- 1Division of Neonatology, Department of Pediatrics, Dokuz Eylul University Faculty of Medicine, Izmir, Turkey
- 2Division of Neonatology, Department of Pediatrics, Tinaztepe University Faculty of Medicine, Izmir, Turkey
High frequency oscillatory ventilation with volume-guarantee (HFOV-VG) is a promising lung protective ventilator mode for the treatment of respiratory failure in newborns. However, indicators of optimal ventilation during HFOV-VG mode are not identified yet. In this study, we aimed to evaluate optimal high-frequency tidal volume (VThf) and the dissociation coefficient of CO2 (DCO2) levels to achieve normocapnia during HFOV-VG after lung recruitment in very low birthweight infants with respiratory distress syndrome (RDS). Preterm babies under the 32nd postmenstrual week with severe RDS that received HFOV-VG using open-lung strategy between January 2014 and January 2019 were retrospectively evaluated. All included patients were treated with the Dräger Babylog VN500 ventilator in the HFOV-VG mode. In total, 53 infants with a mean gestational age of 26.8 ± 2.3 weeks were evaluated. HFOV mean optimal airway pressure (MAPhf) level after lung recruitment was found to be 10.2 ± 1.7 mbar. Overall, the mean applied VThf per kg was 1.64 ± 0.25 mL/kg in the study sample. To provide normocapnia, the mean VThf was 1.61 ± 0.25 mL/kg and the mean DCO2corr was 29.84 ± 7.88 [mL/kg]2/s. No significant correlation was found between pCO2 levels with VThf (per kg) or DCO2corr levels. VThf levels to maintain normocarbia were significantly lower with 12 Hz frequency compared to 10 Hz frequency (1.50 ± 0.24 vs. 1.65 ± 0.25 mL/ kg, p < 0.001, respectively). A weak but significant positive correlation was found between mean airway pressure (MAPhf) and VThf levels. To our knowledge, this is the largest study to evaluate the optimal HFOV-VG settings in premature infants with RDS, using the open-lung strategy. According to the results, a specific set of numbers could not be recommended to achieve normocarbia. Following the trend of each patient and small adjustments according to the closely monitored pCO2 levels seems logical.
Introduction
Despite the rising trend of non-invasive ventilation techniques, up to 50% of extremely preterm infants having respiratory distress syndrome (RDS) need to be intubated and mechanically ventilated. Prevention of repeated opening and closing of the alveoli, avoiding fluctuant and excessive tidal volumes are the fundamentals of the lung protective mechanical ventilation (1). Volume-targeted ventilation (VTV) strategies are increasingly used in the care of neonates and offer many advantages by avoiding disproportionate tidal volumes (2, 3). High-frequency oscillatory ventilation (HFOV) has been used over 30 years in newborn babies with severe respiratory failure (4, 5). HFOV with volume guarantee (HFOV-VG) is a promising new ventilatory mode for the treatment of respiratory failure in newborns. Theoretically, HFOV-VG is expected to result in less lung injury since it reduces fluctuations of high frequency tidal volume (VThf), reduces the number of out-of-target pCO2 values and provides fewer hypoxia attacks compared with HFOV (6, 7).
During HFOV-VG, the clinician can set a target VThf, and the ventilator will automatically adjust the amplitude pressure to supply the targeted VThf. Tight control of VThf and automatic adjustments in amplitude using HFOV-VG may be particularly useful when the respiratory mechanics change rapidly (6, 8). Previous studies demonstrated that during HFOV-VG, the VThf can vary from 1 s to another, but it is kept very close to the target VThf in the long term (9).
CO2 excretion during HFOV is defined by the diffusion coefficient of CO2 (DCO2) as an indicator of alveolar ventilation. Since DCO2 (ml2/s) is formulated by “DCO2=f x VThf2”, even small changes in VThf affect DCO2 more than changes in frequency (10–12). DCO2 has been considered an important parameter in the follow-up of CO2 elimination, however, its value providing normocapnia varies from patient to patient. Recently, weight-corrected DCO2 ([mL/kg]2/s) has been proposed to reduce inter-individual variability (7).
