- 1Indonesia Research Partnership on Infectious Disease, Jakarta, Indonesia
- 2Tangerang District Hospital, Tangerang, Indonesia
- 3Dr. Kariadi Hospital/Diponegoro University, Semarang, Indonesia
- 4Dr. Sardjito Hospital/Universitas Gadjah Mada, Yogyakarta, Indonesia
- 5National Institute of Health Research and Development, Ministry of Health, Republic of Indonesia, Jakarta, Indonesia
- 6National Institute of Allergy and Infectious Diseases, National Institutes of Health, Bethesda, MD, United States
- 7National Cancer Institute, National Institutes of Health, Bethesda, MD, United States
Determining the causative pathogen(s) of community-acquired pneumonia (CAP) in children remains a challenge despite advances in diagnostic methods. Currently available guidelines generally recommend empiric antimicrobial therapy when the specific etiology is unknown. However, shifts in epidemiology, emergence of new pathogens, and increasing antimicrobial resistance underscore the importance of identifying causative pathogen(s). Although viral CAP among children is increasingly recognized, distinguishing viral from bacterial etiologies remains difficult. Obtaining high quality samples from infected lung tissue is typically the limiting factor. Additionally, interpretation of results from routinely collected specimens (blood, sputum, and nasopharyngeal swabs) is complicated by bacterial colonization and prolonged shedding of incidental respiratory viruses. Using current literature on assessment of CAP causes in children, we developed an approach for identifying the most likely causative pathogen(s) using blood and sputum culture, polymerase chain reaction (PCR), and paired serology. Our proposed rules do not rely on carriage prevalence data from controls. We herein share our perspective in order to help clinicians and researchers classify and manage childhood pneumonia.
Introduction
Pneumonia is the leading infectious cause of death amongst children worldwide. It accounts for more than 138 million new cases and almost one million deaths annually, mostly amongst children under 5 years old (1, 2). Research on pneumonia etiologies conducted from the 1970s through the early 1990s showed 2 bacteria—Streptococcus pneumoniae and Haemophilus influenzae type b (Hib)— cause the majority of fatal pneumonia cases in children, primarily in settings that lack access to basic healthcare such as antibiotics and oxygen therapy (3, 4). The World Health Organization (WHO) used these data to develop a clinical case definition for pneumonia, which deliberately increased sensitivity to ensure that all potential pneumonia cases would receive effective antibiotic therapy (5, 6).
In line with WHO guidelines, practice guidelines from the British Thoracic Society (BTS) in 2011 and Royal College of Paediatrics and Child Health (RCPCH) in 2016 recommend amoxicillin as the first choice for oral antibiotic therapy in children with community-acquired pneumonia (CAP) (7–9). The 2011 Pediatric Infectious Diseases Society/Infectious Diseases Society of America (IDSA/PIDS) guideline recommends narrow-spectrum antibiotics (e.g., amoxicillin or amoxicillin-clavulanate) for most children hospitalized with CAP, and macrolides (azithromycin, clarithromycin, or erythromycin) for presumed atypical pathogens (10). Despite evidence that appropriate antibiotics can be lifesaving, rational selection of antibiotics for pneumonia is hampered by low adherence to existing guidelines and scarcity of point-of-care diagnostics (11, 12). Consequently, healthcare providers, particularly those in low-resource settings, are likely to overtreat non-bacterial pneumonia with antibiotics (11).
Overuse of antibiotics can engender resistance to multiple antibiotic classes as well as toxicity. Recent CAP etiology studies, including the large-scale Pneumonia Etiology Research for Child Health (PERCH) and the Global Approach to Biological Research, Infectious diseases and Epidemics in Low-income countries (GABRIEL) studies, have focused on low-income countries (13, 14). They reveal that viral etiologies of CAP in those settings have likely been underestimated due to prior lack of viral diagnostics, shifting pathogen prevalence associated with widespread deployment of Hib and pneumococcal conjugate vaccines (PCV), improved socioeconomic and nutritional status, a sharp decrease in measles incidence, and increased urbanization (3, 15). Thus, empiric antibiotic treatment algorithms for pediatric CAP may need updating (3, 15).
Identification of specific viral and bacterial causes of pneumonia is hindered by non-specificity of clinical and radiographic findings, as well as co-infection and colonization (16). Nasopharyngeal carriage of respiratory bacteria and viruses known to be associated with pneumonia have been reported in apparently healthy children, obscuring their contribution to pneumonia in many cases (17–19). To address this diagnostic challenge, many pneumonia studies include a control group of healthy children (13, 14, 19, 20). Inclusion of control children reduces over-attribution of disease to non-pathogenic organisms by allowing calculation of an adjusted odds ratio (aOR) for each pathogen and estimation of the population-attributable fraction (13, 19). However, obtaining lower respiratory tract specimens from healthy control children, though ideal for study design, may not be feasible in certain situations (21) and is difficult to accomplish in clinical practice.
Our Approach
In order to interpret data collected for a pediatric pneumonia study in Indonesia, rules for assessing relevance of identified organisms were needed. The study, known as “Partnerships for Enhanced Engagement in Research - Pneumonia in Pediatrics (PEER-PePPeS)” was a multi-site observational cohort study that sought to estimate etiologies of CAP amongst children aged 2–59 months of age in Indonesia, where PCV and Hib vaccines are not mandatory (https://sites.nationalacademies.org/PGA/PEER/PEERscience/PGA_174220) and all CAP cases are treated with empiric antibiotics per national guidelines (22). PEER-PePPeS aimed to identify the causative pathogen(s) and etiologic distribution of pneumonia cases via comprehensive diagnostic testing across three research sites. Diagnostics were selected from assays commonly performed in clinical practice, with addition molecular and paired-serology tests in PEER-PePPeS for a comprehensive approach.
