.45), pCO2: 42.1 mmHg (N: 35–45 mmHg), : 18.2 mmol/l (N: 21–28), Base excess: −7.3 (N: 0 ± 3 mmol/l) with normoglycemia (Blood glucose: 59 mg/dl, N: 50–114). Anion gap was slightly elevated (AG: 16.9, N: 7–16). Since an inherited disorder of metabolism was suspected, metabolic screening was performed, which showed repeatedly lactate elevation: preprandial lactate: 6 mmol/l (N: 0.5–1.6), 3-OH-butyrate: 0.1 mmol/l (N: 0.03–0.3) glucose: 57 mg/dl (N: 50–114); postprandial lactate: 4 mmol/l, 3-OH-butyrate: 0.3 mmol/l, glucose: 84 mg/dl; plasmatic alanine elevation: 539.8 μmol/l (N: 116–376) and normal level of lactate in urine (19 mmol/mol creatinine, N 51 (1–156) mmol/mol creatinine). Other parameters (acylcarnitine profile, creatine kinase, aminotransferases, ammonemia, electrolytes, blood count) were normal. Glucose infusion (5 mg/kg/min.) was administered. The patient could have oral intake (suction) and neurological status was normal, with no signs of lethargy or hypotonia. Sonography of the abdomen did not show any pathology, especially no hepatosplenomegaly. Ophthalmologic examination showed normal findings without signs of cataract. The patient developed progressive symptoms of heart failure over the next few days. Echocardiography on the 16th day of life revealed primary pulmonary hypertension and hypertrophic cardiomyopathy of the left heart. Investigation of alpha-glucosidase excluded Pompe disease (19 nmol/mg–normal value). Cardiac catheterization showed pulmonary hypertension (PHT) and hemodynamic failure of the right heart. The myocardial biopsy was contraindicated due to the patient's severe clinical condition. Dobutamine, nitric oxide therapy, sildenafil, and oxygen therapy, with 60% FiO2 was administered. Based on suspicion of a mitochondrial disorder, muscle biopsy from the vastus lateralis muscle was performed for histopathological purposes. No changes for a primary muscle disorder were found. Based on specific enzyme histochemical staining (e.g., combined COX-SDH), no evidence for the presence of a mitochondrial myopathy could be demonstrated. Genetic testing of the ACAD9 gene associated with mitochondrial complex I deficiency, nuclear type 20 (OMIM: # 611126) did not reveal any pathogenic mutation, and therefore whole-exome sequencing was indicated. Because of lasting suspicion of a mitochondrial disorder, treatment with riboflavin (20 mg/kg/d), coenzyme Q10 (30 mg/kg/d), and continuous intravenous glucose was administered. Under this treatment, lactate levels declined but still were above normal values. Levels were elevated during PHT crises (values during PHT crises were 11–13.3 mmol/l). On day 22, cardiac insufficiency worsened, and a first cardiopulmonary resuscitation was necessary. MRI of the brain and thorax performed on day 23 showed a focal hemorrhage in the right frontal subcortical region. The degree of myelination was appropriate for age. Cardiomegaly was evident. Low pleural effusions on both sides of the thorax and dystelectasia/atelectasia in both lungs were seen. On day 31, because of recurrent PHT crisis, dilatation and stent treatment of the foramen ovale was performed but was not successful. The patient was intubated and sedated with ketamine, midazolam, sufentanil, and myorelaxed. Cardiomyopathy progressed despite intensive care and the patient died on day 47 due to cardiopulmonary failure. A few days after the patient's death, the result of the WES analysis confirmed the state of compound heterozygosity for variants in the VARS2 gene, supporting the assumption of a mitochondrial disorder in the child. Following the wish of the parents, no autopsy was performed.
Molecular Genetics Findings
Cytogenetic examination showed a normal male karyotype 46, XY. Genomic imbalances were ruled out by the SNP array. The whole-exome sequencing revealed the following biallelic variants in VARS2 gene: allele 1 [NM_020442.6:exon13: c.1168G>A (p.Ala390Thr)] (https://www.ncbi.nlm.nih.gov/clinvar/variation/522814/); allele 2 [NM_020442.6:exon27: c.2758T>C (p.Tyr920His)] (https://www.ncbi.nlm.nih.gov/clinvar/variation/997679/) (Figures 1A,B). The finding was verified by Sanger sequencing. Examination of the parents showed the paternal VARS2 variant c.1168G>A (p.Ala390Thr) in a heterozygous form and the maternal VARS2 variant c.2758T>C (p.Tyr920His) in a heterozygous form (Figure 1C). Both variants involve residues that are highly conserved among phylogenetically distant organisms. At position 920, tyrosine is most frequently found in some organisms, also the amino acid phenylalanine, both with a phenyl group. In plants, this position is not conserved with serine or threonine in the alignment. Position 390 is highly conserved (Figure 1D). Position 390 corresponds to the tRNA-synthetase domain and position 920 corresponds to the anticodon binding domain of the protein VARS2 (1, 12). These findings support the pathogenicity of the variants found in our patient.
