- 1Division of Neonatology and Pediatric Critical Care, Department of Pediatrics and Adolescent Medicine, Ulm University, Ulm, Germany
- 2Department of Traumatology, Hand, Plastic, and Reconstructive Surgery, Center of Surgery, University of Ulm, Ulm, Germany
- 3Institute of Epidemiology and Medical Biometry, Ulm University, Ulm, Germany
- 4Division of Neonatology, Department of Pediatrics, Sidra Medicine, Doha, Qatar
Background: Evidence for recommendations on the use of volume expansion during cardiopulmonary resuscitation in newborn infants is limited.
Objectives: To develop a newborn piglet model with asphyxia, hemorrhage, and cardiac arrest to test different volume resuscitation on return of spontaneous circulation (ROSC). We hypothesized that immediate red cell transfusion reduces time to ROSC as compared to the use of an isotonic crystalloid fluid.
Methods: Forty-four anaesthetized and intubated newborn piglets [age 32 h (12–44 h), weight 1,220 g (1,060–1,495g), Median (IQR)] were exposed to hypoxia and blood loss until asystole occurred. At this point they were randomized into two groups: (1) Crystalloid group: receiving isotonic sodium chloride (n = 22). (2) Early transfusion group: receiving blood transfusion (n = 22). In all other ways the piglets were resuscitated according to ILCOR 2015 guidelines [including respiratory support, chest compressions (CC) and epinephrine use]. One hour after ROSC piglets from the crystalloid group were randomized in two sub-groups: late blood transfusion and infusion of isotonic sodium chloride to investigate the effects of a late transfusion on hemodynamic parameters.
Results: All animals achieved ROSC. Comparing the crystalloid to early blood transfusion group blood loss was 30.7 ml/kg (22.3–39.6 ml/kg) vs. 34.6 ml/kg (25.2–44.7 ml/kg), Median (IQR). Eleven subjects did not receive volume expansion as ROSC occurred rapidly. Thirty-three animals received volume expansion (16 vs. 17 in the crystalloid vs. early transfusion group). 14.1% vs. 10.5% of previously extracted blood volume in the crystalloid vs. early transfusion group was infused before ROSC. There was no significant difference in time to ROSC between groups [crystalloid group: 164 s (129–198 s), early transfusion group: 163 s (162–199 s), Median (IQR)] with no difference in epinephrine use.
Conclusions: Early blood transfusion compared to crystalloid did not reduce time to ROSC, although our model included only a moderate degree of hemorrhage and ROSC occurred early in 11 subjects before any volume resuscitation occurred.
Introduction
Although adequate ventilation is the key for an effective resuscitation in the delivery room, some newborns require further assistance such as chest compressions (CC), epinephrine and in specific cases even volume resuscitation in particular in the context of neonatal shock (1). Impaired placental function resulting in fetal asphyxia as well as hypovolemia secondary to fetal blood loss (feto-maternal transfusion or a premature placental abruption) and sepsis are factors contributing to poor conditions at birth (2, 3). Therefore, ILCOR guidelines recommend volume replacement using blood or isotonic crystalloid solution (4), if the newborn infant does not respond to ventilation and CPR and this may be lifesaving (2). However, there is only limited information available on the efficacy of different types of volume replacement. In addition, O-negative, CMV-negative blood is not readily available in all delivery services but may be arranged if considered necessary. In some of these cases placental blood transfusion may be used, as this may be readily available, however data is limited to few retrospective observations as prospective studies are extremely difficult (2) (Table 1). Therefore, we designed this study to establish a model of asphyxia and hemorrhage. Based on our established model of asphyxia (15) we added a component of standardized hemorrhage to evaluate different volume resuscitation strategies in neonatal piglets. We hypothesized that use of early red cell transfusion reduces time to return of spontaneous circulation (ROSC) as compared to the use of an isotonic crystalloid fluid during resuscitation.