Current literature was considered during formulation of the rules. PubMed search terms “children,” “pneumonia,” “bacterial pathogen,” “colonization” or “carriage,” “viral pathogen,” “innocent bystanders,” “specimen type,” “culture,” “molecular” and “serology” were used. Additionally, large-scale, case-control studies conducted in developing countries, such as PERCH and GABRIEL, and expert opinion in Indonesia were taken into account. A conceptual summary of the proposed rules is depicted in Figure 1 and further explained in this paper.
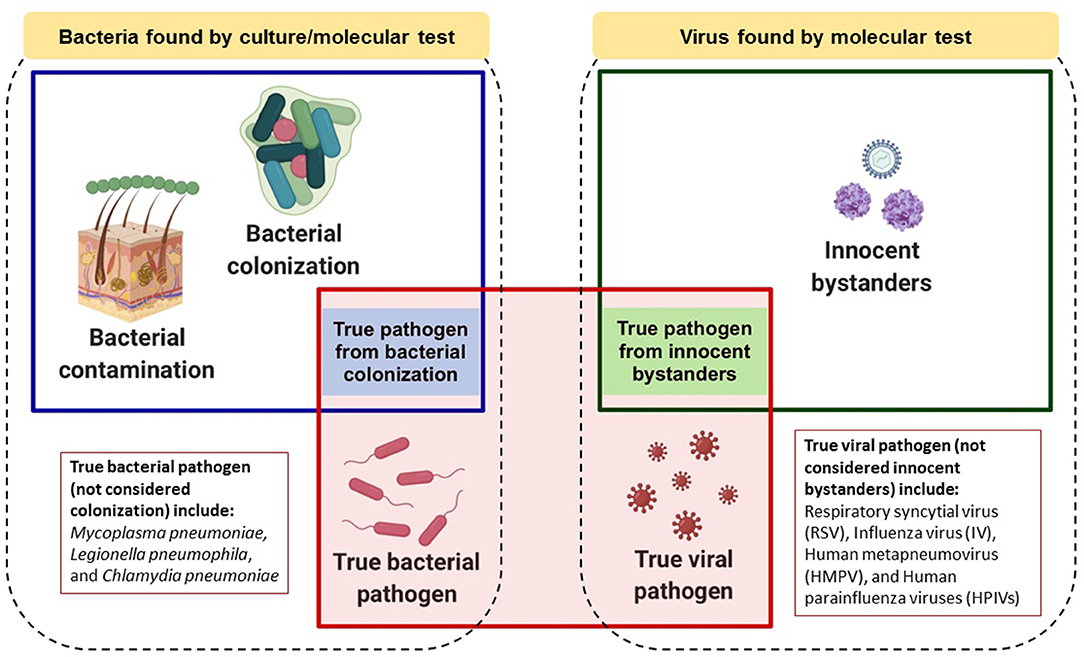
Figure 1. Conceptual model of roles that bacteria or viruses detected by laboratory testing might play in childhood pneumonia. Dotted boxes encompass all bacteria or viruses found by microbiologic and/or molecular testing. Blue or green boxes represent bacteria or viruses, respectively, which could be causative pathogens. The red box represents true causative pathogens identified by the proposed “rules.” Image was created in Biorender.com.
Specimen Types and Detection Methods
Determination of pneumonia etiology is ideally based upon isolation of causative pathogens, or characteristic histology in the case of non-infectious pneumonia, at the site of disease (23). However, effective sampling from the lower respiratory tract (LRT) is frequently not clinically feasible. Upper respiratory tract (URT) specimens such as nasopharyngeal (NP)/oropharyngeal (OP) swabs must often serve as a proxy. These URT specimens can be particularly difficult to interpret due to confounding by asymptomatic carriage of non-pathogenic organisms (24).
The nasopharynx is a complex and dynamic environment; any organism that could cause pneumonia may also be a colonizer, including Streptococcus pneumoniae, Haemophilus influenzae, Staphylococcus aureus, and Moraxella catarrhalis (17, 25, 26). Furthermore, asymptomatic NP carriage may responsible for transmission and often precedes LRT infection (27). Klebsiella pneumoniae is an opportunistic gram-negative bacteria that can colonize the NP, although it more commonly colonizes the gut (28, 29). Studies have reported a lower prevalence of NP carriage in children (range 1.4–7%) than in adults (20–32.5%), which may be related to poor hygiene (29–31). However, in terms of its pathogenicity, the presence of K. pneumonia in symptomatic children should be considered a pathogen (28, 32).
Previous studies have suggested that children with pneumonia have a higher pathogen density in the URT than children without pneumonia, though there is heterogeneity by study and pathogen (25, 33). Likewise, respiratory viruses found in respiratory specimens by molecular tests during acute disease may be “innocent bystanders” without a causal role (34). The presence of a respiratory virus, such as human Bocavirus (hBoV), Adenovirus (AdV), non-SARS human Coronavirus (hCoV), Enterovirus (EV), and Rhinovirus (RV), in the URT of healthy children has been observed in several studies (13, 19, 20). This could represent carriage, nascent infection, prolonged shedding, or subclinical infection (18, 34, 35). Some respiratory viruses, such as hBoV, can shed for several months after an illness (36).
Secretions from the LRT are also diagnostically helpful as they originate at the site of infection (24). However, children have difficulty expectorating sputum, leading to frequent use of induction techniques to obtain LRT specimens. Sputum induction is performed by administration of hypertonic saline via nebulizer, followed by percussion of the chest wall to mobilize secretions (21). The presence of <10 squamous epithelial cells (SECs) and significant number of polymorphonuclear cells/PMNs (for which criteria vary among studies) per low-power field (100× magnification) have long been regarded as ideal (24, 37). However, the criterion of large numbers of PMNs has been questioned given that pneumonia may not necessarily be associated with production of purulent sputum (38). Recent studies showed <10 SECs is the key quality measure in children since it corresponds with low quantities of NP/OP flora (38, 39). However, interpretation should take into account that contamination may occur even with meticulous technique (24).
