- 1Department of Physiology, Faculty of Medicine, Universiti Kebangsaan Malaysia Medical Centre, Kuala Lumpur, Malaysia
- 2Centre for Tissue Engineering and Regenerative Medicine, Faculty of Medicine, Universiti Kebangsaan Malaysia Medical Centre, Kuala Lumpur, Malaysia
- 3Future Cytohealth Sdn Bhd, Bandar Seri Petaling, Kuala Lumpur, Malaysia
- 4School of Pharmacy, Faculty of Health and Medical Sciences, Taylor's University, Subang Jaya, Malaysia
- 5School of Biosciences, Faculty of Health and Medical Sciences, Taylor's University, Subang Jaya, Malaysia
- 6Department of Paediatrics, Faculty of Medicine, Universiti Kebangsaan Malaysia Medical Centre, Kuala Lumpur, Malaysia
Mesenchymal stromal cells (MSCs) can be derived from various tissue sources, such as the bone marrow (BMSCs), adipose tissue (ADSCs), umbilical cord (UC-MSCs) and umbilical cord blood (UCB-MSCs). Clinical trials have been conducted to investigate the potential of MSCs in ameliorating neonatal diseases, including bronchopulmonary dysplasia (BPD), intraventricular hemorrhage (IVH) and necrotizing enterocolitis (NEC). In preclinical studies, MSC therapy has been tested for the treatment of various neonatal diseases affecting the heart, eye, gut, and brain as well as sepsis. Up to date, the number of clinical trials using MSCs to treat neonatal diseases is still limited. The data reported thus far positioned MSC therapy as safe with positive outcomes. However, most of these trials are still preliminary and generally smaller in scale. Larger trials with more appropriate controls and a longer follow-up period need to be conducted to prove the safety and efficacy of the therapy more conclusively. This review discusses the current application of MSCs in treating neonatal diseases, its mechanism of action and future direction of this novel therapy, including the potential of using MSC-derived extracellular vesicles instead of the cells to treat various clinical conditions in the newborn.
Introduction
Mesenchymal stromal cells (MSCs) possess several unique properties which render them an ideal candidate for cell-based therapy in various neonatal diseases. MSCs are multipotent and can migrate to the damaged tissues or organs in response to the inflammatory mediators. At the injured sites, the cells can replicate and differentiate to regenerate the loss or damaged tissues. Besides, MSCs also secrete paracrine factors such as growth factors, cytokines and chemokines which diffuse to local tissue environment and interact with the surrounding cells. These paracrine factors can remedy tissue injury and inflammation as well as mediate tissue repair and regeneration by exerting its anti-inflammatory, anti-apoptotic and pro-mitotic effects (1–3).
MSCs are classified into various groups according to the cell source, such as bone marrow-derived MSCs (BMSCs), adipose-derived MSCs (ADSCs), umbilical cord-derived MSCs (UC-MSCs), and umbilical cord blood-derived MSCs (UCB-MSCs) (4). BMSCs were favored by some researchers since the cells are proven to be safe, easily accessible and can be extracted from the patient's bone marrow (autologous) thus minimizing the risk of immune rejection (5, 6). On the other hand, UC-MSCs and UCB-MSCs appear as attractive sources of allogeneic MSCs due to their availability and immune evasive nature (7–9). Generally, the procedure of BMSC preparation is more time consuming and costlier compared to UC-MSCs and UCB-MSCs, thus the use of UC-MSCs and UCB-MSCs may be preferred.
Regardless of the tissue sources, MSCs have low immunogenicity and can suppress the activation of immune cells (10). Therefore, both autologous and allogeneic MSCs can be used clinically as the cells have a very low risk of being rejected by the host immune system. A significant advantage of allogeneic MSCs is that the cells can be developed into an off-the-shelf product that is convenient to be used clinically. Table 1 highlights the advantages and disadvantages of different MSCs.
MSC therapy can be instituted during the first month of life and is reportedly quite safe. MSC therapy has been introduced to treat neonatal diseases such as severe intraventricular hemorrhage (IVH), bronchopulmonary dysplasia (BPD), and necrotizing enterocolitis (NEC) (11–13) (Figure 1). MSCs are more widely used clinically to treat neonatal diseases as the cells do not have ethical concerns and have a very low risk of tumor formation compared to the embryonic stem cells (ESCs) and induced pluripotent stem cells (iPSCs). In preclinical studies, MSCs have been experimented to treat congenital heart, eye, gut and brain diseases as well as sepsis.
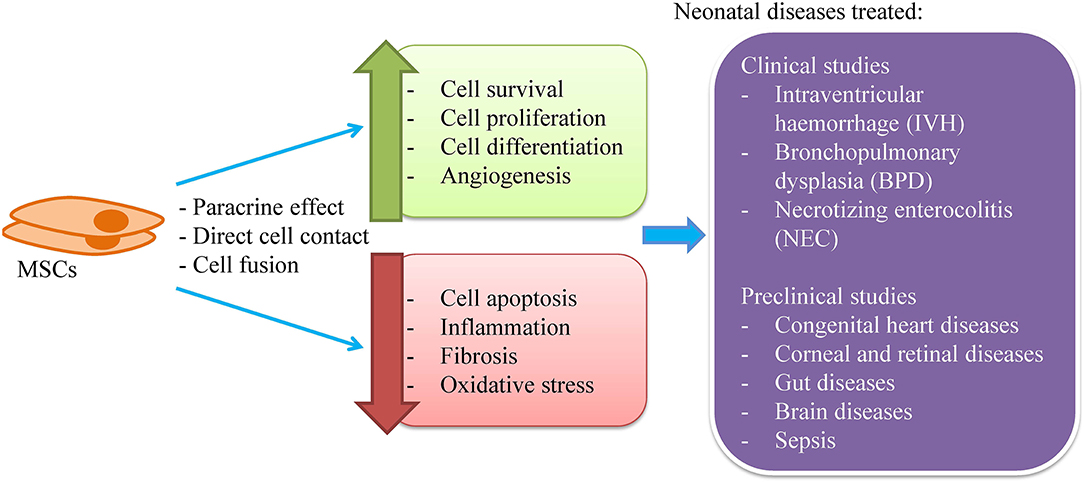
Figure 1. MSCs for the treatment of neonatal diseases. MSCs are known to exert its therapeutic effect through paracrine secretion, direct cell contact and cell fusion to enhance cell survival, proliferation and differentiation, and angiogenesis as well as to reduce cell apoptosis, inflammation, fibrosis, and oxidative stress. Clinically, MSCs have been used to treat intraventricular hemorrhage, bronchopulmonary dysplasia, and necrotizing enterocolitis. Besides, MSCs have been tested for the treatment of congenital heart diseases, eye, gut, and brain diseases as well as sepsis.
In this review, literature search was conducted using the keywords (1) neonate and (2) clinical and (3) mesenchymal stem cells on Pubmed and Medline databases and all the relevant articles were included in the review.
MSCs for the Treatment of Neonatal Lung Diseases
Bronchopulmonary Dysplasia (BPD)
BPD is a chronic lung disease affecting mostly preterm neonates and is characterized by disrupted alveolar growth and pulmonary vascularization. Recent years have witnessed an increased incidence of BPD due to the spike in the population of preterm survivors as a result of the improvement in neonatal care. Pulmonary hypertension is common in severe cases of BPD. Affected infants may require oxygen support and commonly develop respiratory problems that need hospitalization (14, 15).
Currently, clinical trials evaluating MSC therapeutic and preventive effects on BPD are still limited (Table 2). The majority of the studies are small in scale, non-randomized and lack proper comparison groups. To the best of our knowledge, the first clinical study was reported by Chang et al. in 2014 which followed-up the patients up to 2 years (11, 16). The study examined the safety and feasibility of single intratracheal transplantation of 1 × 107 and 2 × 107 allogeneic UCB-MSCs/kg in 9 preterm infants with a high risk of developing BPD at the age of 7–14 days. Results showed that UCB-MSC therapy helped to reduce BPD severity and inflammatory markers as well as to minimize the risk of neurodevelopmental morbidities. Powell et al. treated 12 preterm infants with BPD using the same protocol as Chang et al. and found that disease severity improved after the stem cell treatment (19). Alvarez-Fuente et al. reported suppression of pro-inflammatory as well as improvement in pulmonary hypertension and diminished levels of surfactant protein D (SP-D) expression, a biomarker for lung injury, in 2 preterm infants given multiple dosages of allogeneic BMSCs intravenously (17). Nonetheless, both patients received stem cell therapy at the very advanced stages of BPD and passed away 6 weeks after the initiation of MSC therapy. Lin et al. reported improvement in respiratory functions after intratracheal administration of maternal BMSC (6.25 × 106 cells/kg) in a 10-month old preterm infant with BPD that developed acute respiratory distress syndrome (ARDS) (18). Results from these clinical studies suggested that the beneficial effects of MSCs might be attributed to paracrine effects that stimulate alveolarization and vascularization rather than cell engraftment and proliferation as traces of donor cells in the recipients were absent.