Methods
Animal Preparation
This experiment was approved by the governmental animal care committee (Regierungspraesidium Tuebingen, Permit No.1262). Forty-four newborn piglets [age: 32 (12–44) h; weighing: 1,220 (1,060–1,495) g; Median (IQR)] were anesthetized with propofol/fentanyl, intubated with uncuffed ET tubes and mechanically ventilated (FiO2 = 0.3; PIP = 15 cmH2O; PEEP = 5 cmH2O; inspiratory time = 0.4 s.; initial ventilator rate = 20/min which was adjusted thereafter to achieve a target PaCO2 of 35–45 mmHg) using a newborn-ventilator (Stephanie, Stephan GmbH, Gackenbach, Germany). Rectal temperature was maintained at 39.0–39.5°C using a heating mattress and an overhead warmer. A femoral double-lumen arterial line was placed to measure continuous blood pressure and to obtain routinely arterial blood gas analyses (ABG) and for blood sampling. Blood gases were analyzed using a blood gas analyzer (ABL 800, Radiometer, Willich, Germany) and special heparinized syringes. A femoral double-lumen venous line was placed to measure central venous pressure and for administration of i.v. drugs, maintenance fluids, and for administering volume. Airway, esophageal, and vascular pressures were measured continuously using a standard monitoring system (MP 50, Philips, Hamburg, Germany) and a custom-made data acquisition system (ixTrend, ixellence GmbH, Wildau, Germany).
Resuscitation Procedure
After instrumentation, FiO2 was decreased to 0.21 and after 15 min baseline measurements were recorded, and an ABG/blood sample was taken. Thereafter, progressive hypoxia was induced by reducing FiO2 to 0.08, adding CO2 (FiCO2 = 0.07) and by reducing ventilator rate by 10/min every 10 min. At 12 min or once a pH < 7.0 was achieved (whichever occurred first), hypovolemia was induced by continuous and standardized removal of blood (2 ml/kg/min) from the arterial line, using a negative pressure laboratory pump (Syringe pump LA-160, Landgraf Laborsysteme, Langenhagen, Germany). This approach was used to limit variability in the degree of hemorrhage and asphyxia components and the time limit was based on data from of our previous study (15). Blood volume depletion was continued until cardiac arrest occurred. The extracted blood was anticoagulated using a standard anticoagulant mixture and stored in perfusion syringes to be used eventually for re-transfusion. Asystole was defined as loss of pulsatility in the arterial blood pressure waveform along with loss of regular ECG activity. At this time blood extraction was stopped, an ABG was taken, respiratory support was discontinued for 30 s [mimicking the period of initial steps of the Neonatal Resuscitation Program algorithm (4)] and restarted thereafter. Animals were then randomized into one of the following two groups (Figure 1):
1. Crystalloid group: receiving sodium chloride solution 0.9%, and
2. Early transfusion group: receiving the animal's own anticoagulated blood
During resuscitation both groups received mechanical ventilation (PIP/PEEP = 25/5 cmH2O, f = 30/min, Tinsp = 0.4 s). According to current guidelines a FiO2 = 0.21 was used initially. After 30 s of adequate respiratory support CC were applied with a rate of 90/min with inflations interposed to CC using a 3:1 ratio and the 2-thumb-technique (16). Resuscitation was implemented by a trained 5-member team and CC were always given by the same team member. The appropriate rate of CC was guided using a metronome and CC depth was goal-directed to generate a systolic arterial blood pressure of 50 mmHg. Ninety seconds after asystole, FiO2 was increased to 1.0 and epinephrine (20 μg/kg) was given via the central venous line and repeated every 3 min. When resuscitation was required to be continued 120 s after asystole, volume expansion was started at a rate of 10 ml/kg over 2 min, with the volume choice dependent on the group allocation. A maximum of 3 × 10 ml/kg volume boluses were infused immediately after each other until ROSC occurred, but the infused total volume never exceeded the total volume previously extracted. Resuscitative efforts were continued until a heart rate >60/min was detected, as measured by ECG and a visible arterial blood pressure, which was defined as ROSC in our model. ABG were taken every 2 min during CPR and immediately after ROSC.
A 4-h monitoring period followed ROSC. In case of arterial hypotension [mean arterial pressure (MAP) < 50 mmHg] a single bolus of normal saline (10 ml/kg over 15 min) was given i.v. If MAP did not increase after the bolus, a second bolus of normal saline (10 ml/kg over 15 min) was given and a continuous infusion of Dopamine was started with the dose adjusted according to the response (max. 15 μg/kg/min).
