- 1Research Centre for Medical Genetics, Moscow, Russia
- 2Department of Paediatrics, Russian Medical Academy of Continuous Postgraduate Education, Moscow, Russia
- 3Voino-Yasenetsky Krasnoyarsk State Medical University, Krasnoyarsk, Russia
- 4Department of Paediatrics, Stavropol State Medical University, Stavropol, Russia
Background: The problem of vitamin D deficiency is particularly relevant for the entire territory of Russia, since most parts of the country are located above the 42nd geographical latitude and the residents are therefore at risk of vitamin D deficiency. Despite the urgency of the problem, a comprehensive study of the molecular and genetic mechanisms and exogenous factors of vitamin D deficiency in children living in various geographical areas of the Russian Federation has not been conducted. Different variants in the loci of the genes responsible for the synthesis, hydroxylation, and transport of vitamin D (such as DHCR7, CYP2R1, CYP24A1, and GC), as well as VDR gene polymorphisms may also be associated with the risk of vitamin D deficiency. The aim of this study was to analyze the influence of exogenous factors on the blood levels of 25-hydroxyvitamin D (25(OH)D) in children of three regions of the Russian Federation, as well as the relationship of blood 25(OH)D levels with polymorphic variants of cytochrome P450 genes and VDR gene.
Methods: We conducted blood 25(OH)D level analysis in 333 healthy children and adolescents in three regions located in different geographical zones of the Russian Federation. We studied the polymorphic variants c.1075A>C (I359L, rs1057910, CYP2C9*3) and c.430C>T (R144C, rs1799853, CYP2C9*2) in the CYP2C9 gene, c.1334T>C (M445T, rs4986910, CYP3A4*3), and CYP3A4*1B (c.-392C>T, rs2740574) in the CYP3A4 gene, 1846G>A, (rs3892097, CYP2D6*4) in the CYP2D6gene, TaqI (NM_000376.2: c.1056T>C; rs731236), FokI (NM_000376.2:c.2T>C; (rs2228570), and BsmI (NM_000376.2: c.1024+283G>A; rs1544410) in the VDR gene. We also analyzed the influence of exogenous factors on the level of 25(OH)D in children of the three study regions, as well as the relationship of the level of 25(OH)D with variants CYP2C9*2 (c.430C>T; R144C), CYP2C9*3 (c,1075A>C; I359L), CYP2D6*4 (1846G>A), CYP3A4*3 (c.1334T>C), and CYP3A4*1B (c.-392C>T) and rs731236, rs2228570 and rs1544410 in the VDR gene.
Results: We found that the blood level of 25(OH)D depended on the geographical location and the number of sunny days per year. The average blood level of 25(OH)D in adolescent boys was statistically significantly lower than in girls of this age group. The level of 25(OH)D also significantly depended on the prophylactic dose of cholecalciferol administered to the subjects. In the study, it was shown that a dose of cholecalciferol ≥1,000 IU per day can achieve a normal level of 25(OH)D in healthy children. We found no statistically significant association between single-nucleotide polymorphic variants of cytochrome P450 genes (CYP2C9*3, CYP3A4*3, CYP2C9*2, CYP2D6*4, and CYP3A4*1B) and blood level of 25(OH)D in the subjects. We also did not find a relationship between the TaqI, FokI, and BsmI polymorphisms of the VDR gene and serum 25(OH)D concentration.
Conclusion: Exogenous factors (time of year, place of residence, and prophylactic administration of cholecalciferol), as well as endogenous factors (age and sex), play a determining role in the development of vitamin D deficiency and insufficiency; in contrast to genetic factors—polymorphic variants of the genes of xenobiotic phase 1 enzymes (CYP2C9, CYP2C19, CYP2D6, and CYP3A4) and the VDR gene—which do not play such role. This study shows the need to create a diagnostic algorithm for Vitamin D deficiency based on the age, season of the year, and prophylactic dose of cholecalciferol.
Introduction
Vitamin D, which belongs to the group of fat-soluble vitamins, plays important roles in the human body. Its main role is in the metabolism of bone tissue—maintaining mineralization, bone growth, and the process of bone remodeling. However, the functions of vitamin D are not limited to the regulation of calcium-phosphorus metabolism: it also affects the processes of cell proliferation and differentiation, neuromuscular impulse transmition, and modulation of immune response and inflammation (1, 2). Important for Vitamin D synthesis in the body is the presence of ultraviolet rays of sunlight and their contact with the skin (3–5). Vitamin D, obtained from food and in the form of food additives, as well as formed during exposure to the sun is biologically inert. To be converted into an active form−1,25(OH)2D, it must undergo two processes of hydroxylation in the body. At the first stage of hydroxylation in the liver by the action of 25-hydroxylase (CYP2R1), 25-hydroxyvitamin D [25(OH)D, also called calcidiol] is formed. While the second hydroxylation in the kidneys by the action of CYP27B1 (1α-hydroxylase), leads to formation of the biologically active form, 1,25(OH)2D (6). In enzyme identification studies, cytochrome P450 isoforms—CYP2C9, CYP3A4, and CYP2D6—have been shown to participate in vitamin D hydroxylation reactions (7, 8). It was also found that 1,25(OH)2D induces the expression of the CYP3A4, CYP2B6, and CYP2C9 genes in primary human hepatocytes, and vitamin D receptors (VDRs) are able to bind and transactivate various motifs recognized by xenobiotics via the X-pregnan receptor and constitutive androstane receptors in the promoter regions of these cytochrome genes (9).
Due to the low content of vitamin D in most foods, the main source of this vitamin is its formation under the influence of ultraviolet rays. Recently, due to reduction in sunlight, vitamin D deficiency has become very common in both adults and children (10). The problem of vitamin D deficiency is particularly relevant in the entire territory of Russia, since most parts of the country are located above the 42nd geographical latitude, and the residents are thus at risk of vitamin D deficiency (11). However, no research has been conducted about vitamin D deficiency for different regions and different seasons of the year in Russia.
Different variants in the loci of genes (such as DHCR7, CYP2R1, CYP24A1, and GC) that are responsible for vitamin D synthesis, hydroxylation, and transport, as well as VDR gene polymorphisms, may be associated with the risk of vitamin D deficiency (12, 13). Most tissues and cells in the body contain VDR. Following the genomic pathway in cells the active form of vitamin D binds to VDR and forms a complex. In the nucleus, this complex heterodimerizes with the retinoic acid x-receptor and affects the transcription of vitamin D-dependent genes. VDR is a nuclear transcription factor and has similarities to steroid and thyroid hormone receptors. It is encoded by the VDR gene located on chromosome 12 at position 13.11 (12q13.11).