The optimal dosage regimen, including the optimal MSC per dose, number of doses, timing of transplantation, time between doses, and route of administration, is essential in determining MSC efficacy. However, this regimen has not been established yet. The identified clinical studies used MSC dose of 1–20 × 106 cells/kg body weight. Studies that compared high and low doses (1 × 107 vs. 2 × 107 cells/kg) found no difference in outcome (11, 19). Majority of the studies gave a single dose except for Alvarez-Fuente et al. that applied multiple infusions (17). Chang et al. noticed that respiratory function improvement started to decline after 7 days of transplantation (11). This finding supports the notion of the inadequacy of a single-dose approach and the authors suggested that day 7 could be the time for the second dosage of MSCs. Another issue is determining the optimal timing of MSC transplantation. Generally, researchers agreed that early intervention is more beneficial for BPD patients. Most studies administered the cells using the intratracheal route to ensure high cell homing at the targeted tissue while evading systemic side effects (11, 18, 19). Besides, the airway is easily accessible through the endotracheal tube in preterm neonates assisted with mechanical ventilation. Although there are concerns over the risk of delivering a large number of MSCs to the premature lungs, nonetheless, intratracheal administration has been shown to be safe and feasible. Intravenous administration also has been reported to be safe and this route might be preferred in infants that do not require invasive ventilation (17). Preclinical studies showed that intravenously administered MSCs reach the lungs within 24 h and dissipate in 7 days after infusion with no traces in other organs (20).
In spite of the inconsistencies on the degree of effectiveness of MSCs, all reported studies support the apparent safety profile of MSCs regardless of the dose and route of administration. There was no anaphylactic shock, no immune rejection, no mass formation and no dose-limiting toxicities reported. This has led to much excitement about the potential of using MSCs in the prevention and treatment of BPD. There are many more studies that are currently underway (Table 3).
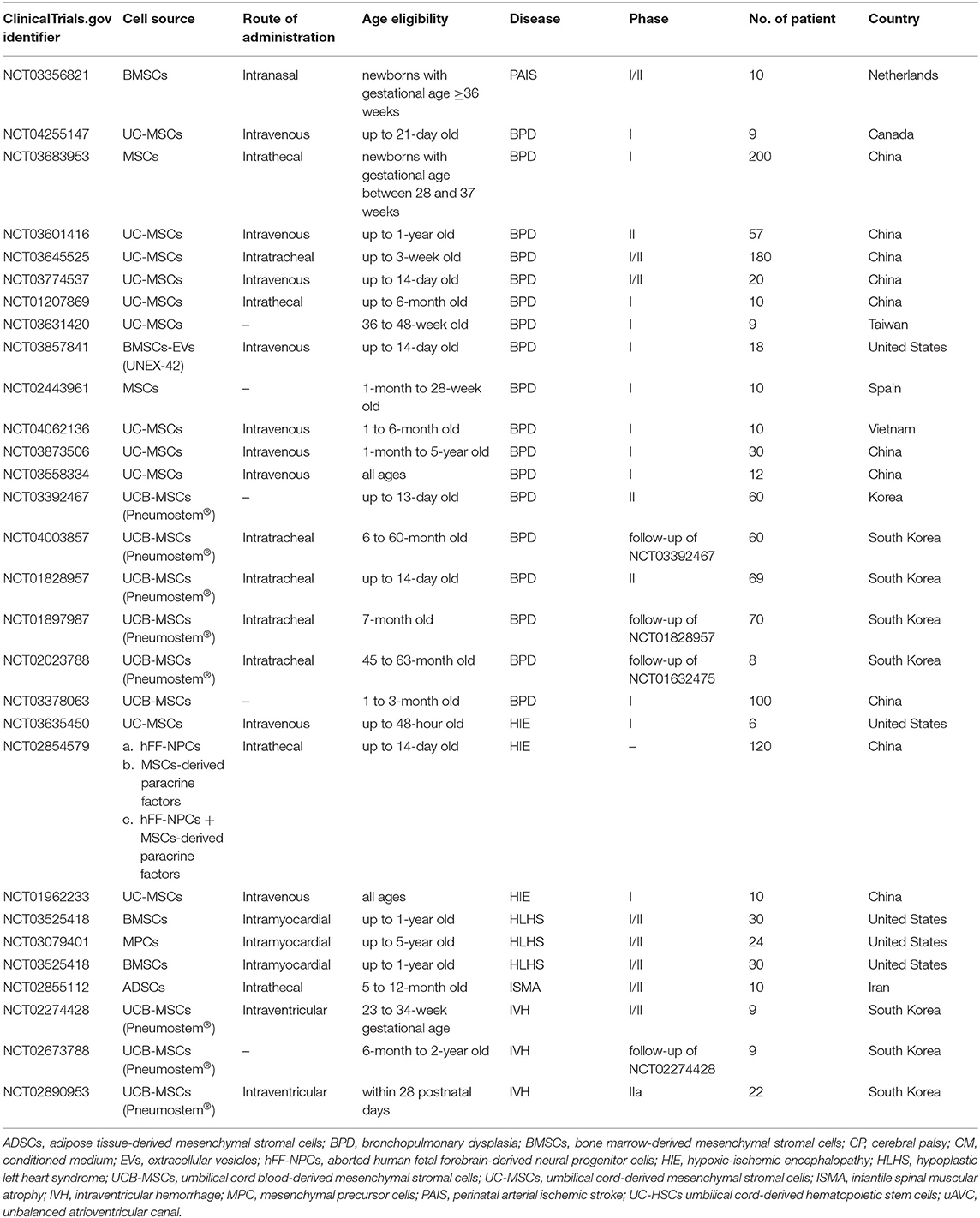
Table 3. Clinical trials using MSCs to treat neonatal diseases recorded in ClinicalTrials.gov.
MSCs For the Treatment of Neonatal Brain Diseases
Neonatal brain diseases, including intraventricular hemorrhage (IVH), neonatal stroke (NS), hypoxic-ischemic encephalopathy (HIE) and periventricular leukomalacia (PVL), are the major contributor to the high mortality and morbidity rates among the neonate (21). Experimental investigations have identified multiple causes for these diseases, including perinatal-induced neuronal cell death due to depleted tissue energy reserve, placental disruption, prolapsed umbilical cord, dysfunctional mitochondria, accumulated free oxygen radical species, persistent inflammatory reactions, defective maturation, and myelination of neuronal cells, as well as the loss of endogenous stem cell pool that is responsible for cell differentiation and tissue repair (22, 23). In the developing brain, pre-oligodendrocytes normally present in high number to form mature oligodendrocytes for the production of myelin sheath. Unfortunately, these pre-oligodendrocytes are more vulnerable than its mature counterpart, hence are more easily lost in the presence of the injurious assaults leading to the development of neonatal brain diseases (22). Despite the advances and progress in neonatal therapeutic medicine, there is still a lack of therapy that is effective to prevent or cure developmental brain injuries in neonates. Therefore, research for a newer, safer and more effective therapeutic approach is necessary to improve the outcome of these conditions. Thus, far, the use of stem cell-based therapy has been well-researched as an intervention to ameliorate complications associated with brain diseases. MSCs have been used clinically to treat IVH, whilst pre-clinical studies have been conducted to unveil the potential of MSCs to attenuate or to repair the brain injury present in various disease models, including NS, HIE, and PVL.
Intraventricular Hemorrhage (IVH)
IVH is a perinatal brain morbidity that results from the rupture and bleeding of the germinal matrix that is restricted to the ventricular area of the brain. Premature neonates are at a higher risk of developing IVH (24). IVH continues to be one of the leading causes of mortality and lifetime disability in infants. A recent systematic review concluded that prematurity and IVH are major contributing factors to the increased risk for cerebral palsy (CP) in neonates (25).
Clinical research examines MSC therapeutic and preventive effect on IVH is still in its infancy. To the best of our knowledge, the first clinical trial for the evaluation of MSC therapy in IVH patients was revealed by Ahn et al. in South Korea in 2018 (12). It was an uncontrolled phase I dose-escalation study intended to investigate the safety and feasibility of the intraventricular transplantation of allogeneic UCB-MSCs. Nine premature newborns of gestational age 23–34 weeks with severe IVH of grade 3 and 4 were recruited and received a single dose of UCB-MSCs. The average age at transplantation was 11.6 days, around 7 days after IVH diagnosis. UCB-MSCs were injected directly to the lateral ventricle through the anterior fontanelle. Two different UCB-MSC concentrations were tested. The lowest dose used was 5 × 106 cells/kg and the highest dose was 1 × 107 cells/kg given to 3 and 6 patients, respectively. Preliminary findings showed that transplantation was well-tolerated with no immune rejection, anaphylactic shock, dose-related toxicity, death, or any MSC infusion-related severe adverse events. Eight infants developed prematurity related disorders including BPD, sepsis, retinopathy of prematurity, and NEC. Additionally, 5/9 MSC recipients needed shunt placement. The 2-year follow-up study to evaluate the safety of MSC transplantation on these infants is still ongoing (NCT02673788). The same research group is currently conducting a phase II trial to assess the therapeutic efficacy of MSC therapy in preterm infants with IVH (NCT02890953). Of note, the narrow therapeutic window and utilization of brain injury biomarkers should be considered when designing MSC therapeutic protocols for IVH. More well-designed clinical studies to confirm the safety and efficacy of MSC transplantation in IVH patients are necessary.
