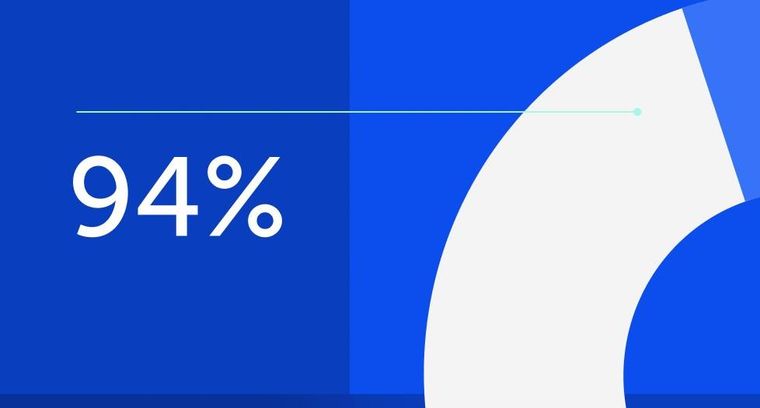
94% of researchers rate our articles as excellent or good
Learn more about the work of our research integrity team to safeguard the quality of each article we publish.
Find out more
MINI REVIEW article
Front. Pediatr., 04 October 2018
Sec. Pediatric Gastroenterology, Hepatology and Nutrition
Volume 6 - 2018 | https://doi.org/10.3389/fped.2018.00271
This article is part of the Research TopicPediatric Obesity: A Focus on Treatment OptionsView all 13 articles
Introduction: Childhood obesity is occurring at alarming rates in both developed and developing countries. “Obesogenic” environmental factors must be associated with variants of different risk alleles to determine polygenic or common obesity, and their impact depends on different developmental stages.The interaction between obesogenic environment and genetic susceptibility results in the so-called polygenic forms of obesity. In contrast, monogenic and syndromic obesity are not influenced by environmental events. Therefore, this review aimed to underline the roles of some of the most studied genes in the development of monogenic and polygenic obesity in children.
Results: Among the most common obesity related genes, we chose the fat mass and obesity-associated (FTO) gene, leptin gene and its receptor, tumor necrosis factor alpha (TNF-α), the melanocortin 4 receptor gene (MC4R), Ectoenzyme nucleotide pyrophosphate phosphodiesterase 1 (ENPP1), and others, such as peroxisome proliferator-activated receptor gamma (PPARG), angiotensin-converting enzyme (ACE), glutathione S-transferase (GST), and interleukin-6 (IL-6) genes. The roles of these genes are complex and interdependent, being linked to different cornerstones in obesity development, such as appetite behavior, control of food intake and energy balance, insulin signaling, lipid and glucose metabolism, metabolic disorders, adipocyte differentiation, and so on.
Conclusions: Genetic predisposition is mandatory, but not enough to trigger obesity.Dietary interventions and proper lifestyle changes can prevent obesity development in genetically predisposed children. Further studies are needed to identify the precise role of both genetic and obesogenic factors in the development of childhood obesity in order to design effective preventive methods.
Childhood obesity has become recently a global epidemic due to its high rates in both developed and developing countries. In Romania, a recent study found that one in four children is overweight or obese, and identified male gender, prepuberal age, and urban environment as risk factors for overweight. This major public health problem is a result of the interaction between environmental factors and individual genetic susceptibility. “Obesogenic” environmental factors must be associated with variants of different risk alleles to determine polygenic or common obesity, and their impact depends on different developmental stages.
Recently, childhood obesity has become a global epidemic, occurring at high rates in both developed and developing countries (1). Most studies regarding this health burden were performed on adult populations, and only a few of them included children. In Romania, a recent study found that one in four children is overweight or obese and identified male sex, prepubertal age, and urban environment as risk factors for incidences of overweight (2). This major public health problem is a result of the interaction between environmental factors and individual genetic susceptibility. Heritability plays a key role in the development of childhood obesity, with a rate of determinism as high as 70%, and it was proven to be higher in children than adults (3, 4). Obesogenic environment is only a trigger, and not the leading-cause for excessive weight gain, a genetic susceptibility to fat gain being mandatory for an individual to become obese (5–7). Therefore, environmental factors just favor the phenotypic expression in individuals programmed to become obese (8). Moreover, the impact of obesogenic environment differs during different developmental stages. For example, Silvenstoinen et al. performed a systematic review on twins and adopted children and proved that environmental factors moderately influence BMI variation only up to the age of 13 years, their effect disappearing beyond this age (6). It is true BMI curve in children is therefore genetically programmed, but at the same time environmental circumstances are able to modify this curve (8). Another well-documented fact is that fetal and early postnatal environmental events, such as maternal nutritional status during pregnancy, maternal smoking, gestational diabetes, increased birth weight, rapid weight gain, or feeding practices, may also influence the development of obesity later in life (8–16). In addition, the expression of genetic risks predominates during infancy and early childhood leading to an earlier adiposity rebound and higher BMI in children that carry a genetic susceptibility (17–19).
Therefore, it is apparent that “obesogenic” environmental factors alone are not responsible for the development of obesity; they must be associated with variants of different risk alleles to determine polygenic or common obesity (20). Many studies focused on assessing the role of different genes in the determination of obesity and found that certain variants are associated with weight regulation and adipose tissue accumulation (21). Therefore, recent genome-wide association studies identified over 50 genetic loci that can be associated with obesity (22), resulting in polygenic forms of obesity. Polygenic' variants are defined as any of a group of alleles at different gene loci that express a combined effect on controlling the inheritance of a quantitative phenotype or which can modify the expression of a qualitative character. Therefore, certain traits can be the result of the simultaneous presence of DNA changes in multiple genes. In case of quantitative traits, it was proved that each allele owns a small effect and these effects can be additive or non-additive. It is generally assumed that many of these polygenic variants are involved in body weight regulation and in individuals who harbor multiple of these variants, obesity can occur. Thus, the hypothesis of polygenic obesity implies that every obese individual carries his own specific set of polygenic variants which are unlikely to be same in another subject with obesity (21).
On the other hand, there are also rare forms of obesity that lack the influence of environmental factors, the so-called recessive monogenic obesity; examples include mutations affecting the leptin gene or its receptor, proopiomelanocortin and pro-hormone convertase subtilisin/kesin type, all leading to a fast and dramatic weight gain (8). These forms present complete penetrance in comparison to the particular form of obesity related to MC4R, and probably MC3R as well; polymorphisms in these genes are associated with forms of obesity that are more severe than typical polygenic obesity but less severe than homozygous gene mutations (8). In contrast to these types of monogenic obesity, the syndromic ones usually occur after infancy, such as Prader-Willi syndrome, Bardet-Biedl syndrome, Albright's hereditary osteodystrophy, Alström syndrome, and WAGR (Wilms' tumor, aniridia, genitourinary anomalies, and retardation) syndrome. Apart from obesity, these syndromes involve dysmorphic features, cognitive impairment, and malformations of major organs (23).