Furthermore, piglets from the crystalloid group were randomized 1 h after ROSC in two sub-groups to investigate the effects of a late transfusion on hemodynamic parameters. One sub-group received late blood transfusion (10 ml/kg over 15 min) and the other sub-group received an infusion of normal saline (10 ml/kg over 15 min). The animals in the early blood-group received an infusion of normal saline at the same time in order to establish comparability regarding volume status. Four hours after ROSC piglets were euthanized using an intravenous overdose of potassium chloride during ongoing anesthesia with subsequent autopsy, where structural and immunological alterations of the heart were analyzed to be reported in another research publication.
Sample Size and Data Analysis
Based on results of a previous study done by our group, the study was powered to detect a difference in time to ROSC of 90 s (SD 102 s) between the groups with a power of 80% and a two-sided type 1 error of 0.05 (15). The calculated number of animals was 22 per group. Mean (standard deviation) or median (interquartile range) values are given as appropriate. For comparisons of crystalloid and early transfusion group, continuous data were analyzed using a non-parametric Mann-Whitney-U test and categorical variables using a chi-square or Fisher's exact test. For comparison of variables across time beginning 1 h after ROSC continuous data were analyzed using a non-parametric Kruskal-Wallis test. Statistical significance was accepted with a p < 0.05, and all secondary variables were tested in an explorative manner.
Results
Baseline Characteristics
There were no significant differences between the groups at baseline for demographic or outcome variables, except for blood glucose measurements. Blood glucose was slightly but significantly lower in the crystalloid group (Table 2).
Characteristics of Cardiac Arrest
Time of hypoxia and induction of hemorrhage to achieve asystole was similar in both groups. Average blood loss at this time was 30.7 (22.3–39.6) ml/kg in the crystalloid and 34.6 (25.2–44.7) ml/kg in the early transfusion group; Median (IQR). There was no significant difference in any of the variables between the two groups at this time (Table 3).
Characteristics of Resuscitation
Thirty-three (of the 44) animals received volume expansion, 16 in the crystalloid group and 17 in the early transfusion group (p = 0.728), the other 11 animals did not. The amount of volume expansion infused until ROSC occurred was relatively small with 14.1% and 10.5% of extracted blood because ROSC occurred early. The time until ROSC was not significantly different between the two groups. All animals received epinephrine with no significant difference in dosing between groups (Table 4).
Return of Spontaneous Circulation (ROSC)
All animals achieved ROSC. One animal in the crystalloid group and three animals in the early transfusion group died during monitoring period after successful resuscitation (p = 0.607).
Median (IQR) time to ROSC was similar with 164 (129–198) s vs. 163 (162–199) s comparing the crystalloid group and the early transfusion group (Table 4).
Arterial blood gases immediately after ROSC were similar for the two groups, except for partial pressure of oxygen (PaO2), potassium, and lactate. Significantly higher levels for potassium and lactate were measured in the early transfusion group and a higher PaO2 was observed in the crystalloid group (Table 4, Figure 2). Hemodynamic parameters were similar, except for mean arterial blood pressure, which was significantly higher in the crystalloid group (Table 4).