Neonatal Stroke (NS)
NS involves injury to the cerebral tissue, usually caused by a disruption in arterial blood flow or major cerebral vein due to the presence of thrombus or embolism. It is a neonatal brain disease with high mortality and morbidity. The use of MSCs has been attempted by Kim et al. for the attenuation of brain injuries in a middle cerebral artery occlusion-induced newborn rat model (26). They observed a reduction in brain infarct volume, improvement of functional tests and enhanced astrogliosis post-MSC transplantation. Similarly, van Velthoven et al. delivered MSCs intranasally to the stroke-affected newborn rats and discovered that the extent of brain injury was reduced, as shown by the decreased size of the infarct, reduced loss of brain matter as well as improved motor functions (27). In another study, exosomes isolated from MSCs were used to treat lipopolysaccharide-induced neuroinflamed 3-day old rat pups intranasally (28). As a result, the expression of inflammatory genes and pro-inflammatory cytokines were suppressed by the exosomes, therefore decreasing the microglia-mediated brain injuries. These exosomes primarily worked by interfering with the Toll-like receptor 4 signaling of microglial cells, hence preventing degradation of NFκB inhibitor (IκBα), as well as phosphorylation of MAP kinase family of proteins.
Hypoxic-ischemic Encephalopathy (HIE)
HIE refers to a type of brain injury whereby insufficient blood flow is delivered to the brain tissue, resulting in death or severe disabilities such as epilepsy, intellectual disability and CP (29). The current widely used treatment option for HIE is hypothermia, although it only provides a neuroprotective effect rather than a neurorestorative function. Therefore, researchers are working hard to establish a therapy that could improve the prognosis of this neonatal disease.
The combined therapy of hypothermia and UCB-MSCs significantly reduced the cerebral infarcted area and improved the sensorimotor function of rats with HIE (30). This improved diseased condition is thought to be contributed by the paracrine factors released by MSCs, including IGF-1, bFGF, neural cell adhesion molecules, nerve growth factors, and anti-inflammatory cytokines, which stimulated neurogenesis. Moreover, MSCs enhanced expression of genes that triggered cell proliferation while suppressing genes involved in inflammatory responses. Through the inhibition of apoptosis, induction of nerve fibers to remyelinate and axons to regenerate, the administered MSCs were also able to reduce the loss of brain matter while improving sensorimotor functions (31, 32). The potential neurorestorative function of MSCs is further demonstrated in the study by van Velthoven et al. (27). In the control group, inflammatory and cell death activities in the affected rodent brain tissue stopped after 4 days, indicating the therapeutic window to be up to 4 days after the initial attack. However, intranasal transplantation of 1 × 107 MSCs/kg at day 10 post-hypoxia-ischemia attack in the experimental group was still able to limit the brain tissue damage. This implies that tissue reparative activity is ongoing and responsive to MSCs that migrated to the ischemic zone to induce a microenvironmental change which favors neurogenesis (27, 33). Functionality wise, newborn rodents treated with MSCs also showed long-lasting improvement in cognitive and motor functions, up to 14 weeks post-injury (34). In a pre-clinical study, Ophelders et al. reported that intravenous administration of BMSC-derived extracellular vesicles (EVs) to the fetus improved the brain function using a preterm sheep model of hypoxic-ischemic brain injury (35).
Periventricular Leukomalacia (PVL)
PVL is characterized by a loss of oligodendrocyte and its progenitor cell. This disease is one of the clinical complications of HIE that lead to CP. Other than HIE, the predisposing factors include birth trauma, and immature brain development. In neonates affected by PVL, their periventricular tissue undergoes necrosis while the surrounding white matter experiences diffused gliosis. These changes in brain tissue will disrupt the cognitive, adaptive, motor, and social functions in the infant. Unlike other brain diseases with some limited therapeutic option, there is no specific treatment for PVL except for expectant and supportive management (36). Thus, researchers have begun exploring the use of MSCs for the treatment of PVL.
In the cystic PVL rat model used by Chen et al., excitotoxic ibotenic acid was injected into the white matter of rat brain leading to loss of myelin with transient formation of cysts, activation of microglia and development of CP-like behavioral defects (37). With this animal model, the research group injected the animals intracerebrally with BMSCs 1 day after PVL induction. Results showed that MSCs migrated to the lesion areas in the brain to increase the proliferation of glial cells, hence resulting in improvement of the myelination process with better motor function outcome. Using a different PVL study model that is established through ligation of the left common carotid artery inducing 4 h of hypoxia, the rats were treated with intraperitoneal UC-MSC injection for 3 consecutive days (38). Similarly, functional outcomes improved with decreased microglia and astrocyte activities. Another study by Morioka et al. also demonstrated the suppression of pro-inflammatory cytokines in the affected brain treated with UC-MSCs to reverse the lipopolysaccharide-induced PVL-like injury in newborn rats (39).
MSCs for the Treatment of Neonatal Gut Diseases
There are several types of neonatal gut diseases that affect the neonate, with higher prevalence in the preterm infant. Currently, MSC therapy has been used to treat NEC in one clinical study involving a neonatal patient. Conversely, several preclinical studies have examined the safety and efficacy of MSC therapy to ameliorate NEC and gastroschisis.
Necrotizing Enterocolitis (NEC)
NEC is the most common devastating gastrointestinal disease affecting the neonate, with a mortality rate between 15 and 30 % (40). It may affect both full-term and preterm neonates with the latter group having an increased risk. Predisposing factors include prematurity, low birth weight, formula feeding, intrauterine growth retardation, postnatal asphyxia, sepsis, and congenital heart diseases (41, 42). In NEC, bowel inflammation causes necrosis and perforation which may lead to intestinal surgical removal, death or a lifetime severe neurodevelopmental impairment (43, 44)
MSCs have emerged as a promising therapeutic choice for intestinal anomalies such as ulcerative colitis and Crohn's disease (45, 46). However, the therapeutic benefit of MSCs for NEC has yet to be fully explored in clinical setting. A case report of a full-term, 22-day old male neonate weighed 3.350 kg who experienced NEC as a consequence to repeated supraventricular tachycardia (SVT) leading to the resection of 60 cm of the intestine because of gangrene revealed a noticeable improvement after intravenous infusion of MSCs (13). A single dose of 1 × 107 allogeneic UC-MSCs was transfused 4 days after colectomy with no complications. Improved intestinal blood supply was confirmed 3 days post-transplantation, enteral nutrition was resumed at day 8, and the jejunal stoma was closed 46 days post-transplantation. The authors speculated that MSC therapy helped to salvage the remaining of the resected intestine thus prevent short bowel syndrome. Overall, MSC transplantation may offer substantial practical advantages to NEC patients. Nonetheless, findings of this single case report should be interpreted with caution.
In an animal study conducted by Taymen et al. BMSCs were administered to a rat model of NEC (47). The rat showed significant weight gain and improvement in clinical sickness scores after BMSC transplantation. The number of BMSCs homing to the bowel was significantly higher in the treated group. In fact, the severity of bowel damage was significantly reduced in histopathological evaluation. A similar study was conducted by McCulloh et al. using 4 different sources of stem cells, i.e., BMCSs, amniotic fluid-derived MSCs (AF-MSCs), amniotic fluid-derived neural stem cells and neonatal enteric neural stem cells (48). The results indicated that all sources of stem cells reduced the incidence and severity of experimental NEC equally.
Gastroschisis
Gastroschisis is a full-thickness paraumbilical defect in the abdominal wall that results in herniation of the fetal midgut with a significant mortality rate of 5–10% (49). Feng et al. evaluated the efficacy of intraamniotic delivery of concentrated AF-MSCs to treat gastroschisis (50). The cells were injected into rat fetuses with surgically-created gastroschisis. A significantly thicker muscular layer was observed in the AF-MSC group compared to the control group. It was concluded that AF-MSCs mitigated bowel damage in experimental gastroschisis after intraamniotic injection. A further study was conducted by the same research group but in a larger animal model, i.e., the rabbit (51), which reported similar results.
Since heterotopic cell fusion has been demonstrated between MSCs and various cells, as such, the similar mechanism of protection was also proposed in congenital gut diseases. Nevertheless, preclinical data do not support this hypothesis (52, 53). It seems that MSC homing and paracrine effects are better accepted as the protective mechanism in congenital gut diseases. MSCs may be attracted to the injured site, differentiate to replace damaged cells or interact with native intestinal stem cells to upregulate the Wnt/β-catenin pathway, which promotes auto-regeneration of the intestinal epithelium (54). MSCs secrete a myriad of paracrine factors that promote gut healing and reconstruction. MSC secretome has been shown to improve the severity in a rat NEC model (47).
MSCs for the Treatment of Neonatal Eye Diseases
Corneal disease is a challenging condition to manage particularly in the neonatal age group. However, successful preclinical studies in the past 10 years have shed some light on the usage of MSCs to treat neonatal corneal diseases. Liu et al. evaluated the efficacy of MSC transplantation in treating congenital corneal disease in vivo (55). The corneal disease was simulated using a Lumican null mice model where the cornea is manifested as thin and cloudy due to the disorganization of stromal extracellular collagen matrix and down-regulated expression of keratocan. Results showed that stromal thickness increased significantly and transparency improved in MSC-treated corneas as early as 8 weeks after treatment compared to the control group. This exciting finding has spurred the interest of other research groups to use the similar strategy to treat neonatal patients with corneal diseases.