This review summarizes information about the roles of genetic mutations and polymorphisms in the pathogenesis of polygenic obesity in childhood.
The FTO gene is located on chromosome 16, position 16q12.2, and comprises 9 exons. This gene is expressed in the hypothalamus, at the level of the arcuate nucleus, which is responsible for appetite behavior and fatty acid metabolism, among others (24, 25). Therefore, a recent cross-sectional study was performed on 406 Brazilian children and adolescents aged 7–17 years, among whom 34.5% were overweight or obese. The study proved a positive association between AA genotype of the rs9939609 FTO gene polymorphism and the risk of overweight/obesity (26). Similarly, Cecil et al. stated that the A allele is related to increased fat mass and body mass index (BMI) in Scottish children aged 4–10 years (27). In addition, a study performed on 289 subjects aged 6–19 years proved that the carriers of the AT or AA genotype more frequently expressed a tendency toward intake of food with higher fat content and a loss of food intake control (28). Similarly, a Chinese study performed on children and adolescents found that the AA genotype carriers expressed a preference for a meat-based diet in comparison with those carrying the TT genotype, who preferred a plant-based diet (29). Contradictory results were reported by de Araújo Pereira et al. who did not find any association between FTO gene polymorphisms and overweight or obesity risk in a study performed on 195 obese/overweight individuals with a mean age of 11 years (30). Another study that included 478 African-American children also failed in identifying any association between different FTO gene variants and BMI (31). Therefore, ethnicity might be an important variable regarding the role of FTO gene polymorphism in obesity development. A recent study performed on Polish young adults, which assessed multiple polymorphisms of the FTO gene (rs1121980, rs1421085, rs9930506, and rs9939609), found that this population expressed two disparate haplotypes of the FTO gene variants: TCGA risk haplotype and CTAT protective haplotype, the alleles provided in the previously mentioned order (20). Nevertheless, the genetic susceptibility for obesity can be influenced by dietary interventions in combination with exercise, as stated by Zou et al. in a study performed on obese children carrying the at-risk FTO rs9939609 genotype (32). The rs9939609 FTO gene polymorphism was associated with certain obesity complications, such as high blood pressure, percentage body fat and fat mass, plasma insulin levels, and insulin resistance (33). In contrast, anthropometrical neonatal parameters can influence the nutritional status further on in life. Therefore, a recent study emphasized that a lower PI [i.e., PI, an index computed as birth weight (kg) divided by birth length (m) cubed] suggests that an individual is more vulnerable to the negative effects of the A risk allele of FTO polymorphism on body fat mass (34). In contrast, it was also shown that mothers carrying the variant A allele of rs9939609 FTO polymorphism had newborns with a lower BMI (9). Further functional studies are needed to determine the precise role of the FTO gene in determining obesity and its complications.
Leptin is a hormone synthesized and secreted into the blood flow by white adipocytes and plays a key role in regulating control of food intake and energy balance. It was proven that, in obese individuals, there is an endogenous leptin resistance mechanism that limits these regulatory effects, explaining the correlation between serum leptin levels and body fat mass (35). This leptin resistance mechanism was related to the presence of the A allele variant of the FTO gene (36), partially explaining the associations of the FTO gene polymorphisms with increased dietary consumption (37) or a hyperphagic phenotype (27, 28). During pregnancy, leptin is synthesized by not only the adipose tissues of both the mother and fetus but also the placenta, and it was proven that the leptin levels in the umbilical cord are positively correlated with birth weight (38, 39). Leptin exerts its roles through the leptin receptor; therefore, the leptin receptor gene is considered a biological pathway related to obesity development (9). A recent study focused on the positive effect of neonatal and maternal leptin gene receptor rs1137101 polymorphism on birth weight and BMI (9). The congenital deficit of leptin results in morbid obesity, severe hyperphagia, hyperinsulinemia or type 2 diabetes mellitus, hypogonadotropic hypogonadism, hypofunction of T cells, and endocrine or metabolic dysfunctions (40, 41). However, mutations in the leptin gene are associated with T cells of normal function and moderate obesity (42). It is well-documented that serum leptin levels depend on age and sex and are correlated with body fat mass (42–44). A study performed on white children assessing 223, 492, and 1019 leptin receptor gene polymorphisms concluded that the most frequent combinations in children with obesity were AG/GG/GA, AG/GG/GG, and AA/GG/GA (10). The same study also underlined that anthropometrical parameters and leptin and adiponectin levels are correlated with the variant genotype of the leptin receptor 223 gene. Therefore, it is well documented that leptin gene receptor 223, 1019, 492, and 976 polymorphisms can modulate the nutritional status in both normal and overweight/obese children (10), but these effects also depend on environmental, nutritional, and social factors. Thus, it was proven that even from birth, weight is positively correlated with maternal fat mass, total body water, body metabolism rate, and metabolic age, whereas it is negatively correlated with maternal smoking status (11). Leptin and leptin gene receptor polymorphisms play a well-established role in childhood obesity.
TNF-α is a proinflammatory cytokine expressed as a cell surface transmembrane protein involved in the pathogenesis of multiple inflammatory disorders located on the chromosome 6, site p21.1–21.3. It is also proven to have a catabolic role in infection and cancer but can also be a mediator of cachexia with associated hyperlipidemia (45). Therefore, TNF-α is involved in lipid metabolism leading to hypertriglyceridemia as a result of decreasing lipoprotein lipase activity (46) and increasing the hepatic de novo synthesis of fatty acids (47). It is well known that in subjects with obesity, TNF-α expression is high and correlated with hyperinsulinemia (48). Furthermore, TNF-α was found to regulate leptin expression and secretion (49). A G/A substitution in the promoter region (G-308) of the TNF-α gene was identified (50). Thus, the G-308A polymorphism of this gene is associated with hypertension, leptin levels, and hypercholesterolemia, resulting in metabolic syndrome development (51). In contrast, studies on Caucasian (52) and Chinese (53) populations found correlations between TNF-α 308 G allele and obesity risk. Regarding the presence of different genotypes, Sobti et al. emphasized that the AA and GA genotypes of this gene are more frequently associated with obesity in men, whereas in women, the AG genotype was associated with a higher risk for obesity (54). Nonetheless, a study performed on Iranian subjects failed to prove any correlation between TNF-α 308 G>A gene polymorphism and obesity (55). Similarly, a study performed on Romanian children showed that the variant genotype of TNF-α 308 G>A gene polymorphism was found most frequently in normal weight children (12). Based on all previously mentioned studies, the role of TNF-α 308 G>A gene polymorphism is not clear, but obesity can indeed be considered an inflammatory status.
