- 1Inflammation, Repair and Development Section, National Heart and Lung Institute, Imperial College London, London, United Kingdom
- 2Respiratory Pediatrics, The Royal Brompton Hospital, London, United Kingdom
Although a rare disease, severe therapy-resistant asthma in children is a cause of significant morbidity and results in utilization of approximately 50% of health-care resources for asthma. Improving control for children with severe asthma is, therefore, an urgent unmet clinical need. As a group, children with severe asthma have severe and multiple allergies, steroid resistant airway eosinophilia, and significant structural changes of the airway wall (airway remodeling). Omalizumab is currently the only add-on therapy that is licensed for use in children with severe asthma. However, limitations of its use include ineligibility for approximately one-third of patients because of serum IgE levels outside the recommended range and lack of clinical efficacy in a further one-third. Pediatric severe asthma is thus markedly heterogeneous, but our current understanding of the different mechanisms underpinning various phenotypes is very limited. We know that there are distinctions between the factors that drive pediatric and adult disease since pediatric disease develops in the context of a maturing immune system and during lung growth and development. This review summarizes the current data that give insight into the pathophysiology of pediatric severe asthma and will highlight potential targets for novel therapies. It is apparent that in order to identify novel treatments for pediatric severe asthma, the challenge of undertaking mechanistic studies using age appropriate experimental models and airway samples from children needs to be accepted to allow a targeted approach of personalized medicine to be achieved.
Introduction
Severe asthma is thought to be rare in children, affecting approximately 2–5% (1) of all patients; however, we have little idea of the actual size of the problem (2). The proportion of health-care resources utilized by patients with severe disease is disproportionate to prevalence, whereby, they use up to 50% of all health-care costs for asthma (2). Improving control for patients with severe asthma is, therefore, a significant unmet clinical need. Pediatric asthma is a heterogeneous disease, and within that, severe asthma is also recognized to be heterogeneous with numerous clinical, pathological, and physiological phenotypes (3). It is apparent that, in order to identify novel treatments for pediatric severe asthma, the mechanisms that mediate the disease in children need to be investigated so that a targeted approach of personalized medicine can be achieved. However, mechanistic data in childhood studies are rare, partly because obtaining airway samples from children is a challenge and also because there is a reluctance to generate age-specific experimental models. This review will summarize the current data that give insight into the pathophysiology of pediatric severe asthma and will highlight potential targets for novel therapies (Figure 1). Avenues for future research and approaches that will enable mechanistic studies to be undertaken more readily in children will also be discussed.
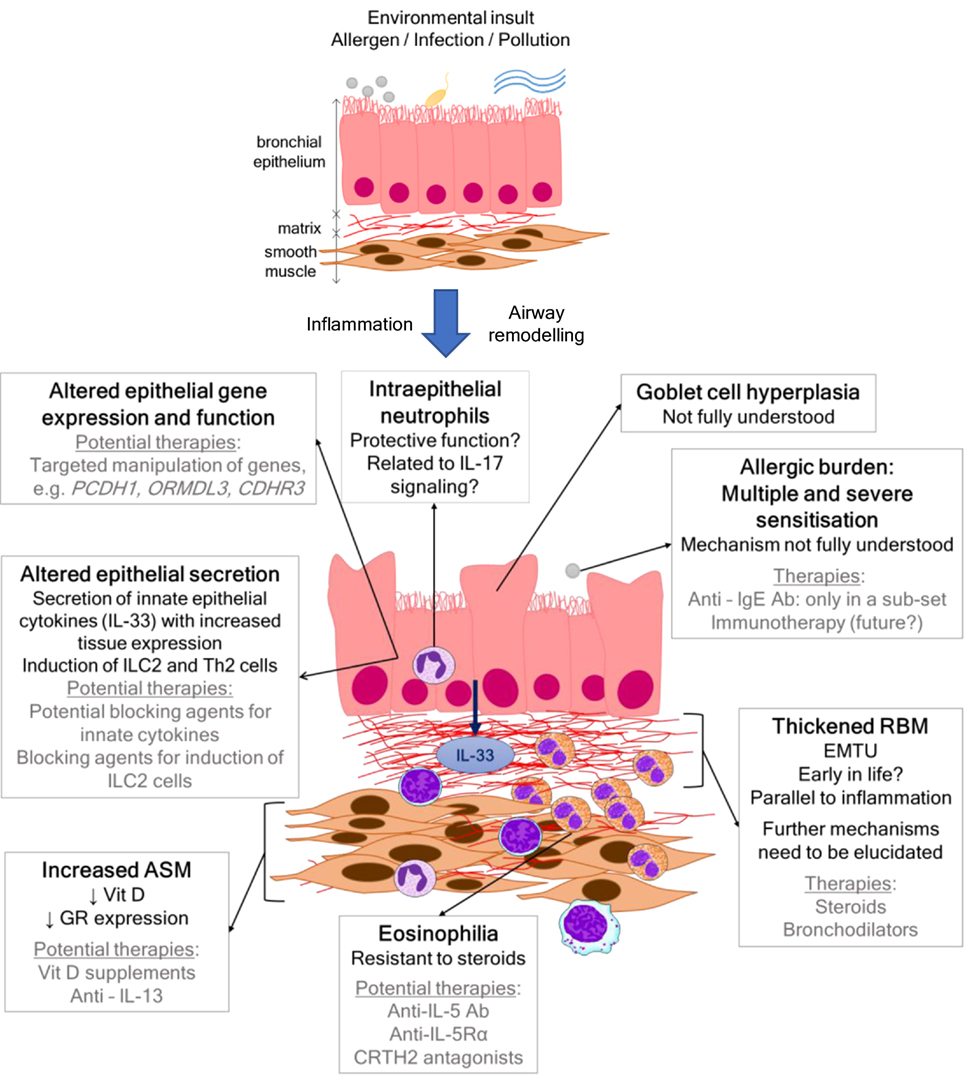
Figure 1. Scheme showing airway wall features, mechanisms, and current and/or potential therapies in pediatric severe asthma.
Diagnosis of Severe Asthma in Children
In order to accurately identify the mechanisms mediating severe pediatric asthma, it is essential that the diagnosis is correct. Objective measures supporting the key pathophysiological features of asthma including reversible airflow obstruction, airway hyperresponsiveness, chronic airway inflammation, and the presence of confirmed wheeze and breathlessness are essential in confirming the diagnosis. The ERS/ATS guidelines for the diagnosis and management of severe asthma include children (4) and must be adhered to in the assessment of these patients. In particular, the guidelines stipulate it is important that children on maximal maintenance treatment and poor control are not automatically labeled as having severe asthma. The umbrella term used to describe children who have poor control despite maximal prescribed treatment [GINA steps 4/5, or maintenance inhaled steroids ≥800 mcg daily budesonide and long-acting beta-agonists (LABAs)] is problematic severe asthma (5). Within this is a subgroup with difficult asthma, in whom, underlying modifiable factors such as poor adherence to treatment, explain persistent symptoms and poor control (6). After modifiable factors have been optimized and addressed (7), there remains a group of children with good adherence and persistent poor control, these are patients with true severe asthma (5), and will form the focus of the data discussed in this review.
Pathology of Severe Asthma in Children: Inflammatory Phenotypes
Airway Eosinophilia: Utility As a Therapeutic Target?
Studies that have included children with true severe asthma have shown that the airway pathology is characterized by luminal [bronchoalveolar lavage (BAL)] and tissue (endobronchial biopsy) eosinophilia (8). This eosinophilic airway inflammation persists despite systemic steroids in the majority of patients (9). Whether there is an association between airway eosinophil number and atopy (8) or not (10) is still uncertain. Although there is no disputing the steroid resistant eosinophilia that characterizes pediatric severe asthma (8), we have little idea of the functional impact of eosinophilia on disease manifestation. There is little clinical correlation between airway eosinophilia and symptom control or lung function. Murine experimental studies have shown that eosinophil-deficient mice have a similar phenotype to wild-type mice and, therefore, eosinophils play little role in the development of house dust mite (HDM)-induced allergic immunity (11) or airway hyperresponsiveness (12). It, therefore, appears that the eosinophilia does not contribute to altered lung function or daily symptom control. However, targeting eosinophilic inflammation using a monoclonal antibody to interleukin (IL)-5 has shown a significant reduction in exacerbations in adults with severe asthma (13). But interestingly, the subgroup that benefited most had both eosinophilic disease and frequent exacerbations (14). This suggests a specific impact of eosinophils in promoting exacerbations, but has not been confirmed mechanistically. Few studies that have targeted airway eosinophils have been undertaken in children with severe asthma. Disappointingly, one pediatric study that compared the effect of titrating maintenance inhaled steroid therapy according to sputum eosinophils or to clinical guidelines and symptoms-based management showed no benefit of the eosinophil-guided strategy in reducing exacerbations (15). This was in contrast to a prior study undertaken in adults (16). A possible explanation for the lack of effect in children may be that there is marked within patient variability in airway eosinophils over time which is independent of clinical disease status or changes in treatment (17). A systematic review of studies in children, which have used exhaled nitric oxide as a non-invasive surrogate marker for eosinophilic inflammation to guide treatment have shown some benefit in reducing exacerbations, but no effect on daily symptom control or maintenance treatment (18). The current evidence suggests that targeting airway eosinophils is most likely to be successful in children with a frequently exacerbating phenotype and may be less effective in those with persistent symptoms, and there is unlikely to be any benefit on improving lung function. To date, trials of the efficacy of monoclonal antibodies that target either IL-5 or its receptor have not been undertaken in children with severe asthma, but given the prevalence of airway eosinophilia in children, this seems an obvious avenue to pursue. Mepolizumab is an anti-IL-5 humanized monoclonal antibody that reduces circulating eosinophils. In adults and adolescents (aged 12–17 years), exacerbations decreased without improvement in FEV1 nor quality of life with Mepolizumab (13). However, only a very small number of adolescents were included. Studies specifically assessing efficacy in children with severe asthma have not yet been undertaken. However, no differences in adverse effects were observed in the adolescent group enrolled in the phase 3 trial compared to the overall population (13, 19). An ongoing clinical trial (20) studying the pharmacological properties of subcutaneous administration of Mepolizumab in children aged 6–11 years with severe eosinophilic asthma will help to determine safety in younger children. Recently, another anti-IL-5 humanized monoclonal antibody, reslizumab, has been approved by the European Medicines Agency as add-on therapy in adults with uncontrolled severe eosinophilic asthma (blood eosinophil count ≥400 cells/μl). It decreased exacerbations, improved lung function and quality life (21). It will be assessed in patients aged 12 years and older with severe eosinophilic asthma (22). Benralizumab targets the receptor for IL-5 (IL-5Rα) and in a phase III study in patients aged 12 years and above with severe uncontrolled asthma, it was well tolerated and depleted blood eosinophils, reduced exacerbation rates, and improved lung function (23). However, none of the trials that have been undertaken to assess the efficacy of blocking IL-5 in severe asthma have assessed efficacy in children alone. Therefore, at present, we do not know whether the data from the studies in adults can be extrapolated to pediatric severe disease. This is an obvious gap in our knowledge that needs to be addressed especially because of the marked pulmonary eosinophilia that characterizes pediatric severe asthma.