Microbiologic analysis of LRT specimens, including induced sputum, by techniques such as culture and gram stain can reveal an etiology and guide targeted management (21, 40). Polymerase chain reaction (PCR) for bacterial DNA by is particularly helpful in culture-negative cases, highlighting the complementary role of molecular methods (41). Results should be interpreted in the context of immune status, as opportunistic pathogens such as Pneumocystis jirovecii and cytomegalovirus (CMV) are often pathogenic in children with HIV but not in HIV-uninfected children (42).
Etiologic assessment of pneumonia may also be done using blood samples, which may be easier to collect than LRT specimens and NP swabs. As blood is normally sterile, culture of an organism is generally considered indicative of active infection (24). However, prevalence of blood culture contamination may be as high as 2–3% (43) and bacteremia only occurs in 2.1% of pneumonia cases (44). Thus interpretation must account for the possibility of contamination, which we have incorporated in our pathogen identification rules. Blood specimens may also undergo molecular testing. This is especially helpful for some organisms. For instance, PCR detection of S. pneumoniae in blood may be associated with invasive pneumococcal disease (44, 45).
Antigen detection on several specimen types, including urine, pleural fluid and NP/OP swabs, is commonly used for point-of-care (PoC) diagnosis (23). Although antigen detection tests are only available for select organisms, they can play a critical role in treatment decisions. For example, rapid recognition of S. pneumonia or Legionella pneumophila, can guide antibiotic selection. A limitation of antigen detection assays is their reliance on detectable quantities of antigen; performance is thus suboptimal compared with molecular tests (45).
Serological testing of paired acute and convalescent samples, typically collected at least 7 days apart, can also be used for pathogen identification (23). Serology is most helpful for retrospective confirmation, especially for fastidious bacteria, and may reveal the accuracy of a previous empiric diagnosis (45). It may be particularly useful for viruses with prolonged NP shedding or high prevalence in a control population (24). IgM only tests are less sensitive and specific than IgG and IgM antibody titers from paired specimens as IgM kinetics vary (23) and IgM seroconversion might not occur in the setting of repeat infection (46). A serologic diagnosis generally requires a 2-fold or greater increase in titers between paired serum specimens. Reliance on convalescent specimens means that actionable results are not typically available during acute illness, limiting application in the acute-care setting (47).
Proposed Rules to Assess Bacterial Pathogens
Our rules to determine the bacterial etiology of pneumonia incorporate commonly collected specimens and routine laboratory assays. Blood culture and whole-blood PCR are considered first-tier due to high specificity, followed by induced sputum culture and molecular testing (PCR) of NP/OP swabs or induced sputum specimens (45). Serological evaluation of paired acute and convalescent specimens is also used for diagnosis, especially of atypical bacterial agents (48) for which PCR showed low sensitivity and poor concordance compared with the paired serology (49). The proposed pathogen determination rules are shown in Table 1.
All bacteria detected by blood culture, except for contaminants, are considered potential pathogens. The following were classified as contaminants based on relatedness to community-acquired pneumonia: Coagulase-negative staphylococci, Micrococcus spp., Propionibacterium spp., Alpha-hemolytic streptococci (except pneumococcus, Streptococcus anginosus, and Streptococcus mitis), Enterococcus spp., Corynebacterium spp. (diphtheroids), Bacillus spp. (except Bacillus anthracis), Pseudomonas spp. (except Pseudomonas aeruginosa), Stomatococcus, Aeroccocus, Neiserria subflava, Veillonella spp., other environmental non-fermenting Gram-negative rods, and Candida spp. (14, 20). Any bacteria found in whole blood by PCR and not listed as a contaminant is classified as a pathogen (20).
Good quality sputum specimens should undergo gram stain and bacterial culture simultaneously to optimize microbiological yield (21, 50). Gram stain results that are consistent with sputum culture can define the microbiological diagnosis of pneumonia (21). The gram stain should be interpreted as follows: Gram-positive lancet-shaped diplococci (GPDC) suggest S. pneumoniae; Gram-positive diplococci (GPDC) or cocci in chains suggest Streptococcus pyogenes; Gram-positive cocci in clusters (GPC-cluster) suggest S. aureus; Gram-negative coccobacilli (GNCB) suggest H. influenzae, Bordetella pertussis or Acinetobacter baumannii; Gram-negative diploccoci (GNDC) suggest M. catarrhalis; large Gram-negative rods (GNR-large) suggest Klebsiella pneumoniae or Escherichia coli; and small Gram-negative rods (GNR-small) suggest P. aeruginosa (40).
For semiquantitative measurement on sputum culture, predominant organisms were identified and quantified according to the furthest quadrant with visible colonies (first quadrant, scanty; second quadrant, 1+; third quadrant, 2+; fourth quadrant, 3+). Organisms isolated in quantities of 2+ or 3+ and with compatible Gram stain morphotype are regarded as pathogens (38). For quantitative culture, colony counts of <104/ml suggest contamination, counts of 104-105/ml are indeterminate, and counts of >105/ml of a major isolate suggest a potential pathogen (51). If the organism grows on culture, conventional biochemical tests (e.g., catalase or coagulase) or automated system evaluations, including rapid fluorescence-based methodology, can be performed to identify the organism (52, 53).
Culture yield depends on sputum quality, pathogen burden, transport time, storage period, nutrients, and incubation conditions that maintain viability (50, 54). Receipt of antibiotics before specimen collection may prevent growth on culture (41) as well as affect gram stain morphology. In this setting, culture-negative bacteria should remain in the differential and be sought by a molecular method targeting DNA (41). For bacteria identified by PCR of respiratory specimens (NP, OP or induced sputum), careful consideration must be given to distinguishing colonizers from true pathogens (14, 17).