The MC4R gene is located on chromosome 18q21.32 and, similar to the FTO gene, plays a regulatory role in food intake control and energy balance (56, 57). The common rs17782313 MC4R gene polymorphism was associated with obesity in both European adults and children showing a synergistic effect with FTO gene on obese phenotype (58–60). Rarely, mutations in MC4R gene leading to function loss can lead to monogenic forms of obesity (58), but MC4R-linked obesity is better defined as a particular form which stands between rare recessive monogenic obesity forms and common polygenic ones (8). The rs12970134 and rs17782313 polymorphisms of the MC4R gene were identified to be associated with child and adult obesity, respectively in both Asian and European populations (58, 61–65). A recent study underlined a strong effect of MC4R rs17782313 on body size and fat distribution, proving that the C/C genotype is associated with higher BMI (66). Another study that assessed the same polymorphism of MC4R gene highlighted a significant association with obesity in Mexican children (67). In addition, the authors also identified that both MC4R and FTO risk genotypes increase the metabolic risk in children with obesity (67). The same findings were also identified in Greek children and adolescents with obesity, but the study failed to show an association with the metabolic profile of these children (60). Another important recent discovery is that adiponectin may be involved in mediating the effect of MC4R gene on obesity, based on the findings of Wu et al. who reported that the rs17782313 MC4R gene polymorphism is associated with adiponectin in Chinese children (68). A study performed on newborns in Greece assessed both risk FTO and MC4R variants and concluded that approximately 80% of the Greek population are genetically predisposed to obesity development further on in life (69). According to the results of several studies, the individuals at high risk for obesity development may be homozygous for both FTO and MC4R genes or may be homozygous for one of the two genes and heterozygous for the other (70–72). In contrast, it has also been proven that the rs17782313 MC4R polymorphism has a key role in the eating behavior and control of the eating behavior. Therefore, a recent study performed on children with obesity showed that obese girls that carry the C allele of this genotype express lower satiety responsiveness and higher uncontrolled eating scores in comparison to noncarriers, whereas, in obese boys, the carriers of the same allele present a lower rewarding value of food compared with those who do not carry the C allele Similarly, other studies performed on both adults and children showed that the expression of MC4R and FTO genes can be modulated by lifestyle and physical activity (73, 74).
ENPP1 or plasma cell glycoprotein 1 (PC-1) is located on chromosome 6q23.2 and was identified to encode a protein that inhibits insulin signaling (75). The role of this gene in energy metabolism and fat tissue physiology has been widely assessed in the literature (76). K121Q polymorphism was associated with insulin resistance, type 2 diabetes mellitus, and obesity, but the results are contradictory (75, 77–81). In addition to this polymorphism, Bockenski et al. also identified the rs997509 ENPP1 gene polymorphism as a potential contributor to the development of type 2 diabetes mellitus among individuals with obesity (82). Similarly, a study performed on children with obesity underlined the potential role of K121Q ENPP1 polymorphism in not only early perturbations of glucose and insulin metabolism but also subsequent obesity development (83). A large study performed on 1,685 obese and normal-weight Mexican children found nominal associations between different gene polymorphisms, like those in ENPP1 rs7754561 and MC4R rs17782313, and obesity risk or BMI (84). Interestingly, the same authors identified a different pattern for the risk allele of ENPP1 rs7754561 in Mexican individuals showing a protective role in obesity development compared with that in European individuals where the same gene presented positive associations with obesity risk (84). It has also been proven that the overexpression of ENPP1 in human adipocyte cell lines leads to impaired adipocyte maturation (85). In addition to insulin resistance, the K121Q polymorphism was also proven to be associated with different obesity-related phenotypes such as percentage of body fat, fat mass, and plasma insulin levels (86).
The peroxisome proliferator-activated receptor gamma (PPARG), located on chromosome 3p25.2, plays a role in regulating adipocyte differentiation and influences BMI and glucose metabolism (87). The rs1801282 Pro12Ala polymorphism of this gene has been associated with obesity risk, insulin resistance, type 2 diabetes mellitus, and cardiovascular events (88, 89). A recent study confirmed the association between PPARG and obesity in young adults (66). In addition, a study performed on mothers and their newborns emphasized that homozygous CC carriers of the PPARG genotype presented a higher risk for obesity than heterozygous newborns (13). Moreover, the same authors showed that mothers carrying the homozygous CC genotype of the same gene gave birth to newborns with increased risk for obesity (13).
Angiotensin-converting enzyme (ACE) located on chromosome 17q23.3 has also been shown to have a potential role in obesity development. The ACE rs4646994 has been associated with adiposity and metabolic disorders (90, 91). A potential functional correlation between the PPARG and ACE genes has been underlined based on the fact that PPARG controls the renin-angiotensin system through the transcriptional modulation of renin, angiotensinogen, ACE, and angiotensin II receptor 1 (92). Nevertheless, the results reported in the literature remain contradictory. Certain studies performed on children found that I/I carriers of the ACE gene are associated with higher BMI,14 whereas others reported that the D/D genotype of the same gene is linked with increased values of the anthropometric parameters (91, 93). Moreover, other findings have failed to identify any association between ACE gene polymorphisms and body composition (66).
Another class of gene associated with a potential role in obesity development is the glutathione S-transferase (GST), which is involved in different intermediary chemical reactions with glutathione (94). It was proven that the null genotype of both the M1 and T1 alleles of the GST gene (GSTM1 and GSTT1) plays a major protective cellular role against xenobiotic toxic substances and oxidative stress (95, 96). Multiple studies focused on the correlations between these genotypes and neonatal birth weight (15, 97–102).
Interleukin (IL) 6 is a proinflammatory cytokine found to modulate the function of adipose tissue by regulating energy balance, presenting high levels in both obesity and cardiovascular diseases (103–106). Certain genetic studies proved that different polymorphisms of the IL-6 gene present a key role in transcriptional regulation and influence plasmatic cytokine levels, supporting the fact that this gene interacts with metabolic modulation, resulting in different metabolic conditions, such as obesity (107). The G alleles of both IL-6 174 and IL-6 572 genes were associated with obesity and type 2 diabetes mellitus (108). Similarly, in a study performed on Caucasian children, the IL-6572CC, IL-6 190 CC, and IL-6 174 CG genotypes were encountered more frequently in children with obesity, whereas the IL-6 174 CC genotype was found to be a protective factor for childhood obesity (16). Nevertheless, further studies are needed to fully understand the role of the genome in childhood obesity onset.
Even though recent genetic studies identified over 50 genetic loci, dietary interventions and proper lifestyle changes can prevent obesity development in genetically predisposed people. Therefore, screening programs could be useful in identifying high-risk children, who could benefit from proper prophylactic measures. Perhaps, further studies should also focus on developing targeted genetic therapies designed for children that carry the burden of obesity risk.