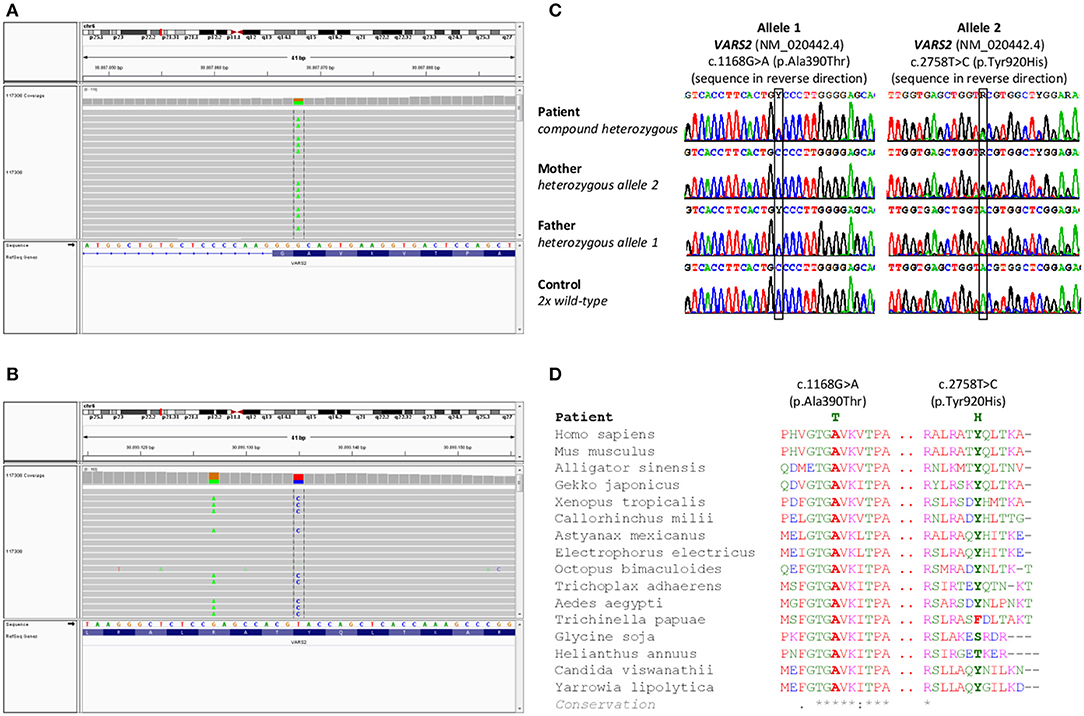
Figure 1. Results of genetic testing. (A,B) Whole exome sequencing results in a patient with a finding of biallelic variants in the VARS2 gene, heterozygous variant c.2758T>C (p.Tyr920His) and heterozygous variant c.1168G>A (p.Ala390Thr). (C) Sanger sequencing confirmed both variants in the patient. Heterozygous variant c.2758T>C (p.Tyr920His) was found in patient's mother and heterozygous variant c.1168G>A (p.Ala390Thr) was found in patient's father. These findings confirmed biallelic position of variants in the patient, (D) shows phylogenetic conservation of VARS2 protein (NP 065175.4) in various organisms. Multiple sequence alignment was performed with Clustal Omega (https://www.ebi.ac.uk/Tools/msa/clustalo/).