To ensure optimal benefit from the HFOV, the alveoli should be opened and kept open using optimal continuous distension pressures (13, 14). Though lung volume recruitment and appropriate tidal volume settings are considered important strategies for the success of HFOV-VG, optimal VThf parameters are not identified yet for preterm infants during the acute phase of RDS. This study's objective is to evaluate optimal VThf and DCO2 levels to achieve normocapnia during HFOV-VG using open-lung strategy in very premature infants with RDS.
Materials and Methods
Study Design, Patients, and Interventions
The retrospective observational study was carried out at the third level neonatal intensive care unit (NICU) at Dokuz Eylul University Hospital, between January 2014 and January 2019. The Ethical Committee of Dokuz Eylul University Faculty of Medicine approved the study protocol. Infants suffering from RDS between 23 and 32 weeks of gestation, who started HFOV-VG with lung recruitment maneuver within 72 h of life, were eligible for the study. Infants who had (i) congenital anomalies affecting the cardiopulmonary system or (ii) endotracheal tube leaks of over 40% or (iii) air leak syndromes or (iv) pulmonary hypertension were excluded.
All interventions were performed according to the unit's ventilation protocol by considering the infants' characteristics. Surfactant treatment was given according to the European Consensus Guidelines of that period. If surfactant was considered, 200 mg/kg (poractant alpha) was given to the first dose and repeated doses were given as 100 mg/kg (15, 16). The endotracheal tube diameter was selected according to the current NRP guidelines and the maximum tolerable tube leakage was 40% according to the operating principles of the ventilator and our unit's ventilation protocol (17). Suction was avoided unless clinically indicated. If suction was needed a closed system is preferred. All of these patients received fentanyl analgesia; strong sedatives and muscle relaxants were not used.
Ventilation Strategies
We have been using HFOV-VG as elective or early-rescue ventilation mode for infants who were failing conventional ventilation or would benefit from HFOV according to the opinion of the attending clinician. In general, HFOV-VG ventilation has been started with the following reasons: higher VT need in conventional VG ventilation (> 6 ml/ kg), high peak positive pressure requirement (over 20 mbar), diffuse lung atelectasis requiring lung recruitment, or high FiO2 need (40%) despite proper PEEP and surfactant therapy.
All patients were treated with a ventilator (Dräger Babylog VN500) in HFOV-VG mode. An optimal volume strategy was applied in all infants. Depending on HFOV-VG starting time, MAPhf level was initiated with 8 or 2 mbar above the MAPhf in conventional mechanical ventilation. The MAPhf was increased with steps of 1 mbar every 2–3 min until a critical opening pressure, where oxygenation no longer improved, or the fraction of inspired oxygen (FiO2) was ≤ 0.30, to give an arterial oxygen saturation of 90–94%. Next, the MAPhf level was decreased by 1–2 mbar stepwise every 2–3 min to find the closing distending pressure. Finally, the lung was reopened again with the previously defined critical opening pressure and a MAPhf level 2 mbar above closing pressure was set, corresponding to optimal continuous distending pressure (18). The lung recruitment maneuver was performed under VG mode to allow amplitude fluctuations to obtain stable VThf levels. Recruitment was stopped in case of bradycardia (heart rate <100) or hypotension.
All of the infants received a frequency between 10 and 12 Hz and an oscillatory inspiratory/expiratory ratio of 1:1. The amplitude limit (Ampl max) was set at 10–15% above the average amplitude required to reach the target VThf. Subsequently, the set VThf was adjusted by the clinical team up or down in increments of 0.1–0.2 mL/kg if the pCO2 value was outside the target range. Capillary blood gases were assessed 30 min after the initiation of HFOV-VG and repeated at intervals of 4–6 h or more often as needed using a blood gas analyzer (ABLTM 700 Radiometer, Copenhagen, Denmark). The consecutive blood gases that belong to the period in which HFOV was applied continuously without interruption were selected for the study. The normocapnia was defined as pCO2 ranging from 40 to 55 mm Hg. Fractional inspired oxygen concentration (FiO2) was adjusted to obtain a SaO2 between 90 and 94% by a pulse oximeter.