The VDR gene has 11 exons (8 of them encoding) and contains approximately 75 kb. Several polymorphic variants of the VDR gene have been identified, but the most studied are four single-nucleotide polymorphic variants, which are traditionally designated by the names of the restriction endonucleases with which they were detected: BsmI, ApaI, TaqI, and FokI (14). The TaqI (NM_000376.2: c.1056T>C; rs731236) polymorphism is located in exon 11 (8th encoding exon) near the 3' end of the VDR gene and results in a synonymous change in p. Ile352 due to the replacement of c. 1056T>C. The FokI polymorphism (rs2228570, NM_000376.2:c.2T>C; NP_000367.1:p.Met1Thr) is located in the 4th exon of the gene (1st encoding exon), and its presence leads to a change in the starting codon. The VDR BsmI (rs1544410, NM_000376.2: c.1024+283G>A) and ApaI (rs7975232, NM_000376.2:c.1025-49G>T) VDR polymorphisms are located in intron 10 at the 3' end of the gene: these polymorphisms do not alter the amino acid sequence of the VDR protein, but they can affect gene expression through changes in mRNA stability, disruption of splicing sites for mRNA transcription, or changes in intron regulatory elements. Studies have established the association of VDR gene polymorphism with diseases such as osteoporosis, diabetes, tuberculosis, hyperparathyroidism, chronic renal failure, and various neoplasms, as well as various cardiovascular diseases (14–16). A relationship between vitamin D levels and certain diseases has also been found (17, 18). Despite this, no previous study on the prevalence of vitamin D deficiency in children in the Russian Federation including a comprehensive approach to assessing both genetic factors and exogenous factors has been done.
The purpose of this study was to analyze the influence of exogenous factors on the blood levels of 25(OH)D in children of three regions of the Russian Federation, as well as the relationship of blood 25(OH)D levels with polymorphic variants of CYP2C9*2 (c.430C>T; R144C), CYP2C9*3 (c.1075A>C; I359L), CYP2D6*4 (1846G>A), CYP3A4 (c.1334T>C), and CYP3A4*1B (c.-392C>T) and BsmI, TaqI, and FokI polymorphisms of the VDR gene.
Materials and Methods
Study Subjects
This prospective, non-randomized, multicenter, cohort study included healthy children and adolescents aged 0–18 years (n = 333): 145 subjects were from the Moscow region (64 boys and 81 girls; average age, 7.1 ± 4.7 years; median age, 6.3 years), 137 subjects were from the Krasnoyarsk territory (62 boys and 75 girls; average age, 7.0 ± 5.2 years; median age, 6.7 years), and 51 subjects were from the Stavropol territory (21 boys and 30 girls; average age, 8.1 ± 4.8 years; median age, 9.0 years). The subjects were examined in the four seasons of the year (Table 1).

Table 1. Number of samples and studies conducted in healthy subjects in different seasons of the year.
The inclusion criteria were absence of chronic diseases and acute respiratory infections 1 month before blood collection and signing of informed consent by parents/guardians. Children were included in the study after informed consent was obtained.
In the analysis, subjects were divided into three age groups (Table 2). Children were classified into the 0–3 years group, 4–10 years group, and 11–18 years group, in accordance with the periodization of children's ages by the Russian Federation (19).
The study was approved by the EC of the Research Center for Medical Genetics, Protocol “Algorithm for diagnosis and correction of vitamin D deficiency in children of the Russian Federation” No. 9, dated December 8, 2017.
25(OH)D Assay
All biochemical and genetic analyses were performed at the Research Center for Medical Genetics laboratory.
The blood samples were collected at the children's outpatient department (Moscow, Krasnoyarsk, Stavropol) and were transported and stored at 25°C. All samples were taken simultaneously at each season to determine the level of 25(OH)D. The analyses as performed using the same equipment by the same laboratory assistants.
The blood level of vitamin D was assessed by assaying its intermediate metabolite, 25(OH)D. Enzyme immunoassays were performed with EUROIMMUN AG kits (Germany) using the EnSpire flatbed spectrofluorometer (PerkinElmer, Finland), Biosan Laboratory Centrifuge LMC-3000, and Biosan Thermo-Shaker PST-60-HL-4.
Results of the 25(OH)D assay were interpreted in accordance with the recommendations of the International Society of Endocrinologists (2011): severe deficiency, 25(OH)D level <10 ng/ml; deficiency, 10–20 ng/ml; insufficiency, 21–29 ng/ml; and normal level, 30–100 ng/ml. Levels more than 100 ng/ml were considered excessive and required adjustment of the dose of vitamin D (20).
Data on the duration of sunshine (DS) (the number of solar hours per month during the years of study) were obtained from the archives of the meteorological service for each region (Table 3).
Molecular and Genetic Analysis
DNA was extracted from peripheral blood leukocytes using a commercial set of DNA extraction reagents manufactured by Wizard® (Genomic DNA Purification Kit).
The analysis of polymorphic variants of cytochrome and VDR genes was performed by polymerase chain reaction, followed by restriction with specific endonucleases. Polymorphisms of CYP2C9*3 (c.1075A>C; I359L, rs1057910), CYP3A4*3 (c.1334T>C; M445T, rs4986910), CYP2C9*2 (c.430C>T; R144C, rs1799853), CYP2D6*4 (1846G>A, rs3892097), and CYP3A4*1B (c.−2212392C>T, rs2740574), and polymorphic variants of the VDR gene— NM_000376.2: c.1056T>C (rs731236), NM_000376.2:c.2T>C (rs2228570), NM_000376.2: c.1024+283G>A (rs1544410)—were studied. The used primers, restriction endonucleases, the length of amplified fragments and the length of restriction products are shown in Table 4.