Histopathology, Immunohistochemistry, Enzymatic Analysis, Substrate Oxidation, and Western Blot
Western blot analysis revealed a reduction of VARS2 protein amount in muscle homogenates of the affected patient compared to seven controls (Figures 2A,C; Supplementary Table 1). VARS2 was normalized to four loading proteins namely VDAC1, CS, GPI, and SDHA. Residual VARS2 protein levels were between 16 and 34% of the control levels. No obvious histopathological findings pointing toward a mitochondrial disorder were found. The enzymatic analysis showed values in or above the normal range for the mitochondrial marker enzyme citrate synthase (summary of the results is shown in Table 1). Absolute complex I activity was significantly reduced in patient muscle compared to controls. Consistently, the complex I activities normalized to the citrate synthase and complex II were significantly lower in patients muscle (Table 1). Normalized to complex II which is not dependent on mitochondrial translation a downregulation of complex IV was found. An increase in combined normalized complex II-III activity was present. A compensatory upregulation of unaffected complexes is a well-known phenomenon in mitochondrial disorders. In substrate oxidation analysis some activities were in relation to the protein content in the normal range, others were elevated. Also in relation to citrate synthase similar activities were high. In relation to complex II the oxidation rate of pyruvate + carnitine was slightly elevated. These elevated activities are likely due to compensatory upregulation of mitochondria. In contrast, the ratio of the long oxidation path via pyruvate + malate compared to pyruvate + carnitine was somewhat reduced with a value of 0.63 (normal 0.68–1.09). This is consistent with a defect in the respiratory chain, while the reaction from pyruvate to acetyl-carnitine works better. This shortcut of the full oxidation route forms only one NADH, while the whole path creates 4 NADH and 1 FADH2. Consistently, western blot analysis revealed a reduction of complexes I, III and IV in patient homogenate compared to controls (Figure 2). The reduction was present compared to several normalization proteins VDAC1 (outer mitochondrial membrane), CS (mitochondrial matrix), GPI (cytosol) and SDHA (inner mitochondrial membrane and independent from mitochondrial translation) (Figure 2; Supplementary Figure 1; Supplementary Table 1). Also in immunohistochemical staining of VDAC1 (Voltage-dependent anion-selective channel 1) and subunits of the OXPHOS complexes in skeletal muscle showed a slight reduction of NDUFB8 (complex I subunit) and UQCRC2 (complex III subunit) in the affected individual compared to controls (Supplementary Figure 2). A compensatory upregulation of complex II was also present in immunohistochemical staining.
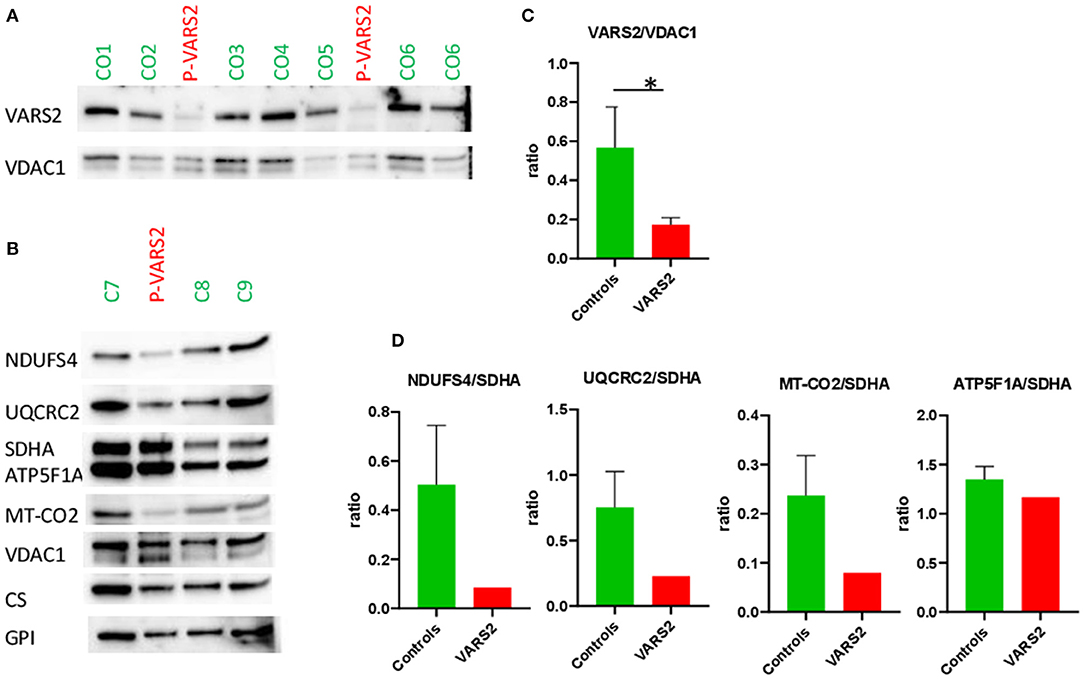
Figure 2. (A,B) Western blot analysis of the muscle sample of the patient compared to healthy controls, using antibodies against VARS2, NADH dehydrogenase [ubiquinone] iron-sulfur protein 4 (NDUFS4), ubiquinol-cytochrome c reductase core protein 2 (UQCRC2), succinate dehydrogenase complex flavoprotein subunit A (SDHA), ATP synthase F1 subunit alpha (ATP5F1A), mitochondrially encoded cytochrome c oxidase II (MT-CO2), voltage-dependent anion-selective channel 1 (VDAC1, in outer mitochondrial membrane), citrate synthase (CS), glucosephosphate isomerase (GPI). (C,D) Bar graphs show the densitometry of the western blot bands. The intensity of the bands was determined using evaluation software (Imagelab, Biorad). The subunits of the OXPHOS complexes were evaluated in relation to VDAC1, CS, and GPI as loading controls. The results showed reduction of complex I in comparison to SDHA; moderate reduction of complex III and IV in comparison to SDHA and compensatory up-regulation/increase of complexes II and V. The ratios to VDAC1, CS, and GPI are shown in Supplementary Table 1. *p < 0.05.