Mucopolysaccharidosis (MPS) VII is a disease caused by the mutation of β-glucuronidase. Patients with this disease often suffer from developmental defects including short stature, corneal clouding and delayed development. In 2013, Coulson-Thomas et al. transplanted UC-MSCs into the cornea of MPS VII mice (56). MSC-transplanted corneas demonstrated reduced corneal haze 1–3 months post-transplantation. Corneas treated during the first and second month presented significantly improved corneal integrity in comparison to those treated at the third month, suggesting that prophylactic treatment upon diagnosis could prevent the development of corneal clouding. An overall decrease in glycosaminoglycan content was detected in corneas treated at all time points in comparison to the untreated corneas.
Collagen V is a critical protein in the regulation of corneal collagen fibrillogenesis and function development. It has been reported that collagen V knockout stroma demonstrated severe dysfunctional regulation of fibrillogenesis (57). Call et al. treated this congenital and acquired corneal opacity associated with the loss of collagen V in Col5a1Δst/Δst mice (58). The mice were subjected to keratectomy in which the corneal epithelium and the anterior stroma were removed before received UC-MSC transplantation via intrastromal injection. MSC therapy reduced corneal opacity by re-establishing the collagen fibril arrangement via the production of collagen V.
Retinal neovascularization is an unhealthy development which leads to visual diminution and even blindness. Xu et al. studied the therapeutic effect of BMSCs against retinal neovascularization and found that BMSCs migrated and integrated into the host retina, significantly inhibited retinal neovascular tufts and remodeled the capillary network after injection (59). In addition, BMSCs increased the retinal vascular density, decreased the number of acellular capillaries and inhibited retinal cell death.
The therapeutic effect of MSCs in congenital cornea diseases is believed to be attributed to supplementation or replacement of corneal cells. In lumican null cornea model by Liu et al., the injected UC-MSCs acquired the characteristics of keratocytes in which lumican and keratocan were laid down in the stroma cornea (55). The treated animal regained normal corneal thickness and transparency due to reorganization of the collagen fibrils in the cornea. Likewise, MSC transplanted into MPS VII mice cornea also acquired the characteristics of keratocytes. The transplanted cells supplied functional β-glucuronidase to degrade accumulated glycosaminoglycans thereby assisting in lysosome recycling (60). On the other hand, MSCs also secrete paracrine factors that exert anti-inflammation and anti-fibrosis functions, thus suppressing inflammatory neovascularization (61, 62). Through these mechanisms, MSCs can mitigate the inflammation, fibrosis and neovascularisation of the cornea.
MSCs for the Treatment of Neonatal Heart Diseases
Research has been conducted to investigate the application of MSCs in 28-day old mice/rats for the treatment of heart diseases. In an in vitro experiment, it was found that neonatal thymus-derived MSCs (ntMSCs) secreted higher level of sonic hedgehog (Shh) in comparison to neonatal bone marrow-derived MSCs (nbMSCs) (63). Shh is involved in cell growth, cell specialization and morphogenesis of the corpus during embryonic development. Animal studies have revealed that ntMSC transplantation improved the left ventricular function, increased revascularization, decreased scar size and decreased cardiomyocyte death, 4 weeks after infarction. The protective mechanism in this study could be attributed to heterotopic cell fusion. This mechanism occurs when two cells from different lineages merge into one cell, transmitting information and mediators in the process (64). Fusion has been demonstrated between BMSCs with cardiomyocytes, Purkinje neurons and hepatocytes (65). These results warrant further consideration as therapy for heart diseases resulting in early-onset heart failure, such as the cardiomyopathies.
Kang et al. compared the pro-angiogenic, anti-apoptotic and inflammation-modulatory potential of MSCs derived from patients of cyanotic congenital heart disease (C-CHD) and acyanotic congenital heart disease (A-CHD) (66). The purpose of the study is to locate the best source of MSCs for engineered heart tissue treatment (EHT). In vitro results showed that MSCs from C-CHD patients expressed higher levels of VEGF-A and VEGFR2, and secreted more pro-angiogenic and anti-inflammatory cytokines under hypoxic condition in comparison to the cells from A-CHD patients. Interestingly, 4 weeks after right ventricular outflow tract reconstruction, cytokine-immobilized patches seeded with C-CHD MSCs exhibited preserved morphology, prolonged cell survival and enhanced angiogenesis compared to A-CHD MSCs. Considering the “naturally hypoxic preconditioning,” MSCs from C-CHD donors might be more adaptable to the hypoxic environment upon transplantation.
MSCs for the Treatment of Neonatal Sepsis
In sepsis, the host immune system responsible for the clearing of infection may be dysregulated and becomes no longer protective to the host when it is too exuberant. Such an injurious hyperinflammatory response frequently involves excessive release of cytokines and over-activation of the complement system. Unnecessary stimulation of the coagulation system and pro-thrombogenesis is another characteristic of sepsis, which eventually leads to poorer tissue perfusion, cell death and organ dysfunction (67).
A variety of animal sepsis models have been established depending on the use of different bacterial pathogens such as P. aeruginosa and E.coli, induction with a toxic agent such as lipopolysaccharide (LPS) as well as disruption to the tissue barrier integrity such as cecal ligation and puncture (CLP) (67–70). Using these animal models, the effects of a variety of MSC variables have been tested, including dosage, timing, and route of MSC administration. Reports have shown that MSC dosage as low as 2.5 × 105 cells was effective in reducing the mortality rate of the septic animals though the effect was better when increasing the cell number to 106 cells per animal. Furthermore, the treatment efficacy may be affected by the timing of MSC administration as some researchers failed to identify any positive effects of MSC when administered 6 h post-CLP induction while other identified positive correlation when used at 24 h pre-CLP induction and up to 24 h post-induction. Similar contradictory findings were seen in the route of administration used whereby intraperitoneal injections of MSCs were able to decrease demise of LPS-induced septic mice in one study while the same method resulted in slightly more deaths among CLP-induced septic rats in another investigation (68, 70).
Notably, most of the studies reported that the higher survival rate of septic animals post-MSC treatment is attributable to improved organ function presumably from the MSC anti-apoptotic and anti-oxidant properties as well as reduction of the inflammation-associated tissue injury (68, 71). This is because the administration of MSCs is capable in limiting immune cell infiltration to target organs such as the liver, intestine, lung, and kidney. MSC administration also balances the enhanced expression of pro-inflammatory cytokines seen during the sepsis with higher production of anti-inflammatory cytokines (72).
In addition, MSC may reduce the bacterial burden causing sepsis and increase the survival rate of septic animals. In the study by Zhu et al., treatment with UC-MSCs enhanced the clearance of E. coli in 3-day old septic rats with a decreased influx of neutrophils coupled with the increased phagocytic activity of macrophages (73). The antimicrobial activity of MSCs is also due in part to its ability to synthesize anti-microbial peptides. Krasnodembskaya et al. discovered the secretion of LL-37 anti-microbial peptide by MSCs after exposure to E. coli, P. aeruginosa, and S. aureus (74). Likewise, Lee et al. reported the increased clearance of E. coli through the effects of KGF secreted by MSCs (75). A more recent study demonstrated the potential of pre-treating MSCs with polyinosinic:polycytidylic acid (an agonist for Toll-like receptor 3) to further improve its anti-microbial activity (76).
List of Registered Clinical Trials
Apart from the various completed clinical studies discussed earlier, there are various clinical trials currently registered in the ClinicalTrials.gov database that have yet to publish results (Table 3). These clinical trials use MSCs to treat BPD, perinatal arterial ischemic stroke (PAIS), infantile spinal muscular atrophy (ISMA), hypoplastic left heart syndrome (HLHS), HIE, and IVH. The treatment of neonatal diseases with MSCs is still at the early stage whereby the majority of these trials are either phase I, II or I/II. Of note, MSC-derived EVs, and paracrine factors are also being evaluated clinically for the treatment of BPD (NCT03857841) and HIE (NCT02854579).
Future Perspectives
Large-Scale Production of MSCs
A sustainable strategy of MSC expansion is essential to fulfilling the large cell number needed for clinical applications. To achieve a scalable MSC expansion, multi-layered flask, spinner flask, and bioreactor were introduced and gained more attention as a potential for mass production. The multi-layered flask is a static culture system while the spinner flasks and bioreactors are dynamic expansion system. Each of the aforementioned systems has its unique features, whereby the selection should base on preferences or requirements in different situations.
Multi-layered flask offers a simple and straight forward up-scale process. However, due to its larger size compared to conventional flasks, cell monitoring throughout the culture period is almost impossible. Also, the uniform distribution of cells will be relatively harder to achieve and possibly will lead to culture heterogeneity and suboptimal yield of cells (77).