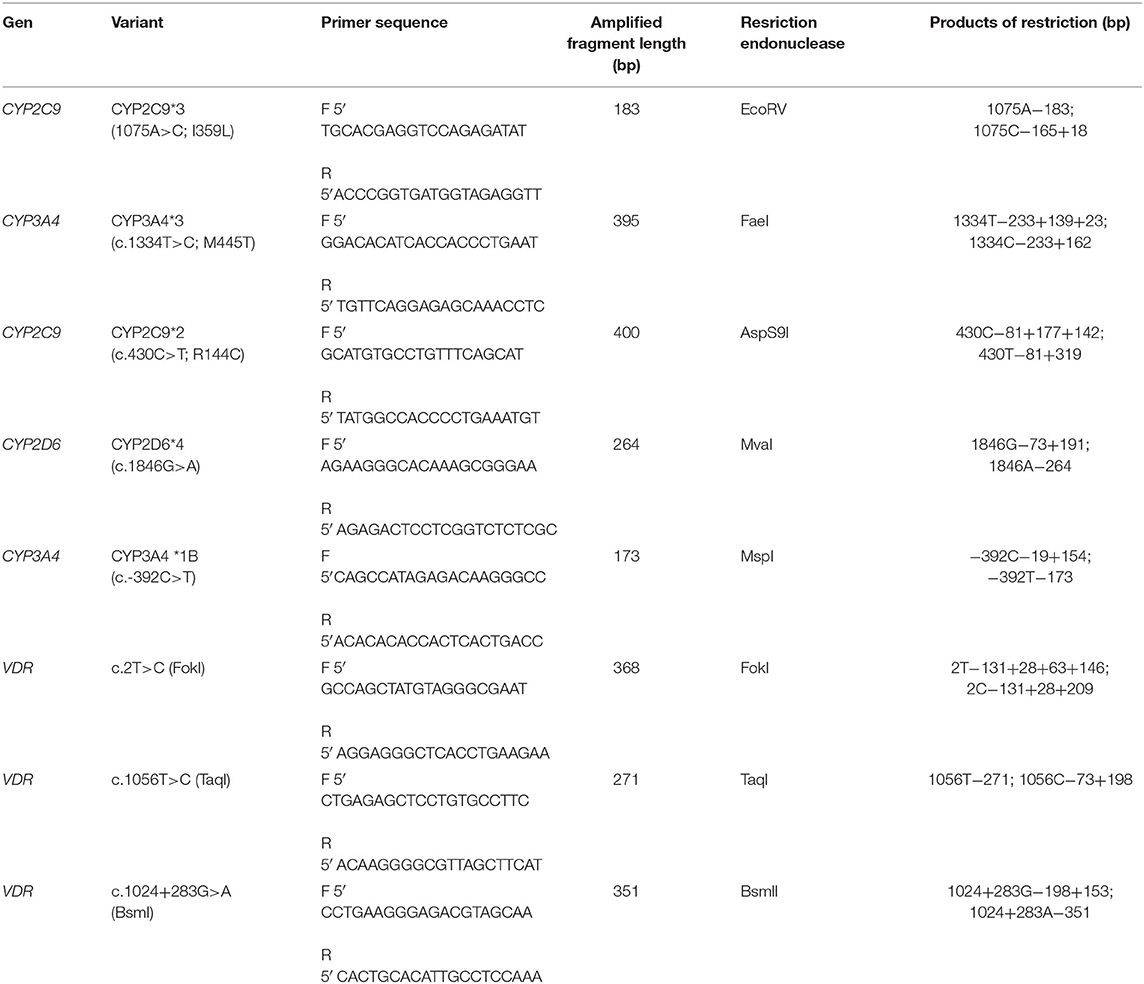
Table 4. Primer sequences and restriction endonucleases for determining polymorphic variants of cytochrome and VDR genes.
The genotypes of the variants studied were in Hardy-Weinberg Equilibrium (Table 5).
Statistical Analysis
Statistical data was processed using the IBM Statistical Package for the Social Sciences, version 24. Arithmetic mean and standard deviation were used as measures to describe the initial sample, while the interpretation of the results obtained (which do not have a normal distribution) was carried out using median, as well as lower and upper quartiles: Q1 (25%) and Q3 (75%). When comparing averages or medians, the Mann–Whitney U-test was used. In the study of genetic polymorphism among groups, Fischer's exact test was also used. Differences were considered statistically significant at p < 0.05.
For genetic studies, we applied the parametric one-way analysis of variance (pairwise comparison with Scheffe test), non-parametric analysis of variance Kruskal–Wallis (pairwise comparison by Mann–Whitney), Chi-square test of independence for contingency table, and analysis of the proportion of children with various contents vitamin D with the help of z-test of equality of shares.
Results
Children from 0–3 years of age had an average age of 1.92 ± 1.10 years; the median was 1.96 years (Q1–Q3= 1.00–2.77). The average age of children aged 4–10 years was 7.16 ± 2.02 years, and the median was 6.94 years (Q1–Q3= 5.52–8.72), while the average age of subjects aged 11–18 years was 13.85 ± 1.69 years, and the median was 13.59 years (Q1–Q3= 12.51–15.31).
The distribution of samples in the three age groups, depending on the prophylactic dose of cholecalciferol administered, is shown in Table 6. The number of subjects who did not receive prophylactic vitamin D was smallest at the age of 3 years; with age, the number of subjects who had vitamin D prophylaxis increased and was maximum among adolescents.

Table 6. The distribution of samples in the three study regions depending on prophylactic doses of cholecalciferol.
The number of blood samples received from healthy children who took prophylactic dose of cholecalciferol >1,000 IU in Stavropol was 57 (36.1%), and in Moscow and Krasnoyarsk, these numbers were significantly lower−8 (5.3%) and 25 (17.3%), respectively (p < 0.05). About half of the subjects did not receive vitamin D prophylaxis (Table 7).

Table 7. The distribution of samples in the three age groups, depending on prophylactic doses of cholecalciferol.
The results of the study showed that the average blood level of vitamin D in healthy subjects was 32.74 ± 17.47 ng/ml (median = 29.60, Q1–Q3 = 21.68–39.70). In general, a normal blood level of 25(OH)D was observed in 45.5% of cases, insufficiency in 30.1%, deficiency in 19.8%, and severe deficiency in 1.6%.
In a comparative analysis of the average level of 25(OH)D in children of different ages and sexes, significant difference was found between girls and boys in the older age group (p = 0.033). Adolescent boys had lower values of 25(OH)D, which was 25.70 ± 11.21 (median = 24.10, Q1–Q3= 17.40–30.51) ng/ml, compared with adolescent girls who had 28.70±14.01 (median = 26.18, Q1–Q3= 18.80–33.56) ng/ml, which allows them to be classified as a risk group at this age.
Calcidiol level reduced with increasing age: in healthy children, it decreased from 41.36 ng/ml at an early age to 26.94 ng/ml in adolescence (p < 0.001; Table 8). In addition, age patterns of vitamin D availability in the subjects were uniform—as a rule, from the maximum level reached in the first year of life, against the background of prophylactic administration of cholecalciferol, a progressive decrease occurred with increasing age, culminating in the development of vitamin D deficiency and insufficiency by school age.
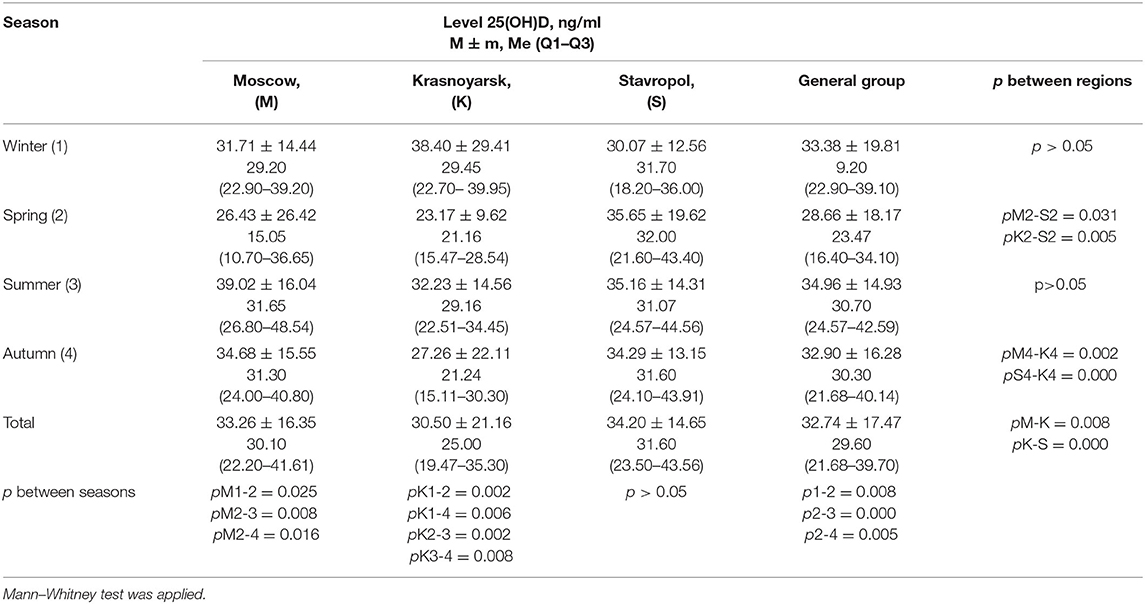
Table 8. Average level of 25(OH)D (ng/ml) in children and adolescents of the three study regions in different seasons.