Discussion
Mitochondriopathies associated with mutations in mitochondrial aminoacyl-tRNA synthetases present a wide spectrum of miscellaneous disorders based on various genetic changes. Recently, several mutations in VARS2 gene were associated with clinical features such as hypotonia, psychomotor delay, encephalopathy, cardiomyopathy, hyperlactatemia, but the correlation between genotype and phenotype remains unclear (1, 15). To date, 19 families with more than 23 affected individuals have been described in the literature worldwide (1, 4, 6–15) whereby c.1100C>T (p.Thr367Ile) is the most common variant in the VARS2 gene present in 60.8% (14/23) of the published cases (see Table 2). Common features of homozygous carriers for variant c.1100C>T (p.Thr367Ile) include microcephaly, global psychomotor delay, and hypotonia, less often nystagmus, limb spasticity, and difficulties with feeding. In the first 12 months of life, ataxia, dystonic movements, and seizures can occur, which later (from 2 to 4 years) almost always lead to refractory epilepsy and status epilepticus based on mitochondrial encephalopathy (1, 6, 13). Nevertheless, hypertrophic cardiomyopathy (HCM) has never been observed in these patients (eight patients of which five had no HCM, in three cases the results of cardiologic examination are not available) (1, 6, 13, 15). However, in compound heterozygotes (for variant c.1100C>T (p.Thr367Ile) and another, or two other pathogenic variants, hypertrophic cardiomyopathy was present in 14/15 cases (1, 4, 7–12, 14). These additional findings support Bruni et al. (1) assumption that “c.1100C>T variant could have a lesser effect to the heart.” In the present paper we describe a compound heterozygous case with a recurrent c.1168G>A (p.Ala390Thr) and a novel missense variant c.2758T>C (p.Tyr920His) in the VARS2 gene. Position 390 corresponds to the tRNA-synthetase domain and position 920 corresponds to the anticodon binding domain of the protein VARS2 (1, 12). Both variants show a relatively high level of phylogenetic conservation (Figure 1D). The p.Ala390Thr was reported in ClinVar four times (Accession number: VCV000522814.3). Bruni et al. (1) published a case with a homozygous p.Ala390Thr underlining its pathologic relevance. However, we for the first time proved pathogenicity of this obviously recurrent variant with biochemical and immunohistochemical methods. Here we show that the recurrent p.Ala390Thr in combination with a novel missense variant causes a severe reduction of VARS2 protein amount. In contrast with published data, in our case, HCM was a leading feature accompanied by hyperlactatemia and pulmonary hypertension, which led to early death (on 47th day of life) without any other accompanying signs. We see a certain similarity in the case of a patient with pathogenic VARS2 mutations c.643C>T (p.His215Tyr) and c.1354A>G (p.Met452Val) who presented with poor sucking at birth, poor motor activity, hyporeflexia, hypertonia, persistent pulmonary hypertension of the newborn (PPHN), metabolic