For bacteria not considered NP colonizers (e.g., Mycoplasma pneumoniae), any positive PCR indicates a definite pathogen (13, 19). For bacteria than can be both a colonizer and pathogen, higher density has been associated with pathogenic status. For example, higher density is associated with causality and considered significant if the copy number exceeds 6.9 log10 copies/mL for S. pneumoniae (26), 5.9 log10 copies/mL for H. influenzae (25), and 7.5 log10 copies/mL for S. aureus (33). Copy number cut-offs were transformed to corresponding Ct values per our assay; the Ct cut-off for S. pneumonia was ≤ 24.2; H. influenzae ≤ 30.3 and S. aureus ≤ 30.2. We nonetheless recognize that Ct value cut-offs may differ depending on the PCR system used. Lower copy numbers are considered indicative of colonization. However, studies have failed to identify an association between density and pathogen-confirmed pneumonia for M. catarrhalis, indicating that PCR alone cannot be used to determine its role in childhood pneumonia (25, 33).
Despite limited use in acute management of pneumonia, serological techniques are heavily utilized in clinical research (45). In comprehensive epidemiology studies, serology may identify infections missed by other methods such as culture and PCR (45) and could also distinguish colonization since nasopharyngeal carriage does not cause seroconversion between paired sera (55). A study in children over 2-years old throughout Asia demonstrated that a combination of paired serology, direct antigen and DNA tests increases diagnostic yield of atypical pathogens such as M. pneumoniae, Chlamydia pneumoniae, and Legionella pneumophila (56).
For interpretation of paired serologic testing, the following are considered indicative of infection: (a) Initial detection of specific antibodies after a prior negative sample (seroconversion), or (b) A two to four-fold increase, depending on the test, in antibody titers in the convalescent specimen (47). Serologic confirmation of pneumonia etiology is especially useful in prolonged hospitalization/ICU admission cases (57), and epidemiologic research or disease surveillance, as opposed to the acute clinical setting (45).
At present, few antigen detection assays are available for diagnosis of bacterial causes of childhood pneumonia. Notable examples include antigen detection assays for S. pneumoniae and L. pneumophila (45). The immunochromatographic urine test used for pneumococcal disease detects the polysaccharide cell wall antigen (58). Unfortunately, the test is not reliable in children since it cannot distinguish between carriage and pathogenic pneumococcus (59). Likewise, detection of soluble Legionella antigen in urine is limited to L. pneumophila serogroup 1 (60). Given their low sensitivity compared with PCR testing, use of these antigen assays in children is not recommended (45) and not included in our proposed rules.
Proposed Rules to Assess Viral Pathogens
Detection of viruses by direct immunofluorescence microscopy and isolation in cell culture have long been considered the “gold standard” of respiratory viral pathogen diagnosis (45). However, viral culture is resource-intensive, requires maintenance of cell-lines over long time periods, and poses exposure risk to laboratory personnel (61). Direct fluorescent-antibody (DFA) and immunofluorescent-antibody (IFA) assays of cell smears to detect specific viruses are commercially available and use standardized reagents (e.g., FLUA/B, PIVs 1 to 3, ADV, hMPV, and RSV), but are labor-intensive, require a fluorescence microscope and skilled microscopist, and are susceptible to reader error (62). Therefore, these “gold standard” methods are being replaced by more sensitive, high-throughput, and less labor-intensive molecular tests (45, 48).
A PCR test on NP/OP swabs or induced sputum with Ct (cycle threshold) value ≤ 40 is considered diagnostic for viruses well-known to cause pneumonia, including Respiratory syncytial virus (RSV), Influenza virus (IV), Human metapneumovirus (HMPV), and Human parainfluenza viruses (HPIVs) (13, 19). However, some viruses normally detected in healthy children or that have prolonged/intermittent shedding (innocent bystanders), including RV (63), AdV (64), hBoV (65), non-SARS hCoV (13), and EV (66), merit additional justification. To avoid attributing disease to innocent bystanders, we consider only those with high viral load (Ct value <24) as true pathogens (18, 67).
Viruses confirmed by serodiagnosis are also regarded as causative pathogens. Serology may be particularly useful for identifying respiratory viral infections that trigger secondary bacterial pneumonia as the virus may no longer be detectable in respiratory specimens (45). Addition of serology to PCR evaluation increases positive diagnostic yield (68). In one study, 29 of 88 cases of viral pneumonia were diagnosed by serology alone (69). Serology is a helpful adjunct to the PCR in assessing etiologic contribution, but may not reveal exact timing (68).
Antigen testing for respiratory specimens is attractive as a potential PoC diagnostic test for viral causes of childhood pneumonia. It has been used for detection of seasonal influenza and RSV (45). However, performance is suboptimal; sensitivity of rapid diagnostic tests ranges from 10 to 96% for influenza (70, 71) and 71–95% for commercial RSV (72, 73). Variable sensitivity is not surprising, as low viral loads would predispose to false negatives. Furthermore, low pre-test probability outside respiratory virus season undermines predictive utility (62). Antigen destruction may occur with freezing and on repository swabs, which can also decrease accuracy (74). Based on sensitivity, antigen testing is inferior to PCR for detection of viruses causing pneumonia (62) and is not included in our rules.
Conclusion
In conclusion, identifying bacterial and viral etiologies of pneumonia in children is necessary yet challenging. NP colonization and prolonged shedding of innocent bystander respiratory viruses may obscure assay interpretation. Our rules employ culture, molecular, and serology testing for determination of the pathogen(s) causing pneumonia in children. These rules will support effective clinical management of and research on childhood pneumonia. Our proposed rules are advantageous in their comprehensive approach, which may increase accuracy of diagnosis. This is very useful for research and well-equipped facilities. However, cost and complexity may present barriers in many laboratories and hospitals, limiting feasibility in low-resource settings. Additionally, reliance on paired serum (acute-convalescent) for assessment could delay diagnosis. Further studies should be performed to assess the utility of these proposed rules for reducing antimicrobial use and resistance rates, as well as correlation with biomarkers used to guide treatment (e.g., procalcitonin, C-reactive protein).
Data Availability Statement
The original contributions presented in the study are included in the article/supplementary material, further inquiries can be directed to the corresponding author/s.