Data Acquisition and Analysis
The flow of gases in the airway and tidal volume was measured continuously using a hot wire anemometer positioned in the airway entrance while undertaking HFOV+VG. Critical parameters such as MAPhf (Paw), ΔPhf (swinging pressure around the mean Paw), VThf (ml), and DCO2 (mL2/s) were followed using VentView 2.n software (Draeger, Lubeck, Germany). The mean values of these parameters were assessed over 10 min before blood gas analysis was recorded. All calculations and correlations regarding VThf were performed according to the tidal volume normalized to body weight and given as mL/kg. Daily respiratory mechanics and blood gas analysis results and follow-up data were collected from the electronic and published patients' files.
Statistics
The normality of data was determined by the Shapiro-Wilk test. According to the distribution pattern, continuous data were presented as mean ± standard deviation (SD) or median (25–75 percentile). The statistical significance of mean differences between two independent groups was tested using the independent t-test. To test the significance of the difference between the means or medians of parameters in three or more independent groups, one-way ANOVA or Kruskal Wallis tests were performed, respectively. The relationship between categorical variables was tested with chi-square analysis. Pearson's correlation analysis was performed to determine the correlations between selected parameters. The effect of multiple independent variables on pCO2 and optimal VThf levels was tested using the general linear model.
Post-hoc power analysis for differences of means was performed using an online statistical tool, “OpenEpi” (19). SPSS software was used for all statistical analyses (IBM SPSS Statistics for Windows, Version 24.0. Armonk, NY). Statistical significance was set at p < 0.05.
Results
In total, 53 babies were included in the study between January 2014 and January 2019. Mean gestational age and birth weight of the infants were 26.3 ± 2.3 weeks and 882 ± 286 grams, respectively.
All of the infants received surfactant therapy in the delivery room or in the NICU according to the intubation time. Poractant alfa was instilled at 200 mg/kg for the first dose and then repeated at 100 mg/kg if needed according to RDS guidelines (15, 16). Delivery room intubation was performed for 38 infants. Median intubation time for the remaining patients was 10 h (min-max: 1–53 h). In 14 of these patients, a 12 Hz frequency was used according to the decision of the consultant neonatologist. The patient characteristics are shown in Table 1.
Overall, the mean applied VThf per kg was 1.64 ± 0.25 mL/kg in the study sample. Of the 274 blood gases evaluated, 178 (65.2%) were in the normocarbic range, while the remaining were within the hypocarbic (53 blood gases, 19.4%) or hypercarbic (42 blood gases, 15.4%) range. Only nine patients needed VThf ≥2 mL/kg to overcome hypercarbia during follow-up, and none of these babies required VThf > 2.4 mL/kg and DCO2corr >50 [mL/kg]2/s to control hypercapnia. Four patients needed VThf settings smaller than 1.25 mL/kg for maintaining normocarbia. The patients that need very low VThf volumes were extremely preterm infants born at gestational age under 25 weeks.
The mean VThf level yielding normocapnic blood gases was 1.61 ± 0.25 mL/kg. Mean VThf levels corresponding to these three pCO2 categories did not significantly differ between one or the other (Table 2). No significant correlation was found between VThf values and the corresponding pCO2 values during the follow-up period (Figure 1A). When the ventilatory settings corresponding to normocapnic blood gases were examined, there was a very weak but significant positive correlation between VThf and MAP values (0.319, p < 0.001) (Figure 1B).