acidosis, severe lactic acidosis, and hypertrophic cardiomyopathy (12). The patient died on the 16th day of life due to cardiac arrest due to pulmonary hypertension and hypertrophic cardiomyopathy. Our patient had a normal neurological assessment with no hypotonia and no signs of encephalopathy, neither clinically nor on brain MRI on the 23rd day of life; this finding contrasts with published data (1, 15). Finally, we correlated published histopathological findings of skeletal muscle tissue with negative findings in our patient. Five of 13 patients reported by Bruni et al. (1) underwent muscle biopsy (quadriceps muscle). Two patients showed normal histopathologic findings, one case had mild unspecific myopathic changes with COX-deficient/SDH-positive fibers, and one patient showed signs of neurogenic atrophy. The fifth patient showed at the electron microscopy level mitochondria that were relatively decreased in number and size, with mostly unremarkable morphology, although some were distorted or atypical (with atypical cristae). The data from San Millan et al. (8) demonstrate an uneven involvement of muscles with predominant involvement of myocardium and diaphragm. Our patient had no obvious histopathological findings pointing toward a mitochondrial disorder in vastus lateralis muscle. Unfortunately, a myocardial biopsy was contraindicated in our patient due to the patient's serious clinical condition and an autopsy was not done following the wish of the patient's parents. It would be interesting to evaluate the lactate peak by MR spectroscopy and determine lactate and alanine in cerebrospinal fluid (1, 6, 11, 14), but these were unfortunately not performed during the patient's life. OXPHOS activity was evaluated in eight patients from Bruni et al. (1), Diodato et al. (6) San Millan et al. (8), and Begliuomini et al. (15). However, OXPHOS activity was decreased only in six of them (the first patient had low complex IV activity, the second patient had combined complex I + complex IV deficiencies, the third patient had low complex IV activity, the fourth had low complex I activity, the fifth had low complex IV activity, and the sixth showed reduced complex I and III activity). In our case the results showed a strong reduction of complex I, a moderate reduction of complex III and IV, and compensatory upregulation in complex II and V. A review of all published patients so far is shown in Table 2. Clinical manifestations, disease severity, and life expectancy vary significantly between published cases. We assume that the different phenotypic manifestations in the reported cases could result from the variable expression of valyl-tRNA synthetase in different tissues at different time points after birth, and/or due to unknown epigenetic effects, but further investigations in this field are needed.
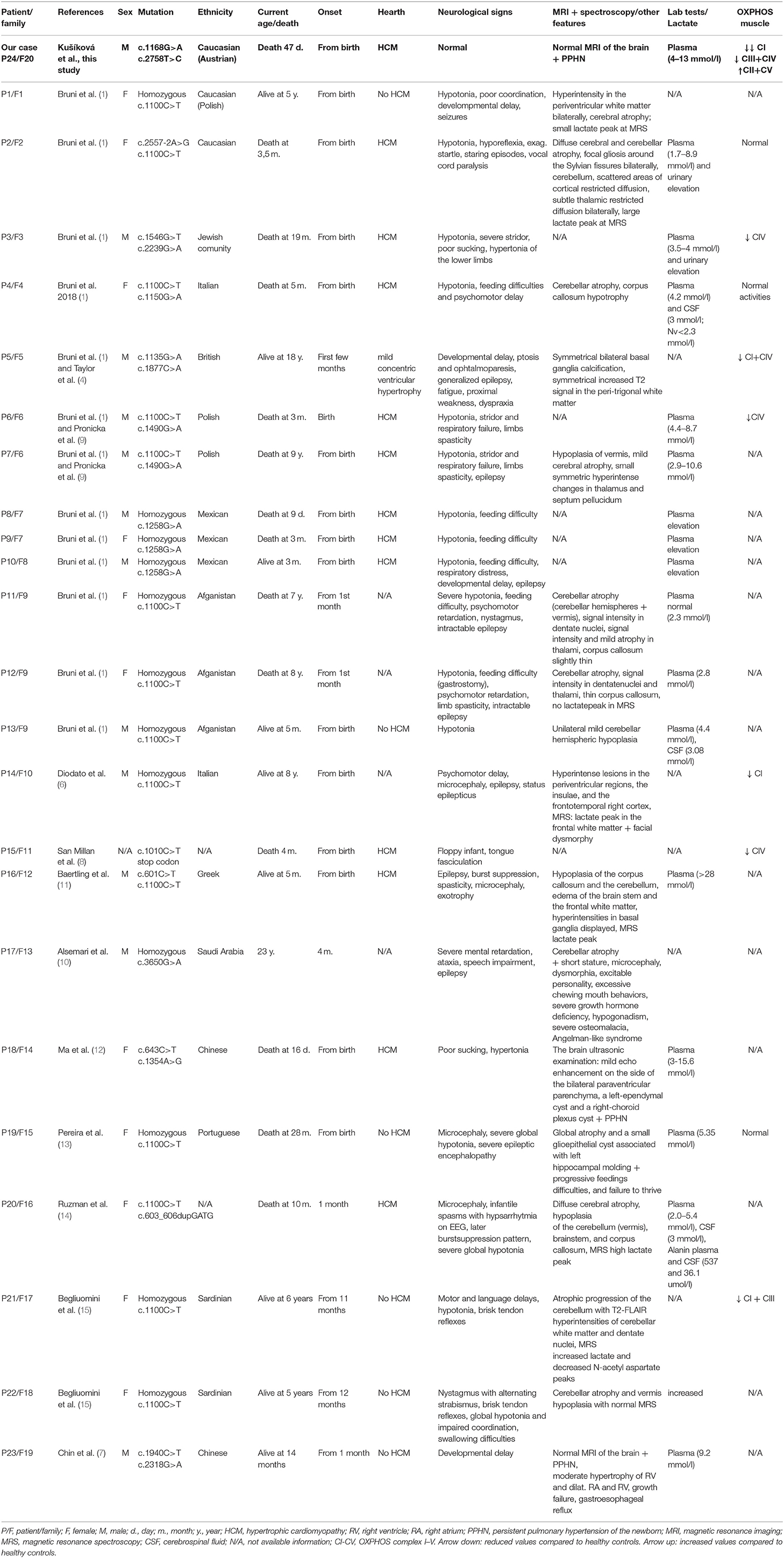
Table 2. Review of published cases and new patient with VARS2 deficiency – clinical characteristics, laboratory and molecular genetics findings [modified after (1)].