An important point to consider when defining biomarkers that may help to identify patients most likely to benefit from treatments that target eosinophilic inflammation is the relationship between peripheral blood eosinophils and airway eosinophils. When adherence to maintenance high-dose inhaled steroids has been optimized, and those with true severe asthma have been identified, there may be little relationship between compartments, whereby, elevated airway eosinophils may persist despite a normal blood eosinophil count (24). In adult studies, a cut-off of 0.3 × 109 cell/l (25) for blood eosinophils gave approximately 75% sensitivity and specificity for sputum eosinophilia. Therefore, there is no single peripheral or non-invasive biomarker that can be used to represent airway eosinophilia, and a composite measure is likely to be most helpful.
Dendritic Cells (DCs)
The antigen-presenting cells of the lung, DCs, capture allergens reaching the airway epithelium, process them into peptides, and load them onto the major histocompatibility complexes class II. In contrast to gut and skin, airways are immunologically immature at the time of birth (26), and DCs are not present in the airways at birth but stimuli such as microbes or pollutants can activate pattern-recognition receptors (PRRs) on epithelial cells that produce cytokines and chemokines attracting immature pre-DCs (27, 28). Activation of epithelial PRRs also results in release of cytokines, such as IL-25 (29), IL-33 (30), or thymic stromal lymphopoietin (TSLP) (31), and danger signals, such as uric acid (32), which further activate DCs. Activated DCs migrate to draining lymph nodes where along with costimulatory molecules will bind and activate T cell receptors (TCRs) on the surface of naïve CD4 T cells (33).
Two subpopulations of DCs have been identified: myeloid or conventional DCs (cDCs) or DC1 (CD11c+ CD123dim+ in cytometric analysis) and lymphoid or plasmacytoid DCs (pDCs) or DC2 (CD11c− CD123high+) (34). Apart from the cDCs, mouse studies have reported that a different subset of DCs, monocyte-derived DCs, orchestrate the pro-inflammatory environment in the airways by secreting chemokines that attract inflammatory cells during allergen challenge (35). Both mDC and pDCs take up inhaled allergen and present it to T cells in animal models of allergic airways disease (36). Depletion of pDCs during allergen challenge resulted in allergic airways disease whereas adoptive transfer of pDCs before sensitization prevented disease, suggesting a protective role of pDCs that could be applied in clinic (36).
Activated DCs can form tight junctions with the airway epithelium and detect inhaled allergens without disturbing the epithelial barrier (37) and have upregulated chemokine receptors and costimulatory molecules so they have more capacity to migrate to the lymph nodes and stimulate naïve T cells (38). In a study including 50 atopic children and 40 healthy controls, serum OX40L levels were higher in children experiencing acute severe asthma exacerbations and during stable severe persistent asthma compared to mild/moderate exacerbations and mild or moderate persistent asthma, respectively, and this correlated positively with blood eosinophil counts (39). It has been reported that sputum from asthmatic children treated with inhaled steroids contain increased airway DCs with reduced expression of the costimulator CD86, suggesting that either asthma or steroid therapy may impair DC trafficking and/or maturation reducing the pro-inflammatory responses (40). Flow cytometry analysis of DCs in cord blood of neonates from allergic and non-allergic parents and in peripheral blood of allergic and healthy children has allowed the identification of a new DC population CD11c− CD123dim+ named “less differentiated” DCs (ldDCs). This population was the predominant DC population in cord blood and decreased with age. It was also increased in children with atopic dermatitis whereas was decreased in asthmatics receiving high-dose inhaled corticosteroids. So it was proposed that ldDC could be involved in the severity of allergy/asthma. No differences in DC populations were found in cord blood from neonates with low versus high risk for allergic disorders (41). In contrast, blood pDCs were increased in both atopic and non-atopic asthmatic adults (42).
Although targeting DCs may be an attractive approach in the treatment of asthma, there are currently no specific therapies, either from experimental studies, or being tested in clinical trials that target DC numbers or function. It is still necessary to understand the complex cellular and molecular pathways involved in altering pDC function in pediatric asthma before therapeutic applications can be considered.
Airway Epithelium: Interactions Between Innate and Adaptive Immunity in Pediatric Severe Asthma
The airway epithelium is the first site of contact between the host and environment. Allergens, viruses, and other environmental exposures directly stimulate and interact with the epithelium. It has a role not only as a physical barrier but also contributes to the development of the immune response and maintenance of inflammation. Therefore, it is not surprising that the airway epithelial barrier is altered in asthma (33, 43). In recent years, genetic studies of bronchial epithelial cells have discovered several genes, such as protocadherin 1 (PCDH1) (44), periostin (POSTN), serpin family B member 2 (SERPINB2), and chloride channel accessory 1 (CLCA1) (45), associated with asthma phenotypes. Of these, PCDH1 was very specifically associated with childhood asthma (46). Consequently, it has been suggested that many of the different pathological mechanisms underlying asthma phenotypes may originate in the airway epithelium (47).
Allergens, microorganisms, and allergen-derived protease activities not only activate DCs but also airway epithelial cells through the activation of toll-like receptors, which leads to secretion of cytokines and danger signals. These signals can be propagated through the dysregulation of the epithelial–mesenchymal trophic unit (EMTU), which is the bidirectional interaction between epithelium and mesenchyme involving the release of growth factors and cytokines, resulting in the amplification of inflammation and structural changes (remodeling) (43, 48). It is thought that the drivers of remodeling may be recruited CD34+ fibrocytes located at areas of collagen deposition and in BAL acting as myofibroblasts (49, 50), but it has also been proposed that they could stimulate the differentiation of resident mesenchymal cells (50). Epithelial cells can also transdifferentiate into fibroblasts/myofibroblasts by epithelial–mesenchymal transition (51), but this has not been proven in asthma (52).
Innate Epithelial Cytokines and Type 2 Lymphoid Cells in Pediatric Severe Asthma
Innate immunity is being increasingly recognized as being an equal contributor to asthma pathogenesis as adaptive immunity. Upon exposure to environmental stimuli (allergens, infection, and pollution), the activated epithelium releases cytokines, such as IL-25, IL-33, or TSLP and danger signals, such as uric acid, which contribute to the onset of innate immune mechanisms resulting in disease initiation and propagation. DCs (as discussed above), mast cells (MCs) (53), type 2 innate lymphoid cells (ILC2) (54), and basophils (55) are all induced by the release of the innate epithelial cytokines. Consequently, targeting IL-25, IL-33, and TSLP is an interesting therapeutic approach for severe asthma and is actively being pursued.
Specifically, in pediatric severe asthma, we have shown increased expression of the innate epithelial cytokine IL-33 in the bronchial tissue and an association with increased levels and both airway remodeling and steroid resistance (56). More recently, we have shown that a specific sub-phenotype of patients with severe asthma and fungal sensitization have even higher levels of IL-33 in both BAL and biopsy (57). It is now also apparent that the downstream effector cells that are induced by IL-33, ILC2 cells are increased in the airways of children with severe asthma compared to non-asthmatic controls (58). Interestingly, a specific association between type 2 ILCs and severe asthma has also been demonstrated in adults (59). The ILCs present in BAL from pediatric patients were characterized by lineage negative markers (absence of the T cell antigens) and presence of the type 2 receptor CRTH2. In contrast to Th2 cells, they were a rare cell population, making up only 0.2% of lymphoid cells. Of note, however, both cell types did express CRTH2. Although increased numbers of both ILC2s and Th2 cells have been demonstrated in pediatric severe asthma, their functional and clinical relevance remains unknown, since there were no clear correlations between cell numbers in BAL and symptoms or lung function (58).
Asthma has been typically considered a Th2 disorder since the predominant inflammatory phenotype is eosinophilic as observed in BAL and endobronchial biopsies from children with severe disease (8). Although studies have investigated T lymphocytes in peripheral blood from children, data relating to the airway inflammatory phenotype have been lacking. We have recently shown that children with severe asthma have increased numbers of CD4+ T cells in BAL compared to non-asthmatic controls, and that these cells make up approximately 40% of all airway CD3+ lymphocytes and express the CRTH2 receptor (58). Interestingly, when we had previously quantified CD4+ cells in endobronchial biopsy, numbers were not significantly different to non-asthmatic controls (8), suggesting that there may be differences in the luminal and tissue compartments.
Mechanistically, naïve T cells in draining lymph nodes differentiate to Th2 cells via IL-4-mediated activation of STAT6 and GATA3. Th2 cells migrate to the airway mucosa and secrete the Th2 cytokines IL4, IL-5, and IL-13. But, detection of Th2 cytokines in severe asthmatic children remains controversial (3, 8). It has been generally accepted that IL-5 mediates the recruitment of eosinophils by the expression of epithelium-derived chemokines named eotaxins [CC-chemokine ligand 11, CCL24, and CCL26]. It also promotes bone marrow development and mobilization of eosinophil precursors. Children with STRA have airway remodeling and eosinophilic inflammation, but in the absence of detectable levels of Th2 cytokines, without neutrophilia nor MC infiltration (8).
Mast Cells
Cross-linking of FcεRI following MC exposure to allergen can result in MC activation, which is characterized by degranulation and production/secretion of preformed histamine, lipid mediators, enzymes (proteases, hydrolases, cathepsin G, and carboxypeptidase), and cytokines (including tumor necrosis factor, IL-4, IL-5, IL-6, IL-13, 3 CCL3, IL-33, and granulocyte-macrophage colony-stimulating factor) (60). The arachidonic acid-derived mediators are prostaglandin (PG)D2, leukotriene (LT) C4, and platelet-activating factor, which can induce bronchoconstriction, mucus secretion, and edema (61). The wide repertoire of cytokines has several effects ranging from IgE synthesis to neutrophil and eosinophil activation to fibroblast growth. Similarly, the secreted enzymes have wide effects, such as degradation of allergens, enhanced airway smooth muscle (ASM) contractility, and enhanced IL-33 activity (60). But the role of MCs in asthma is not based solely on its products but also on their strategic location. Whereas in healthy airways MCs are predominantly located near blood vessels and within the lamina propria (62), MCs tend to relocate to the airway epithelium (63), submucosa (64), submucosal glands (65), and ASM (66) in asthma. However, these data are all from adult studies. The contribution of MCs to pediatric asthma is less well known. Several studies have reported that MC frequency was similar in the subepithelium (8, 10, 67, 68) and in the ASM (8, 69) between wheezing or asthmatic children and controls. In a recent study in biopsies of severe asthmatic children, it was reported that ASM MC numbers were associated with the number of severe exacerbations and eosinophilia, but not with remodeling or lung function (70).