In healthy children from 0–3 years of age, the average level of 25(OH)D was normal in all seasons. In children aged 4–10 years, significantly lower levels of 25(OH)D were recorded in spring than in summer. The average level of 25(OH)D in older children was below normal in all seasons, and the lowest levels were observed in spring and winter in adolescents (Figure 1).
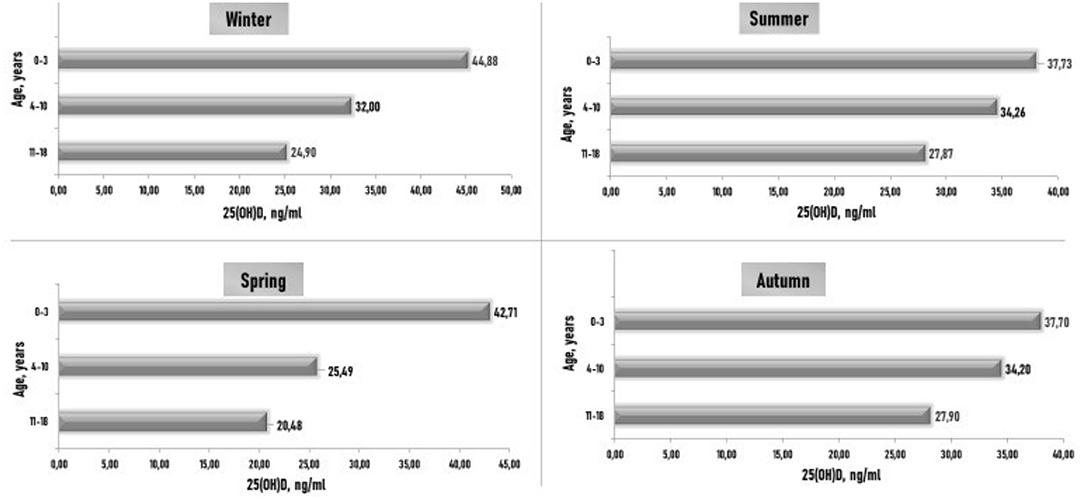
Figure 1. Subjects' blood 25(OH)D (ng/ml) concentrations in various age groups and different seasons. p is the level of significance of differences: p1–3 (1) < 0.05 is the significance between the level of 25(OH)D in winter and summer in children aged 0–3 years; p2–3 (2) < 0.05 is the significance between the levels of 25(OH)D in spring and in summer in children aged 4–10 years; p2–3 (3) < 0.05 is the significance between the levels of 25(OH)D in spring and in summer in children aged 11–18 years.
The blood levels of 25(OH)D in healthy subjects in different regions varied (Figure 2). The number of children with normal level of 25(OH)D was significantly higher in the Moscow region and the Stavropol territory than in the Krasnoyarsk territory (p < 0.05). There were no statistically significant differences in the number of subjects in the surveyed regions with respect to deficiency, insufficiency, and normal vitamin D availability.
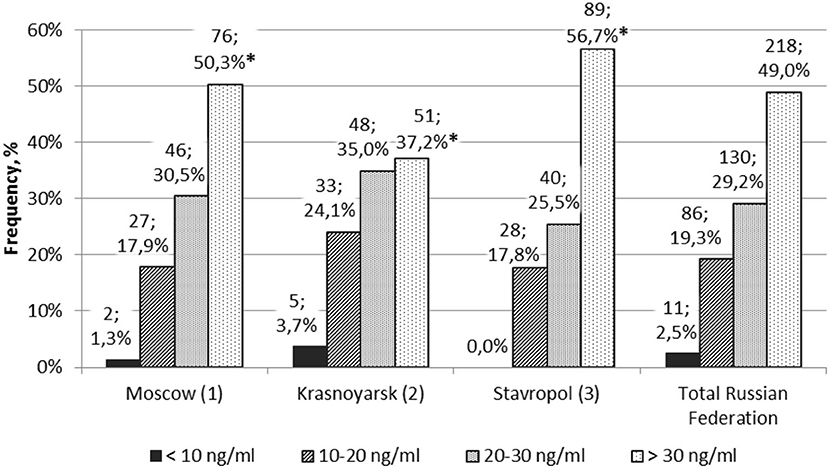
Figure 2. Frequency of healthy children and adolescents with different levels of vitamin D (n, %) in different regions. *p1–2 < 0.05 and p2–3 < 0.05 are Z criteria for comparing the proportions of children.
The average serum levels of 25(OH)D (ng/ml) among subjects in different seasons in the three regions of the Russian Federation studied are shown in Table 8. Significant differences were observed when the values of 25(OH)D were compared among the regions in spring and autumn. This corresponds to a greater number of sunny days in the Stavropol territory compared to the Moscow region and the Krasnoyarsk territory. Thus, in spring, the DS was 742.2 h in the Moscow region, 561.3 h in the Krasnoyarsk territory, and 687 h in the Stavropol territory. DS in the summer was 883.8 h in the Moscow region, 896.6 h in the Krasnoyarsk territory, and 1,013.3 h in the Stavropol territory. In the fall, DS was 391.7 h in the Moscow region, 345.2 h in the Krasnoyarsk territory, and 559.3 h in the Stavropol territory.
In the Stavropol territory, there were no significant differences in the blood levels of 25(OH)D across the seasons, which is primarily due to the fact that subjects in the region more often took prophylactic doses of cholecalciferol than subjects in other regions. In the Moscow region, higher blood levels of 25(OH)D were observed in summer than in spring. In the Krasnoyarsk territory, subjects had the highest average 25(OH)D levels in winter, while the lowest levels were detected in spring. This may be due to greater commitment to preventing vitamin D deficiency in patients during winter. In addition, the DS during winter was 2 times higher (175.6 h) in the Krasnoyarsk territory than in other regions: 81.7 h in the Moscow region and 87.4 h in the Stavropol territory. When comparing the level of vitamin D in the general group of children, a significantly low value of 25(OH)D is also celebrated in the spring.
The most important stage of the analysis was the comparison of calcidiol levels in healthy subjects with respect to the daily prophylactic dose of cholecalciferol administered (Figure 3).