Conclusion
The data and finding of a novel variants in the VARS2 gene of our case expands the spectrum of known mutations in humans and the spectrum of associated clinical features. Nowadays, thanks to increasing number of publish data we can assume a reduced impact of the homozygous c.1100C>T (p.Thr367Ile) mutation on the myocardium, but we cannot postulate that specific OXPHOS complexes or organ systems are impaired due to specific mutations in the VARS2 gene. Further investigations to find specific genotype-phenotype correlations are needed in this field. It is known that biallelic mutations in the VARS2 gene cause systemic impairment. Structural cardiac abnormalities and hypertrophic cardiomyopathy (HCM) could be the first manifestation of the disease leading to early death in the newborn period or in early infancy before developing other clinical features. That is why we think that it is of utmost importance to consider the presence of a possible mitochondriopathy in these patients and to include the analysis of the VARS2 gene in the genetic diagnostic algorithm in cases with early manifesting and rapidly progressing HCM with hyperlactatemia.
Data Availability Statement
Publication of the complete exome data is not included in the consent for clinical exome sequencing. The VARS2 variants have been made publicly available via ClinVar. Requests to access the datasets in more detail can be directed to the corresponding author.
The ClinVar registration numbers are: SUB9123334, SUB9123465 and they are publicly available:
Variant 1: https://www.ncbi.nlm.nih.gov/clinvar/variation/522814/
Variant 2: https://www.ncbi.nlm.nih.gov/clinvar/variation/997679/.
Ethics Statement
The studies involving human participants were reviewed and approved by Ethics Committee of the Land Salzburg (number 415-E/2552/10-2019). Written informed consent to participate in this study was provided by the participants' legal guardian/next of kin.
Author Contributions
This manuscript was written by KK under the guidance of DW. RF, SW, and JM conceptualized figures, revised, and edited the manuscript. H-CD performed sample collection. BC provided clinical data. OK performed muscle biopsy. All authors contributed to the article and approved the submitted version.
Funding
This work was supported by the Austrian Science Fund (FWF) [I 4695-B, GENOMIT] to JM.
Conflict of Interest
The authors declare that the research was conducted in the absence of any commercial or financial relationships that could be construed as a potential conflict of interest.
Acknowledgments
The authors would like to thank the patient's family. The patient's parents signed informed consent for publication.
Supplementary Material
The Supplementary Material for this article can be found online at: https://www.frontiersin.org/articles/10.3389/fped.2021.660076/full#supplementary-material
Supplementary Table 1. Densitometric analysis of the western blotting experiments shown in Figure 2 and Supplementary Figure 1.
Supplementary Figure 1. Western blot analysis of the muscle sample of the patient compared to healthy controls, using antibodies against VARS2, NADH dehydrogenase [ubiquinone] iron-sulfur protein 4 (NDUFS4), ubiquinol-cytochrome c reductase core protein 2 (UQCRC2), succinate dehydrogenase complex flavoprotein subunit A (SDHA), ATP synthase F1 subunit alpha (ATP5F1A), voltage-dependent anion-selective channel 1 (VDAC1), citrate synthase (CS), glucosephosphate isomerase (GPI). Because of a low gel resolution ATP5F1A and UQCRC2 cannot be visually separated in the technical replicate 1 of the western blot.