Despite the apparent importance of MCs in the pathology of severe asthma, to date, therapies that are MC stabilizers such as cromolyn sodium and nedocromil that inhibit MC degranulation have proven very disappointing in the clinic. MC predominance in the airway submucosa and epithelium has been associated with severe asthma in adults because of increases in PGD2 levels, which is produced mainly by MCs but also Th2 cells, macrophages, and eosinophils (64). PGD2 binds to smooth muscle cells leading to vasodilatation and bronchoconstriction and can also bind the chemoattractant receptor-homologous molecule expressed on TH2 lymphocytes (CRTH2) inducing Th2 cytokine production and further promoting activation of MCs and PGD2 production in asthma (64).
PGD2 Receptor 2 (CRTH2) Antagonists in Severe Asthma: A Novel Therapeutic Approach
A recent novel class of drug that is undergoing phase II studies in adults and seems attractive for pediatric severe asthma is the CRTH2 antagonists (71). CRTH2 is present on MCs, but also on eosinophils, Th2 cells, and ILC2. Given the overwhelming evidence that pediatric severe asthma is associated with severe atopy, is eosinophilic, and associated with significantly increased numbers airway of ILC2, the strategy of blocking the CRTH2 receptor is very appealing. A randomized, parallel double blind placebo-controlled trial of a CRTH2 antagonist in adults with persistent, moderate-to-severe asthma, and an elevated sputum eosinophil count showed a reduction in sputum eosinophils in the active group, and no associated significant adverse effects (72). Another potential advantage of these compounds is that they can be administered orally. However, clinical efficacy is yet to be proven. Small phase II trials have suggested efficacy in achieving an improvement in symptoms and lung function (73), but they did not target the population that had eosinophilic or type 2 high diseases. It is increasingly apparent that as more and more add-on therapies become available and may potentially be utilized in children, the need to identify the right drug for the right patient phenotype will be essential (74).
Regulatory T Cells
After TCR engagement, activation of T cells can be suppressed by regulatory CD4+CD25+ T cells (Treg) (75). Therefore, Treg cells can control allergen-specific immune responses and low numbers or dysfunctional Treg cells may contribute to allergic disease and asthma. However, few studies have investigated the role of Treg cells in pediatric severe asthma. Low Treg cells in blood and sputum as well as impaired suppressive function during exacerbations have been reported in severe refractory asthmatic adults compared to healthy controls (76). In contrast, another small study comparing numbers of Treg cells in BAL from moderate to severe asthmatic adults compared to mild asthmatics reported that Treg cells were increased in the severe group (77). In asthmatic children, Treg cells were lower in BAL (78) and blood (79). Peripheral Treg cell levels were lower compared to healthy controls, especially in the acute phase and in the severe group. Th1/Th2 ratio correlated positively with Treg cells and negatively with disease severity (79).
Treg cells can act through perforin-mediated cytolysis as well as IL-10 and TGF-β. IL-10 is a potent anti-inflammatory cytokine expressed by several cell types, including T cell subpopulations. IL-10 suppresses the production of inflammatory cytokines, the DC-mediated antigen presentation to T cells as well as the function of MCs and eosinophils (80). In addition, IL-10 inhibits IgE and favors IgG4 to IgE (81). Lower levels of IL-10 are produced by macrophages and mononuclear cells from asthmatics (82, 83). Defective IL-10 expression has been associated with increased steroid resistance in children with severe asthma (84), and vitamin D enhances the frequency of both IL-10+ and Foxp3+ Treg cells in children with severe asthma (85). In a translational setting, these data suggest that vitamin D supplementation may be effective in enhancing the frequency of Treg cells in pediatric severe asthma.
Other studies have suggested that TGF-β, rather than IL-10, may be more important and serve as a biomarker of asthma control in atopic asthma (86). TGF-β is a pleiotropic cytokine with numerous functions that are vital in maintenance of pulmonary homeostasis, such as inhibiting Th2 and Th1 cell responses or inhibiting IgE production (87). In children, polymorphisms in TGF-β2 have been associated with atopic asthma (88). PCR analysis of bronchial and nasal epithelial cells concluded that TGF-β2 was differentially expressed in pediatric asthmatics compared to atopic non-asthmatics and healthy children (89). However, a much better understanding of the complex TGF-β signaling network in pediatric severe asthma is required before specific molecules can be targeted in a valid clinical study.
Airway Remodeling: Mechanisms and Therapeutic Targets
Children with severe asthma have evidence of all of the structural airway wall changes (remodeling) that are apparent in adults. They have increased thickness of the reticular basement membrane (RBM) (8, 90), increased ASM (8, 91, 92), goblet cell and submucosal gland hyperplasia (93), and evidence of angiogenesis (67). Of these changes, increased bronchial ASM has been closely related to worse lung function and greater bronchodilator reversibility (91, 92, 94).
Relationships between Inflammation and Remodeling
It is often proposed that remodeling occurs as a consequence of chronic airway inflammation. Payne et al. (90) compared RBM thickness in 19 children with difficult asthma prescribed high-dose inhaled steroids (6–16 years) and 10 age-matched non-asthmatics children with healthy, steroid-naive asthmatic adults, and life-threatening asthmatic adults. RBM thickness was not associated with severity, asthma symptoms, age, or airway inflammation. Fedorov et al. (95) compared bronchial biopsies between non-asthmatic, moderate, and severe asthmatic children (5–15 years) and showed excess deposition of interstitial collagen in the RBM occurred early in life but did not correlate with submucosal eosinophils and suggested that RBM thickness is established early in life due to an abnormal EMTU. Both studies proposed that remodeling is dissociated from eosinophilic inflammation. The dissociation between airway remodeling and eosinophilic inflammation has been demonstrated in a mouse model in which HDM-induced airways remodeling was equivocal in eosinophil-deficient and wild-type mice (96). Mechanistic data from a neonatal mouse model of inhaled HDM exposure have shown that remodeling is unlikely a consequence of inflammation, but that both processes occur in parallel (97). Remodeling can, therefore, develop in the absence of an inflamed airway with just excessive bronchoconstriction (98), and thus, there is an urgent need for therapies that can target structural changes alone, as many children with severe asthma remain symptomatic with significant airway hyperresponsiveness in the absence of inflammation (99).
Airway Smooth Muscle
The importance of targeting ASM remodeling as a therapeutic approach is made apparent by the very consistent association with increased ASM and worse lung function in both adult and pediatric studies. Increasing ASM has also been associated with lower serum vitamin D levels and worse asthma control in children (94). Mechanistically, a relationship between increased airway remodeling and a vitamin D-deficient diet has also been shown in a neonatal mouse model of HDM-induced allergic airways disease (100). These data suggest studies that focus on investigating ASM function in pediatric severe asthma are likely to be helpful in discovering novel therapeutic targets. In addition, that vitamin D supplementation to achieve normal serum levels in children with severe asthma is an important consideration as it may minimize remodeling.
There is evidence that ASM function is specifically impaired in adult severe asthma, and that the mechanism is related to glucocorticoid resistance, whereby glucocorticoid receptor expression is reduced with impaired nuclear translocation (101). In contrast to ASM, few functional consequences have been reported in association with the thickness of the subepithelial RBM. Moreover, increased thickness is not an isolated finding in asthma, although the degree of thickening is greater in severe asthma, this feature may also be present in children with cystic fibrosis (102) and adults with COPD (103). Thus, it is difficult to know the impact that therapies, which target increased RBM thickness may have on disease manifestation.
Importance of Allergy in Pediatric Severe Asthma: Mechanisms and Anti-IgE Antibody Therapy
More than 85% of children with severe asthma are atopic, defined by serum IgE antibodies and a positive skin prick test to common aeroallergens (8). One of the key clinical features that allows distinction between children with difficult asthma (poor control with poor adherence) and severe therapy resistant asthma (poor control despite good adherence) is significantly more severe asthmatics were polysensitised to several allergens, and more patients had food allergy (104). However, perhaps the most important distinctive feature of severe asthma is when atopy is quantified, rather than assessed as simply being present or not (105, 106). Children with severe asthma have a much worse and higher allergic burden (107). This suggests allergic sensitization plays a critical role in the pathogenesis of severe asthma in children (108). The role of allergy in pediatric severe asthma needs to be understood to help identify underlying mechanisms of disease progression, which will impact both on the choice of add-on therapies for these patients, but also on the discovery of novel therapeutics. In this regard, the one therapy that has been approved for use in children with severe asthma is the recombinant DNA-derived humanized monoclonal antibody against IgE (omalizumab), which works by reducing the quantity of cell-bound IgE, downregulation of high-affinity IgE receptors FcεRI on MCs, basophils, and DCs, and prevention of mediator release from effector cells (109, 110). Decreased sputum and bronchial eosinophils, as well as T cells were observed in adult bronchial biopsies after omalizumab (109). In a study (111) involving 334 children aged 6–12 years with moderate-to-severe atopic asthma treated with beclomethasone dipropionate and omalizumab or placebo, omalizumab reduced number of exacerbations as well as the frequency of exacerbations when withdrawing ICS. Another smaller 16-week study in children with severe asthma reported that omalizumab allowed a significant reduction in daily prednisolone dose and improved control and life quality (112).
However, the current licensed indication requires serum IgE levels to be within a set range, the maximum being 1,500 IU/ml. At least one-third of children with severe asthma have an IgE greater than 1,500 IU/ml because of severe and multiple allergies (8). In addition, approximately one-third of children who are eligible and are given a trial of treatment do not have a clinical response (113, 114). Therefore, there is still a subgroup of children with very severe disease and marked morbidity for whom currently no licensed add-on therapies are available. Interestingly, given the burden of monthly, or two weekly injections posed by omalizumab, and that specific subgroups, adolescents in particular, who are at high risk of asthma death, but more likely to be non-compliant with maintenance therapy, an approach of giving omalizumab prior to the Autumn increase in asthma exacerbations has been efficacious (115). Omalizumab therapy was associated with improved IFN-α responses to rhinovirus. However, changes in allergen-stimulated cytokine responses in peripheral blood T cells, or changes in T regulatory cells were not seen (116). Given the clinical benefit, this suggests the effects of omalizumab are unlikely via an impact on T cell responses, and more likely via other immune effector cell types such as MCs (116).
Allergy in Preschool Wheeze: A Possible Window for Asthma Prevention?