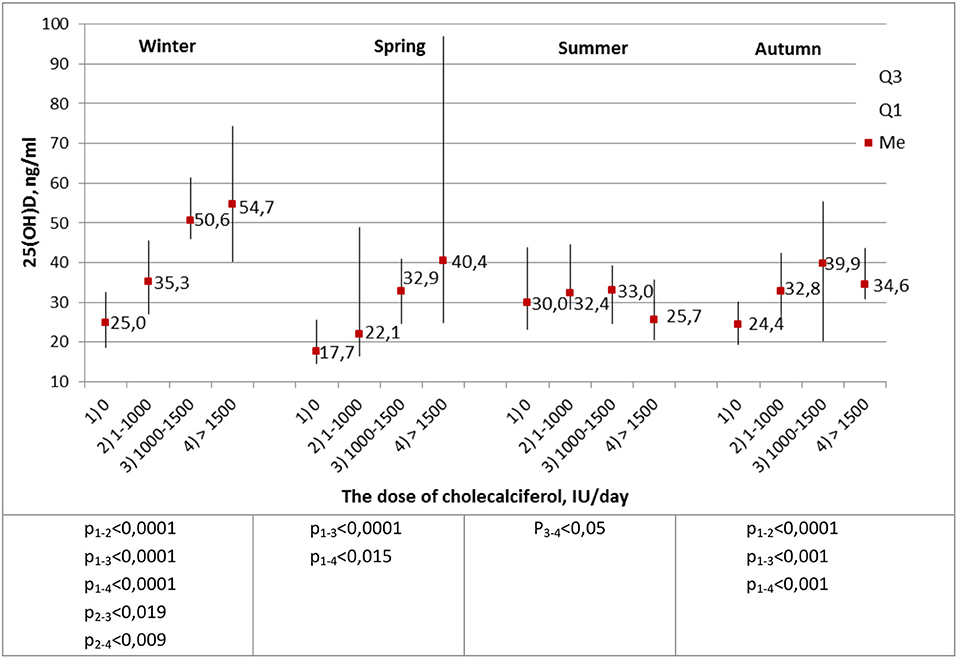
Figure 3. Average blood levels of 25(OH)D (ng/ml) in healthy children and adolescents, depending on the prophylactic dose of cholecalciferol. The Kruskal–Wallis criterion.
During winter, the level of 25(OH)D significantly increased depending on the prophylactic dose of cholecalciferol administered. In spring and autumn, significantly low concentrations of calcidiol were recorded in subjects who did not take prophylactic cholecalciferol. Thus, a dose of cholecalciferol ≥1,000 IU per day was enough to achieve normal blood level of 25(OH)D in healthy subjects, regardless of the season of the year.
The high level of 25(OH)D among subjects in the Stavropol territory can be explained by their high compliance with cholecalciferol prophylaxis, as some of them participated in studies on the prevalence of vitamin D deficiency and insufficiency in the Russian Federation (Rodnichok, Rodnichok 2) (21, 22).
The relationship between blood level of 25(OH)D in healthy subjects and variants of CYP2C9*3 (c.1075A>C; I359L, rs1057910), CYP3A4*3 (c.1334T>C; M445T, rs4986910), CYP2C9*2 (c.430C>T; R144C, rs1799853), CYP2D6*4 (1846G>A, rs3892097), and CYP3A4*1B (c.−392C>T, rs2740574) was studied in winter and spring due to low calcidiol levels in these seasons of the year. However, no relationships were established (Table 9).
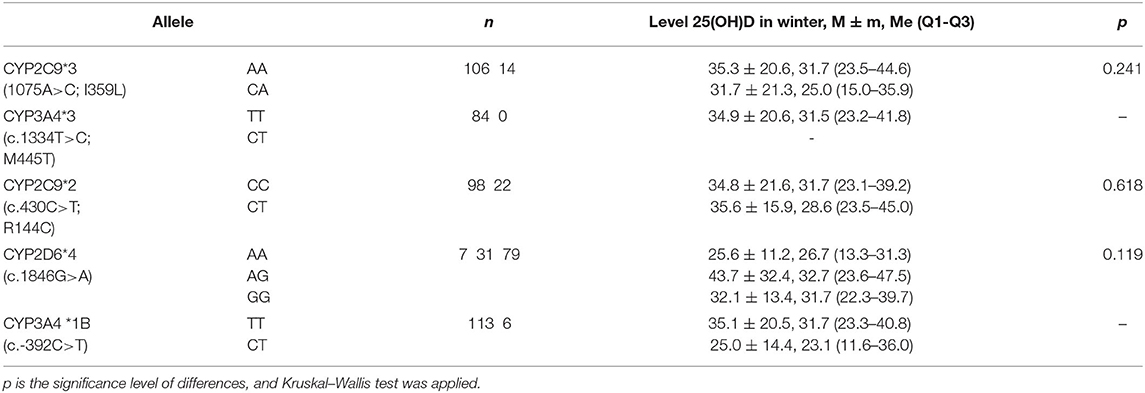
Table 9. Average level of 25(OH)D (ng/ml) in subjects in winter, depending on the genotypes of cytochrome gene polymorphisms.
Molecular effects, including effects on levels of hormones, growth and inflammation factors, proteins, and, of course, blood calcium levels, are manifested through Vitamin D receptors (23–25).
It was found that the TT and TC genotypes of the c.1056T>C (TaqI) polymorphism were more common (~4-fold) in the Russian population than the CC genotype (Table 10). The frequency of the C allele was 2 times lower than that of the T allele. For the c.2T>C (FokI) polymorphism, children with CC and TC genotypes predominated. The frequency of homozygous TT genotype in children was lower (17.83%) than the CC and TC genotypes. In relation to the polymorphic variant c.1024+283G>A (BsmI), GG and GA genotypes occurred more frequently than AA homozygotes (12.75%). The frequency of the A allele was 2 times lower than that of the G allele.
Discussion
The purpose of this study was to analyze the influence of exogenous factors on the blood levels of 25(OH)D in children of three regions of the Russian Federation, as well as the relationship of blood 25(OH)D levels with polymorphic variants of cytochrome P450 genes and VDR gene. The problem of vitamin D deficiency is currently relevant due to its widespread prevalence among both adults and children, including those in the Russian Federation. In this study, it was found that, in children, the level of 25(OH)D in the serum reduced with increasing age, and in adolescents, vitamin D deficiency was observed in all seasons of the year, with the lowest levels of 25(OH)D recorded in spring and winter.
A 2016 study of vitamin D deficiency in Europe also suggests that adolescents, on average, have a higher risk of vitamin D deficiency than the other age groups (26). In an American study on a sample of children from 1 to 11 years of age, the results showed lower levels of 25(OH)D in children aged 6–11 years (27). Russian studies have also shown better vitamin D availability in young children compared to adolescents (28, 29). It is obvious that age-related patterns of vitamin D availability in children of the Russian Federation depend on prophylactic administration of cholecalciferol at an early age, which is achieved by ongoing programs (the National Program for Optimizing Vitamin and Mineral Availability in Children in Russia, and the national program “vitamin D deficiency in children and adolescents in the Russian Federation: modern approaches to correction” (20). However, the recommendations of these programs are not followed in older children, and there is a progressive decrease in calcidiol levels, culminating in the development of vitamin D deficiency and insufficiency by school age.