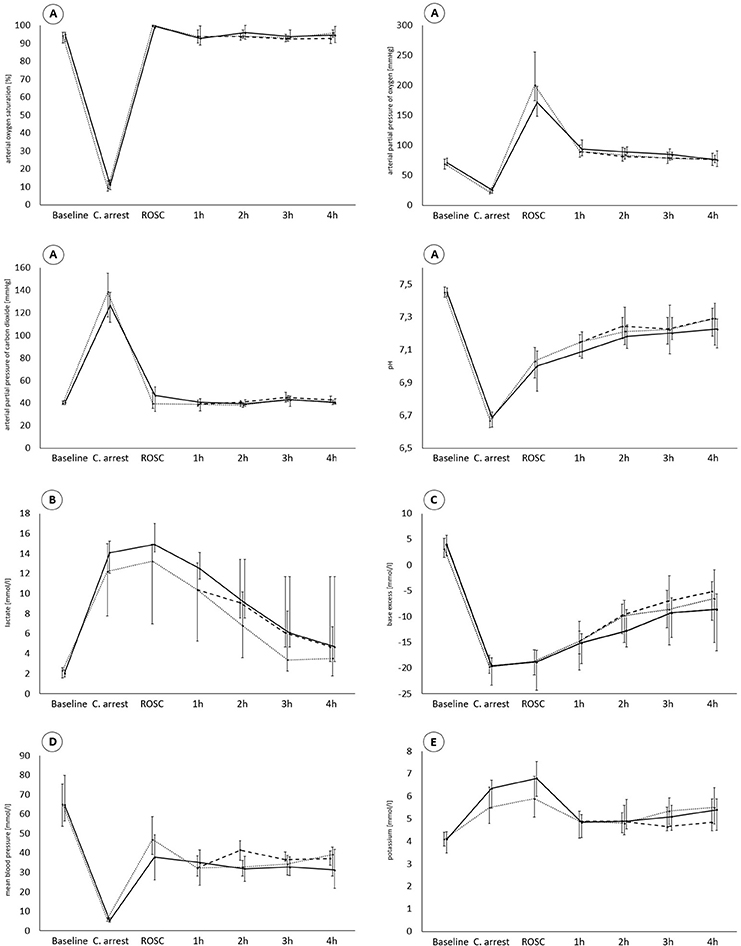
Figure 2. Traces show parameters of gas exchange (A), lactate (B), base excess (C), mean arterial blood pressure (D), and potassium (E) during all phases of experiment. ________, early transfusion group; ···············, crystalloid group; – – – – –, late transfusion (in crystalloid group); Values are presented as median (IQR).
Post-Resuscitation Period
There were no significant differences between the groups regarding blood gases, metabolic, or hemodynamic parameters during the monitoring period (see Supplementary Tables 1–3 for detailed values), except for a significantly higher lactate 1 h after ROSC in the early transfusion group and a significantly higher mean arterial blood pressure 2 h after ROSC in the subgroup (of crystalloid group) receiving late transfusion 1 h after ROSC (Table 5, Figure 2). There were also no significant differences in the use of dopamine or volume boluses supporting circulation (Table 5).
Discussion
Current resuscitation guidelines recommend volume expansion for newborn infants not responding to ventilation and CC, especially if there is a history suggesting fetal blood loss. Guidelines for volume administration were not changed in the current AHA guidelines (2015); therefore, the 2010 recommendations remain in effect (4). Administration of blood or crystalloids with no clear preference for either one is currently recommended (17). Furthermore, ERC guidelines in relation to volume administration were not changed in 2015 (18). Nevertheless, they state that in the absence of suitable blood isotonic crystalloid is the solution of choice. In this guideline four publications are referenced in relation to this topic, including an older case report by Kirkman and Riley from 1959 (5), a retrospective review from Wyckoff et al. (6) and only one randomized study in asphyxiated piglets [comparing use of crystalloids with colloidal infusion showing no difference between groups 7]. A recent review by Finn et al. states that data on different fluids for volume expansion is very limited, because prospective studies remain impracticable and until now only few studies are available on this topic (2).
Crystalloids are readily available, and their costs are low. However, they have a lower intravascular half-life of 30–60 min secondary to immediate loss of fluid into the interstitial space, as well as the lack of oxygen transport capacity as compared to blood. There are also (low) risks for infection (19) and transfusion reactions when blood is used without cross-match (20), as well as for hyperkalemia and increased lactate (21, 22).
Because of paucity of data we used an established model of hypoxic cardiac arrest in newborn piglets (15, 23) and added a standardized protocol for hemorrhage.
Our hypothesis that early blood transfusion reduces time to ROSC during resuscitation compared to using a crystalloid solution was not confirmed. We followed a protocol and were able to reproduce severe asphyxia with very similar degrees of acidosis and hypoxemia in both study groups, confirming that this model is quite stable. We followed closely the published resuscitation guidelines and used epinephrine before volume expansion and all animals received at least one dose of epinephrine, which, because of its positive effect on coronary blood flow, is considered to be important for successful resuscitation (24). Volume depletion was approximately 1/3 of the estimated blood volume. However, it seems that we were able to resuscitate many animals successfully without substantial volume resuscitation, as indicated by the fact that ROSC occurred in 25% of the animals before any volume infusion. It is likely that the degree of hemorrhagic shock was not severe enough to affect successful resuscitation as at ROSC only 12 (7–21) % [Median (IQR)] of depleted blood volume was re-transfused. This is further supported by the fact that time to ROSC is very similar to our previous study (with 150–180 s), which used exactly the same protocol to induce asphyxia, but did not include the hemorrhagic component (15). However, it also indicates that, despite relevant blood loss and asphyxia, resuscitation may be possible in a relevant proportion of subjects with no or only a minor amount of volume replacement, which may be potentially harmful (2), and therefore needs a clear indication.