Preschool children with wheezing disorders may or may not progress to develop asthma. Airway inflammation can be assessed in BAL and endobronchial biopsies from children with severe wheezing. BAL from wheezing children contains increased lymphocytes, polymorphonuclear cells, and macrophages/monocytes as well as LT B4, C4, PG E2, and the potentially epithelial-derived 15-hydroxyeicosatetraenoic acid were all increased (117). Bronchial biopsy studies in infants under 2 years with severe wheeze have reported an absence of RBM thickening and eosinophilic inflammation (118). However, when older children at a median age of 3 years with severe recurrent wheezing were compared to non-wheezing controls, they had increased airway eosinophils and RBM thickness (119). Birth cohort studies have repeatedly shown that the most prominent risk factor for progression of preschool wheeze to asthma is early allergen sensitization (120). Also, in a similar manner to older children with severe asthma, the risk of developing asthma and the greatest reduction in lung function is in those preschool wheezers who have both early and multiple allergic sensitization (121, 122). Unfortunately, targeting eosinophils with early inhaled steroids is not disease modifying (123), and this is explained by the mechanistic data that shows an absence of eosinophils does not impact the phenotype of allergic airways disease (96). However, given the definite association between early allergic sensitization and progression of preschool wheeze to asthma, several alternative interventions have been proposed to achieve disease prevention (124). One of these, to investigate the role of omalizumab in preschool wheeze to achieve disease modification is currently being tested in a clinical trial (125).
Emerging Therapies for Severe Asthma
According to the ATS/ERS guidelines, severe asthmatics have poor control despite treatment with high-dose inhaled or oral corticosteroids combined with LABAs. A summary of the emerging add-on therapies that are being trialled for severe asthma is provided below. However, it is important to remember that, of these, only omalizumab is currently licensed for use in children with severe asthma.
Muscarinic Antagonists
These drugs act as bronchodilators by non-specifically antagonizing the muscarinic acetylcholine receptor and inhibiting smooth muscle cell contraction and mucus secretion. Short-acting muscarinic antagonists (SAMAs), such as ipratropium bromide, can be used in severe asthmatic children and adults during asthma exacerbations (126) and to reduce β-agonist doses in order to avoid side effects, but they are less effective than inhaled beta-agonists (127). In 1998, Qureshi et al. already reported that adding this drug to a combined therapy of albuterol and corticosteroids decreased hospitalizations for severe asthmatic children (aged 2–18 years). More recently, a meta-analysis reported that the combination of SAMAs and SABAs in children during exacerbation improves lung function and reduces the risk of tremor and the risk of admission (128).
Longer-acting muscarinic antagonists are an interesting option as controller medications. Inhaled Tiotropium (Spiriva Respimat®) was first indicated in COPD treatment. Currently, it is approved as an add-on maintenance bronchodilator in adults with asthma taking ICS/LABAs and who have experienced at least one severe exacerbation in the previous year. It was shown to improve lung function and symptoms in uncontrolled moderate-to-severe asthmatic adults and reduced the risk of exacerbations in those treated with ICS/LABAs (129, 130). US FDA has recently approved it as an asthma maintenance treatment in children aged 6 years and over (131). However, it has not been approved in Europe. Its efficacy as an add-on therapeutic in pediatric severe asthma remains unknown. Pediatric trials that will allow selection of patients that are most likely to benefit are needed.
Immunomodulators
Molecular-based therapies, which allow treatment according to the predominant inflammatory phenotype, are of particular interest in severe asthmatic children who do not respond to standard therapy. Apart from specificity, they provide long-term control and allow a reduction in ICS and oral steroid dose. An important aim in the utility of novel therapeutics for children is not just as add-on treatments that will allow disease control, but also as steroid sparing therapies to minimize the significant adverse effects of high-dose corticosteroids. Several antibody-based treatments are available.
Neutralizing IgE: Omalizumab
The mechanism of action and specific utility of omalizumab in pediatric severe asthma has been discussed in the section on allergy in children above.
In the UK, omalizumab is indicated as an add-on therapy to improve control in adults and children aged 6 years and over with severe persistent confirmed IgE-mediated asthma, and who need continuous or frequent treatment with oral corticosteroids (defined as four or more courses in the previous year) (132). The predominant benefit is a reduction in exacerbations. However, limitations for children include the upper limit of serum IgE for which it can be prescribed and at least one-third do not have a clinical response.
Blocking IL-5 Signaling: Mepolizumab
Mepolizumab is an anti-IL-5 humanized monoclonal antibody that reduces circulating eosinophils. It is indicated as an add-on to standard therapy in severe refractory eosinophilic asthma in adults when the blood eosinophil count is ≥300 cells/μl in the previous 12 months and ≥4 exacerbations needing systemic corticosteroids in the previous 12 months need for continuous oral corticosteroids equivalent of more of prednisolone 5 mg/day over the previous 6 months (133). Exacerbations in adults decreased without improvement in FEV1 nor quality of life. The data for efficacy in children are currently lacking, and clinical trials that address this unmet need are urgently needed.
Blocking Th2 (IL-13 and IL-4) Signaling: Lebrikizumab, Dupilumab, and Pitrakinra
Lebrikrizumab, a humanized monoclonal antibody against IL-13, has been evaluated for severe asthmatic adults. Initial studies showed Lebrikizumab treatment resulted in improvement in lung function in adults with uncontrolled asthma taking ICS, especially in those with higher levels of serum POSTN. There was also a decrease in exacerbation rates in those patients with higher blood eosinophils and IgE levels (134). However, subsequent confirmatory studies in adults have been disappointing (135) and it is not yet indicated for adult severe asthma.
Animal studies have shown that IL-4 can induce IL-13-independent AHR and goblet cell hyperplasia, suggesting dual inhibition of both IL-4 and IL-13 could suppress these events (136). Dupilumab is a human monoclonal antibody against the IL-4 receptor α chain (IL-4Rα) blocking downstream signaling via both the IL-4 and IL-13 receptors. In 2013, Wenzel and colleagues performed a 12-week study (phase 2a) to asses Dupilumab in persistent moderate-to-severe asthmatic adults with high blood (≥300/μl) or sputum (≥3%) eosinophils (Th2 high disease) (137). Dupilumab was effective in reducing exacerbations, increasing lung function and reduced Th2-associated inflammation, as well as allowing a reduction and/or stopping of maintenance dose of ICS while maintaining improvement of asthma. More recently, in a 24-week study (phase 2b) in uncontrolled persistent asthmatic adults, injection with Dupilumab every 2 weeks as an add-on therapy to medium-to-high-dose ICS and LABAs led to improvement of FEV1, reduction in exacerbations, and better asthma control regardless of baseline eosinophil count (138). During the treatment patients with at least 300 eosinophils/μl at baseline had an increase in blood eosinophils, so the next clinical trial (139) excluded patients with high eosinophils and also included children (from 12 years). Efficacy of this antibody in uncontrolled persistent asthmatic children (aged from 6 to 12 years) will be assessed in a clinical trial starting on 2017 (139). Pitrakinra is a recombinant human IL-4 antibody containing mutations that allow it to prevent the assembly of IL-4Rα with either IL-2Rγ or IL-13Rα (140). In a recent study, a dose–response for asthma exacerbations was identified in a specific subgroup according to the SNPs genotype in IL4RA gene (141).
Blocking Th17 Signaling: The Role of IL-17 and Neutrophils in Pediatric Severe Asthma
In adults with severe asthma, high levels of IL-17A have been reported in sputum, BAL fluid, and peripheral blood (142–144), and this was associated with increased disease severity (145). In children, IL-17A has been reported to induce neutrophilic airway inflammation and promote steroid resistance (146). Levels of IL-17A in sputum, nasal wash, and plasma as well as levels of circulatory T cells expressing IL-17 were studied in children with moderate asthma, and it was suggested that IL-17 could be associated with asthma severity (147). In contrast to the data from studies in adults, we have shown levels of IL-17A are not elevated in either the BAL or endobronchial biopsies of children with severe asthma compared to non-asthmatic controls (148). However, we did show increased expression of IL-17Rα in the airway submucosa and epithelium of children with severe asthma. Furthermore, numbers of neutrophils were also similar in the BAL and submucosa of children with severe asthma and controls (8, 148). However, there was a subgroup of patients with increased numbers of neutrophils only within the epithelium, and these patients had better symptom control improved lung function, symptom control, and were prescribed lower dose maintenance inhaled steroids. Therefore, unlike adult severe asthma, neutrophils might be beneficial in pediatric severe asthma pathophysiology. Therefore, the role of neutrophils and IL-17 is not completely understood in children with STRA and requires further investigation before therapies that are either antineutrophilic or block IL-17 are tested. Interestingly, Brodalumab, a human anti-IL-17 receptor monoclonal antibody, has shown no benefit in adults with moderate-to-severe disease (149).
Anti-TSLP Antibody: AMG157
The monoclonal antibody AMG157 blocks the binding of TSLP with its receptor and has been shown to reduce bronchoconstriction and attenuate the early and late phase response in allergic asthmatic adults (150). It also reduced blood and sputum eosinophil counts and FeNO before allergen challenge, suggesting that TSLP may be an important upstream regulator of type 2 inflammation in the airways. The efficacy of AMG157 is currently being assessed in adolescents (12–17 years old) with mild-to-moderate asthma (151) and will be assessed in uncontrolled severe asthmatic adults (152). The efficacy of blocking TSLP in children with severe asthma remains to be seen. There is no evidence to date that levels of TSLP are elevated in the airways of children; therefore, a direct extrapolation of findings from adult studies to pediatric studies may not be beneficial.
Anti-PGD2
Prostaglandin D2 is a lipid inflammatory mediator produced by cyclooxygenases and PGD2 synthases mainly in MCs, but also in Th2 cells, macrophages, and much less in eosinophils and basophils. They subsequently bind to D prostanoid 1 and chemoattractant receptor-homologous molecule expressed on Th2 cells (CRTH2) receptors triggering anti-inflammatory effects as well as pro-inflammatory effects through PGD2-CRTH2 pathway, which is upregulated in asthma (153). This is a particularly attractive therapeutic for children and has been discussed in detail above in the section on airway inflammation in pediatric severe asthma.
Future Directions
We have discussed two add-on treatments that are currently available for use in adults that mechanistically are attractive therapeutic options for children with severe asthma and need to be pursued. Monoclonal antibodies to IL-5 or its receptor, and CRTH2 antagonists, both of which may help to ameliorate the persistent steroid resistant eosinophilia and elevated Th2 and ILC2 cells that are apparent. Although both types of interventions should be efficacious, we know that omalizumab, the currently licensed add-on treatment for children, does not work or cannot be used in about 50%, so similarly, it is likely that the other two therapeutics will also only work in a subgroup. In contrast to adult studies, given the current mechanistic data from children, it seems much less likely that antibodies that target IL-13, IL-4, or IL-17 will be beneficial in children. This is because these data showing elevated levels of these mediators in pediatric airways are scarce. A molecular target for which therapeutic agents are not currently available, but would certainly be worth pursuing for children, is IL-33. This is because it is elevated in children, is relatively steroid resistant, promotes airway remodeling (56), and is more specifically associated with the sub-phenotype of severe asthma with fungal sensitization (57).