Taking into account the peculiarities of vitamin D synthesis and the geographical location of most parts of the Russian Federation in the northern hemisphere above the 42nd parallel (30), the majority of children do not have optimal vitamin D levels in winter and spring (31).
The results of this study revealed that the region of residence also affects the availability of vitamin D. The regions that were studied are located at different geographical latitudes: Moscow at 55° s. l., Krasnoyarsk at 56° s. l., and Stavropol at 45° s. l. and, they have different numbers of sunny days per year and in different seasons of the year. The lowest DS during the year was recorded in the Krasnoyarsk territory, and this explains the low percentage of children with normal levels of 25(OH)D in the province.
The average level of 25(OH)D in adolescent boys was statistically significantly lower than that in adolescent girls. According to recent data, there are publications about similar conclusions among young adolescences (32).
We also found that the level of 25(OH)D significantly depended on the prophylactic dose of cholecalciferol administered. A dose of cholecalciferol ≥1,000 IU per day could achieve normal level of 25(OH)D in healthy children. This is consistent with the data of the national program (the National Program for Optimizing Vitamin and Mineral Availability in Children in Russia, and the national program “vitamin D deficiency in children and adolescents in the Russian Federation: modern approaches to correction”), which suggests a scheme for preventing hypovitaminosis D in children of the Russian Federation by administering 1,000–1,500 IU per day of cholecalciferol (20).
We therefore studied the frequency of alleles and genotypes of the VDR gene among the study subjects. The results obtained, presented in Table 9, completely coincide with the distribution of genotypes in the adult Russian population and partially coincide with the genotypes in populations of France and Japan (33–36).
We found no statistically significant association between single-nucleotide polymorphic variants of cytochrome P450 genes (CYP2C9*3, CYP3A4*3, CYP2C9*2, CYP2D6*4, and CYP3A4*1B) and blood level of 25(OH)D in the study subjects. As noted above, the enzymes encoded by these genes are involved in the process of hydroxylation, but they do not affect the content of serum vitamin D. In a 2016 review, Jolliffe et al. analyzed the association of 11 gene polymorphisms (DHCR7, CYP2R1, CYP3A4, CYP27A1, DBP, LRP2, CUB, CYP27B1, CYP24A1, VDR, and RXRA) with extracostal effects and circulating 25(OH) levels. In that study, statistically significant associations were found for 55 polymorphic variants in the 11 studied genes (37).
Based on the results of the present study, the distribution of frequencies of alleles of the VDR gene was studied (Table 10). Similar data were obtained by Kozlov et al. who studied the frequency of VDR genotypes by FokI and BsmI alleles and found that the Russian population had more carriers of the TT genotype than the CC genotype of TaqI polymorphism (28 and 13%, respectively) and that the GG genotype was predominant compared to the AA genotype of Bsml polymorphism (49 and 7%, respectively) (38). Baroncelli et al. studied 209 healthy children and revealed a 2-fold predominance of the G allele of BsmI polymorphism compared to the A allele (39). In a study of 202 children in Saudi Arabia, similar results were obtained (40).
Analysis of the level of 25(OH)D in the subjects did not reveal dependence on genotypes of the three polymorphic variants of the VDR gene (Table 11), which coincides with data from international studies (41). For example, in a study of 642 healthy children in Denmark, VDR gene polymorphism was not proven to have any effect on vitamin D levels (42). Similar results were also obtained in a survey of 222 adults in the UK (43). It can therefore be assumed that polymorphic variants do not affect the serum level of vitamin D, but can affect its function by changing its binding sites. In addition, a change in the carrier of the FokI polymorphic variant leads to the formation of a short form of the VDR protein, which leads to higher transcriptional activity and increased cytokine expression, and may be associated with autoimmune and infectious diseases (44).
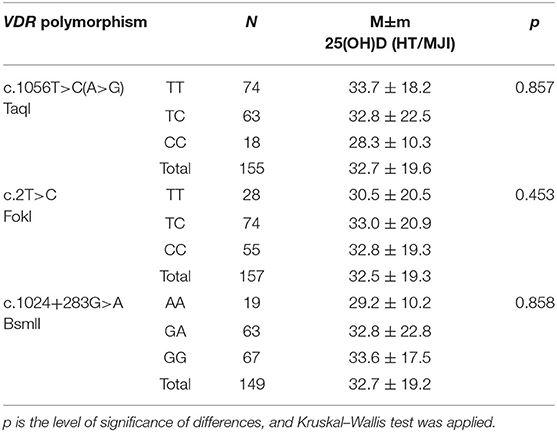
Table 11. Average level of 25(OH)D (ng/ml) in subjects depending on the genotypes of VDR gene polymorphisms.
The following conclusions can be drawn from this study. The subjects that were most at risk for vitamin D deficiency among healthy subjects in the study sample from the three studied regions of Russia were adolescents, especially males. In winter and spring, the lowest levels of 25(OH)D were recorded, regardless of the region of residence. Children of the Moscow region and the Stavropol territory had higher serum levels of 25(OH)D than children of the Krasnoyarsk territory. A prophylactic dose of ≥1,000 IU cholecalciferol per day can achieve normal level of 25(OH)D in healthy children.
However, there were limitations to this study. Samples used to evaluate the vitamin D levels were not taken from the same subject during different seasons of the year. The inclusion of new patients allowed us to evaluate prevention strategies for vitamin D deficiency in children during different seasons of the year. This study determined the duration of sunshine in the regions, not the children's exposure to the sun.
Therefore, for healthy children of the Russian Federation, exogenous factors such as time of the year, place of residence, and preventive administration of cholecalciferol, as well as intrinsic factors such as age and sex, play a determining role in the development of vitamin D deficiency; while genetic factors—polymorphic variants of the genes of phase 1 enzymes of xenobiotic metabolism (CYP2C9, CYP2C19, CYP2D6, CYP3A4) and the VDR gene—do not. A diagnostic algorithm incorporating age, season of the year, and prophylactic dose of cholecalciferol to identify patients at risk for vitamin D deficiency will be beneficial for the Russian population.
Data Availability Statement
The raw data supporting the conclusions of this article will be made available by the authors, without undue reservation.
Ethics Statement
The studies involving human participants were reviewed and approved by EC of the Research Center for Medical Genetics. Written informed consent to participate in this study was provided by the participants' legal guardian/next of kin.
Author Contributions
EK and SK performed the conceptualization and provided the proof outline for the research. IZ contributed to the original idea. NI and LK supervised the research. NP, AZ, EZ, VC, SD, AV, VS, YM, RB, and VK all carried out the research. EK wrote the manuscript with support from AZ, EZ, and EL. All authors discussed the results and contributed to the final manuscript.
Funding
This work was carried out within the framework of the state task of the Ministry of Education and Science of Russia and with the support of RFBR, Grant No. 18-015-00482.