We observed higher values for potassium and lactate in the early transfusion group after ROSC. This can be explained by the additional acid and potassium load caused by the early transfusion of stored blood in this group. Lactic acidosis may also affect cardiac contractility and thus may explain the lower blood pressure at this time point in the early transfusion group, as described elsewhere (25). The increased lactate was detectable only up to 1 h after ROSC, suggesting that our animals had adequate perfusion and metabolic homeostasis after successful resuscitation. The higher potassium level had already decreased at this time. With a larger transfusion volume, a relevant potassium load needs to be considered along with its side-effects on the heart. Nevertheless, there are clear advantages using blood instead of crystalloids such as a longer intravascular half-life as well as the oxygen transport capacity by red blood cells (26). The higher mean blood pressure observed in the subgroup with the late blood transfusion 1 h after ROSC, may be explained by a more stable blood volume as compared to the subgroup receiving crystalloids. We do not have a clear explanation for the slightly but significantly lower blood glucose in the crystalloid group at the baseline, but we speculate that it may be related to the somewhat younger postnatal age and its related metabolic instability. Glucose supply given by the infusion solution ensured similar conditions during the experiment including the phase of resuscitation.
Our study has several limitations. First, the study was not blinded. However, we applied a standardized protocol for CC and hemodynamic support. Second, we used the animals' whole blood, instead of packed red blood cells, which would contain higher haematocrit and may impose a higher load of potassium due to the longer storage phase. However, in some cases placental blood (with similar hematocrit) may be used as it may be readily available. Third, our animals were anesthetized, which differs from delivery room procedures and may cause interference with ROSC. The second randomization of the animals within the crystalloid group at one hour after ROSC may limit the power to show differences between groups, but only for the secondary outcomes after that time point. Furthermore, our animals had already passed their early phase of postnatal transition. However, despite this limitation, the piglet model is well-established and many groups have used it to study asphyxia and neonatal resuscitation (23). One future direction for research may be cord milking once severe bradycardia or asystole is confirmed, which may induce immediate blood transfusion via the umbilical cord. This should be studied in a transitional model, such as the lamb model of asphyxia arrest. Finally, as mentioned before the degree of volume depletion may not have been severe enough to affect resuscitation significantly and thus our findings may only apply for a mild-moderate degree of hemorrhage.
In summary, we developed our animal model with a hemorrhagic component to assess the influence of different volume resuscitation strategies on ROSC. Our hypothesis that early blood transfusion reduces time to ROSC during resuscitation compared to using a crystalloid solution was not confirmed. However, our model is limited by the fact that only 75% of the animals needed transfusion for ROSC, which occurred already at a time when only 12 (7–21)% [Median (IQR)] of depleted blood volume was re-transfused suggesting that hemorrhagic shock was not severe enough to limit resuscitation success substantially to test our hypothesis. However, we were able to show that, despite severe asphyxia and blood loss, resuscitation procedures were successful with no, or minimal transfusion. Animals required at least one adrenaline dose for successful resuscitation, confirming the central importance of this drug. Further studies are needed to investigate the influence of different volume expansion strategies in a refined model of a more severe degree of hemorrhage to identify a potential threshold of blood loss, where emergency blood transfusion might improve outcome.
Author Contributions
MM and HH conceived and designed the study. MM, SS, LH, SK, BW, and SH performed the animal experiments. MM, LH, SK, MK and BM analyzed the data. MM, BM, and HH interpreted the results obtained. MM drafted the manuscript. MM and HH revised the manuscript. All authors read and approved the final version of the manuscript and agreed to be accountable for all aspects of the work.
Conflict of Interest Statement
Fritz Stephan GmbH (Gackenbach, Germany) provided the mechanical ventilator.
The authors declare that the research was conducted in the absence of any commercial or financial relationships that could be construed as a potential conflict of interest.