Author Contributions
YM, AM, C-YL, HK, and DL prepared the concept and design of the study. YM, AM, HK, and C-YL reviewed and analyzed the literature. YM and AM drafted the manuscript. YM, AM, HK, C-YL, AK, and DL reviewed the draft of the manuscript, provided critical insights, edited and prepared the final version of the manuscript. YM, AM, DL, HF, AA, NL, MK, HK, AK, and C-YL analyzed, reviewed, and edited the manuscript's final version and approved it for publication. All authors contributed to the article and approved the submitted version.
Funding
This manuscript has been funded in whole or in part with MoH Indonesia, National Academy of Sciences (Sub-Grant Number: 2000007599), and Federal funds from the NIAID, NIH, under contract Nos. HHSN261200800001E and HHSN261201500003I.
Disclaimer
The content of this publication does not necessarily reflect the views or policies of the Department of Health and Human Services, nor does mention of trade names, commercial products, or organizations imply endorsement by the U.S. Government.
Conflict of Interest
The authors declare that the research was conducted in the absence of any commercial or financial relationships that could be construed as a potential conflict of interest.
Acknowledgments
We are grateful to Dr. H. Clifford Lane, National Institute of Allergy and Infectious Diseases (NIAID) Deputy Director for Clinical Research and Special Project for his support and scientific insight. We also thank the Partnership for Enhanced Engagement in Research (PEER) - Pneumonia in Pediatric Patients Study (PePPeS) team, Indonesia Research Partnership on Infectious Diseases (INA-RESPOND) Network, Tangerang District Hospital, Kariadi Hospital and Sardjito Hospital for their operational support and technical assistance.
References
1. McAllister DA, Liu L, Shi T, Chu Y, Reed C, Burrows J, et al. Global, regional, and national estimates of pneumonia morbidity and mortality in children younger than 5 years between 2000 and 2015: a systematic analysis. Lancet Glob Heal. (2019) 7:e47–57. doi: 10.1016/S2214-109X(18)30408-X
2. Liu L, Oza S, Hogan D, Chu Y, Perin J, Zhu J, et al. Global, regional, and national causes of under-5 mortality in 2000–15: an updated systematic analysis with implications for the sustainable development goals. Lancet. (2016) 388:3027–35. doi: 10.1016/S0140-6736(16)31593-8
3. Levine OS, O'Brien KL, Deloria-Knoll M, Murdoch DR, Feikin DR, DeLuca AN, et al. The pneumonia etiology research for child health project: a 21st century childhood pneumonia etiology study. Clin Infect Dis. (2012) 54 (suppl_2):S93–101. doi: 10.1093/cid/cir1052
4. Scott JAG, Brooks WA, Peiris JSM, Holtzman D, Mulholland EK. Pneumonia research to reduce childhood mortality in the developing world. J Clin Invest. (2008) 118:1291–300. doi: 10.1172/JCI33947
5. Feikin DR, Hammitt LL, Murdoch DR, O'Brien KL, Scott JAG. The enduring challenge of determining pneumonia etiology in children: considerations for future research priorities. Clin Infect Dis. (2017) 64 (suppl_3):S188–96. doi: 10.1093/cid/cix143
6. World Health Organisation. Revised WHO Classification and Treatment of Childhood Pneumonia at Health Facilities: Evidence Summaries. Geneva: World Health Organisation (2014).
8. Mathur S, Fuchs A, Bielicki J, Van Den Anker J, Sharland M. Antibiotic use for community-acquired pneumonia in neonates and children: WHO evidence review. Paediatr Int Child Health. (2018) 38 (Suppl. 1):S66–75. doi: 10.1080/20469047.2017.1409455
9. Harris M, Clark J, Coote N, Fletcher P, Harnden A, McKean M, et al. British Thoracic Society guidelines for the management of community acquired pneumonia in children: update 2011. Thorax. (2011) 66 (Suppl. 2):ii1 LP–ii23. doi: 10.1136/thoraxjnl-2011-200598
10. Bradley JS, Byington CL, Shah SS, Alverson B, Carter ER, Harrison C, et al. Pediatric Infectious Diseases Society and the Infectious Diseases Society of America. The management of community-acquired pneumonia in infants and children older than 3 months of age: clinical practice guidelines by the Pediatric Infectious Diseases Soci. Clin Infect Dis. (2011) 53:e25–76. doi: 10.1093/cid/cir531
11. Ginsburg AS, Klugman KP. Vaccination to reduce antimicrobial resistance. Lancet Glob Heal. (2017) 5:e1176–7. doi: 10.1016/S2214-109X(17)30364-9
12. Tannous R, Haddad RN, Torbey PH. Management of community-acquired pneumonia in pediatrics: adherence to clinical guidelines. Front Pediatr. (2020) 8:302. doi: 10.3389/fped.2020.00302
13. Bénet T, Sánchez Picot V, Messaoudi M, Chou M, Eap T, Wang J, et al. Microorganisms associated with pneumonia in children <5 years of age in developing and emerging countries: the GABRIEL pneumonia multicenter, prospective, case-control study. Clin Infect Dis. (2017) 65:604–12. doi: 10.1093/cid/cix378
14. O'Brien KL, Baggett HC, Brooks WA, Feikin DR, Hammitt LL, Higdon MM, et al. Causes of severe pneumonia requiring hospital admission in children without HIV infection from Africa and Asia: the PERCH multi-country case-control study. Lancet. (2019) 394:757–79. doi: 10.1016/S0140-6736(19)30721-4
15. Rhedin SA, Eklundh A, Ryd-Rinder M, Naucler P, Mårtensson A, Gantelius J, et al. Introducing a new algorithm for classification of etiology in studies on pediatric pneumonia: protocol for the trial of respiratory infections in children for enhanced diagnostics study. JMIR Res Protoc. (2019) 8:e12705. doi: 10.2196/12705
16. Simoes EAF, Cherian T, Chow J, Shahid-Salles SA, Laxminarayan R, John TJ. Acute respiratory infections in children. In: Jamison DT, Breman JG, Measham AR, Alleyne G, Claeson M, Evans DB, et al., editors. Disease Control Priorities in Developing Countries. 2nd ed. London: The World Bank and Oxford University Press (2006). p. 483–97.