Conflict of Interest
The authors declare that the research was conducted in the absence of any commercial or financial relationships that could be construed as a potential conflict of interest.
Abbreviations
DS, duration of sunshine; VDR, vitamin D receptor.
References
1. Bischoff-Ferrari HA, Burckhardt P, Quack-Loetscher K, Gerber B, I'Allemand D, Laimbacher J, et al. Vitamin D deficiency: evidence, safety, and recommendations for the Swiss population. In: Report Written by a Group of Experts on Behalf of the Federal Commission for Nutrition (FCN). (2012) Available online at: http://www.iccidd.org/p142000804.html
2. Kalinchenko S, Yu Pigarova EA, Gusakova DA, Pleshcheva AV. Vitamin D and urolithiasis. Consilium Medicum. (2012) 14:97–102.
3. Holick MF, Richtand NM, McNeill SC, Holick SA, Frommer JE, Henley JW Jr, et al. Isolation and identification of previtamin D3 from the skin of rats exposed to ultraviolet irradiation. Biochemistry. (1979) 18:1003–8. doi: 10.1021/bi00573a011
4. Holick MF, MacLaughlin JA, Clark MB, Holick SA, Potts JT Jr, Anderson RR, et al. Photosynthesis of previtamin D3 in human skin and the physiologic consequences. Science. (1980) 210:203–5. doi: 10.1126/science.6251551
5. Holick MF, MacLaughlin JA, Doppelt SH. Regulation of cutaneous previtamin D3 photosynthesis in man: skin pigment is not an essential regulator. Science. (1981) 211:590–3. doi: 10.1126/science.6256855
6. Lips P, Hosking D, Lippuner K, Norquist JM, Wehren L, Maalouf G, et al. The prevalence of vitamin D inadequacy amongst women with osteoporosis: an international epidemiological investigation. J Intern Med. (2006) 260:245–54. doi: 10.1111/j.1365-2796.2006.01685.x
7. Maltsev SV, Mansurova GSh. The metabolism of vitamin D and the ways to implement its basic functions. Pract Med. (2014) 9:12–18.
8. Prosser DE, Jones G. Enzymes involved in the activation and inactivation of vitamin D. Trends Biochem Sci. (2004). 29:664–73. doi: 10.1016/j.tibs.2004.10.005
9. Drocourt L, Ourlin JC, Pascussi JM, Maurel P, Vilarem MJ. Expression of CYP3A4, CYP2B6, and CYP2C9 is regulated by the vitamin D receptor pathway in primary human hepatocytes. J Biol Chem. (2002) 277:25125–32. doi: 10.1074/jbc.M201323200
10. Munns CF, Simm PJ, Rodda CP, Garnett SP, Zacharin MR, Ward LM, et al. Incidence of vitamin D deficiency rickets among Australian children: an Australian paediatric surveillance unit study. Med J Aust. (2012). 196:466–8. doi: 10.5694/mja11.10662
11. Zakharova IN, Tvorogova TM, Gromova OA, Evseeva EA, Lazareva SI, Maikova ID, et al. Vitamin D deficiency in adolescents: results year-round screening in Moscow. Pediatric Pharmacol. (2015) 12:528–31. doi: 10.15690/pf.v12i5.1453
12. Wang TJ, Zhang F, Richards JB, Kestenbaum B, van Meurs JB, Berry D, et al. Common genetic determinants of vitamin D insufficiency: a genome-wide association study. Lancet. (2010) 376:180–8. doi: 10.1016/S0140-6736(10)60588-0
13. McGrath JJ, Saha S, Burne TH, Eyles DW. A systematic review of the association between common single nucleotide polymorphisms and 25-hydroxyvitamin D concentrations. J Steroid Biochem Mol Biol. (2010) 121:471–7. doi: 10.1016/j.jsbmb.2010.03.073
14. Uitterlinden AG, Fang Y, van Meurs JB, Pols HA, van Leeuwen JP. Genetics and biology of vitamin D receptor polymorphisms. Gene. (2004) 338:143–56. doi: 10.1016/j.gene.2004.05.014
15. Thakkinstian A, D'Este C, Attia J. Haplotype analysis of VDR gene polymorphisms: a meta-analysis. Osteoporos Int. (2004) 15:729–34. doi: 10.1007/s00198-004-1601-x
16. Lind L, Hänni A, Lithell H, Hvarfner A, Sörensen OH, Ljunghall S. Vitamin D is related to blood pressure and other cardiovascular risk factors in middle-aged men. Am J Hypertens. (1995) 8:894–901. doi: 10.1016/0895-7061(95)00154-H
17. Biswas S, Kanwal B, Jeet C, Seminara RS. Fok-I, Bsm-I, and Taq-I variants of vitamin D receptor polymorphism in the development of autism spectrum disorder: a literature review. Cureus. (2018) 10:e3228. doi: 10.7759/cureus.3228
18. Abbassy H, Abo Elwafa R, Omar O. Bone mineral density and vitamin D receptor genetic variants in Egyptian children with beta thalassemia on vitamin D supplementation. Mediterr J Hematol Infect Dis. (2019) 11:e2019013. doi: 10.4084/mjhid.2019.013
20. Zakharova I. The national program “Vitamin D insufficiency in children and adolescents of the Russian Federation: modern approaches to correction”/Union of Pediatricians of Russia [and others]. Clin Pediatr. (2018). 96.
21. Zakharova IN, Maltsev SV, Borovik TE, Yatsyk GV, Malyavskaya SI, Vakhlova IV, et al. The results of a multicenter study “Rodnichok” on the study of vitamin D deficiency in young children in Russia. Pediatrics. (2015) 94:62–7.
22. Zakharova IN, Ya KL, Maltsev SV, Malyavskaya SI, Gromova OA, Kuryaninova VA, et al. Correction of vitamin D deficiency in young children in the Russian Federation (results of the Rodnichok-2 study). Pediatrics. (2017):73–81.
23. Benevolenskaya LI. Guide to Osteoporosis: Official Publication of the Russian Association for Osteoporosis. Moscow: BINOM, Laboratory of Knowledge (2003). 524p.
24. Eisman JA. Pharmacogenetics of the vitamin D receptor and osteoporosis. Drug Metabol Deposit. (2001) 29:505–12.
26. Cashman KD, Dowling KG, Škrabáková Z, Gonzalez-Gross M, Valtueña J, de Henauw S, Moreno L, et al. Vitamin D deficiency in Europe: pandemic? Am J Clin Nutr. (2016) 103:1033–44. doi: 10.3945/ajcn.115.120873
27. Mansbach JM, Ginde AA, Camargo CA Jr. Serum 25-hydroxyvitamin D levels among US children aged 1 to 11 years: do children need more vitamin D? Pediatrics. (2009) 124:1404–10. doi: 10.1542/peds.2008-2041
28. Maltsev SV, Shakirova EM, Safina LZ, Zakirova AM, Ya SZ. Vitamin D assessment of children adolescents. Pediatrics. (2014) 93:32–8.