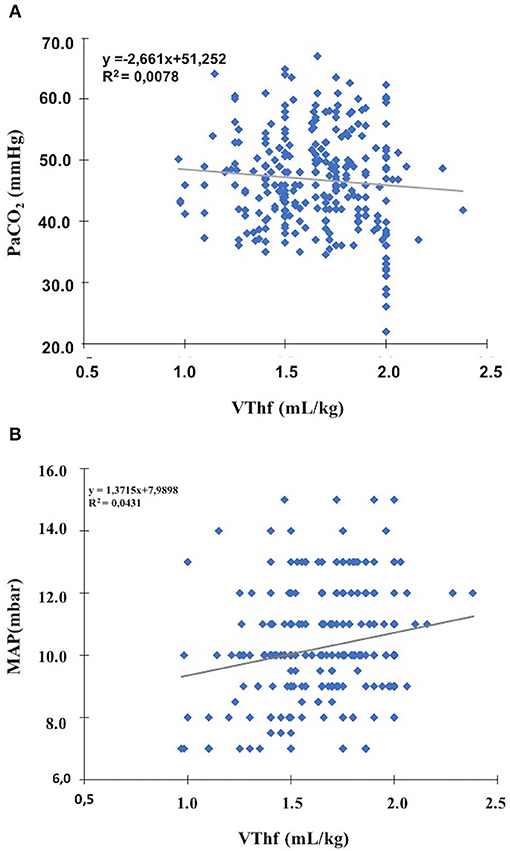
Figure 1. Pearson correlation analysis between VThf, pCO2, and MAPhf levels (A) no significant correlation between all VThf and pCO2 levels (Pearson coefficient = 0.01, p = 0.899) (B) a very weak, but significant correlation between optimal VThf and MAPhf levels (Pearson coefficient = 317, p < 0.001).
Subgroup analysis according to the frequency demonstrated that VThf levels to achieve normocarbia were significantly lower with 12 Hz frequency compared to 10 Hz frequency (1. 50 ± 0.24 vs. 1.65 ± 0.25 ml/ kg, p < 0.001) (Table 2).
Overall, the mean DCO2 level to obtain normocarbia was 25.67 ± 16.55 ml2/s and weight-corrected mean DCO2corr level providing normocarbia was calculated as 29.84 ± 7.88 [mL/kg]2/s (Table 2). There was no significant difference in DCO2corr levels between normocapnic, hypercapnic, or hypocapnic blood gases levels (p = 0,415) (Table 2). A significant correlation could not have been found between DCO2corr levels and pCO2 values.
To test the effect of multiple factors on optimal VThf values (ml/kg), birth weight, gestational age, frequency, and MAPhf levels were tested in a model using a general linear model. The results demonstrated that frequency and MAPhf levels were the main effectors of optimal VThf value (p = 0.02 and p = 0.003, respectively). Main ventilatory parameters were given as Supplementary Data (Table S1).
Discussion
To our knowledge, this is the largest case series to evaluate HFOV-VG settings in premature infants with RDS. Results demonstrated that neither optimal VThf (mL/ kg) nor DCO2corr levels ([mL/kg]2/s) correlated with pCO2 levels. It was also confirmed that higher frequencies need lower delivered tidal volumes for adequate ventilation during HFOV-VG.