17. Dunne EM, Murad C, Sudigdoadi S, Fadlyana E, Tarigan R, Indriyani SAK, et al. Carriage of Streptococcus pneumoniae, Haemophilus influenzae, Moraxella catarrhalis, and Staphylococcus aureus in Indonesian children: a cross-sectional study. PLoS ONE. (2018) 13:e0195098. doi: 10.1371/journal.pone.0195098
18. Jansen RR, Wieringa J, Koekkoek SM, Visser CE, Pajkrt D, Molenkamp R, et al. Frequent detection of respiratory viruses without symptoms: toward defining clinically relevant cutoff values. J Clin Microbiol. (2011) 49:2631–6. doi: 10.1128/JCM.02094-10
19. Bhuiyan MU, Snelling TL, West R, Lang J, Rahman T, Granland C, et al. The contribution of viruses and bacteria to community-acquired pneumonia in vaccinated children: a case-control study. Thorax. (2019) 74:261–9. doi: 10.1136/thoraxjnl-2018-212096
20. Jain S, Self WH, Wunderink RG, Fakhran S, Balk R, Bramley AM, et al. Community-acquired pneumonia requiring hospitalization among U.S. Adults. N Engl J Med. (2015) 373:415–27. doi: 10.1056/NEJMoa1500245
21. Lahti E, Peltola V, Waris M, Virkki R, Rantakokko-Jalava K, Jalava J, et al. Induced sputum in the diagnosis of childhood community-acquired pneumonia. Thorax. (2009) 64:252 LP−7. doi: 10.1136/thx.2008.099051
22. Subuh M. Pedoman Tatalaksana Pneumonia Balita. Jakarta Kementeri Kesehat Republik Indones (2015).
23. Loens K, Van Heirstraeten L, Malhotra-Kumar S, Goossens H, Ieven M. Optimal sampling sites and methods for detection of pathogens possibly causing community-acquired lower respiratory tract infections. J Clin Microbiol. (2009) 47:21–31. doi: 10.1128/JCM.02037-08
24. Hammitt LL, Murdoch DR, Scott JAG, Driscoll A, Karron RA, Levine OS, et al. Specimen collection for the diagnosis of pediatric pneumonia. Clin Infect Dis. (2012) 54 (Suppl. 2):S132–9. doi: 10.1093/cid/cir1068
25. Park DE, Baggett HC, Howie SRC, Shi Q, Watson NL, Brooks WA, et al. Colonization density of the upper respiratory tract as a predictor of pneumonia-Haemophilus influenzae, Moraxella catarrhalis, Staphylococcus aureus, and Pneumocystis jirovecii. Clin Infect Dis. (2017) 64 (suppl_3):S328–36. doi: 10.1093/cid/cix104
26. Baggett HC, Watson NL, Deloria Knoll M, Brooks WA, Feikin DR, Hammitt LL, et al. Density of upper respiratory colonization with Streptococcus pneumoniae and its role in the diagnosis of pneumococcal pneumonia among children aged <5 years in the PERCH study. Clin Infect Dis. (2017) 64 (suppl_3):S317–27. doi: 10.1093/cid/cix100
27. Adegbola RA, DeAntonio R, Hill PC, Roca A, Usuf E, Hoet B, et al. Carriage of Streptococcus pneumoniae and other respiratory bacterial pathogens in low and lower-middle income countries: a systematic review and meta-analysis. PLoS ONE. (2014) 9:e103293. doi: 10.1371/journal.pone.0103293
28. Dorman MJ, Short FL. Klebsiella pneumoniae: When a Colonizer Turns Bad. London: Nature Publishing Group (2017).
29. Dao TT, Liebenthal D, Tran TK, Vu BNT, Nguyen DNT, Tran HKT, et al. Klebsiella pneumoniae oropharyngeal carriage in rural and urban Vietnam and the effect of alcohol consumption. PLoS ONE. (2014) 9:e91999. doi: 10.1371/journal.pone.0091999
30. Lima ABM, Leão LSN de O, Oliveira LS da C, Pimenta FC. Nasopharyngeal Gram-negative bacilli colonization in Brazilian children attending day-care centers. Braz J Microbiol. (2010) 41:24–7. doi: 10.1590/S1517-83822010000100005
31. Farida H, Severin JA, Gasem MH, Keuter M, van den Broek P, Hermans PWM, et al. Nasopharyngeal carriage of Klebsiella pneumoniae and other Gram-negative bacilli in pneumonia-prone age groups in Semarang, Indonesia. J Clin Microbiol. (2013) 51:1614–6. doi: 10.1128/JCM.00589-13
32. Paczosa MK, Mecsas J. Klebsiella pneumoniae: going on the offense with a strong defense. Microbiol Mol Biol Rev. (2016) 80:629–61. doi: 10.1128/MMBR.00078-15
33. Nathan AM, Teh CSJ, Jabar KA, Teoh BT, Tangaperumal A, Westerhout C, et al. Bacterial pneumonia and its associated factors in children from a developing country: A prospective cohort study. PLoS ONE. (2020) 15:e0228056. doi: 10.1371/journal.pone.0228056
34. Shi T, Arnott A, Semogas I, Falsey AR, Openshaw P, Wedzicha JA, et al. The etiological role of common respiratory viruses in acute respiratory infections in older adults: a systematic review and meta-analysis. J Infect Dis. (2020) 222 (Suppl_7):S563–9. doi: 10.1093/infdis/jiy662
35. Praznik A, Vinšek N, Prodan A, Erčulj V, Pokorn M, Mrvič T, et al. Risk factors for bronchiolitis severity: a retrospective review of patients admitted to the university hospital from central region of Slovenia. Influenza Other Respi Viruses. (2018) 12:765–71. doi: 10.1111/irv.12587
36. von Linstow M-L, Høgh M, Høgh B. Clinical and epidemiologic characteristics of human bocavirus in Danish infants: results from a prospective birth cohort study. Pediatr Infect Dis J. (2008) 27:897–902. doi: 10.1097/INF.0b013e3181757b16