COM, CM, and LEM conceptualized and designed the study, drafted the initial manuscript, and reviewed and revised the manuscript. All authors approved the final manuscript as submitted and agree to be accountable for all aspects of the work.
The authors declare that the research was conducted in the absence of any commercial or financial relationships that could be construed as a potential conflict of interest.
The reviewer SM and handling editor declared their shared affiliation.
The English proofreading was managed by Editage Language Editing Services (https://app.editage.com/orders/download-files/CRARG_4).
This research was partially supported by the Collective Research Grants of the University of Medicine and Pharmacy Tîrgu Mureş, Romania (The role of mother's genetic determinism in child's obesity correlated with bioimpedance and anthropometric parameters no.275/4/11.01.2017).
ACE, angiotensin-converting enzyme; ENPP1, ectoenzyme nucleotide pyrophosphate phosphodiesterase 1; FTO, fat mass and obesity-associated gene, GST, glutathione S-transferase; IL, interleukin; MC4R, melanocortin 4 receptor gene; PC-1, plasma cell glycoprotein 1; PI, ponderal index; PPARG, peroxisome proliferator-activated receptor gamma; TNF-α, tumor necrosis factor alpha.
1. Danese A, Tan M. Childhood maltreatment and obesity: systematic review and meta-analysis. Mol Psychiatry (2014) 19:544–54. doi: 10.1038/mp.2013.54
2. Chirita-Emandi A, Barbu CG, Cinteza EE, Chesaru BI, Gafencu M, Mocanu V, et al. Overweight and underweight prevalence trends in children from Romania - pooled analysis of cross-sectional studies between 2006 and 2015. Obes Facts (2016) 9:206–20. doi: 10.1159/000444173
3. Herrera BM, Keildson S, Lindgren CM. Genetics and epigenetics of obesity. Maturitas (2011) 69:41–9. doi: 10.1016/j.maturitas.2011.02.018
4. Elks CE, den Hoed M, Zhao JH, Sharp SJ, Wareham NJ, Loos RJ, et al. Variability in the heritability of body mass index: a systematic review and meta-regression. Front Endocrinol. (2012) 3:29. doi: 10.3389/fendo.2012.00029
5. Wardle J, Carnell S, Haworth CM, Plomin R. Evidence for a strong genetic influence on childhood adiposity despite the force of the obesogenic environment. Am J Clin Nutr. (2008) 87:398–404. doi: 10.1093/ajcn/87.2.398
6. Silventoinen K, Rokholm B, Kaprio J, Sørensen TI. The genetic and environmental influences on childhood obesity: a systematic review of twin and adoption studies. Int J Obes. (2010) 34:29–40. doi: 10.1038/ijo.2009.177
7. Bouchard C. Defining the genetic architecture of the predisposition to obesity: a challenging but not insurmountable task. Am J Clin Nutr. (2010) 91:5–6. doi: 10.3945/ajcn.2009.28933
8. Tounian P. Programming towards childhood obesity. Ann Nutr Metab. (2011) 58 (Suppl. 2):30–41. doi: 10.1159/000328038
9. Mărginean C, Mărginean CO, Iancu M, Melit LE, Tripon F, Bănescu C. The FTO rs9939609 and LEPR rs1137101 mothers-newborns gene polymorphisms and maternal fat mass index effects on anthropometric characteristics in newborns: a cross-sectional study on mothers-newborns gene polymorphisms-The FTO-LEPR Study (STROBE-compliant article). Medicine (2016) 95:e5551. doi: 10.1097/MD.0000000000005551
10. Mărginean CO, Mărginean C, Voidăzan S, Melit L, Crauciuc A, Duicu C, et al. Correlations between leptin gene polymorphisms 223 A/G, 1019 G/A, 492 G/C, 976 C/A, and anthropometrical and biochemical parameters in children with obesity: a prospective case-control study in a Romanian population-The Nutrichild Study. Medicine (2016) 95:e3115. doi: 10.1097/MD.0000000000003115
11. Mărginean C, Mărginean CO, Bănescu C, Melit L, Tripon F, Iancu M. Impact of demographic, genetic, and bioimpedance factors on gestational weight gain and birth weight in a Romanian population: a cross-sectional study in mothers and their newborns: the Monebo study (STROBE-compliant article). Medicine (2016) 95:e4098. doi: 10.1097/MD.0000000000004098
12. Mărginean C, Mărginean C, Iancu M, Moldovan V, Meliş L, Bănescu C. The impact of TNF-α 308G>A gene polymorphism in child's overweight risk coupled with the assessment of biochemical parameters – a cross-sectional single center experience. Pediatr Neonatol. (2018) 2018:1–9. doi: 10.1016/j.pedneo.2018.03.003
13. Mărginean C, Mărginean CO, Iancu M, Szabo B, Cucerea M, Melit LE, et al. The role of TGF-β1 869 T > C and PPAR γ2 34 C > G polymorphisms, fat mass, and anthropometric characteristics in predicting childhood obesity at birth: a cross-sectional study according the parental characteristics and newborn's risk for child obesity (the newborns obesity's risk) NOR study. Medicine (2016) 95:e4265. doi: 10.1097/MD.0000000000004265
14. Mărginean CO, Bănescu C, Duicu C, Voidăzan S, Mărginean C. Angiotensin-converting enzyme gene insertion/deletion polymorphism in nutritional disorders in children. Eur J Nutr. (2015) 54:1245–54. doi: 10.1007/s00394-014-0802-0
15. Mărginean C, Bănescu CV, Mărginean CO, Tripon F, Melit LE, Iancu M. Glutathione S-transferase (GSTM1, GSTT1) gene polymorphisms, maternal gestational weight gain, bioimpedance factors and their relationship with birth weight: a cross-sectional study in Romanian mothers and their newborns. Romanian J Morphol Embryol. (2017) 58:1285–93.