It is essential to remember that pediatric severe asthma is markedly heterogeneous, and in contrast to adult disease, our current understanding of the underlying sub-phenotypes and endotypes is very limited. This is because it is a rare disease, but also because mechanistic studies that use either age appropriate experimental models or airway samples from children are a challenge to undertake. We know that there are distinctions between the factors that drive pediatric and adult disease, most importantly, pediatric disease is present in the context of a maturing immune system and during lung growth and development. Given the acknowledged heterogeneity and the relatively small number of patients that are affected, it is essential that we now undertake multicenter, national and if possible, international unified studies to assess the efficacy of novel therapeutics and to investigate the mechanisms of action of these drugs in children. Only such an approach will allow us to understand the mechanisms mediating disease, and to identify important endotypes, which will allow us to stratify patients and ensure add-on treatments are accurately targeted to achieve effective personalized medicine.
Author Contributions
AM and SS contributed equally to this work.
Conflict of Interest Statement
The authors declare that the research was conducted in the absence of any commercial or financial relationships that could be construed as a potential conflict of interest.
Funding
SS is an NIHR Career Development Fellow (grant CDF-07-019) and is funded by Asthma UK (Innovation Grant) AUK-IG-2016-339.
References
1. Lang A, Carlsen KH, Haaland G, Devulapalli CS, Munthe-Kaas M, Mowinckel P, et al. Severe asthma in childhood: assessed in 10 year olds in a birth cohort study. Allergy (2008) 63:1054–60. doi:10.1111/j.1398-9995.2008.01672.x
2. Ellis D, Walker S. Severe Asthma: The Unmet Need and the Global Challenge. (2017). 23 p. Available from: https://www.asthma.org.uk/globalassets/get-involved/external-affairs-campaigns/publications/severe-asthma-report/auk_severeasthma_2017.pdf
3. Fitzpatrick AM, Higgins M, Holguin F, Brown LAS, Teague WG; National Institutes of Health/National Heart, Lung, and Blood Institute’s Severe Asthma Research Program. The molecular phenotype of severe asthma in children. J Allergy Clin Immunol (2010) 125:851–7.e18. doi:10.1016/j.jaci.2010.01.048
4. Chung KF, Wenzel SE, Brozek JL, Bush A, Castro M, Sterk PJ, et al. International ERS/ATS guidelines on definition, evaluation and treatment of severe asthma. Eur Respir J (2014) 43:343–73. doi:10.1183/09031936.00202013
5. Bush A, Saglani S. Management of severe asthma in children. Lancet (2010) 376:814–25. doi:10.1016/S0140-6736(10)61054-9
6. Saglani S, Fleming L. How to manage a child with difficult asthma? Expert Rev Respir Med (2016) 10:873–9. doi:10.1080/17476348.2016.1191355
7. Cook J, Beresford F, Fainardi V, Hall P, Housley G, Jamalzadeh A, et al. Managing the pediatric patient with refractory asthma: a multidisciplinary approach. J Asthma Allergy (2017) 10:123–30. doi:10.2147/JAA.S129159
8. Bossley CJ, Fleming L, Gupta A, Regamey N, Frith J, Oates T, et al. Pediatric severe asthma is characterized by eosinophilia and remodeling without T(H)2 cytokines. J Allergy Clin Immunol (2012) 129:974–82.e13. doi:10.1016/j.jaci.2012.01.059
9. Bossley CJ, Fleming L, Ullmann N, Gupta A, Adams A, Nagakumar P, et al. Assessment of corticosteroid response in pediatric patients with severe asthma by using a multidomain approach. J Allergy Clin Immunol (2016) 138:413–20.e6. doi:10.1016/j.jaci.2015.12.1347
10. Turato G, Barbato A, Baraldo S, Zanin ME, Bazzan E, Lokar-Oliani K, et al. Nonatopic children with multitrigger wheezing have airway pathology comparable to atopic asthma. Am J Respir Crit Care Med (2008) 178:476–82. doi:10.1164/rccm.200712-1818OC
11. Fattouh R, Al-Garawi A, Fattouh M, Arias K, Walker TD, Goncharova S, et al. Eosinophils are dispensable for allergic remodeling and immunity in a model of house dust mite-induced airway disease. Am J Respir Crit Care Med (2011) 183:179–88. doi:10.1164/rccm.200905-0736OC
12. Pauwels RA, Brusselle GJ, Kips JC. Cytokine manipulation in animal models of asthma. Am J Respir Crit Care Med (1997) 156:S78–81. doi:10.1164/ajrccm.156.4.12-tac-1
13. Pavord ID, Korn S, Howarth P, Bleecker ER, Buhl R, Keene ON, et al. Mepolizumab for severe eosinophilic asthma (DREAM): a multicentre, double-blind, placebo-controlled trial. Lancet (2012) 380:651–9. doi:10.1016/S0140-6736(12)60988-X
14. Oberle AJ, Mathur P. Precision medicine in asthma. Curr Opin Pulm Med (2017) 23:254–60. doi:10.1097/MCP.0000000000000372
15. Fleming L, Wilson N, Regamey N, Bush A. Use of sputum eosinophil counts to guide management in children with severe asthma. Thorax (2012) 67:193–8. doi:10.1136/thx.2010.156836
16. Green RH, Brightling CE, McKenna S, Hargadon B, Parker D, Bradding P, et al. Asthma exacerbations and sputum eosinophil counts: a randomised controlled trial. Lancet (2002) 360:1715–21. doi:10.1016/S0140-6736(02)11679-5
17. Fleming L, Tsartsali L, Wilson N, Regamey N, Bush A. Sputum inflammatory phenotypes are not stable in children with asthma. Thorax (2012) 67:675–81. doi:10.1136/thoraxjnl-2011-201064
18. Petsky HL, Kew KM, Chang AB. Exhaled nitric oxide levels to guide treatment for children with asthma. Cochrane Database Syst Rev (2016) 11:CD011439. doi:10.1002/14651858.CD011439.pub2
19. GlaxoSmithKline. Nucala, Highlights of Prescribing Information. (2017). Available from: http://www.accessdata.fda.gov/drugsatfda_docs/label/2012/202155s000lbl.pdf
20. GlaxoSmithKline. Pharmacokinetics and Pharmacodynamics of Mepolizumab Administered Subcutaneously in Children. (2017). Available from: https://clinicaltrials.gov/ct2/show/NCT02377427
21. Castro M, Zangrilli J, Wechsler ME, Bateman ED, Brusselle GG, Bardin P, et al. Reslizumab for inadequately controlled asthma with elevated blood eosinophil counts: results from two multicentre, parallel, double-blind, randomised, placebo-controlled, phase 3 trials. Lancet Respir Med (2015) 3:355–66. doi:10.1016/S2213-2600(15)00042-9
22. Teva Branded Pharmaceutical Products R&D Inc. A Study of Reslizumab in Patients 12 Years of Age and Older with Severe Eosinophilic Asthma. (2017). Available from: https://clinicaltrials.gov/ct2/show/NCT03052725?term=NCT03052725&rank=1
23. Fitzgerald JM, Bleecker ER, Nair P, Korn S, Ohta K, Lommatzsch M, et al. Benralizumab, an anti-interleukin-5 receptor α monoclonal antibody, as add-on treatment for patients with severe, uncontrolled, eosinophilic asthma (CALIMA): a randomised, double-blind, placebo-controlled phase 3 trial. Lancet (2016) 388:2128–41. doi:10.1016/S0140-6736(16)31322-8
24. Ullmann N, Bossley CJ, Fleming L, Silvestri M, Bush A, Saglani S. Blood eosinophil counts rarely reflect airway eosinophilia in children with severe asthma. Allergy (2013) 68:402–6. doi:10.1111/all.12101
25. Korevaar DA, Westerhof GA, Wang J, Cohen JF, Spijker R, Sterk PJ, et al. Diagnostic accuracy of minimally invasive markers for detection of airway eosinophilia in asthma: a systematic review and meta-analysis. Lancet Respir Med (2015) 3:290–300. doi:10.1016/S2213-2600(15)00050-8
26. Holt PG, Jones CA. The development of the immune system during pregnancy and early life. Allergy (2000) 55:688–97. doi:10.1034/j.1398-9995.2000.00118.x
27. Hammad H, Chieppa M, Perros F, Willart MA, Germain RN, Lambrecht BN. House dust mite allergen induces asthma via toll-like receptor 4 triggering of airway structural cells. Nat Med (2009) 15:410–6. doi:10.1038/nm.1946
28. Nathan AT, Peterson EA, Chakir J, Wills-Karp M. Innate immune responses of airway epithelium to house dust mite are mediated through β-glucan-dependent pathways. J Allergy Clin Immunol (2009) 123:612–8. doi:10.1016/j.jaci.2008.12.006
29. Angkasekwinai P, Park H, Wang Y-H, Wang Y-H, Chang SH, Corry DB, et al. Interleukin 25 promotes the initiation of proallergic type 2 responses. J Exp Med (2007) 204:1509–17. doi:10.1084/jem.20061675
30. Kondo Y, Yoshimoto T, Yasuda K, Futatsugi-Yumikura S, Morimoto M, Hayashi N, et al. Administration of IL-33 induces airway hyperresponsiveness and goblet cell hyperplasia in the lungs in the absence of adaptive immune system. Int Immunol (2008) 20:791–800. doi:10.1093/intimm/dxn037
31. Sebastian K, Borowski A, Kuepper M, Friedrich K. Signal transduction around thymic stromal lymphopoietin (TSLP) in atopic asthma. Cell Commun Signal (2008) 6:5. doi:10.1186/1478-811X-6-5
32. Kool M, Willart MAM, van Nimwegen M, Bergen I, Pouliot P, Virchow JC, et al. An unexpected role for uric acid as an inducer of T helper 2 cell immunity to inhaled antigens and inflammatory mediator of allergic asthma. Immunity (2011) 34:527–40. doi:10.1016/j.immuni.2011.03.015