Supplementary Figure 2. Immunohistochemical staining of VDAC1 and subunits of the OXPHOS complexes in skeletal muscle of affected individual and controls. (A–C) VDAC1 staining; (D–F) NDUFB8 staining; (G–I) SDHA staining; (J–L) UQCRC2 staining; (M–O) MT-CO1 staining; (P–R) ATP5F1A staining; (A,D,G,J,M,P) control 1; (B,E,H,K,N,Q) control 2; (C,F,I,L,O,R) affected individual. Images were taken with a 400x magnification. A slight reduction of NDUFB8 and UQCRC2 was present in muscle of the affected individual compared to controls.
References
1. Bruni F, Meo Di I, Bellacchio E, Webb BD, McFarland R, Chrzanowska-Lightowlers ZMA, et al. Clinical, biochemical, and genetic features associated with VARS2-related mitochondrial disease. Hum Mutat. (2018) 39:563–78. doi: 10.1002/humu.23398
2. Ghezzi D, Zeviani M. Assembly factors of human mitochondrial respiratory chain complexes: physiology and pathophysiology. In: Kadenbach B, editor. Mitochondrial Oxidative Phosphorylation. Advances in Experimental Medicine and Biology, Vol 748. New York, NY: Springer (2012). p. 65–106. doi: 10.1007/978-1-4614-3573-0_4
3. Rötig A. Human diseases with impaired mitochondrial protein synthesis. Biochim Biophys Acta. (2011) 1807:1198–205. doi: 10.1016/j.bbabio.2011.06.010
4. Taylor RW, Pyle A, Griffin H, Blakley EL, Duff J, He L, et al. Use of whole-exome sequencing to determine the genetic basis of multiple mitochondrial respiratory chain complex deficiencies. JAMA. (2014) 312:68–77. doi: 10.1001/jama.2014.7184
5. Spinazzola A, Zeviani M. Mitochondrial diseases: a cross-talk between mitochondrial and nuclear genomes. In: Espinós C, Felipo V, Palau F, editors. Inherited Neuromuscular Diseases. Advances in Experimental Medicine and Biology, Vol 652. Dordrecht: Springer (2009). p. 69–84. doi: 10.1007/978-90-481-2813-6_6
6. Diodato D, Melchionda L, Haack TB, Dallabona C, Baruffini E, Donnini C, et al. VARS2 and TARS2 mutations in patients with mitochondrial Encephalomyopathies. Hum Mutat. (2014) 35:983–9. doi: 10.1002/humu.22590
7. Chin HL, Goh DL, Wang FS, Tay SKH, Heng CK, Donnini C, et al. A combination of two novel VARS2 variants causes a mitochondrial disorder associated with failure to thrive and pulmonary hypertension. J Mol Med. (2019) 97:1557–66. doi: 10.1007/s00109-019-01834-5
8. San Millan B, Blazquez A, Delmiro A, Ruffian L, Navarro C, Teijeira S, et al. Variable skeletal muscle involvement in VARS2 mitochondrial encephalomyopathy. NMD. (2016) 26:178. doi: 10.1016/j.nmd.2016.06.333
9. Pronicka E, Piekutowska-Abramczuk D, Ciara E, Trubicka J, Rokicki D, Karkucinska-Wieckowska A, et al. New perspective in diagnostics of mitochondrial disorders: two years' experience with whole-exome sequencing at a national paediatric centre. J Transl Med. (2014) 14:174. doi: 10.1186/s12967-016-0930-9
10. Alsemari A, Al-Younes B, Goljan E, Jaroudi D, BinHumaid F, Meyer BF, et al. Recessive VARS2 mutation underlies a novel syndrome with epilepsy, mental retardation, short stature, growth hormone deficiency, and hypogonadism. Human Genomics. (2017) 11:28. doi: 10.1186/s40246-017-0130-6
11. Baertling F, Alhaddad B, Seibt A, Budaeus S, Meitinger T, Strom TM, et al. Neonatal encephalocardiomyopathy caused by mutations in VARS2. Metab Brain Dis. (2017) 32:267–70. doi: 10.1007/s11011-016-9890-2
12. Ma K, Xie M, He X, Liu G, Lu X, Peng Q, et al. A novel compound heterozygous mutation in VARS2 in a newborn with mitochondrial cardiomyopathy: a case report of a Chinese family. BMC Med Genet. (2018) 19:202. doi: 10.1186/s12881-018-0689-3