16. Mărginean CO, Bănescu C, Duicu C, Pitea AM, Voidăzan S, Mărginean C. The role of IL-6 572 C/G, 190 C/T, and 174 G/C gene polymorphisms in children's obesity. Eur J Pediatr. (2014) 173:1285–96. doi: 10.1007/s00431-014-2315-5
17. Elks CE, Loos RJ, Sharp SJ, Langenberg C, Ring SM, Timpson NJ, et al. Genetic markers of adult obesity risk are associated with greater early infancy weight gain and growth. PLoS Med. (2010) 7:e1000284. doi: 10.1371/journal.pmed.1000284
18. Elks C, Loos R, Hardy R. Adult obesity susceptibility variants are associated with greater childhood weight gain and a faster tempo of growth: the 1946 British Birth Cohort Study. Am J Clin Nutr. (2012) 95:1150–56. doi: 10.3945/ajcn.111.027870
19. Belsky DW, Moffitt TE, Houts R, Bennett GG, Biddle AK, Blumenthal JA, et al. Polygenic risk, rapid childhood growth, and the development of obesity: evidence from a 4-decade longitudinal study. Arch Pediatr Adolesc Med. (2012) 166:515–21. doi: 10.1001/archpediatrics.2012.131
20. Kolačkov K, Łaczmanski Ł, Lwow F, Ramsey D, Zdrojowy-Wełna A, Tupikowska M, et al. The frequencies of haplotypes of FTO gene variants and their association with the distribution of sody fat in non-obese poles. Adv Clin Exp Med. (2016) 25:33–42. doi: 10.17219/acem/60645
21. Hinney A, Vogel CI, Hebebrand J. From monogenic to polygenic obesity: recent advances. Eur Child Adolesc Psychiatry (2010) 19:297–310. doi: 10.1007/s00787-010-0096-6
22. Loos RJ. Genetic determinants of common obesity and their value in prediction. Best Pract Res Clin Endocrinol Metab. (2012) 26:211–26. doi: 10.1016/j.beem.2011.11.003
23. Mason K, Page L, Balikclouglu P. Screening for hormonal, monogenic, and syndromic disorders in obese infants and children. Pediatr Ann. (2014) 43:e218–24. doi: 10.3928/00904481-20140825-08
24. Lima W, Glaner M, Taylor A. Fenótipo da gordura, fatores associados e o polimorfismo rs9939609 do gene FTO. Rev Bras Cineantropometria Amp Desempenho Hum. (2010) 12:164–72. doi: 10.5007/1980-0037.2010v12n2p164
25. Apalasamy YD, Ming MF, Rampal S, Bulgiba A, Mohamed Z. Genetic association of SNPs in the FTO gene and predisposition to obesity in Malaysian Malays. Braz J Med Biol Res Rev Bras Pesqui Medicas E Biol. (2012) 45:1119–26. doi: 10.1590/S0100-879X2012007500134
26. Reuter CP, Burgos MS, Bernhard JC, Tornquist D, Klinger EI, Borges TS, et al. Association between overweight and obesity in schoolchildren with rs9939609 polymorphism (FTO) and family history for obesity. J Pediatr. (2016) 92:493–8. doi: 10.1016/j.jped.2015.11.005
27. Cecil JE, Tavendale R, Watt P, Hetherington MM, Palmer CNA. An obesity-associated FTO gene variant and increased energy intake in children. N Engl J Med. (2008) 359:2558–66. doi: 10.1056/NEJMoa0803839
28. Tanofsky-Kraff M, Han JC, Anandalingam K, Shomaker LB, Columbo KM, Wolkoff LE, et al. The FTO gene rs9939609 obesity-risk allele and loss of control over eating. Am J Clin Nutr. (2009) 90:1483–8. doi: 10.3945/ajcn.2009.28439
29. Yang M, Xu Y, Liang L, Fu J, Xiong F, Liu G, et al. The effects of genetic variation in FTO rs9939609 on obesity and dietary preferences in Chinese Han children and adolescents. PloS ONE (2014) 9:e104574. doi: 10.1371/journal.pone.0104574
30. Pereira P, de A Alvim-Soares AM, Sandrim VC, Moreira Lanna CM, Souza-Costa DC, de Almeida Belo V, et al. Lack of association between genetic polymorphism of FTO, AKT1 and AKTIP in childhood overweight and obesity. J Pediatr. (2016) 92:521–7. doi: 10.1016/j.jped.2015.12.007
31. Grant SF, Li M, Bradfield JP, Kim CE, Annaiah K, Santa E, et al. Association analysis of the FTO gene with obesity in children of Caucasian and African ancestry reveals a common tagging SNP. PloS ONE (2008) 3:e1746. doi: 10.1371/journal.pone.0001746
32. Zou ZC, Mao LJ, Shi YY, Chen JH, Wang LS, Cai W. Effect of exercise combined with dietary intervention on obese children and adolescents associated with the FTO rs9939609 polymorphism. Eur Rev Med Pharmacol Sci. (2015) 19:4569–75.
33. Prakash J, Mittal B, Srivastava A, Awasthi S, Srivastava N. Association of FTO rs9939609 SNP with obesity and obesity- associated phenotypes in a North Indian population. Oman Med J. (2016) 31:99–106. doi: 10.5001/omj.2016.20
34. Labayen I, Ruiz JR, Ortega FB, Gottrand F, Huybrechts J, Dallongeville J, et al. Body size at birth modifies the effect of fat mass and obesity associated (FTO) rs9939609 polymorphism on adiposity in adolescents: the Healthy Lifestyle in Europe by Nutrition in Adolescence (HELENA) study. Br J Nutr. (2012) 107:1498–504. doi: 10.1017/S0007114511004600
35. Könner AC, Klöckener T, Brüning JC. Control of energy homeostasis by insulin and leptin: targeting the arcuate nucleus and beyond. Physiol Behav. (2009) 97:632–8. doi: 10.1016/j.physbeh.2009.03.027
36. Labayen I, Ruiz JR, Ortega FB, Dallongeville J, Jiménez-Pavón D, Castillo MJ, et al. Association between the FTO rs9939609 polymorphism and leptin in European adolescents: a possible link with energy balance control. The HELENA study. Int J Obes. (2011) 35:66–71. doi: 10.1038/ijo.2010.219
37. Timpson NJ, Emmett PM, Frayling TM, Rogers I, Hattersley AT, McCarthy MI, et al. The FTO/obesity associated locus and dietary intake in children. Am J Clin Nutr. (2008) 88:971–8. doi: 10.1093/ajcn/88.4.971
38. Souren NY, Paulussen AD, Steyls A, Loos RJ, Stassen AP, Gielen M, et al. Common SNPs in LEP and LEPR associated with birth weight and type 2 diabetes-related metabolic risk factors in twins. Int J Obes. (2008) 32:1233–9. doi: 10.1038/ijo.2008.68
39. Hauguel-de Mouzon S, Lepercq J, Catalano P. The known and unknown of leptin in pregnancy. Am J Obstet Gynecol. (2006) 194:1537–45. doi: 10.1016/j.ajog.2005.06.064
41. Mazen I, El-Gammal M, Abdel-Hamid M, Amr K. A novel homozygous missense mutation of the leptin gene (N103K) in an obese Egyptian patient. Mol Genet Metab. (2009) 97:305–8. doi: 10.1016/j.ymgme.2009.04.002
42. Fischer-Posovszky P, von Schnurbein J, Moepps B, Lahr G, Strauss G, Barth TF, et al. A new missense mutation in the leptin gene causes mild obesity and hypogonadism without affecting T cell responsiveness. J Clin Endocrinol Metab. (2010) 95:2836–40. doi: 10.1210/jc.2009-2466
43. Kelesidis T, Kelesidis I, Chou S, Mantzoros CS. Narrative review: the role of leptin in human physiology: emerging clinical applications. Ann Intern Med. (2010) 152:93–100. doi: 10.7326/0003-4819-152-2-201001190-00008
44. Arora S. Leptin and its metabolic interactions: an update. Diabetes Obes Metab. (2008) 10:973–93. doi: 10.1111/j.1463-1326.2008.00852.x
45. Beutler B, Cerami A. Cachectin (tumor necrosis factor): a macrophage hormone governing cellular metabolism and inflammatory response. Endocr Rev. (1988) 9:57–66. doi: 10.1210/edrv-9-1-57
46. Morin CL, Schlaepfer IR, Eckel RH. Tumor necrosis factor-alpha eliminates binding of NF-Y and an octamer-binding protein to the lipoprotein lipase promoter in 3T3-L1 adipocytes. J Clin Invest. (1995) 95:1684–9. doi: 10.1172/JCI117844
47. Feingold KR, Grunfeld C. Role of cytokines in inducing hyperlipidemia. Diabetes (1992) 41 (Suppl. 2):97–101. doi: 10.2337/diab.41.2.S97
48. Hotamisligil GS, Arner P, Caro JF, Atkinson RL, Spiegelman BM. Increased adipose tissue expression of tumor necrosis factor-alpha in human obesity and insulin resistance. J Clin Invest. (1995) 95:2409–15. doi: 10.1172/JCI117936
49. Trujillo ME, Lee MJ, Sullivan S, Feng J, Schneider SH, Greenberg AS, et al. Tumor necrosis factor alpha and glucocorticoid synergistically increase leptin production in human adipose tissue: role for p38 mitogen-activated protein kinase. J Clin Endocrinol Metab. (2006) 91:1484–90. doi: 10.1210/jc.2005-1901
50. Wilson AG, di Giovine FS, Blakemore AI, Duff GW. Single base polymorphism in the human tumour necrosis factor alpha (TNF alpha) gene detectable by NcoI restriction of PCR product. Hum Mol Genet. (1992) 1:353.