37. Del Rio-Pertuz G, Gutiérrez JF, Triana AJ, Molinares JL, Robledo-Solano AB, Meza JL, et al. Usefulness of sputum gram stain for etiologic diagnosis in community-acquired pneumonia: a systematic review and meta-analysis. BMC Infect Dis. (2019) 19:403. doi: 10.1186/s12879-019-4048-6
38. Murdoch DR, Morpeth SC, Hammitt LL, Driscoll AJ, Watson NL, Baggett HC, et al. Microscopic analysis and quality assessment of induced sputum from children with pneumonia in the PERCH study. Clin Infect Dis. (2017) 64 (suppl_3):S271–9. doi: 10.1093/cid/cix083
39. Murdoch DR, Morpeth SC, Hammitt LL, Driscoll AJ, Watson NL, Baggett HC, et al. The diagnostic utility of induced sputum microscopy and culture in childhood pneumonia. Clin Infect Dis. (2017) 64 (suppl_3):S280–8. doi: 10.1093/cid/cix090
40. Fukuyama H, Yamashiro S, Kinjo K, Tamaki H, Kishaba T. Validation of sputum Gram stain for treatment of community-acquired pneumonia and healthcare-associated pneumonia: a prospective observational study. BMC Infect Dis. (2014) 14:534. doi: 10.1186/1471-2334-14-534
41. Lleo MM, Ghidini V, Tafi MC, Castellani F, Trento I, Boaretti M. Detecting the presence of bacterial DNA by PCR can be useful in diagnosing culture-negative cases of infection, especially in patients with suspected infection and antibiotic therapy. FEMS Microbiol Lett. (2014) 354:153–60. doi: 10.1111/1574-6968.12422
42. Punpanich W, Groome M, Muhe L, Qazi SA, Madhi SA. Systematic review on the etiology and antibiotic treatment of pneumonia in human immunodeficiency virus-infected children. Pediatr Infect Dis J. (2011) 30:e192–202. doi: 10.1097/INF.0b013e31822d989c
43. Weddle G, Jackson MA, Selvarangan R. Reducing blood culture contamination in a pediatric emergency department. Pediatr Emerg Care. (2011) 27:179–81. doi: 10.1097/PEC.0b013e31820d652b
44. Shah SS, Dugan MH, Bell LM, Grundmeier RW, Florin TA, Hines EM, et al. Blood cultures in the emergency department evaluation of childhood pneumonia. Pediatr Infect Dis J. (2011) 30:475–9. doi: 10.1097/INF.0b013e31820a5adb
45. Murdoch DR, O'Brien KL, Driscoll AJ, Karron RA, Bhat N. Laboratory methods for determining pneumonia etiology in children. Clin Infect Dis. (2012) 54 (Suppl. 2):S146–52. doi: 10.1093/cid/cir1073
46. Lin L-J, Chang F-C, Chi H, Jim W-T, Tsung-Ning Huang D, Kung Y-H, et al. The diagnostic value of serological studies in pediatric patients with acute Mycoplasma pneumoniae infection. J Microbiol Immunol Infect. (2020) 53:351–6. doi: 10.1016/j.jmii.2018.09.001
47. Wellinghausen NA, HM EM, Fingerle V. MiQ Immunological Methods for the Detection of Infectious Diseases. Munich: Munich Dustri Verlag Dr Karl Feistle (2016).
48. Torres A, Lee N, Cilloniz C, Vila J, Van der Eerden M. Laboratory diagnosis of pneumonia in the molecular age. Eur Respir J. (2016) 48:1764–78. doi: 10.1183/13993003.01144-2016
49. Herrera M, Aguilar YA, Rueda ZV, Muskus C, Vélez LA. Comparison of serological methods with PCR-based methods for the diagnosis of community-acquired pneumonia caused by atypical bacteria. J Negat Results Biomed. (2016) 15:3. doi: 10.1186/s12952-016-0047-y
50. Budayanti NS, Suryawan K, Iswari IS, Sukrama DM. The quality of sputum specimens as a predictor of isolated bacteria from patients with lower respiratory tract infections at a tertiary referral hospital, Denpasar, Bali-Indonesia. Front Med. (2019) 6:64. doi: 10.3389/fmed.2019.00064
51. Campbell S, Forbes BA. The clinical microbiology laboratory in the diagnosis of lower respiratory tract infections. J Clin Microbiol. (2011) 49 (9 Suppl.):S30–3. doi: 10.1128/JCM.00789-11
52. Gavin PJ, Warren JR, Obias AA, Collins SM, Peterson LR. Evaluation of the Vitek 2 system for rapid identification of clinical isolates of gram-negative bacilli and members of the family Streptococcaceae. Eur J Clin Microbiol Infect Dis. (2002) 21:869–74. doi: 10.1007/s10096-002-0826-x
54. Lagier J-C, Edouard S, Pagnier I, Mediannikov O, Drancourt M, Raoult D. Current and past strategies for bacterial culture in clinical microbiology. Clin Microbiol Rev. (2015) 28:208–36. doi: 10.1128/CMR.00110-14
55. Don M, Fasoli L, Paldanius M, Vainionpää R, Kleemola M, Räty R, et al. Aetiology of community-acquired pneumonia: serological results of a paediatric survey. Scand J Infect Dis. (2005) 37:806–12. doi: 10.1080/00365540500262435
56. Ngeow Y-F, Suwanjutha S, Chantarojanasriri T, Wang F, Saniel M, Alejandria M, et al. An Asian study on the prevalence of atypical respiratory pathogens in community-acquired pneumonia. Int J Infect Dis. (2005) 9:144–53. doi: 10.1016/j.ijid.2004.06.006
57. Cilloniz C, Martin-Loeches I, Garcia-Vidal C, San Jose A, Torres A. Microbial etiology of pneumonia: epidemiology, diagnosis and resistance patterns. Int J Mol Sci. (2016) 17:2120. doi: 10.3390/ijms17122120
58. Werno AM, Murdoch DR. Medical microbiology: laboratory diagnosis of invasive pneumococcal disease. Clin Infect Dis. (2008) 46:926–32. doi: 10.1086/528798