HFOV- VG mode makes it possible to maintain DCO2 and normocapnia while lowering VThf and increasing the frequency in an attempt to minimize lung injury (20, 21). In the present study, mean VThf levels corresponding normocapnic blood gases were around 1.50 cc mL /kg for 12 Hz, and 1.65 mL/ kg for 10 Hz. González-Pacheco et al. demonstrated that adequate VThf was 1.46 mL/kg for ELBW infants and 1.57 mL/kg for infants weighing 1,000–2,000 g using higher frequencies up to 17 Hz (21). In our study a VThf level over 2.4 mL/kg was not required. Consistent with these results, Belteki et al. demonstrated that VThf or DCO2 have poor correlation with CO2 levels but a volume of >2.5 mL/kg VThf is rarely needed (7). Parallel to our results, Zimova-Herknerova et al. showed that the median delivered normocapnic VThf during HFOV was 1.67 mL/kg in a heterogeneous group of newborns ventilated by HFOV at any time during their hospital stay (12). Few studies support higher VThf requirement during HFOV varying between 1.75–1.90 mL/kg using a constant frequency of 10 Hz (6, 22). Besides the frequency, gestational age, HFOV starting time, practicing open lung strategies, and severity of lung disease are possible determiners of VThf need. Our population was composed of a near- homogeneous group of patients suffering from RDS. We started HFOV as an early-rescue mode rather than rescue HFOV and median starting time (8 h) is indicating the initial phase of RDS rather than the other problems, such as hemodynamically significant PDA, pulmonary edema, VILI, chronic lung disease etc.
Although VThf is considered a key element for minute ventilation in HFOV, our study did not demonstrate a correlation between VThf and pCO2 levels. Even the same patients had out-of-target pCO2 levels with the same VThf levels. As a possible reason, allowing spontaneous breathing without the use of heavy sedatives or muscle relaxants may have affected the gas exchange in these infants in different ways. The narrow range of VThf values applied in this study may explain the similarity of mean VThf values corresponding to normocapnic and hypo or hypercarbic blood gases. If we used higher VT values above 2 ml/kg, we might have seen the expected relationship between hypocarbia and high VT. Furthermore, we considered a relative narrow pCO2 range between 40 and 55 mmHg as normocarbia. Despite this, 65% of the blood gases were normocarbic. If a larger range (5–8 kpa = 37.5–60 mmHg) had been considered as in the other studies (9) the rate of normocarbic blood gases would have been increased to 87.6%, a successful rate considering the high incidence of hypocapnia during HFOV.
Currently, a VThf requirement according to the patient's characteristics such as birth weight and disease severity is not as well-known as in conventional VG ventilation. However, a weak but significant correlation between MAPhf and optimal VThf levels in this study may indicate the need for higher VThf levels in infants with more severe lung disease or inadequate lung recruitment. Patients who responded well to recruitment probably needed lower MAPhf and VThf levels due to decreased physiologic dead space and shunt, similar to ARDS patients (23). However, there is no strong evidence to support this assumption.
In our study, the DCO2 level was highly variable according to the birth weight of the infants. Then we considered the calculation of the DCO2corr levels as previously recommended (7). Although DCO2 is considered the best predictor of CO2 elimination during HFOV, our study could not demonstrate a significant correlation between optimal VThf and DCO2 levels. Targeting a weight-corrected DCO2corr achieved more static levels; however, this adjustment does not change the results because there was not a significant correlation between DCO2corr and VThf levels.
This study has several limitations. The most important limitation was its retrospective design. This patient group was selected since it was a relatively homogenous population composed of very preterm infants and started HFOV-VG because of severe RDS in the first 72 h of life. Although the selected study population is not heterogeneous, these results may not be generalized for all premature infants with RDS. Although the retrospective design and longer study period may be questionable, over the 5 years, our unit's RDS and HFOV-VG protocols have not been modified too much. The lack of continuous CO2 monitoring during the infants' course is another important limitation. This restriction may lead to an incomplete representation of the real-time relationship between evaluated parameters. Ideally, instant monitoring of pCO2 and ventilation parameters prospectively in a homogeneous patient group will allow a clear understanding of the relationship between them.
In summary, results could not allow making any recommendation on an optimal starting value for VThf. Optimal levels are dynamic and vary according to the instantaneous features of individuals. Therefore, it is unrealistic to recommend a general VThf value to all patients. The needs of each patient should be monitored within itself and the settings should be titrated according to close pCO2 monitoring.
Data Availability Statement
All datasets generated for this study are included in the article/Supplementary Material.
Ethics Statement
The studies involving human participants were reviewed and approved by Dokuz Eylul University Clinical Researches Ethical Commity. Written informed consent from the participants' legal guardian/next of kin was not required to participate in this study in accordance with the national legislation and the institutional requirements.