33. Lambrecht BN, Hammad H. Lung dendritic cells in respiratory viral infection and asthma: from protection to immunopathology. Annu Rev Immunol (2012) 30:243–70. doi:10.1146/annurev-immunol-020711-075021
34. Robinson SP, Patterson S, English N, Davies D, Knight SC, Reid CD. Human peripheral blood contains two distinct lineages of dendritic cells. Eur J Immunol (1999) 29:2769–78. doi:10.1002/(SICI)1521-4141(199909)29:09<2769::AID-IMMU2769>3.0.CO;2-2
35. Plantinga M, Guilliams M, Vanheerswynghels M, Deswarte K, Branco-Madeira F, Toussaint W, et al. Conventional and monocyte-derived CD11b+ dendritic cells initiate and maintain T helper 2 cell-mediated immunity to house dust mite allergen. Immunity (2013) 38:322–35. doi:10.1016/j.immuni.2012.10.016
36. de Heer HJ, Hammad H, Soullié T, Hijdra D, Vos N, Willart MAM, et al. Essential role of lung plasmacytoid dendritic cells in preventing asthmatic reactions to harmless inhaled antigen. J Exp Med (2004) 200:89–98. doi:10.1084/jem.20040035
37. Sung S-SJ, Fu SM, Rose CE, Gaskin F, Ju S-T, Beaty SR. A major lung CD103 (alphaE)-beta7 integrin-positive epithelial dendritic cell population expressing Langerin and tight junction proteins. J Immunol (2006) 176:2161–72. doi:10.4049/jimmunol.176.4.2161
38. Willart MAM, Lambrecht BN. The danger within: endogenous danger signals, atopy and asthma. Clin Exp Allergy (2009) 39:12–9. doi:10.1111/j.1365-2222.2008.03118.x
39. Ezzat MHM, Imam SS, Shaheen KYA, Elbrhami EM. Serum OX40 ligand levels in asthmatic children: a potential biomarker of severity and persistence. Allergy Asthma Proc (2011) 32:313–8. doi:10.2500/aap.2011.32.3447
40. Brugha R, Mushtaq N, McCarthy NE, Stagg AJ, Grigg J. Respiratory tract dendritic cells in paediatric asthma. Clin Exp Allergy (2015) 45:624–31. doi:10.1111/cea.12457
41. Hagendorens MM, Ebo DG, Schuerwegh AJ, Huybrechs A, Van Bever HP, Bridts CH, et al. Differences in circulating dendritic cell subtypes in cord blood and peripheral blood of healthy and allergic children. Clin Exp Allergy (2003) 33:633–9. doi:10.1046/j.1365-2222.2003.01649.x
42. Matsuda H, Suda T, Hashizume H, Yokomura K, Asada K, Suzuki K, et al. Alteration of balance between myeloid dendritic cells and plasmacytoid dendritic cells in peripheral blood of patients with asthma. Am J Respir Crit Care Med (2002) 166:1050–4. doi:10.1164/rccm.2110066
43. Holgate ST. The epithelium takes centre stage in asthma and atopic dermatitis. Trends Immunol (2007) 28:248–51. doi:10.1016/j.it.2007.04.007
44. Koppelman GH, Meyers DA, Howard TD, Zheng SL, Hawkins GA, Ampleford EJ, et al. Identification of PCDH1 as a novel susceptibility gene for bronchial hyperresponsiveness. Am J Respir Crit Care Med (2009) 180:929–35. doi:10.1164/rccm.200810-1621OC
45. Woodruff PG, Boushey HA, Dolganov GM, Barker CS, Yang YH, Donnelly S, et al. Genome-wide profiling identifies epithelial cell genes associated with asthma and with treatment response to corticosteroids. Proc Natl Acad Sci U S A (2007) 104:15858–63. doi:10.1073/pnas.0707413104
46. Mortensen LJ, Kreiner-Møller E, Hakonarson H, Bønnelykke K, Bisgaard H. The PCDH1-gene and asthma in early childhood. Eur Respir J (2014) 43:792–800. doi:10.1183/09031936.00021613
47. Lloyd CM, Saglani S. Asthma and allergy: the emerging epithelium. Nat Med (2010) 16:273–4. doi:10.1038/nm0310-273
48. Pillai P, Corrigan CJ, Ying S. Airway epithelium in atopic and nonatopic asthma: similarities and differences. ISRN Allergy (2011) 2011:195846. doi:10.5402/2011/195846
49. Schmidt M, Mattoli S. A mouse model for evaluating the contribution of fibrocytes and myofibroblasts to airway remodeling in allergic asthma. Methods Mol Biol (2013) 1032:235–55. doi:10.1007/978-1-62703-496-8_19
50. Nihlberg K, Larsen K, Hultgårdh-Nilsson A, Malmström A, Bjermer L, Westergren-Thorsson G. Tissue fibrocytes in patients with mild asthma: a possible link to thickness of reticular basement membrane? Respir Res (2006) 7:50. doi:10.1186/1465-9921-7-50
51. Zhang M, Zhang Z, Pan H-Y, Wang D-X, Deng Z-T, Ye X-L. TGF-β1 induces human bronchial epithelial cell-to-mesenchymal transition in vitro. Lung (2009) 187:187–94. doi:10.1007/s00408-009-9139-5
52. Davies DE. The role of the epithelium in airway remodeling in asthma. Proc Am Thorac Soc (2009) 6:678–82. doi:10.1513/pats.200907-067DP
53. Allakhverdi Z, Comeau MR, Jessup HK, Yoon B-RP, Brewer A, Chartier S, et al. Thymic stromal lymphopoietin is released by human epithelial cells in response to microbes, trauma, or inflammation and potently activates mast cells. J Exp Med (2007) 204:253–8. doi:10.1084/jem.20062211
54. Mjösberg J, Bernink J, Golebski K, Karrich JJ, Peters CP, Blom B, et al. The transcription factor GATA3 is essential for the function of human type 2 innate lymphoid cells. Immunity (2012) 37:649–59. doi:10.1016/j.immuni.2012.08.015
55. Siracusa MC, Saenz SA, Hill DA, Kim BS, Headley MB, Doering TA, et al. TSLP promotes interleukin-3-independent basophil haematopoiesis and type 2 inflammation. Nature (2011) 477:229–33. doi:10.1038/nature10329
56. Saglani S, Lui S, Ullmann N, Campbell GA, Sherburn RT, Mathie SA, et al. IL-33 promotes airway remodeling in pediatric patients with severe steroid-resistant asthma. J Allergy Clin Immunol (2013) 132:676–85.e13. doi:10.1016/j.jaci.2013.04.012
57. Castanhinha S, Sherburn R, Walker S, Gupta A, Bossley CJ, Buckley J, et al. Pediatric severe asthma with fungal sensitization is mediated by steroid-resistant IL-33. J Allergy Clin Immunol (2015) 136:312–22.e7. doi:10.1016/j.jaci.2015.01.016
58. Nagakumar P, Denney L, Fleming L, Bush A, Lloyd CM, Saglani S. Type 2 innate lymphoid cells in induced sputum from children with severe asthma. J Allergy Clin Immunol (2016) 137:624–6.e6. doi:10.1016/j.jaci.2015.06.038
59. Smith SG, Chen R, Kjarsgaard M, Huang C, Oliveria J-P, O’Byrne PM, et al. Increased numbers of activated group 2 innate lymphoid cells in the airways of patients with severe asthma and persistent airway eosinophilia. J Allergy Clin Immunol (2016) 137:75–86.e8. doi:10.1016/j.jaci.2015.05.037
60. Bradding P, Arthur G. Mast cells in asthma – state of the art. Clin Exp Allergy (2016) 46:194–263. doi:10.1111/CEA.12675
61. Henderson WR. Eicosanoids and platelet-activating factor in allergic respiratory diseases. Am Rev Respir Dis (1991) 143:S86–90. doi:10.1164/ajrccm/143.5_Pt_2.S86
62. Bradley B, Azzawi M, Jacobson M, Assoufi B, Collins J, Irani AM, et al. Eosinophils, T-lymphocytes, mast cells, neutrophils, and macrophages in bronchial biopsy specimens from atopic subjects with asthma: comparison with biopsy specimens from atopic subjects without asthma and normal control subjects and relationship to bronc. J Allergy Clin Immunol (1991) 88:661–74. doi:10.1016/0091-6749(91)90160-P
63. Amin K, Janson C, Boman G, Venge P. The extracellular deposition of mast cell products is increased in hypertrophic airways smooth muscles in allergic asthma but not in nonallergic asthma. Allergy (2005) 60:1241–7. doi:10.1111/j.1398-9995.2005.00823.x
64. Balzar S, Fajt ML, Comhair SAA, Erzurum SC, Bleecker E, Busse WW, et al. Mast cell phenotype, location, and activation in severe asthma. Am J Respir Crit Care Med (2011) 183:299–309. doi:10.1164/rccm.201002-0295OC
65. Carroll NG, Mutavdzic S, James AL. Distribution and degranulation of airway mast cells in normal and asthmatic subjects. Eur Respir J (2002) 19:879–85. doi:10.1183/09031936.02.00275802
66. Brightling CE, Bradding P, Symon FA, Holgate ST, Wardlaw AJ, Pavord ID. Mast-cell infiltration of airway smooth muscle in asthma. N Engl J Med (2002) 346:1699–705. doi:10.1056/NEJMoa012705
67. Barbato A, Turato G, Baraldo S, Bazzan E, Calabrese F, Panizzolo C, et al. Epithelial damage and angiogenesis in the airways of children with asthma. Am J Respir Crit Care Med (2006) 174:975–81. doi:10.1164/rccm.200602-189OC
68. Baraldo S, Turato G, Bazzan E, Ballarin A, Damin M, Balestro E, et al. Noneosinophilic asthma in children: relation with airway remodelling. Eur Respir J (2011) 38:575–83. doi:10.1183/09031936.00168210
69. O’Reilly R, Ullmann N, Irving S, Bossley CJ, Sonnappa S, Zhu J, et al. Increased airway smooth muscle in preschool wheezers who have asthma at school age. J Allergy Clin Immunol (2013) 131:1024–32, 1032.e1–16. doi:10.1016/j.jaci.2012.08.044
70. Lezmi G, Galmiche-Rolland L, Rioux S, Jaubert F, Tillie-Leblond I, Scheinmann P, et al. Mast cells are associated with exacerbations and eosinophilia in children with severe asthma. Eur Respir J (2016) 48:1320–8. doi:10.1183/13993003.00947-2016
71. Santus P, Radovanovic D. Prostaglandin D2 receptor antagonists in early development as potential therapeutic options for asthma. Expert Opin Investig Drugs (2016) 25:1083–92. doi:10.1080/13543784.2016.1212838