Three-dimensional culture in bioreactor simulates the environment of cells in vivo, therefore providing an optimal condition that possibly enhances cellular activities that are not observed in standard monolayer culture (78). Unlike traditional MSC culture protocol, microcarriers are usually added into the culture to provide sufficient growth areas for cells (79). Compared to the multi-layered flask, bioreactor offers better monitoring of cells throughout the culture period and precise control of the culture environment can be done with the aid of computerized sensors in the bioreactor. For more information about the large-scale expansion of MSCs, the readers may refer to the systematic review by Hassan et al. (80).
Cell-Free Approaches
Recently, EVs, including microvesicles and exosomes, have been identified as one of the vital components of stem cell paracrine potency (Figure 2). Many researchers suggested that, if purified and concentrated, the therapeutic potential of EVs can be greater compared to the parent cell (81, 82). In addition, it is still unclear in the long-term regarding the safety of MSC transplantation, e.g., the possibility of malignant transformation. Hence, the use of EVs may be a safer approach and a better alternative to MSC therapy.
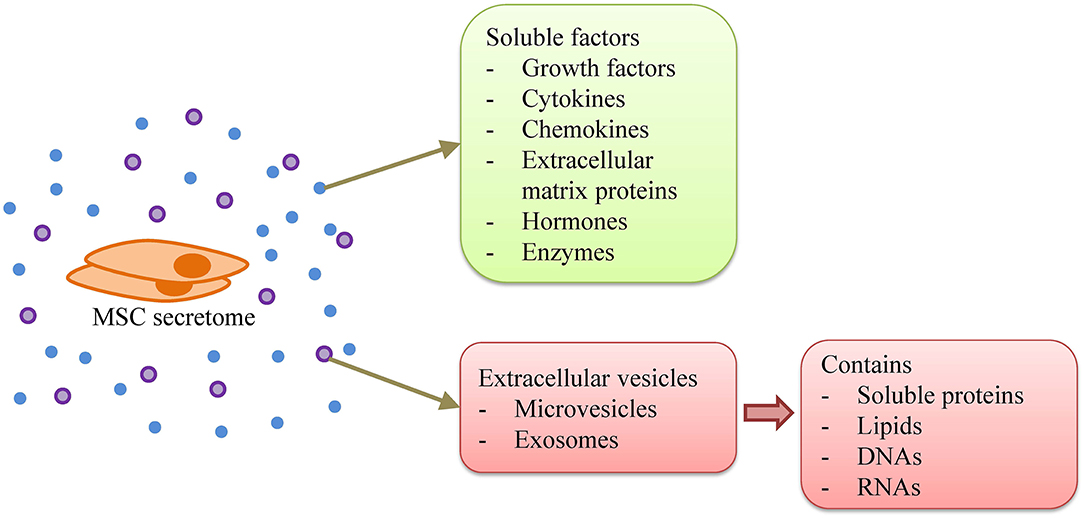
Figure 2. MSC secretome. MSC secretome consists of soluble factors, i.e., growth factors, cytokines, chemokines, extracellular matrix proteins, hormones, and enzymes, as well as extracellular vesicles that contain soluble proteins, lipids, DNAs, and RNAs.
EVs are nano-sized particles secreted by cells and are delimited by a phospholipid bilayer. However, EVs do not possess a functional nucleus, hence do not replicate (83). EVs carry various proteins, lipids, and nucleic acids, particularly in the form of RNA, which function in intercellular signaling (84).
EV therapy has been demonstrated to exhibit promising results in ameliorating neonatal diseases in animal model studies (28, 35). A clinical trial (NCT03857841) has recently been developed to evaluate EV therapeutic potential. Nonetheless, there are several challenges to overcome in the bench to bedside translation of EV therapy. One of the major challenges is to identify and quantify the content of EVs, especially the nature of their therapeutically active components. Much effort is made to understand the bioactive elements of these EV-cargoes. EVs are reported to contain proteins, lipids, and nucleic acids, yet there is still a lack of understanding regarding the role of some of these components, particularly the non-coding RNA species. Similarly, the function and mechanism of action of the DNA found in EVs remain unclear.
Besides these cargoes, another vital aspect to consider in EV therapy is the potency. Currently, there are several methods to quantify EV doses based on cell equivalent calculation, protein concentration, EV number and particle size using specialized quantitative analytical measurements, such as nanoparticle tracking analysis (85). EVs are known for having short half-life, as such the therapeutic effect could be short-lived (86). Therefore, a repeat or multiple dosage model is preferred compared to single administration for sustained therapeutic effects over time (87). Sjöqvist et al. observed that repeated administration of EVs was more important than the dose in promoting wound healing in a pig model of esophageal wound repair (88). Furthermore, repeat administration of a lower dose reduces the risk of toxicity and immunogenicity (89, 90).
Another major challenge is to isolate highly purified EVs (91). Currently, purity of EVs is normally expressed as the ratio between protein content and the number of EVs. It has been reported that the purity of EVs is directly related to the isolation technique (91). The isolation technique may impact on EV yield, size distribution and potential biological effects. Currently, many techniques, including ultracentrifugation, size-based filtration, immunoaffinity capture, precipitation and microfluidics-based isolation are used to isolate EVs (92). Different isolation methods yielded a different amount of EVs and Antounians et al. recently reported that significant biological variations were also observed when EVs isolated with different methods were used to treat an in vitro lung epithelial injury model (93). The dose-dependent effects have been reported by in several studies (94–96). To date, it is uncertain which isolation technique can provide an optimal yield and the best outcome.
Apart from purity considerations, quality control of EV preparation is also vital. It is proposed that the doses for EVs should be specifically quantified, e.g., by the copies of their microRNAs, so as to reduce the inherent biological variation.
Conclusion
MSC therapy is a promising treatment for neonatal diseases. The safety and efficacy of MSC therapy have been reported in numerous preclinical and several clinical studies. MSC transplantation can be conducted safely as early as the first month of life. Future studies should be well-designed, i.e., controlled, randomized, involving a larger group of patients, and with a longer follow-up period, to further assure the safety and efficacy of this novel therapy. Besides, more focus is needed to improve on the dosage regimen, to investigate the influence of adjunct therapies on MSC effectiveness and to identify molecular biomarkers to indicate MSC efficacy. In addition, MSC-EV has emerged as a highly potential therapy for neonatal diseases as MSC exerts its therapeutic potential via the paracrine mechanism.
Author Contributions
FC and JL revised it critically for important intellectual content. All authors substantially contributed to the conception and design of the article and interpreting the relevant literature, drafted the article, and approved the final draft.
Funding
This work was supported by research grants from the Universiti Kebangsaan Malaysia Medical Center (FF-2019-450/1 and FF-2020-038).
Conflict of Interest
The authors declare that the research was conducted in the absence of any commercial or financial relationships that could be construed as a potential conflict of interest.
References
1. Liau LL, Ruszymah BHI, Ng MH, Law JX. Characteristics and clinical applications of Wharton's jelly-derived mesenchymal stromal cells. Curr Res Transl Med. (2020) 68:5–16. doi: 10.1016/j.retram.2019.09.001
2. Hofer HR, Tuan RS. Secreted trophic factors of mesenchymal stem cells support neurovascular and musculoskeletal therapies. Stem Cell Res Ther. (2016) 7:131. doi: 10.1186/s13287-016-0394-0
3. Looi QH, Eng SP, Liau LL, Tor YS, Bajuri MY, Ng MH, et al. Mesenchymal stem cell therapy for sports injuries-From research to clinical practice. Sains Malaysiana. (2020) 49:825–38. doi: 10.17576/jsm-2020-4904-12
4. Lim WL, Liau LL, Ng MH, Shiplu RC, Law JX. Current progress in tendon and ligament tissue engineering. Tissue Eng Regen Med. (2019) 16:549–71. doi: 10.1007/s13770-019-00196-w
5. Hafez P, Chowdhury SR, Jose S, Law JX, Ruszymah BHI, Ramzisham ARM, et al. Development of an in vitro cardiac ischemic model using primary human cardiomyocytes. Cardiovasc Eng Technol. (2018) 9:529–38. doi: 10.1007/s13239-018-0368-8
6. Hafez P, Jose S, Chowdhury SR, Ng MH, Ruszymah BHI, Abdul Rahman Mohd R. Cardiomyogenic differentiation of human sternal bone marrow mesenchymal stem cells using a combination of basic fibroblast growth factor and hydrocortisone. Cell Biol Int. (2016) 40:55–64. doi: 10.1002/cbin.10536
7. Lim J, Razi ZRM, Law JX, Nawi AM, Idrus RBH, Chin TG, et al. Mesenchymal stromal cells from the maternal segment of human umbilical cord is ideal for bone regeneration in allogenic setting. Tissue Eng Regen Med. (2018) 15:75–87. doi: 10.1007/s13770-017-0086-6
8. Lim J, Razi ZRM, Law J, Nawi AM, Idrus RBH, Ng MH. MSCs can be differentially isolated from maternal, middle and fetal segments of the human umbilical cord. Cytotherapy. (2016) 18:1493–502. doi: 10.1016/j.jcyt.2016.08.003
9. Lee M, Jeong SY, Ha J, Kim M, Jin HJ, Kwon SJ, et al. Low immunogenicity of allogeneic human umbilical cord blood-derived mesenchymal stem cells in vitro and in vivo. Biochem Biophys Res Commun. (2014) 446:983–9. doi: 10.1016/j.bbrc.2014.03.051
10. Liau LL, Looi QH, Eng SP, Yazid MD, Sulaiman N, Busra MFM, et al. Mesenchymal stem cells for the treatment of immune-mediated diseases. In: Haider KH, editor. Stem Cells. Singapore: World Scientific (2020). p. 178–210.