Acknowledgments
We would like to thank Christoph Theurer (Philips GmbH, Hamburg, Germany), Harald Hötzel (Radiometer, Willich, Germany), and Michael Sossalla (Stephan GmbH, Gackenbach, Germany) who provided expert technical support for this study. We also like to thank Nicola Jayne Robertson (MB ChB, Ph.D., Div. of Neonatology, Sidra Medicine, Doha, Qatar) for her editorial support.
Supplementary Material
The Supplementary Material for this article can be found online at: https://www.frontiersin.org/articles/10.3389/fped.2018.00192/full#supplementary-material
References
1. Wall SN, Lee AC, Niermeyer S, English M, Keenan WJ, Carlo W, et al. Neonatal resuscitation in low-resource settings: what, who, and how to overcome challenges to scale up? Int J Gynaecol Obs. (2010) 107(Suppl. 1):S47–64. doi: 10.1016/j.ijgo.2009.07.013
2. Finn D, Roehr CC, Ryan CA, Dempsey EM. Optimising intravenous volume resuscitation of the newborn in the delivery room: practical considerations and gaps in knowledge. Neonatology (2017) 2:163–71. doi: 10.1159/000475456
3. Rainaldi MA, Perlman JM. Pathophysiology of birth asphyxia. Clin Perinatol. (2016) 43:409–22. doi: 10.1016/j.clp.2016.04.002
4. Wyckoff MH, Aziz K, Escobedo MB, Kapadia VS, Kattwinkel J, Perlman JM, et al. Part 13: Neonatal resuscitation: 2015 American Heart Association guidelines update for cardiopulmonary resuscitation and emergency cardiovascular care. Circulation (2015) 132:S543–60. doi: 10.1161/CIR.0000000000000267
5. Kirkman HN, Riley HD. Posthemorrhagic anemia and shock in the newborn. Pediatrics (1959) 24:97–105.
6. Wyckoff MH, Perlman JM, Laptook AR. Use of volume expansion during delivery room resuscitation in near-term and term infants. Pediatrics (2005) 115:950–5. doi: 10.1542/peds.2004-0913
7. Wyckoff M, Garcia D, Margraf L, Perlman J, Laptook A. Randomized trial of volume infusion during resuscitation of asphyxiated neonatal piglets. Pediatr Res. (2007) 61:415–20. doi: 10.1203/pdr.0b013e3180332c45
8. Mayock DE, Gleason CA. Cerebrovascular effects of rapid volume expansion in preterm fetal sheep. Pediatr Res. (2004) 55:395–9. doi: 10.1203/01.PDR.0000111284.29388.E7
9. Roger C, et al. Does the type of fluid affect rapidity of shock reversal in an anaesthetized-piglet model of near-fatal controlled haemorrhage? A randomized study. Br J Anaesth. (2014) 112:1015–23. doi: 10.1093/bja/aet375
10. Mauch J, Madjdpour C, Kutter AP, Spielmann N, Bettschart-Wolfensberger R, Weiss M, et al. Effect of rapid fluid resuscitation using crystalloids or colloids on hemostasis in piglets. Paediatr Anaesth. (2013) 23:258–64. doi: 10.1111/pan.12106
11. So K, Fok TF, Ng PC, Wong WW, Cheung KL. Randomised controlled trial of colloid or crystalloid in hypotensive preterm infants. Arch Dis Child Fetal Neonatal Ed. (1997) 76:43–6.