72. Gonem S, Berair R, Singapuri A, Hartley R, Laurencin MFM, Bacher G, et al. Fevipiprant, a prostaglandin D2 receptor 2 antagonist, in patients with persistent eosinophilic asthma: a single-centre, randomised, double-blind, parallel-group, placebo-controlled trial. Lancet Respir Med (2016) 4:699–707. doi:10.1016/S2213-2600(16)30179-5
73. Kuna P, Bjermer L, Tornling G. Two phase II randomized trials on the CRTh2 antagonist AZD1981 in adults with asthma. Drug Des Devel Ther (2016) 10:2759–70. doi:10.2147/DDDT.S105142
74. Chung KF. New treatments for severe treatment-resistant asthma: targeting the right patient. Lancet Respir Med (2013) 1:639–52. doi:10.1016/S2213-2600(13)70128-0
75. Lloyd CM, Hawrylowicz CM, Leduc D, Dumarey C, Huerre M, Vargaftig BB, et al. Regulatory T cells in asthma. Immunity (2009) 31:438–49. doi:10.1016/j.immuni.2009.08.007
76. Mamessier E, Nieves A, Lorec A-M, Dupuy P, Pinot D, Pinet C, et al. T-cell activation during exacerbations: a longitudinal study in refractory asthma. Allergy (2008) 63:1202–10. doi:10.1111/j.1398-9995.2008.01687.x
77. Smyth LJC, Eustace A, Kolsum U, Blaikely J, Singh D. Increased airway T regulatory cells in asthmatic subjects. Chest (2010) 138:905–12. doi:10.1378/chest.09-3079
78. Hartl D, Koller B, Mehlhorn AT, Reinhardt D, Nicolai T, Schendel DJ, et al. Quantitative and functional impairment of pulmonary CD4+CD25hi regulatory T cells in pediatric asthma. J Allergy Clin Immunol (2007) 119:1258–66. doi:10.1016/j.jaci.2007.02.023
79. Yang Y-L, Pan Y-Q, He B-S, Zhong T-Y. Regulatory T cells and Th1/Th2 in peripheral blood and their roles in asthmatic children. Transl Pediatr (2013) 2:27–33. doi:10.3978/j.issn.2224-4336.2012.04.03
80. O’Garra A, Barrat FJ, Castro AG, Vicari A, Hawrylowicz C. Strategies for use of IL-10 or its antagonists in human disease. Immunol Rev (2008) 223:114–31. doi:10.1111/j.1600-065X.2008.00635.x
81. Till SJ, Francis JN, Nouri-Aria K, Durham SR. Mechanisms of immunotherapy. J Allergy Clin Immunol (2004) 113:1025–34. doi:10.1016/j.jaci.2004.03.024
82. John M, Lim S, Seybold J, Jose P, Robichaud A, O’Connor B, et al. Inhaled corticosteroids increase interleukin-10 but reduce macrophage inflammatory protein-1 α, granulocyte-macrophage colony-stimulating factor, and interferon-γ release from alveolar macrophages in asthma. Am J Respir Crit Care Med (1998) 157:256–62. doi:10.1164/ajrccm.157.1.9703079
83. Lim S, Crawley E, Woo P, Barnes PJ. Haplotype associated with low interleukin-10 production in patients with severe asthma. Lancet (1998) 352:113. doi:10.1016/S0140-6736(98)85018-6
84. Gupta A, Dimeloe S, Richards DF, Chambers ES, Black C, Urry Z, et al. Defective IL-10 expression and in vitro steroid-induced IL-17A in paediatric severe therapy-resistant asthma. Thorax (2014) 69:508–15. doi:10.1136/thoraxjnl-2013-203421
85. Urry Z, Chambers ES, Xystrakis E, Dimeloe S, Richards DF, Gabryšová L, et al. The role of 1α,25-dihydroxyvitamin D3 and cytokines in the promotion of distinct Foxp3+ and IL-10+ CD4+ T cells. Eur J Immunol (2012) 42:2697–708. doi:10.1002/eji.201242370
86. Tsai T-C, Lu J-H, Chen S-J, Tang R-B. Soluble interleukin-10 and transforming growth factor-β in children with acute exacerbation of allergic asthma. J Asthma (2009) 46:21–4. doi:10.1080/02770900802460548
87. Li MO, Sanjabi S, Flavell RA. Transforming growth factor-β controls development, homeostasis, and tolerance of T cells by regulatory T cell-dependent and -independent mechanisms. Immunity (2006) 25:455–71. doi:10.1016/j.immuni.2006.07.011
88. Hatsushika K, Hirota T, Harada M, Sakashita M, Kanzaki M, Takano S, et al. Transforming growth factor-beta(2) polymorphisms are associated with childhood atopic asthma. Clin Exp Allergy (2007) 37:1165–74. doi:10.1111/j.1365-2222.2007.02768.x
89. Lopez-Guisa JM, Powers C, File D, Cochrane E, Jimenez N, Debley JS. Airway epithelial cells from asthmatic children differentially express proremodeling factors. J Allergy Clin Immunol (2012) 129:990–7.e6. doi:10.1016/j.jaci.2011.11.035
90. Payne DNR, Rogers AV, Ädelroth E, Bandi V, Guntupalli KK, Bush A, et al. Early thickening of the reticular basement membrane in children with difficult asthma. Am J Respir Crit Care Med (2003) 167:78–82. doi:10.1164/rccm.200205-414OC
91. Regamey N, Ochs M, Hilliard TN, Mühlfeld C, Cornish N, Fleming L, et al. Increased airway smooth muscle mass in children with asthma, cystic fibrosis, and non-cystic fibrosis bronchiectasis. Am J Respir Crit Care Med (2008) 177:837–43. doi:10.1164/rccm.200707-977OC
92. Tillie-Leblond I, de Blic J, Jaubert F, Wallaert B, Scheinmann P, Gosset P. Airway remodeling is correlated with obstruction in children with severe asthma. Allergy (2008) 63:533–41. doi:10.1111/j.1398-9995.2008.01656.x
93. Jenkins HA, Cool C, Szefler SJ, Covar R, Brugman S, Gelfand EW, et al. Histopathology of severe childhood asthma: a case series. Chest (2003) 124:32–41. doi:10.1378/chest.124.1.32
94. Gupta A, Sjoukes A, Richards D, Banya W, Hawrylowicz C, Bush A, et al. Relationship between serum vitamin D, disease severity, and airway remodeling in children with asthma. Am J Respir Crit Care Med (2011) 184:1342–9. doi:10.1164/rccm.201107-1239OC
95. Fedorov IA, Wilson SJ, Davies DE, Holgate ST. Epithelial stress and structural remodelling in childhood asthma. Thorax (2005) 60:389–94. doi:10.1136/thx.2004.030262
96. Fattouh R, Midence NG, Arias K, Johnson JR, Walker TD, Goncharova S, et al. Transforming growth factor-β regulates house dust mite-induced allergic airway inflammation but not airway remodeling. Am J Respir Crit Care Med (2008) 177:593–603. doi:10.1164/rccm.200706-958OC
97. Saglani S, Mathie SA, Gregory LG, Bell MJ, Bush A, Lloyd CM. Pathophysiological features of asthma develop in parallel in house dust mite-exposed neonatal mice. Am J Respir Cell Mol Biol (2009) 41:281–9. doi:10.1165/rcmb.2008-0396OC
98. Grainge CL, Lau LCK, Ward JA, Dulay V, Lahiff G, Wilson S, et al. Effect of bronchoconstriction on airway remodeling in asthma. N Engl J Med (2011) 364:2006–15. doi:10.1056/NEJMoa1014350
99. Fitzpatrick AM. Severe asthma in children: lessons learned and future directions. J Allergy Clin Immunol Pract (2016) 4:11–9. doi:10.1016/j.jaip.2015.10.008
100. Vasiliou JE, Lui S, Walker SA, Chohan V, Xystrakis E, Bush A, et al. Vitamin D deficiency induces Th2 skewing and eosinophilia in neonatal allergic airways disease. Allergy (2014) 69:1380–9. doi:10.1111/all.12465
101. Chang P-J, Michaeloudes C, Zhu J, Shaikh N, Baker J, Chung KF, et al. Impaired nuclear translocation of the glucocorticoid receptor in corticosteroid-insensitive airway smooth muscle in severe asthma. Am J Respir Crit Care Med (2015) 191:54–62. doi:10.1164/rccm.201402-0314OC
102. Hilliard TN, Regamey N, Shute JK, Nicholson AG, Alton EWFW, Bush A, et al. Airway remodelling in children with cystic fibrosis. Thorax (2007) 62:1074–80. doi:10.1136/thx.2006.074641
103. Bourdin A, Neveu D, Vachier I, Paganin F, Godard P, Chanez P, et al. Specificity of basement membrane thickening in severe asthma. J Allergy Clin Immunol (2007) 119:1367–74. doi:10.1016/j.jaci.2007.01.055
104. Sharples J, Gupta A, Fleming L, Bossley CJ, Bracken-King M, Hall P, et al. Long-term effectiveness of a staged assessment for paediatric problematic severe asthma. Eur Respir J (2012) 40:264–7. doi:10.1183/09031936.00209511
105. Marinho S, Simpson A, Marsden P, Smith JA, Custovic A. Quantification of atopy, lung function and airway hypersensitivity in adults. Clin Transl Allergy (2011) 1:16. doi:10.1186/2045-7022-1-16
106. Marinho S, Simpson A, Lowe L, Kissen P, Murray C, Custovic A. Original article: rhinoconjunctivitis in 5-year-old children: a population-based birth cohort study. Allergy (2007) 62:385–93. doi:10.1111/j.1398-9995.2006.01294.x
107. Just J, Saint-Pierre P, Gouvis-Echraghi R, Laoudi Y, Roufai L, Momas I, et al. Childhood allergic asthma is not a single phenotype. J Pediatr (2014) 164:815–20. doi:10.1016/j.jpeds.2013.11.037
108. Del Giacco SR, Bakirtas A, Bel E, Custovic A, Diamant Z, Hamelmann E, et al. Allergy in severe asthma. Allergy (2017) 72:207–20. doi:10.1111/all.13072
109. Djukanović R, Wilson SJ, Kraft M, Jarjour NN, Steel M, Chung KF, et al. Effects of treatment with anti-immunoglobulin E antibody omalizumab on airway inflammation in allergic asthma. Am J Respir Crit Care Med (2004) 170:583–93. doi:10.1164/rccm.200312-1651OC
110. Holgate S, Casale T, Wenzel S, Bousquet J, Deniz Y, Reisner C. The anti-inflammatory effects of omalizumab confirm the central role of IgE in allergic inflammation. J Allergy Clin Immunol (2005) 115:459–65. doi:10.1016/j.jaci.2004.11.053
111. Milgrom H, Berger W, Nayak A, Gupta N, Pollard S, McAlary M, et al. Treatment of childhood asthma with anti-immunoglobulin E antibody (omalizumab). Pediatrics (2001) 108:E36. doi:10.1542/peds.108.2.e36