11. Chang YS, Ahn SY, Yoo HS, Sung SI, Choi SJ, Oh W Il, et al. Mesenchymal stem cells for bronchopulmonary dysplasia: phase 1 dose-escalation clinical trial. J Pediatr. (2014) 164:966–72. doi: 10.1016/j.jpeds.2013.12.011
12. Ahn SY, Chang YS, Sung SI, Park WS. Mesenchymal stem cells for severe intraventricular hemorrhage in preterm infants: phase I dose-escalation clinical trial. Stem Cells Transl Med. (2018) 7:847–56. doi: 10.1002/sctm.17-0219
13. Akduman H, Dilli D, Ergün E, Çakmakçi E, Çelebi SK, Çitli R, et al. Successful mesenchymal stem cell application in supraventricular tachycardia-related necrotizing enterocolitis: a case report. Fetal Pediatr Pathol. (2019) 1–6. doi: 10.1080/15513815.2019.1693672. [Epub ahead of print].
14. Higgins RD, Jobe AH, Koso-Thomas M, Bancalari E, Viscardi RM, Hartert T V, et al. Bronchopulmonary dysplasia: executive summary of a workshop. J Pediatr. (2018) 197:300–8. doi: 10.1016/j.jpeds.2018.01.043
15. Kwon HW, Kim HS, An HS, Kwon BS, Kim GB, Shin SH, et al. Long-term outcomes of pulmonary hypertension in preterm infants with bronchopulmonary dysplasia. Neonatology. (2016) 110:181–9. doi: 10.1159/000445476
16. Ahn SY, Chang YS, Kim JH, Sung SI, Park WS. Two-year follow-up outcomes of premature infants enrolled in the phase I trial of mesenchymal stem cells transplantation for bronchopulmonary dysplasia. J Pediatr. (2017) 185:49–54.e2. doi: 10.1016/j.jpeds.2017.02.061
17. Álvarez-Fuente M, Arruza L, Lopez-Ortego P, Moreno L, Ramírez-Orellana M, Labrandero C, et al. Off-label mesenchymal stromal cell treatment in two infants with severe bronchopulmonary dysplasia: clinical course and biomarkers profile. Cytotherapy. (2018) 20:1337–44. doi: 10.1016/j.jcyt.2018.09.003
18. Lin HC, Wang CC, Chou HW, Wu ET, Lu FL, Ko BS, et al. Airway delivery of bone marrow-derived mesenchymal stem cells reverses bronchopulmonary dysplasia superimposed with acute respiratory distress syndrome in an infant. Cell Med. (2018) 10:2155179018759434. doi: 10.1177/2155179018759434
19. Powell SB, Silvestri JM. Safety of intratracheal administration of human umbilical cord blood derived mesenchymal stromal cells in extremely low birth weight preterm infants. J Pediatr. (2019) 210:209–13.e2. doi: 10.1016/j.jpeds.2019.02.029
20. Guess AJ, Daneault B, Wang R, Bradbury H, La Perle KMD, Fitch J, et al. Safety profile of good manufacturing practice manufactured interferon γ-primed mesenchymal stem/stromal cells for clinical trials. Stem Cells Transl Med. (2017) 6:1868–79. doi: 10.1002/sctm.16-0485
21. Ahn SY, Chang YS, Park WS. Stem cells for neonatal brain disorders. Neonatology. (2016) 109:377–83. doi: 10.1159/000444905
22. Mitsialis SA, Kourembanas S. Stem cell-based therapies for the newborn lung and brain: possibilities and challenges. Semin Perinatol. (2016) 40:138–51. doi: 10.1053/j.semperi.2015.12.002
23. Mueller M, Wolfs TG, Schoeberlein A, Gavilanes AW, Surbek D, Kramer BW. Mesenchymal stem/stromal cells-a key mediator for regeneration after perinatal morbidity? Mol Cell Pediatr. (2016) 3:6. doi: 10.1186/s40348-016-0034-x
24. Szpecht D, Szymankiewicz M, Nowak I, Gadzinowski J. Intraventricular hemorrhage in neonates born before 32 weeks of gestation-retrospective analysis of risk factors. Childs Nerv Syst. 2016) 32:1399–404. doi: 10.1007/s00381-016-3127-x
25. Gotardo JW, de Freitas Valle Volkmer N, Stangler GP, Dornelles AD, de Athayde Bohrer BB, Carvalho CG. Impact of peri-intraventricular haemorrhage and periventricular leukomalacia in the neurodevelopment of preterms: a systematic review and meta-analysis. PLoS ONE. (2019) 14:e0223427. doi: 10.1371/journal.pone.0223427
26. Kim ES, Ahn SY, Im GH, Sung DK, Park YR, Choi SH, et al. Human umbilical cord blood-derived mesenchymal stem cell transplantation attenuates severe brain injury by permanent middle cerebral artery occlusion in newborn rats. Pediatr Res. (2012) 72:277–84. doi: 10.1038/pr.2012.71
27. van Velthoven CT, Kavelaars A, van Bel F, Heijnen CJ. Mesenchymal stem cell transplantation changes the gene expression profile of the neonatal ischemic brain. Brain Behav Immun. (2011) 25:1342–8. doi: 10.1016/j.bbi.2011.03.021
28. Thomi G, Surbek D, Haesler V, Joerger-Messerli M, Schoeberlein A. Exosomes derived from umbilical cord mesenchymal stem cells reduce microglia-mediated neuroinflammation in perinatal brain injury. Stem Cell Res Ther. (2019) 10:105. doi: 10.1186/s13287-019-1207-z
29. Allen KA, Brandon DH. Hypoxic ischemic encephalopathy: pathophysiology and experimental treatments. Newborn Infant Nurs Rev. (2011) 11:125–33. doi: 10.1053/j.nainr.2011.07.004
30. Park WS, Sung SI, Ahn SY, Yoo HS, Sung DK, Im GH, et al. Hypothermia augments neuroprotective activity of mesenchymal stem cells for neonatal hypoxic-ischemic encephalopathy. PLoS ONE. (2015) 10:e0120893. doi: 10.1371/journal.pone.0120893
31. Bruschettini M, Romantsik O, Moreira A, Ley D, Thébaud B. Stem cell-based interventions for the prevention of morbidity and mortality following hypoxic-ischaemic encephalopathy in newborn infants. Cochrane Database Syst Rev. (2018) 2018:CD013202. doi: 10.1002/14651858.CD013202
32. Cánovas-Ahedo M, Alonso-Alconada D. Combined therapy in neonatal hypoxic-ischaemic encephalopathy. An Pediatría. (2019) 91:59–9.e6. doi: 10.1016/j.anpede.2019.04.007
33. McDonald CA, Djuliannisaa Z, Petraki M, Paton MCB, Penny TR, Sutherland AE, et al. Intranasal delivery of mesenchymal stromal cells protects against neonatal hypoxic-ischemic brain injury. Int J Mol Sci. (2019) 20:2449. doi: 10.3390/ijms20102449
34. Wagenaar N, Nijboer CH, van Bel F. Repair of neonatal brain injury: bringing stem cell-based therapy into clinical practice. Dev Med Child Neurol. (2017) 59:997–1003. doi: 10.1111/dmcn.13528
35. Ophelders DRMG, Wolfs TGAM, Jellema RK, Zwanenburg A, Andriessen P, Delhaas T, et al. Mesenchymal stromal cell-derived extracellular vesicles protect the fetal brain after hypoxia-ischemia. Stem Cells Transl Med. (2016) 5:754–63. doi: 10.5966/sctm.2015-0197
36. Wang Y, Long W, Cao Y, Li J, You L, Fan Y. Mesenchymal stem cell-derived secretomes for therapeutic potential of premature infant diseases. Biosci Rep. (2020) 40:BSR20200241. doi: 10.1042/BSR20200241
37. Chen A, Siow B, Blamire AM, Lako M, Clowry GJ. Transplantation of magnetically labeled mesenchymal stem cells in a model of perinatal brain injury. Stem Cell Res. (2010) 5:255–66. doi: 10.1016/j.scr.2010.08.004
38. Zhu LH, Bai X, Zhang N, Wang SY, Li W, Jiang L. Improvement of human umbilical cord mesenchymal stem cell transplantation on glial cell and behavioral function in a neonatal model of periventricular white matter damage. Brain Res. (2014) 1563:13–21. doi: 10.1016/j.brainres.2014.03.030
39. Morioka C, Komaki M, Taki A, Honda I, Yokoyama N, Iwasaki K, et al. Neuroprotective effects of human umbilical cord-derived mesenchymal stem cells on periventricular leukomalacia-like brain injury in neonatal rats. Inflamm Regen. (2017) 37:1. doi: 10.1186/s41232-016-0032-3
40. Niemarkt HJ, de Meij TG, van Ganzewinkel CJ, de Boer NKH, Andriessen P, Hütten MC, et al. Necrotizing enterocolitis, gut microbiota, and brain development: role of the brain-gut axis. Neonatology. (2019) 115:423–31. doi: 10.1159/000497420
41. Lin H, Mao S, Shi L, Tou J, Du L. Clinical characteristic comparison of low birth weight and very low birth weight preterm infants with neonatal necrotizing enterocolitis: a single tertiary center experience from eastern China. Pediatr Surg Int. (2018) 34:1201–7. doi: 10.1007/s00383-018-4339-9
42. Lu Q, Cheng S, Zhou M, Yu J. Risk factors for necrotizing enterocolitis in neonates: a retrospective case-control study. Pediatr Neonatol. (2017) 58:165–70. doi: 10.1016/j.pedneo.2016.04.002
43. Zozaya C, Shah J, Pierro A, Zani A, Synnes A, Lee S, et al. Neurodevelopmental and growth outcomes of extremely preterm infants with necrotizing enterocolitis or spontaneous intestinal perforation. J Pediatr Surg. (2020). doi: 10.1016/j.jpedsurg.2020.05.013. [Epub ahead of print].