51. Gupta V, Gupta A, Jafar T, Gupta V, Agrawal S, Srivastava N, et al. Association of TNF-α promoter gene G-308A polymorphism with metabolic syndrome, insulin resistance, serum TNF-α and leptin levels in Indian adult women. Cytokine (2012) 57:32–6. doi: 10.1016/j.cyto.2011.04.012
52. Hoffstedt J, Eriksson P, Hellström L, Rössner S, Rydén M, Arner P. Excessive fat accumulation is associated with the TNF alpha-308 G/A promoter polymorphism in women but not in men. Diabetologia (2000) 43:117–20. doi: 10.1007/s001250050015
53. Lee SC, Pu YB, Thomas GN, Lee Zs, Tomlinson B, Cockram CS, et al. Tumor necrosis factor alpha gene G-308A polymorphism in the metabolic syndrome. Metabolism (2000) 49:1021–4. doi: 10.1053/meta.2000.7704
54. Sobti RC, Kler R, Sharma YP, Talwar KK, Singh N. Risk of obesity and type 2 diabetes with tumor necrosis factor-α 308G/A gene polymorphism in metabolic syndrome and coronary artery disease subjects. Mol Cell Biochem. (2012) 360:1–7. doi: 10.1007/s11010-011-0917-z
55. Hedayati M, Sharifi K, Rostami F, Daneshpour MS, Zarif Yeganeh M, Azizi F. Association between TNF-α promoter G-308A and G-238A polymorphisms and obesity. Mol Biol Rep. (2012) 39:825–9. doi: 10.1007/s11033-011-0804-4
56. Razquin C, Marti A, Martinez JA. Evidences on three relevant obesogenes: MC4R, FTO and PPARγ. Approaches for personalized nutrition. Mol Nutr Food Res. (2011) 55:136–49. doi: 10.1002/mnfr.201000445
57. Willer CJ, Speliotes EK, Loos RJ, Li S, Lindgren CM, Held IM, et al. Six new loci associated with body mass index highlight a neuronal influence on body weight regulation. Nat Genet. (2009) 41:25–34. doi: 10.1038/ng.287
58. Loos RJ, Lindgren CM, Li S, Wheeler E, Zhao JH, Prokopenko I, et al. Common variants near MC4R are associated with fat mass, weight and risk of obesity. Nat Genet. (2008) 40:768–75. doi: 10.1038/ng.140
59. Xi B, Chandak GR, Shen Y, Wang Q, Zhou D. Association between common polymorphism near the MC4R gene and obesity risk: a systematic review and meta-analysis. PloS ONE (2012) 7:e45731. doi: 10.1371/journal.pone.0045731
60. Lazopoulou N, Gkioka E, Ntalla I, Pervanidou P, Magiakou AM, Roma-Giannikou E, et al. The combined effect of MC4R and FTO risk alleles on childhood obesity in Greece. Horm Athens Greece (2015) 14:126–133. doi: 10.14310/horm.2002.1524
61. Chambers JC, Elliott P, Zabaneh D, Zhang W, Li Y, Froquest P, et al. Common genetic variation near MC4R is associated with waist circumference and insulin resistance. Nat Genet. (2008) 40:716–18. doi: 10.1038/ng.156
62. Zhao J, Bradfield JP, Li M, Wang K, Zhang H, Kim CE, et al. The role of obesity-associated loci identified in genome-wide association studies in the determination of pediatric BMI. Obesity (2009) 17:2254–7. doi: 10.1038/oby.2009.159
63. Scherag A, Jarick I, Grothe J, Biebermann H, Scherag S, Volckmar AL, et al. Investigation of a genome wide association signal for obesity: synthetic association and haplotype analyses at the melanocortin 4 receptor gene locus. PloS ONE (2010) 5:e13967. doi: 10.1371/journal.pone.0013967
64. Vogel CI, Boes T, Reinehr T, Roth CL, Scherag S, Scherag A, et al. Common variants near MC4R: exploring gender effects in overweight and obese children and adolescents participating in a lifestyle intervention. Obes Facts (2011) 4:67–75. doi: 10.1159/000324557
65. Dwivedi OP, Tabassum R, Chauhan G, Kaur I, Ghosh S, Marwaha RK, et al. Strong influence of variants near MC4R on adiposity in children and adults: a cross-sectional study in Indian population. J Hum Genet. (2013) 58:27–32. doi: 10.1038/jhg.2012.129
66. Bordoni L, Marchegiani F, Piangerelli M, Napolioni V, Gabbianelli R. Obesity-related genetic polymorphisms and adiposity indices in a young Italian population. IUBMB Life (2017) 69:98–105. doi: 10.1002/iub.1596
67. García-Solís P, Reyes-Bastidas M, Flores K, Garcia OP, Rosado JL, Mendez-Villa L, et al. Fat mass obesity-associated (FTO) (rs9939609) and melanocortin 4 receptor (MC4R) (rs17782313) SNP are positively associated with obesity and blood pressure in Mexican school-aged children. Br J Nutr. (2016) 116:1834–40. doi: 10.1017/S0007114516003779
68. Wu L, Gao L, Zhao X, Zhang M, Wu J, Mi J. Associations of two obesity-related single-nucleotide polymorphisms with adiponectin in Chinese children. Int J Endocrinol. (2017) 2017:6437542. doi: 10.1155/2017/6437542
69. Molou E, Schulpis KH, Birbilis C, Thodi G, Georgiou V, Dotsikas T, et al. Early screening of FTO and MC4R variants in newborns of Greek origin. J Pediatr Endocrinol Metab. (2015) 28:619–22. doi: 10.1515/jpem-2014-0320
70. Farajian P, Panagiotakos DB, Risvas G, Karasouli K, Bountziouka V, Voutzourakis N, et al. Socio-economic and demographic determinants of childhood obesity prevalence in Greece: the GRECO (Greek Childhood Obesity) study. Public Health Nutr. (2013) 16:240–7. doi: 10.1017/S1368980012002625