59. Domínguez J, Blanco S, Rodrigo C, Azuara M, Galí N, Mainou A, et al. Usefulness of urinary antigen detection by an immunochromatographic test for diagnosis of pneumococcal pneumonia in children. J Clin Microbiol. (2003) 41:2161–3. doi: 10.1128/JCM.41.5.2161-2163.2003
60. Reller LB, Weinstein MP, Murdoch DR. Diagnosis of legionella infection. Clin Infect Dis. (2003) 36:64–9. doi: 10.1086/345529
61. Das S, Dunbar S, Tang Y-W. Laboratory diagnosis of respiratory tract infections in children - the state of the art. Front Microbiol. (2018) 9:2478. doi: 10.3389/fmicb.2018.02478
62. Charlton CL, Babady E, Ginocchio CC, Hatchette TF, Jerris RC, Li Y, et al. Practical guidance for clinical microbiology laboratories: viruses causing acute respiratory tract infections. Clin Microbiol Rev. (2019) 32:e00042–18. doi: 10.1128/CMR.00042-18
63. Kieninger E, Fuchs O, Latzin P, Frey U, Regamey N. Rhinovirus infections in infancy and early childhood. Eur Respir J. (2013) 41:443–52. doi: 10.1183/09031936.00203511
64. Kalu SU, Loeffelholz M, Beck E, Patel JA, Revai K, Fan J, et al. Persistence of adenovirus nucleic acids in nasopharyngeal secretions: a diagnostic conundrum. Pediatr Infect Dis J. (2010) 29:746–50. doi: 10.1097/INF.0b013e3181d743c8
65. Christensen A, Kesti O, Elenius V, Eskola AL, Døllner H, Altunbulakli C, et al. Human bocaviruses and paediatric infections. Lancet Child Adolesc Heal. (2019) 3:418–26. doi: 10.1016/S2352-4642(19)30057-4
66. Jiao MMA, Apostol LN, de Quiroz-Castro M, Jee Y, Roque VJ, Mapue M 2nd, et al. Non-polio enteroviruses among healthy children in the Philippines. BMC Public Health. (2020) 20:167. doi: 10.1186/s12889-020-8284-x
67. Goikhman Y, Drori Y, Friedman N, Sherbany H, Keller N, Mendelson E, et al. Adenovirus load correlates with respiratory disease severity among hospitalized pediatric patients. Int J Infect Dis. (2020) 97:145–50. doi: 10.1016/j.ijid.2020.06.010
68. Zhang Y, Sakthivel SK, Bramley A, Jain S, Haynes A, Chappell JD, et al. Serology enhances molecular diagnosis of respiratory virus infections other than influenza in children and adults hospitalized with community-acquired pneumonia. J Clin Microbiol. (2016) 55:79–89. doi: 10.1128/JCM.01701-16
69. Jennings LC, Anderson TP, Beynon KA, Chua A, Laing RTR, Werno AM, et al. Incidence and characteristics of viral community-acquired pneumonia in adults. Thorax. (2008) 63:42–8. doi: 10.1136/thx.2006.075077
70. Smit M, Beynon KA, Murdoch DR, Jennings LC. Comparison of the NOW influenza A & B, NOW flu A, NOW flu B, and directigen flu A+B assays, and immunofluorescence with viral culture for the detection of influenza A and B viruses. Diagn Microbiol Infect Dis. (2007) 57:67–70. doi: 10.1016/j.diagmicrobio.2006.11.003
71. Hurt AC, Alexander R, Hibbert J, Deed N, Barr IG. Performance of six influenza rapid tests in detecting human influenza in clinical specimens. J Clin Virol. (2007) 39:132–5. doi: 10.1016/j.jcv.2007.03.002
72. Selvarangan R, Abel D, Hamilton M. Comparison of BD Directigen EZ RSV and Binax NOW RSV tests for rapid detection of respiratory syncytial virus from nasopharyngeal aspirates in a pediatric population. Diagn Microbiol Infect Dis. (2008) 62:157–61. doi: 10.1016/j.diagmicrobio.2008.05.005
73. Borek AP, Clemens SH, Gaskins VK, Aird DZ, Valsamakis A. Respiratory syncytial virus detection by Remel Xpect, Binax Now RSV, direct immunofluorescent staining, and tissue culture. J Clin Microbiol. (2006) 44:1105–7. doi: 10.1128/JCM.44.3.1105-1107.2006
Keywords: rules, pathogen detection, specimens, bacterial, viral, children pneumonia
Citation: Mardian Y, Menur Naysilla A, Lokida D, Farida H, Aman AT, Karyana M, Lukman N, Kosasih H, Kline A and Lau C-Y (2021) Approach to Identifying Causative Pathogens of Community-Acquired Pneumonia in Children Using Culture, Molecular, and Serology Tests. Front. Pediatr. 9:629318. doi: 10.3389/fped.2021.629318
Received: 12 March 2021; Accepted: 04 May 2021;
Published: 28 May 2021.
Edited by:
Yutaka Yoshii, The Jikei University School of Medicine, JapanReviewed by:
Andrew Conway Morris, University of Cambridge, United KingdomRaymond Nagi Haddad, Assistance Publique Hopitaux De Paris, France
Copyright © 2021 Mardian, Menur Naysilla, Lokida, Farida, Aman, Karyana, Lukman, Kosasih, Kline and Lau. This is an open-access article distributed under the terms of the Creative Commons Attribution License (CC BY). The use, distribution or reproduction in other forums is permitted, provided the original author(s) and the copyright owner(s) are credited and that the original publication in this journal is cited, in accordance with accepted academic practice. No use, distribution or reproduction is permitted which does not comply with these terms.
*Correspondence: Herman Kosasih, aGtvc2FzaWhAaW5hLXJlc3BvbmQubmV0