12. Oca MJ, Nelson M, Donn SM. Randomized trial of normal saline versus 5% albumin for the treatment of neonatal hypotension. J Perinatol. (2003) 23:473–6. doi: 10.1038/sj.jp.7210971
13. Lynch SK, Mullett MD, Graeber JE, Polak MJ. A comparison of albumin-bolus therapy versus normal saline-bolus therapy for hypotension in neonates. J Perinatol. (2008) 28, 29–33. doi: 10.1038/sj.jp.7211846
14. Shalish W, Olivier F, Aly H, Sant'Anna G. Uses and misuses of albumin during resuscitation and in the neonatal intensive care unit. Semin Fetal Neonatal Med. (2017) 22:328–35. doi: 10.1016/j.siny.2017.07.009
15. Mendler MR, Weber C, Hassan MA, Huang L, Waitz M, Mayer B, et al. Effect of different respiratory modes on return of spontaneous circulation in a newborn piglet model of hypoxic cardiac arrest. Neonatology (2016) 109:22–30. doi: 10.1159/000439020
16. Christman C, Hemway RJ, Wyckoff MH, Perlman JM. The two-thumb is superior to the two-finger method for administering chest compressions in a manikin model of neonatal resuscitation. Arch Dis Child Fetal Neonatal Ed. (2011) 96:F99–101. doi: 10.1136/adc.2009.180406
17. Kattwinkel J, Perlman JM, Aziz K, Colby C, Fairchild K, Gallagher J, et al. Neonatal resuscitation: 2010 American Heart Association guidelines for cardiopulmonary resuscitation and emergency cardiovascular care. Pediatrics (2010) 126:e1400–13. doi: 10.1542/peds.2010-2972E
18. Wyllie J, Bruinenberg J, Roehr CC, Rüdiger M, Trevisanuto D, Urlesberger B. European Resuscitation Council guidelines for resuscitation 2015. Section 7. Resuscitation and support of transition of babies at birth. Resuscitation (2015) 95:249–63. doi: 10.1016/j.resuscitation.2015.07.029
19. MacDonald NE, O'Brien SF, Delage G. Transfusion and risk of infection in Canada: update 2012. Paediatr Child Heal. (2012) 17: e102–11.
20. Boo NY, Chan BH. Blood transfusion reactions in Malaysian newborn infants. Med J Malaysia (1998) 53:358–64.
21. Vraets A, Lin Y, Callum JL. Transfusion-associated hyperkalemia. Transfus Med Rev (2011) 25:184–96. doi: 10.1016/j.tmrv.2011.01.006
22. Bardyn M, Rappaz B, Jaferzadeh K, Crettaz D, Tissot JD, Moon I, et al. Red blood cells ageing markers: a multi-parametric analysis. Blood Transfus. (2017) 15:239–48. doi: 10.2450/2017.0318-16
23. Solevåg AL, Dannevig I, Wyckoff M, Saugstad OD, Nakstad B. Extended series of cardiac compressions during CPR in a swine model of perinatal asphyxia. Resuscitation (2010) 81:1571–6. doi: 10.1016/j.resuscitation.2010.06.007
24. Kapadia VS, Wyckoff MH. Epinephrine use during newborn resuscitation. Front Pediatr. (2017) 5:97. doi: 10.3389/fped.2017.00097
25. McCullough PA, Beaver TM, Bennett-Guerrero E, Emmett N, Goyal A, Herzog CA, et al. Acute and chronic cardiovascular effects of hyperkalemia: new insights into prevention and clinical management. Rev Cardiovasc Med. (2014) 15:11–23. doi: 10.3909/ricm0727
Keywords: neonatal hemorrhage, neonatal resuscitation, volume resuscitation, crystalloid solution, blood transfusion, neonatal shock, ROSC
Citation: Mendler MR, Schwarz S, Hechenrieder L, Kurth S, Weber B, Höfler S, Kalbitz M, Mayer B and Hummler HD (2018) Successful Resuscitation in a Model of Asphyxia and Hemorrhage to Test Different Volume Resuscitation Strategies. A Study in Newborn Piglets After Transition. Front. Pediatr. 6:192. doi: 10.3389/fped.2018.00192
Received: 07 April 2018; Accepted: 11 June 2018;
Published: 10 July 2018.
Edited by:
Po-Yin Cheung, University of Alberta, CanadaReviewed by:
Anup C. Katheria, Sharp Mary Birch Hospital for Women & Newborns, United StatesBritt Nakstad, University of Oslo, Norway
Aakash Pandita, Sanjay Gandhi Post Graduate Institute of Medical Sciences, India
Copyright © 2018 Mendler, Schwarz, Hechenrieder, Kurth, Weber, Höfler, Kalbitz, Mayer and Hummler. This is an open-access article distributed under the terms of the Creative Commons Attribution License (CC BY). The use, distribution or reproduction in other forums is permitted, provided the original author(s) and the copyright owner(s) are credited and that the original publication in this journal is cited, in accordance with accepted academic practice. No use, distribution or reproduction is permitted which does not comply with these terms.
*Correspondence: Marc R. Mendler, bWFyYy5tZW5kbGVyQHVuaWtsaW5pay11bG0uZGU=