44. Eaton S, Zani A, Pierro A, De Coppi P. Stem cells as a potential therapy for necrotizing enterocolitis. Expert Opin Biol Ther. (2013) 13:1683–9. doi: 10.1517/14712598.2013.849690
45. Shi X, Chen Q, Wang F. Mesenchymal stem cells for the treatment of ulcerative colitis: a systematic review and meta-analysis of experimental and clinical studies. Stem Cell Res Ther. (2019) 10:266. doi: 10.1186/s13287-019-1336-4
46. Lightner AL, Wang Z, Zubair AC, Dozois EJ. A systematic review and meta-analysis of mesenchymal stem cell injections for the treatment of perianal Crohn's disease: progress made and future directions. Dis Colon Rectum. (2018) 61:629–40. doi: 10.1097/DCR.0000000000001093
47. Tayman C, Uckan D, Kilic E, Ulus AT, Tonbul A, Hirfanoglu IM, et al. Mesenchymal stem cell therapy in necrotizing enterocolitis: a rat study. Pediatr Res. (2011) 70:489–94. doi: 10.1203/PDR.0b013e31822d7ef2
48. McCulloh CJ, Olson JK, Zhou Y, Wang Y, Besner GE. Stem cells and necrotizing enterocolitis: a direct comparison of the efficacy of multiple types of stem cells. J Pediatr Surg. (2017) 52:999–1005. doi: 10.1016/j.jpedsurg.2017.03.028
49. Overcash RT, DeUgarte DA, Stephenson ML, Gutkin RM, Norton ME, Parmar S, et al. Factors associated with gastroschisis outcomes. Obstet Gynecol. (2014) 124:551–7. doi: 10.1097/AOG.0000000000000425
50. Feng C, Graham CD, Connors JP, Brazzo J, Pan AHS, Hamilton JR, et al. Transamniotic stem cell therapy (TRASCET) mitigates bowel damage in a model of gastroschisis. J Pediatr Surg. (2016) 51:56–61. doi: 10.1016/j.jpedsurg.2015.10.011
51. Feng C, Graham CD, Shieh HF, Brazzo JA, Connors JP, Rohrer L, et al. Transamniotic stem cell therapy (TRASCET) in a leporine model of gastroschisis. J Pediatr Surg. (2017) 52:30–4. doi: 10.1016/j.jpedsurg.2016.10.016
52. Davies PS, Powell AE, Swain JR, Wong MH. Inflammation and proliferation act together to mediate intestinal cell fusion. PLoS ONE. (2009) 4:e6530. doi: 10.1371/journal.pone.0006530
53. de Jong JH, Rodermond HM, Zimberlin CD, Lascano V, De Sousa E, Melo F, Richel DJ, et al. Fusion of intestinal epithelial cells with bone marrow derived cells is dispensable for tissue homeostasis. Sci Rep. (2012) 2:271. doi: 10.1038/srep00271
54. Zani A, Cananzi M, Fascetti-Leon F, Lauriti G, Smith VV, Bollini S, et al. Amniotic fluid stem cells improve survival and enhance repair of damaged intestine in necrotising enterocolitis via a COX-2 dependent mechanism. Gut. (2014) 63:300–9. doi: 10.1136/gutjnl-2012-303735
55. Liu H, Zhang J, Liu CY, Wang IJ, Sieber M, Chang J, et al. Cell therapy of congenital corneal diseases with umbilical mesenchymal stem cells: Lumican null mice. PLoS ONE. (2010) 5:e10707. doi: 10.1371/journal.pone.0010707
56. Coulson-Thomas VJ, Caterson B, Kao WWY. Transplantation of human umbilical mesenchymal stem cells cures the corneal defects of mucopolysaccharidosis vii mice. Stem Cells. (2013) 31:2116–26. doi: 10.1002/stem.1481
57. Sun M, Chen S, Adams SM, Florer JB, Liu H, Kao WWY, et al. Collagen V is a dominant regulator of collagen fibrillogenesis: dysfunctional regulation of structure and function in a corneal-stroma-specific Col5a1-null mouse model. J Cell Sci. (2011) 124:4096–105. doi: 10.1242/jcs.091363
58. Call M, Elzarka M, Kunesh M, Hura N, Birk DE, Kao WW. Therapeutic efficacy of mesenchymal stem cells for the treatment of congenital and acquired corneal opacity. Mol Vis. (2019) 25:415–26.
59. Xu W, Cheng W, Cui X, Xu G. Therapeutic effect against retinal neovascularization in a mouse model of oxygen-induced retinopathy: bone marrow-derived mesenchymal stem cells versus conbercept. BMC Ophthalmol. (2020) 20:7. doi: 10.1186/s12886-019-1292-x
60. Zhang L, Coulson-Thomas VJ, Ferreira TG, Kao WWY. Mesenchymal stem cells for treating ocular surface diseases. BMC Ophthalmol. (2015) 15:54–65. doi: 10.1186/s12886-015-0138-4
61. Navas A, Magaña-Guerrero FS, Domínguez-López A, Chávez-García C, Partido G, Graue-Hernández EO, et al. Anti-inflammatory and anti-fibrotic effects of human amniotic membrane mesenchymal stem cells and their potential in corneal repair. Stem Cells Transl Med. (2018) 7:906–17. doi: 10.1002/sctm.18-0042
62. Song HB, Park SY, Ko JH, Park JW, Yoon CH, Kim DH, et al. Mesenchymal stromal cells inhibit inflammatory lymphangiogenesis in the cornea by suppressing macrophage in a TSG-6-dependent manner. Mol Ther. (2018) 26:162–72. doi: 10.1016/j.ymthe.2017.09.026
63. Wang S, Huang S, Gong L, Yuan Z, Wong J, Lee J, et al. Human neonatal thymus mesenchymal stem cells promote neovascularization and cardiac regeneration. Stem Cells Int. (2018) 2018:8503468. doi: 10.1155/2018/8503468
64. Doster DL, Jensen AR, Khaneki S, Markel TA. Mesenchymal stromal cell therapy for the treatment of intestinal ischemia: defining the optimal cell isolate for maximum therapeutic benefit. Cytotherapy. (2016) 18:1457–70. doi: 10.1016/j.jcyt.2016.08.001
65. Alvarez-Dolado M, Pardal R, Garcia-Verdugo JM, Fike JR, Lee HO, Pfeffer K, et al. Fusion of bone-marrow-derived cells with Purkinje neurons, cardiomyocytes and hepatocytes. Nature. (2003) 425:968–73. doi: 10.1038/nature02069
66. Kang K, Chuai JB, Xie B dong, Li J zhong, Qu H, Wu H, et al. Mesenchymal stromal cells from patients with cyanotic congenital heart disease are optimal candidate for cardiac tissue engineering. Biomaterials. (2020) 230:119574. doi: 10.1016/j.biomaterials.2019.119574
67. Elman JS, Li M, Wang F, Gimble JM, Parekkadan B. A comparison of adipose and bone marrow-derived mesenchymal stromal cell secreted factors in the treatment of systemic inflammation. J Inflamm. (2014) 11:1. doi: 10.1186/1476-9255-11-1
68. Lombardo E, van der Poll T, DelaRosa O, Dalemans W. Mesenchymal stem cells as a therapeutic tool to treat sepsis. World J Stem Cells. (2015) 7:368–79. doi: 10.4252/wjsc.v7.i2.368
69. Zhao X, Liu D, Gong W, Zhao G, Liu L, Yang L, et al. The toll-like receptor 3 ligand, poly(I:C), improves immunosuppressive function and therapeutic effect of mesenchymal stem cells on sepsis via inhibiting MiR-143. Stem Cells. (2014) 32:521–33. doi: 10.1002/stem.1543
70. Korneev K V. [Mouse models of sepsis and septic shock]. Mol Biol. (2019) 53:799–814. doi: 10.1134/S0026893319050108
71. Horák J, Nalos L, Martínková V, Beneš J, Štengl M, Matějovič M. Mesenchymal stem cells in sepsis and associated organ dysfunction: a promising future or blind alley? Stem Cells Int. (2017) 2017:7304121. doi: 10.1155/2017/7304121
72. Spees JL, Lee RH, Gregory CA. Mechanisms of mesenchymal stem/stromal cell function. Stem Cell Res Ther. (2016) 7:125. doi: 10.1186/s13287-016-0363-7
73. Zhu Y, Xu L, Collins JJP, Vadivel A, Cyr-Depauw C, Zhong S, et al. Human umbilical cord mesenchymal stromal cells improve survival and bacterial clearance in neonatal sepsis in rats. Stem Cells Dev. (2017) 26:1054–64. doi: 10.1089/scd.2016.0329