13. Pereira S, Adrião M, Sampaio M, Basto MA, Rodrigues E, Vilarinho L, et al. Mitochondrial encephalopathy: first Portuguese report of a VARS2 causative variant. JIMD Rep. (2018) 42:113–9. doi: 10.1007/8904_2018_89
14. Ruzman L, Kolic L, Radic Nisevic J, Ruzic Barsic A, Skarpa Prpic I, Prpic I, et al. A novel VARS2 gene variant in a patient with epileptic encephalopathy. Upsala J Med Sci. (2019) 124:273–7. doi: 10.1080/03009734.2019.1670297
15. Begliuomini C, Magli G, Rocco Di M, Santorelli FM, Cassandrini D, Nesti C, et al. VARS2-linked mitochondrial encephalopathy: two case reports enlarging the clinical phenotype. BMC Med Genet. (2019) 20:77. doi: 10.1186/s12881-019-0798-7
16. Feichtinger RG, Mucha BE, Hengel H, Hengel H, Orfi Z, Makowski CH, et al. Biallelic variants in the transcription factor PAX7 are a new genetic cause of myopathy. Genet Med. (2019) 21:2521–31. doi: 10.1038/s41436-019-0532-z
17. Feichtinger RG, Zimmermann F, Mayr JA, Neureiter D, Hauser-Kronberger C, Schillin FH, et al. Low aerobic mitochondrial energy metabolism in poorly- or undifferentiated neuroblastoma. BMC Cancer. (2010) 10:149. doi: 10.1186/1471-2407-10-149
18. Rustin P, Chretien D, Bourgeron T, Gérard B, Rötig A, Saudubray JM, et al. Biochemical and molecular investigations in respiratory chain deficiencies. Clin Chim Acta. (1994) 228:35–51. doi: 10.1016/0009-8981(94)90055-8
19. Bookelman H, Trijbels JM, Sengers RC, Janssen AJ, Veerkamp JH, Stadhouders AM. Pyruvate oxidation in rat and human skeletal muscle mitochondria. Biochem Med. (1978) 20:395–403. doi: 10.1016/0006-2944(78)90089-3
20. Feichtinger RG, Neureiter D, Kemmerling R, Mayr JA, Kiesslich T, Kofler B. Low VDAC1 expression is associated with an aggressive phenotype and reduced overall patient survival in cholangiocellular carcinoma. Cells. (2019) 8:539. doi: 10.3390/cells8060539
21. Feichtinger RG, Schäfer G, Seifarth C, Mayr JA, Kofler B, Klocker H. Reduced levels of ATP synthase subunit ATP5F1A correlate with earlier-onset prostate cancer. Oxid Med Cell Longev. (2018) 2018:1347174. doi: 10.1155/2018/1347174
22. Feichtinger RG, Weis S, Mayr JA, Zimmermann FA, Bogner B, Sperl W, et al. Alterations of oxidative phosphorylation in meningiomas and peripheral nerve sheath tumors. Neu Onc. (2016) 18:184–94. doi: 10.1093/neuonc/nov105
Keywords: VARS2 gene, oxidative phosphorylation, mitochondriopathy, hyperlactatemia, lethal hypertrophic cardiomyopathy, pulmonary hypertension
Citation: Kušíková K, Feichtinger RG, Csillag B, Kalev OK, Weis S, Duba H-C, Mayr JA and Weis D (2021) Case Report and Review of the Literature: A New and a Recurrent Variant in the VARS2 Gene Are Associated With Isolated Lethal Hypertrophic Cardiomyopathy, Hyperlactatemia, and Pulmonary Hypertension in Early Infancy. Front. Pediatr. 9:660076. doi: 10.3389/fped.2021.660076
Received: 28 January 2021; Accepted: 22 March 2021;
Published: 16 April 2021.
Edited by:
Ronald Wanders, University of Amsterdam, NetherlandsReviewed by:
Ann Saada, Hebrew University of Jerusalem, IsraelEmanuela Bottani, University of Verona, Italy
Copyright © 2021 Kušíková, Feichtinger, Csillag, Kalev, Weis, Duba, Mayr and Weis. This is an open-access article distributed under the terms of the Creative Commons Attribution License (CC BY). The use, distribution or reproduction in other forums is permitted, provided the original author(s) and the copyright owner(s) are credited and that the original publication in this journal is cited, in accordance with accepted academic practice. No use, distribution or reproduction is permitted which does not comply with these terms.
*Correspondence: Denisa Weis, ZGVuaXNhLndlaXNAa2VwbGVydW5pa2xpbmlrdW0uYXQ=
†These authors have contributed equally to this work