Author Contributions
FT, BD, and HO contributed to the conception and design of the study. ND, BI, MC, and BD organized the database. FT performed the statistical analysis and wrote the manuscript. BI wrote the first draft of the manuscript. All authors contributed to manuscript revision, read and approved the submitted version.
Conflict of Interest
The authors declare that the research was conducted in the absence of any commercial or financial relationships that could be construed as a potential conflict of interest.
Supplementary Material
The Supplementary Material for this article can be found online at: https://www.frontiersin.org/articles/10.3389/fped.2020.00105/full#supplementary-material
Table S1. Main ventilatory parameters.
References
1. Van Kaam A. Lung-protective ventilation in neonatology. Neonatology. (2011) 99:338–41. doi: 10.1159/000326843
2. Keszler M. Volume-targeted ventilation: one size does not fit all. Evidence-based recommendations for successful use. Arch Dis Child Fetal Neonatal Ed. (2019) 104:F108–12. doi: 10.1136/archdischild-2017-314734
3. Klingenberg C, Wheeler KI, McCallion N, Morley CJ, Davis PG. Volume-targeted versus pressure-limited ventilation in neonates. Cochrane Database Syst Rev. (2017) 10:CD003666. doi: 10.1002/14651858.CD003666.pub4
4. Clark RH, Null DM, Gerstmann DR, deLemos RA. Prospective randomized comparison of high-frequency oscillatory and conventional ventilation in respiratory distress syndrome. Pediatrics. (1992) 89:5–12.
5. Slutsky AS, Drazen JM. Ventilation with small tidal volumes. N Engl J Med. (2002) 347:630–1. doi: 10.1056/NEJMp020082
6. Iscan B, Duman N, Tuzun F, Kumral A, Ozkan H. Impact of volume guarantee on high-frequency oscillatory ventilation in preterm infants: a randomized crossover clinical trial. Neonatology. (2015) 108:277–82. doi: 10.1159/000437204
7. Belteki G, Lin B, Morley CJ. Weight-correction of carbon dioxide diffusion coefficient (DCO2) reduces its inter-individual variability and improves its correlation with blood carbon dioxide levels in neonates receiving high-frequency oscillatory ventilation. Pediatr Pulmonol. (2017) 52:1316–22. doi: 10.1002/ppul.23759
8. Sánchez-Luna M, González-Pacheco N, Belik J, Santos M, Tendillo F. New ventilator strategies: high-frequency oscillatory ventilation combined with volume guarantee. Am. J. Perinatol. (2018) 35:545–8. doi: 10.1055/s-0038-1637763
9. Belteki G, Morley CJ. High-frequency oscillatory ventilation with volume guarantee: a single-centre experience. Arch Dis Childhood-Fetal Neonatal. (2019) 104:F384–9. doi: 10.1136/archdischild-2018-315490
10. Chan V, Greenough A, Milner AD. (1993). The effect of frequency and mean airway pressure on volume delivery during high-frequency oscillation. Pediatr Pulmonol. 15:183–6. doi: 10.1002/ppul.1950150310
11. Singh R, Courtney SE, Weisner MD, Habib RH. Respiratory mechanics during high-frequency oscillatory ventilation: a physical model and preterm infant study. J Appl Physiol. (2011) 112:1105–13. doi: 10.1152/japplphysiol.01120.2011
12. Zimová-Herknerová M, Plavka R. Expired tidal volumes measured by hot-wire anemometer during high-frequency oscillation in preterm infants. Pediatr Pulmonol. (2006) 41:428–33. doi: 10.1002/ppul.20367
13. Mcculloch PR, Forkert PG, Froese AB. Lung volume maintenance prevents lung injury during high frequency oscillatory ventilation in surfactant-deficient rabbits. Am Rev Respir Dis. (1988) 137:1185–92. doi: 10.1164/ajrccm/137.5.1185