74. Krasnodembskaya A, Song Y, Fang X, Gupta N, Serikov V, Lee JW, et al. Antibacterial effect of human mesenchymal stem cells is mediated in part from secretion of the antimicrobial peptide LL-37. Stem Cells. (2010) 28:2229–38. doi: 10.1002/stem.544
75. Lee JW, Krasnodembskaya A, McKenna DH, Song Y, Abbott J, Matthay MA. Therapeutic effects of human mesenchymal stem cells in ex vivo human lungs injured with live bacteria. Am J Respir Crit Care Med. (2013) 187:751–60. doi: 10.1164/rccm.201206-0990OC
76. Park J, Kim S, Lim H, Liu A, Hu S, Lee J, et al. Therapeutic effects of human mesenchymal stem cell microvesicles in an ex vivo perfused human lung injured with severe E. coli pneumonia. Thorax. (2019) 74:43–50. doi: 10.1136/thoraxjnl-2018-211576
77. Hanley PJ, Mei Z, da Graca Cabreira-Hansen M, Klis M, Li W, Zhao Y, et al. Manufacturing mesenchymal stromal cells for phase I clinical trials. Cytotherapy. (2013) 15:416–22. doi: 10.1016/j.jcyt.2012.09.007
78. Levenberg S, Huang NF, Lavik E, Rogers AB, Itskovitz-Eldor J, Langer R. Differentiation of human embryonic stem cells on three-dimensional polymer scaffolds. Proc Natl Acad Sci USA. (2003) 100:12741–6. doi: 10.1073/pnas.1735463100
79. Koh B, Sulaiman N, Fauzi MB, Law JX, Ng MH, Idrus RBH, et al. Three dimensional microcarrier system in mesenchymal stem cell culture: a systematic review. Cell Biosci. (2020) 10:75. doi: 10.1186/s13578-020-00438-8
80. Hassan MNF Bin, Yazid MD, Yunus MHM, Chowdhury SR, Lokanathan Y, Idrus RBH, et al. Large-scale expansion of human mesenchymal stem cells. Stem Cells Int. (2020) 2020:9529465. doi: 10.1155/2020/9529465
81. Adamiak M, Cheng G, Bobis-Wozowicz S, Zhao L, Kedracka-Krok S, Samanta A, et al. Induced pluripotent stem cell (iPSC)-derived extracellular vesicles are safer and more effective for cardiac repair than iPSCs. Circ Res. (2018) 122:296–309. doi: 10.1161/CIRCRESAHA.117.311769
82. Porzionato A, Zaramella P, Dedja A, Guidolin D, Van Wemmel K, Macchi V, et al. Intratracheal administration of clinical-grade mesenchymal stem cell-derived extracellular vesicles reduces lung injury in a rat model of bronchopulmonary dysplasia. Am J Physiol Lung Cell Mol Physiol. (2019) 316:L6–19. doi: 10.1152/ajplung.00109.2018
83. Théry C, Witwer KW, Aikawa E, Alcaraz MJ, Anderson JD, Andriantsitohaina R, et al. Minimal information for studies of extracellular vesicles 2018 (MISEV2018): a position statement of the International Society for Extracellular Vesicles and update of the MISEV2014 guidelines. J Extracell vesicles. (2018) 7:1535750. doi: 10.1080/20013078.2018.1461450
84. Lokanathan Y, Law JX, Yazid MD, Chowdhury SR, Busra MFM, Sulaiman N, et al. Mesenchymal stem cells and their role in hypoxia-induced injury. In: Haider KH, editor. Stem Cells: From Myth to Reality and Evolving. Boston: De Gruyter (2019). p. 166–86.
85. Willis GR, Kourembanas S, Mitsialis SA. Toward exosome-based therapeutics: isolation, heterogeneity, and fit-for-purpose potency. Front Cardiovasc Med. (2017) 4:63. doi: 10.3389/fcvm.2017.00063
86. Bachurski D, Schuldner M, Nguyen P-H, Malz A, Reiners KS, Grenzi PC, et al. Extracellular vesicle measurements with nanoparticle tracking analysis - an accuracy and repeatability comparison between nanoSight NS300 and ZetaView. J Extracell vesicles. (2019) 8:1596016. doi: 10.1080/20013078.2019.1596016
87. Aliotta JM, Pereira M, Wen S, Dooner MS, Del Tatto M, Papa E, et al. Exosomes induce and reverse monocrotaline-induced pulmonary hypertension in mice. Cardiovasc Res. (2016) 110:319–30. doi: 10.1093/cvr/cvw054
88. Sjöqvist S, Ishikawa T, Shimura D, Kasai Y, Imafuku A, Bou-Ghannam S, et al. Exosomes derived from clinical-grade oral mucosal epithelial cell sheets promote wound healing. J Extracell vesicles. (2019) 8:1565264. doi: 10.1080/20013078.2019.1565264
89. Zhu X, Badawi M, Pomeroy S, Sutaria DS, Xie Z, Baek A, et al. Comprehensive toxicity and immunogenicity studies reveal minimal effects in mice following sustained dosing of extracellular vesicles derived from HEK293T cells. J Extracell vesicles. (2017) 6:1324730. doi: 10.1080/20013078.2017.1324730
90. Mendt M, Kamerkar S, Sugimoto H, McAndrews KM, Wu CC, Gagea M, et al. Generation and testing of clinical-grade exosomes for pancreatic cancer. JCI insight. (2018) 3:e99263. doi: 10.1172/jci.insight.99263
91. Duijvesz D, Luider T, Bangma CH, Jenster G. Exosomes as biomarker treasure chests for prostate cancer. Eur Urol. (2011) 59:823–31. doi: 10.1016/j.eururo.2010.12.031
92. Li P, Kaslan M, Lee SH, Yao J, Gao Z. Progress in exosome isolation techniques. Theranostics. (2017) 7:789–804. doi: 10.7150/thno.18133
93. Antounians L, Tzanetakis A, Pellerito O, Catania VD, Sulistyo A, Montalva L, et al. The regenerative potential of amniotic fluid stem cell extracellular vesicles: lessons learned by comparing different isolation techniques. Sci Rep. (2019) 9:1837. doi: 10.1038/s41598-018-38320-w
94. Tassew NG, Charish J, Shabanzadeh AP, Luga V, Harada H, Farhani N, et al. Exosomes mediate mobilization of autocrine Wnt10b to promote axonal regeneration in the injured CNS. Cell Rep. (2017) 20:99–111. doi: 10.1016/j.celrep.2017.06.009
95. Tabak S, Schreiber-Avissar S, Beit-Yannai E. Extracellular vesicles have variable dose-dependent effects on cultured draining cells in the eye. J Cell Mol Med. (2018) 22:1992–2000. doi: 10.1111/jcmm.13505
96. Willis GR, Fernandez-Gonzalez A, Anastas J, Vitali SH, Liu X, Ericsson M, et al. Mesenchymal stromal cell exosomes ameliorate experimental bronchopulmonary dysplasia and restore lung function through macrophage immunomodulation. Am J Respir Crit Care Med. (2018) 197:104–16. doi: 10.1164/rccm.201705-0925OC
Keywords: cell therapy, clinical trial, neonatal diseases, extracellular vesicles, mesenchymal stromal cells
Citation: Liau LL, Al-Masawa ME, Koh B, Looi QH, Foo JB, Lee SH, Cheah FC and Law JX (2020) The Potential of Mesenchymal Stromal Cell as Therapy in Neonatal Diseases. Front. Pediatr. 8:591693. doi: 10.3389/fped.2020.591693
Received: 05 August 2020; Accepted: 05 October 2020;
Published: 04 November 2020.
Edited by:
Eugene Dempsey, University College Cork, IrelandReviewed by:
Kazumichi Fujioka, Kobe University, JapanGangaram Akangire, Children's Mercy Hospital, United States
Copyright © 2020 Liau, Al-Masawa, Koh, Looi, Foo, Lee, Cheah and Law. This is an open-access article distributed under the terms of the Creative Commons Attribution License (CC BY). The use, distribution or reproduction in other forums is permitted, provided the original author(s) and the copyright owner(s) are credited and that the original publication in this journal is cited, in accordance with accepted academic practice. No use, distribution or reproduction is permitted which does not comply with these terms.
*Correspondence: Jia Xian Law, danieljx08@gmail.com; lawjx@ppukm.ukm.edu.my