29. Berestovskaya VS, Laricheva ES, Khlekhlina IuV. Out-of-season vitamin D3 deficiency in children and adolescents in Moscow. Clin Lab Diag. (2012) 12:5–7.
30. Chen TC, Chimeh F, Lu Z, Mathieu J, Person KS, Zhang A, et al. Factors that influence the cutaneous synthesis and dietary sources of vitamin D. Arch Biochem Biophys. (2007) 460:213–7. doi: 10.1016/j.abb.2006.12.017
31. Webb AR, Kline L, Holick MF. Influence of season and latitude on the cutaneous synthesis of vitamin D3: exposure to winter sunlight in Boston and Edmonton will not promote vitamin D3 synthesis in human skin. J Clin Endocrinol Metab. (1988) 67:373–8. doi: 10.1210/jcem-67-2-373
32. AlQuaiz AM, Kazi A, Fouda M, Alyousefi N. Age and gender differences in the prevalence and correlates of vitamin D deficiency. Arch Osteoporos. (2018) 13:49. doi: 10.1007/s11657-018-0461-5
33. Tagieva AN, Smetnik VP, Sukhikh GT, Yu KM, Grechenko AV, Myakotkin VA, et al. A study of the role of Vitamin D receptor (VDR), estrogen α-receptor (ESRa), and type 1 collagen α-1 chain (COLIAI) genes in the incidence of osteoporosis in postmenopausal women. Med Genetics. (2005) 4:90–5.
34. Moskalenko MV, Aseev MV, Kotova SM, Baranov BC. Analysis of the association of alleles of the COLLAL, VDR and CALCR genes with the development of osteoporosis. Environ Genetics. (2004) 2:38–43. doi: 10.17816/ecogen2138-43
35. Garnero P, Borel O, Sornay-Rendu E, Delmas PD. Vitamin D receptor gene polymorphisms do not predict bone turnover andbone mass in healthy premenopausal women. J Bone Miner Res. (1995) 10:1283–8. doi: 10.1002/jbmr.5650100902
36. Tokita A, Matsumoto H, Morrison NA, Tawa T, Miura Y, Fukamauchi K, et al. Vitamin D receptor alleles, bone mineral density and turnover inpremenopausal Japanese women. J Bone Miner Res. (1996) 11:1003–9. doi: 10.1002/jbmr.5650110718
37. Jolliffe DA, Walton RT, Griffiths CJ, Martineau AR. Single nucleotide polymorphisms in the vitamin D pathway associating with circulating concentrations of vitamin D metabolites and non-skeletal health outcomes: review of genetic association studies. J Steroid Biochem Mol Biol. (2016) 164:18–29. doi: 10.1016/j.jsbmb.2015.12.007
38. Kozlov AI, Vershubskaya GG, Negasheva MA. Vitamin D receptor gene (VDR) polymorphism in samples of the population of European Russia and the Urals. Perm Med J. (2016) 33:60–6.
39. Baroncelli GI, Federico G, Bertelloni S, Ceccarelli C, Cupelli D, Saggese G. Vitamin-D receptor genotype does not predict bone mineral density, bone turnover, and growth in prepubertal children. Horm Res. (1999) 51:150–6. doi: 10.1159/000023348
40. Ali R, Fawzy I, Mohsen I, Settin A. Evaluation of vitamin D receptor gene polymorphisms (FokI and BsmI) in T1DM Saudi children. J Clin Lab Anal. (2018) 32:e22397. doi: 10.1002/jcla.22397
41. Hibler EA, Jurutka PW, Egan JB, Hu C, LeRoy EC, Martinez ME, et al. Association between polymorphic variation in VDR and RXRA and circulating levels of vitamin D metabolites. J Steroid Biochem Mol Biol. (2010) 121:438–41. doi: 10.1016/j.jsbmb.2010.03.052
42. Petersen RA, Larsen LH, Damsgaard CT. Common genetic variants are associated with lower serum 25-hydroxyvitamin D concentrations across the year among children at northern latitudes. Br J Nutr. (2017) 117:829–38. doi: 10.1017/S0007114517000538
43. Jolliffe DA, Hanifa Y, Witt KD, Venton TR, Rowe M, Timms PM, et al. Environmental and genetic determinants of vitamin D status among older adults in London UK. J Steroid Biochem Mol Biol. (2016) 164:30–5. doi: 10.1016/j.jsbmb.2016.01.005
Keywords: vitamin D deficiency, polymorfism, age features, insolation, VDR
Citation: Kondratyeva EI, Zakharova IN, Ilenkova NA, Klimov LY, Petrova NV, Zodbinova AE, Zhekaite EK, Chikunov VV, Dolbnya SV, Voronkova AY, Sherman VD, Loshkova EV, Melyanovskaya YL, Budzinskiy RM, Kuryaninova VA and Kutsev SI (2020) Vitamin D Status in Russian Children and Adolescents: Contribution of Genetic and Exogenous Factors. Front. Pediatr. 8:583206. doi: 10.3389/fped.2020.583206
Received: 14 July 2020; Accepted: 26 October 2020;
Published: 19 November 2020.
Edited by:
Mauro Fisberg, Federal University of São Paulo, BrazilReviewed by:
Bianca Bianco, Faculdade de Medicina do ABC, BrazilDaniela Melis, University of Salerno, Italy
Sérgio Cunha Velho Sousa, Centro Hospitalar e Universitário de Coimbra, Portugal
Copyright © 2020 Kondratyeva, Zakharova, Ilenkova, Klimov, Petrova, Zodbinova, Zhekaite, Chikunov, Dolbnya, Voronkova, Sherman, Loshkova, Melyanovskaya, Budzinskiy, Kuryaninova and Kutsev. This is an open-access article distributed under the terms of the Creative Commons Attribution License (CC BY). The use, distribution or reproduction in other forums is permitted, provided the original author(s) and the copyright owner(s) are credited and that the original publication in this journal is cited, in accordance with accepted academic practice. No use, distribution or reproduction is permitted which does not comply with these terms.
*Correspondence: Elena K. Zhekaite, elena_zhekayte@mail.ru
†ORCID: Elena I. Kondratyeva orcid.org/0000-0001-6395-0407
Irina N. Zakharova orcid.org/0000-0003-4200-4598
Natalya A. Ilenkova orcid.org/0000-0001-8058-7806
Leonid Ya. Klimov orcid.org/0000-0001-7248-1614
Aisa E. Zodbinova orcid.org/0000-0003-4745-0618
Elena K. Zhekaite orcid.org/0000-0001-5013-3360
Vladimir V. Chikunov orcid.org/0000-0001-6011-2360
Svetlana V. Dolbnya orcid.org/0000-0002-2056-153X
Victoria D. Sherman orcid.org/0000-0003-2206-1528
Elena V. Loshkova orcid.org/0000-0002-3043-8674
Yuliya L. Melyanovskaya orcid.org/0000-0002-8814-5532
Roman M. Budzinskiy orcid.org/0000-0002-0131-913
Victoria A. Kuryaninova orcid.org/0000-0002-0731-7153
Sergey I. Kutsev orcid.org/0000-0002-3133-8018