71. Walters RG, Jacquemont S, Valsesia A, de Smith AJ, Martinet D, Andersson J, et al. A new highly penetrant form of obesity due to deletions on chromosome 16p11.2. Nature (2010) 463:671–5. doi: 10.1038/nature08727
72. Day FR, Loos RJ. Developments in obesity genetics in the era of genome-wide association studies. J Nutr Nutr. (2011) 4:222–38. doi: 10.1159/000332158
73. Xi B, Wang C, Wu L, Zhang M, Shen Y, Zhao X, et al. Influence of physical inactivity on associations between single nucleotide polymorphisms and genetic predisposition to childhood obesity. Am J Epidemiol. (2011) 173:1256–62. doi: 10.1093/aje/kwr008
74. Ortega-Azorín C, Sorlí JV, Asensio EM, Coltell O, Martinez-Conzalez MA, Salas-Salvado J, et al. Associations of the FTO rs9939609 and the MC4R rs17782313 polymorphisms with type 2 diabetes are modulated by diet, being higher when adherence to the Mediterranean diet pattern is low. Cardiovasc Diabetol. (2012) 11:137. doi: 10.1186/1475-2840-11-137
75. Abate N, Carulli L, Cabo-Chan A, Chandalia M, Snell PG, Grundy SM. Genetic polymorphism PC-1 K121Q and ethnic susceptibility to insulin resistance. J Clin Endocrinol Metab. (2003) 88:5927–34. doi: 10.1210/jc.2003-030453
76. Goldfine ID, Maddux BA, Youngren JF, Reaven G, Accili D, Trischitta V, et al. The role of membrane glycoprotein plasma cell antigen 1/ectonucleotide pyrophosphatase phosphodiesterase 1 in the pathogenesis of insulin resistance and related abnormalities. Endocr Rev. (2008) 29:62–75. doi: 10.1210/er.2007-0004
77. Abate N, Chandalia M, Satija P, Adams-Hust B, Grundy SM, Sandeep S, et al. ENPP1/PC-1 K121Q polymorphism and genetic susceptibility to type 2 diabetes. Diabetes (2005) 54:1207–13. doi: 10.2337/diabetes.54.4.1207
78. Gu HF, Almgren P, Lindholm E, Frittitta L, Pizzuti A, Trischitta V, et al. Association between the human glycoprotein PC-1 gene and elevated glucose and insulin levels in a paired-sibling analysis. Diabetes (2000) 49:1601–3. doi: 10.2337/diabetes.49.9.1601
79. Kubaszek A, Markkanen A, Eriksson JG, Forsen T, Osmond C, Barker DJ, et al. The association of the K121Q polymorphism of the plasma cell glycoprotein-1 gene with type 2 diabetes and hypertension depends on size at birth. J Clin Endocrinol Metab. (2004) 89:2044–7. doi: 10.1210/jc.2003-031350
80. Matsuoka N, Patki A, Tiwari HK, Allison DB, Johnson SB, Gregersen PK, et al. Association of K121Q polymorphism in ENPP1 (PC-1) with BMI in Caucasian and African-American adults. Int J Obes. (2006) 30:233–7. doi: 10.1038/sj.ijo.0803132
81. Meyre D, Bouatia-Naji N, Tounian A, Samson C, Lecoeur C, Vatin V, et al. Variants of ENPP1 are associated with childhood and adult obesity and increase the risk of glucose intolerance and type 2 diabetes. Nat Genet. (2005) 37:863–7. doi: 10.1038/ng1604
82. Bochenski J, Placha G, Wanic K, Malecki M, Sieradzki J, Warram JH, et al. New polymorphism of ENPP1 (PC-1) is associated with increased risk of type 2 diabetes among obese individuals. Diabetes (2006) 55:2626–30. doi: 10.2337/db06-0191
83. Böttcher Y, Körner A, Reinehr T, Enigk B, Kiess W, Stumvoll M, et al. ENPP1 variants and haplotypes predispose to early onset obesity and impaired glucose and insulin metabolism in German obese children. J Clin Endocrinol Metab. (2006) 91:4948–52. doi: 10.1210/jc.2006-0540
84. Mejía-Benítez A, Klünder-Klünder M, Yengo L, Mayre D, Aradillas C, Cruz E, et al. Analysis of the contribution of FTO, NPC1, ENPP1, NEGR1, GNPDA2 and MC4R genes to obesity in Mexican children. BMC Med Genet. (2013) 14:21. doi: 10.1186/1471-2350-14-21
85. Liang J, Fu M, Ciociola E, Chandalia M, Abate N. Role of ENPP1 on adipocyte maturation. PloS ONE (2007) 2:e882. doi: 10.1371/journal.pone.0000882
86. Prakash J, Mittal B, Awasthi S, Agarwal CG, Srivastava N. K121Q ENPP1/PC-1 gene polymorphism is associated with insulin resistance in a North Indian population. J Genet. (2013) 92:571–6. doi: 10.1007/s12041-013-0287-2
87. Tontonoz P, Hu E, Devine J, Beale EG, Spiegelman BM. PPAR gamma 2 regulates adipose expression of the phosphoenolpyruvate carboxykinase gene. Mol Cell Biol. (1995) 15:351–7. doi: 10.1128/MCB.15.1.351
88. Masud S, Ye S, SAS Group Effect of the peroxisome proliferator activated receptor-gamma gene Pro12Ala variant on body mass index: a meta-analysis. J Med Genet. (2003) 40:773–80. doi: 10.1136/jmg.40.10.773
89. Yen CJ, Beamer BA, Negri C, Silver K, Brown KA, Yarnall DP, et al. Molecular scanning of the human peroxisome proliferator activated receptor gamma (hPPAR gamma) gene in diabetic Caucasians: identification of a Pro12Ala PPAR gamma 2 missense mutation. Biochem Biophys Res Commun. (1997) 241:270–4. doi: 10.1006/bbrc.1997.7798
90. Tiret L, Rigat B, Visvikis S, Breda C, Corvol P, Cambien F, et al. Evidence from combined segregation and linkage analysis, that a variant of the angiotensin I-converting enzyme (ACE) gene controls plasma ACE levels. Am J Hum Genet. (1992) 51:197–205.