14. Tingay DG, Mills JF, Morley CJ, Pellicano A, Dargaville PA. Indicators of optimal lung volume during high-frequency oscillatory ventilation in infants. Crit Care Med. (2013) 41:237–44. doi: 10.1097/CCM.0b013e31826a427a
15. Sweet DG, Carnielli V, Greisen G, Hallman M, Ozek E, Plavka R, et al. European consensus guidelines on the management of respiratory distress syndrome−2013 update. Neonatology. (2013) 103:353–68. doi: 10.1159/000349928
16. Sweet DG, Carnielli V, Greisen G, Hallman M, Ozek E, Plavka R, et al. European consensus guidelines on the management of respiratory distress syndrome−2016 update. Neonatology. (2016) 111:107–25. doi: 10.1159/000448985
17. Wyckoff MH, Aziz K, Escobedo MB, Kapadia VS, Kattwinkel J, Perlman JM, et al. Part 13: neonatal resuscitation: 2015 American Heart Association guidelines update for cardiopulmonary resuscitation and emergency cardiovascular care. Circulation. (2015) 132(18_suppl_2):S543–60. doi: 10.1161/CIR.0000000000000267
18. De Jaegere A, van Veenendaal MB, Michiels A, van Kaam AH. Lung recruitment using oxygenation during open lung high-frequency ventilation in preterm infants. Am J Respir Crit Care Med. (2006) 174:639–45. doi: 10.1164/rccm.200603-351OC
19. Dean AG, Sullivan KM, Soe MM. OpenEpi: Open Source Epidemiologic Statistics for Public Health, Version. (2019). Available online at: www.OpenEpi.com (accessed April 04, 2019).
20. Mukerji A, Belik J, Sanchez-Luna M. Bringing back the old: time to reevaluate the high-frequency ventilation strategy. J Perinatol. (2014) 34:464. doi: 10.1038/jp.2014.39
21. González-Pacheco N, Sánchez-Luna M, Ramos-Navarro C, Navarro-Patiño N, de la Blanca AR. Using very high frequencies with very low lung volumes during high- frequency oscillatory ventilation to protect the immature lung. A pilot study. J Perinatol. (2016) 36:306. doi: 10.1038/jp.2015.197
22. Lee SM, Namgung R, Eun HS, Lee SM, Park MS, Park KI. Effective tidal volume for normocapnia in very-low-birth-weight infants using high frequency oscillatory ventilation. Yonsei Med J. (2018) 59:101–6. doi: 10.3349/ymj.2018.59.1.101
Keywords: HFOV, volume guarantee, lung recruitment, VThf, DCO2, frequency, RDS, lung-protective strategies
Citation: Tuzun F, Deliloglu B, Cengiz MM, Iscan B, Duman N and Ozkan H (2020) Volume Guarantee High-Frequency Oscillatory Ventilation in Preterm Infants With RDS: Tidal Volume and DCO2 Levels for Optimal Ventilation Using Open-Lung Strategies. Front. Pediatr. 8:105. doi: 10.3389/fped.2020.00105
Received: 06 November 2019; Accepted: 28 February 2020;
Published: 24 March 2020.
Edited by:
Hans Fuchs, University of Freiburg, GermanyReviewed by:
Ulrich Herbert Thome, Leipzig University, GermanyFilip Cools, Vrije University Brussel, Belgium
Copyright © 2020 Tuzun, Deliloglu, Cengiz, Iscan, Duman and Ozkan. This is an open-access article distributed under the terms of the Creative Commons Attribution License (CC BY). The use, distribution or reproduction in other forums is permitted, provided the original author(s) and the copyright owner(s) are credited and that the original publication in this journal is cited, in accordance with accepted academic practice. No use, distribution or reproduction is permitted which does not comply with these terms.
*Correspondence: Funda Tuzun, ZnVuZGF0dXp1bkBnbWFpbC5jb20=