91. Ponsonby AL, Blizzard L, Pezic A, Cochrane JA, Ellis JA, Morley R, et al. Adiposity gain during childhood, ACE I/D polymorphisms and metabolic outcomes. Obesity (2008) 16:2141–7. doi: 10.1038/oby.2008.302
92. Roszer T, Ricote M. PPARs in the renal regulation of systemic blood pressure. PPAR Res. (2010) 2010:698730. doi: 10.1155/2010/698730
93. Eisenmann JC, Sarzynski MA, Glenn K, Rothschild M, Heelan KA. ACE I/D genotype, adiposity, and blood pressure in children. Cardiovasc Diabetol. (2009) 8:14. doi: 10.1186/1475-2840-8-14
94. Hayes JD, Strange RC. Glutathione S-transferase polymorphisms and their biological consequences. Pharmacology (2000) 61:154–66. doi: 10.1159/000028396
95. Park EY, Hong YC, Lee KH, Im MW, Ha E, Kim YJ, et al. Maternal exposure to environmental tobacco smoke, GSTM1/T1 polymorphisms and oxidative stress. Reprod Toxicol. (2008) 26:197–202. doi: 10.1016/j.reprotox.2008.08.010
96. Dusinská M, Ficek A, Horská A, Petrovská H, Vallová B, Drlicková M, et al. Glutathione S-transferase polymorphisms influence the level of oxidative DNA damage and antioxidant protection in humans. Mutat Res. (2001) 482:47–55. doi: 10.1016/S0027-5107(01)00209-3
97. Suh YJ, Kim YJ, Park H, Park EA, Ha EH. Oxidative stress-related gene interactions with preterm delivery in Korean women. Am J Obstet Gynecol. (2008) 198:541.e1–7. doi: 10.1016/j.ajog.2007.11.013
98. Mustafa MD, Pathak R, Ahmed T, Ahmed RS, Tripathi AK, Guleria K, et al. Association of glutathione S-transferase M1 and T1 gene polymorphisms and oxidative stress markers in preterm labor. Clin Biochem. (2010) 43:1124–8. doi: 10.1016/j.clinbiochem.2010.06.018
99. Sata F, Yamada H, Kondo T, Gong Y, Tozaki S, Kobashi G, et al. Glutathione S-transferase M1 and T1 polymorphisms and the risk of recurrent pregnancy loss. Mol Hum Reprod. (2003) 9:165–9. doi: 10.1093/molehr/gag021
100. Wang X, Zuckerman B, Pearson C, Kaufman G, Chen C, Wang G, et al. Maternal cigarette smoking, metabolic gene polymorphism, and infant birth weight. JAMA (2002) 287:195–202. doi: 10.1001/jama.287.2.195
101. Hong YC, Lee KH, Son BK, Ha EH, Moon HS, Ha M. Effects of the GSTM1 and GSTT1 polymorphisms on the relationship between maternal exposure to environmental tobacco smoke and neonatal birth weight. J Occup Environ Med. (2003) 45:492–8. doi: 10.1097/01.jom.0000063627.37065.a1
102. Lee BE, Hong YC, Park H, Ha M, Koo BS, Chang N, et al. Interaction between GSTM1/GSTT1 polymorphism and blood mercury on birth weight. Environ Health Perspect. (2010) 118:437–43. doi: 10.1289/ehp.0900731
103. Black PH. The inflammatory response is an integral part of the stress response: implications for atherosclerosis, insulin resistance, type II diabetes and metabolic syndrome X. Brain Behav Immun. (2003) 17:350–64. doi: 10.1016/S0889-1591(03)00048-5
104. Henningsson S, Håkansson A, Westberg L, Baghaei F, Rosmond R, Holm G, et al. Interleukin-6 gene polymorphism−174G/C influences plasma lipid levels in women. Obesity (2006) 14:1868–73. doi: 10.1038/oby.2006.216
105. Kubaszek A, Pihlajamäki J, Punnonen K, Karhapää P, Vauhkonen I, Laakso M. The C-174G promoter polymorphism of the IL-6 gene affects energy expenditure and insulin sensitivity. Diabetes (2003) 52:558–61. doi: 10.2337/diabetes.52.2.558
106. Stephens JW, Hurel SJ, Cooper JA, Acharya J, Miller GJ, Humphries SE. A common functional variant in the interleukin-6 gene is associated with increased body mass index in subjects with type 2 diabetes mellitus. Mol Genet Metab. (2004) 82:180–6. doi: 10.1016/j.ymgme.2004.04.001
107. Goyenechea E, Parra D, Martínez JA. Impact of interleukin 6−174G>C polymorphism on obesity-related metabolic disorders in people with excess in body weight. Metabolism (2007) 56:1643–8. doi: 10.1016/j.metabol.2007.07.005
Keywords: obesity, genetics, children, etiology, dietary interventions
Citation: Mǎrginean CO, Mǎrginean C and Meliţ LE (2018) New Insights Regarding Genetic Aspects of Childhood Obesity: A Minireview. Front. Pediatr. 6:271. doi: 10.3389/fped.2018.00271
Received: 21 March 2018; Accepted: 10 September 2018;
Published: 04 October 2018.
Edited by:
Fatima Cody Stanford, Massachusetts General Hospital, Harvard Medical School, United StatesReviewed by:
Sonali Malhotra, Massachusetts General Hospital, Harvard Medical School, United StatesCopyright © 2018 Mǎrginean, Mǎrginean and Meliţ. This is an open-access article distributed under the terms of the Creative Commons Attribution License (CC BY). The use, distribution or reproduction in other forums is permitted, provided the original author(s) and the copyright owner(s) are credited and that the original publication in this journal is cited, in accordance with accepted academic practice. No use, distribution or reproduction is permitted which does not comply with these terms.
*Correspondence: Claudiu Mǎrginean, bWFyZ2luZWFuLmNsdWF1ZGl1QGdtYWlsLmNvbQ==
Disclaimer: All claims expressed in this article are solely those of the authors and do not necessarily represent those of their affiliated organizations, or those of the publisher, the editors and the reviewers. Any product that may be evaluated in this article or claim that may be made by its manufacturer is not guaranteed or endorsed by the publisher.
Research integrity at Frontiers
Learn more about the work of our research integrity team to safeguard the quality of each article we publish.