112. Brodlie M, McKean MC, Moss S, Spencer DA. The oral corticosteroid-sparing effect of omalizumab in children with severe asthma. Arch Dis Child (2012) 97:604–9. doi:10.1136/archdischild-2011-301570
113. Rodrigo GJ, Neffen H. Systematic review on the use of omalizumab for the treatment of asthmatic children and adolescents. Pediatr Allergy Immunol (2015) 26:551–6. doi:10.1111/pai.12405
114. Deschildre A, Marguet C, Langlois C, Pin I, Rittié J-L, Derelle J, et al. Real-life long-term omalizumab therapy in children with severe allergic asthma. Eur Respir J (2015) 46:856–9. doi:10.1183/09031936.00008115
115. Teach SJ, Gill MA, Togias A, Sorkness CA, Arbes SJ, Calatroni A, et al. Preseasonal treatment with either omalizumab or an inhaled corticosteroid boost to prevent fall asthma exacerbations. J Allergy Clin Immunol (2015) 136:1476–85. doi:10.1016/j.jaci.2015.09.008
116. Gruchalla RS, Sampson HA, Liu AH, Shreffler W, Wallace PK, Togias A, et al. Effects of omalizumab on T lymphocyte function in inner-city children with asthma. Pediatr Allergy Immunol (2016) 27:328–31. doi:10.1111/pai.12508
117. Krawiec ME, Westcott JY, Chu HW, Balzar S, Trudeau JB, Schwartz LB, et al. Persistent wheezing in very young children is associated with lower respiratory inflammation. Am J Respir Crit Care Med (2001) 163:1338–43. doi:10.1164/ajrccm.163.6.2005116
118. Saglani S, Malmström K, Pelkonen AS, Malmberg LP, Lindahl H, Kajosaari M, et al. Airway remodeling and inflammation in symptomatic infants with reversible airflow obstruction. Am J Respir Crit Care Med (2005) 171:722–7. doi:10.1164/rccm.200410-1404OC
119. Saglani S, Payne DN, Zhu J, Wang Z, Nicholson AG, Bush A, et al. Early detection of airway wall remodeling and eosinophilic inflammation in preschool wheezers. Am J Respir Crit Care Med (2007) 176:858–64. doi:10.1164/rccm.200702-212OC
120. Illi S, von Mutius E, Lau S, Niggemann B, Grüber C, Wahn U. Multicentre Allergy Study (MAS) group. Perennial allergen sensitisation early in life and chronic asthma in children: a birth cohort study. Lancet (2006) 368:763–70. doi:10.1016/S0140-6736(06)69286-6
121. Simpson A, Lazic N, Belgrave DCM, Johnson P, Bishop C, Mills C, et al. Patterns of IgE responses to multiple allergen components and clinical symptoms at age 11 years. J Allergy Clin Immunol (2015) 136:1224–31. doi:10.1016/j.jaci.2015.03.027
122. Custovic A, Sonntag H-J, Buchan IE, Belgrave D, Simpson A, Prosperi MC. Evolution pathways of IgE responses to grass and mite allergens throughout childhood. J Allergy Clin Immunol (2015) 136:1645–52.e1–8. doi:10.1016/j.jaci.2015.03.041
123. Guilbert TW, Morgan WJ, Zeiger RS, Mauger DT, Boehmer SJ, Szefler SJ, et al. Long-term inhaled corticosteroids in preschool children at high risk for asthma. N Engl J Med (2006) 354:1985–97. doi:10.1056/NEJMoa051378
124. Jackson DJ, Hartert TV, Martinez FD, Weiss ST, Fahy JV. Asthma: NHLBI workshop on the primary prevention of chronic lung diseases. Ann Am Thorac Soc (2014) 11:S139–45. doi:10.1513/AnnalsATS.201312-448LD
125. Phipatanakul W; Boston Children’s Hospital. Controlling and Preventing Asthma Progression and Severity in Kids (CASK). (2017). Available from: https://clinicaltrials.gov/ct2/show/NCT02570984?term=Wanda+Phipatanakul&rank=1
126. Rodrigo GJ, Castro-Rodriguez JA. Anticholinergics in the treatment of children and adults with acute asthma: a systematic review with meta-analysis. Thorax (2005) 60:740–6. doi:10.1136/thx.2005.040444
127. Teoh L, Cates CJ, Hurwitz M, Acworth JP, van Asperen P, Chang AB. Anticholinergic therapy for acute asthma in children. Cochrane Database Syst Rev (2012) 4:CD003797. doi:10.1002/14651858.pub2
128. Griffiths B, Ducharme FM. Combined inhaled anticholinergics and short-acting beta 2-agonists for initial treatment of acute asthma in children. Cochrane Database Syst Rev (2013) 8:CD000060. doi:10.1002/14651858.CD000060.pub2
129. Kerstjens HAM, Disse B, Schröder-Babo W, Bantje TA, Gahlemann M, Sigmund R, et al. Tiotropium improves lung function in patients with severe uncontrolled asthma: a randomized controlled trial. J Allergy Clin Immunol (2011) 128:308–14. doi:10.1016/j.jaci.2011.04.039
130. Kerstjens HAM, Engel M, Dahl R, Paggiaro P, Beck E, Vandewalker M, et al. Tiotropium in asthma poorly controlled with standard combination therapy. N Engl J Med (2012) 367:1198–207. doi:10.1056/NEJMoa1208606
131. Boehringer Ingelheim International GmbH. Spiriva Respimat, Highlights of Prescribing Information. (2017). Available from: http://docs.boehringer-ingelheim.com/Prescribing Information/PIs/Spiriva Respimat/spirivarespimat.pdf
132. National Institute for Health and Care Excellence. Omalizumab for Treating Severe Persistent Allergic Asthma. (2017). Available from: https://www.nice.org.uk/guidance/ta278
133. National Institute for Health and Care Excellence. Mepolizumab for Treating Severe Refractory Eosinophilic Asthma. (2017). Available from: https://www.nice.org.uk/guidance/GID-TAG519
134. Corren J, Lemanske RF, Hanania NA, Korenblat PE, Parsey MV, Arron JR, et al. Lebrikizumab treatment in adults with asthma. N Engl J Med (2011) 365:1088–98. doi:10.1056/NEJMoa1106469
135. Hanania NA, Korenblat P, Chapman KR, Bateman, Kopecky P, Paggiaro P, et al. Efficacy and safety of lebrikizumab in patients with uncontrolled asthma (LAVOLTA I and LAVOLTA II): replicate, phase 3, randomised, double-blind, placebo-controlled trials. Lancet Respir Med (2016) 4:781–96. doi:10.1016/S2213-2600(16)30265-X
136. Perkins C, Wills-Karp M, Finkelman FD. IL-4 induces IL-13-independent allergic airway inflammation. J Allergy Clin Immunol (2006) 118:410–9. doi:10.1016/j.jaci.2006.06.004
137. Wenzel S, Ford L, Pearlman D, Spector S, Sher L, Skobieranda F, et al. Dupilumab in persistent asthma with elevated eosinophil levels. N Engl J Med (2013) 368:2455–66. doi:10.1056/NEJMoa1304048
138. Wenzel S, Castro M, Corren J, Maspero J, Wang L, Zhang B, et al. Dupilumab efficacy and safety in adults with uncontrolled persistent asthma despite use of medium-to-high-dose inhaled corticosteroids plus a long-acting β2 agonist: a randomised double-blind placebo-controlled pivotal phase 2b dose-ranging trial. Lancet (2016) 388:31–44. doi:10.1016/S0140-6736(16)30307-5
139. Sanofi, Regeneron Pharmaceuticals. Evaluation of Dupilumab in Patients with Persistent Asthma (Liberty Asthma Quest). (2017). Available from: https://clinicaltrials.gov/ct2/show/NCT02414854?term=NCT02414854&rank=1
140. Getz EB, Fisher DM, Fuller R. Human pharmacokinetics/pharmacodynamics of an interleukin-4 and interleukin-13 dual antagonist in asthma. J Clin Pharmacol (2009) 49:1025–36. doi:10.1177/0091270009341183
141. Slager RE, Otulana BA, Hawkins GA, Yen YP, Peters SP, Wenzel SE, et al. IL-4 receptor polymorphisms predict reduction in asthma exacerbations during response to an anti-IL-4 receptor α antagonist. J Allergy Clin Immunol (2012) 130:516–22.e4. doi:10.1016/j.jaci.2012.03.030
142. Barczyk A, Pierzchala W, Sozañska E. Interleukin-17 in sputum correlates with airway hyperresponsiveness to methacholine. Respir Med (2003) 97:726–33. doi:10.1053/rmed.2003.1507
143. Molet S, Hamid Q, Davoineb F, Nutku E, Tahaa R, Pagé N, et al. IL-17 is increased in asthmatic airways and induces human bronchial fibroblasts to produce cytokines. J Allergy Clin Immunol (2001) 108:430–8. doi:10.1067/mai.2001.117929
144. Al-Ramli W, Préfontaine D, Chouiali F, Martin JG, Olivenstein R, Lemière C, et al. TH17-associated cytokines (IL-17A and IL-17F) in severe asthma. J Allergy Clin Immunol (2009) 123:1185–7. doi:10.1016/j.jaci.2009.02.024
145. Agache I, Ciobanu C, Agache C, Anghel M. Increased serum IL-17 is an independent risk factor for severe asthma. Respir Med (2010) 104:1131–7. doi:10.1016/j.rmed.2010.02.018
146. Chesné J, Braza F, Mahay G, Brouard S, Aronica M, Magnan A. IL-17 in severe asthma. Where do we stand? Am J Respir Crit Care Med (2014) 190:1094–101. doi:10.1164/rccm.201405-0859PP
147. Albano GD, Di Sano C, Bonanno A, Riccobono L, Gagliardo R, Chanez P, et al. Th17 immunity in children with allergic asthma and rhinitis: a pharmacological approach. PLoS One (2013) 8:e58892. doi:10.1371/journal.pone.0058892
148. Andersson CK, Adams A, Nagakumar P, Bossley C, Gupta A, De Vries D, et al. Intraepithelial neutrophils in pediatric severe asthma are associated with better lung function. J Allergy Clin Immunol (2017) 139(6):1819–29.e11. doi:10.1016/j.jaci.2016.09.022
149. Busse WW, Holgate S, Kerwin E, Chon Y, Feng J, Lin J, et al. Randomized, double-blind, placebo-controlled study of brodalumab, a human anti–IL-17 receptor monoclonal antibody, in moderate to severe asthma. Am J Respir Crit Care Med (2013) 188:1294–302. doi:10.1164/rccm.201212-2318OC
150. Gauvreau GM, O’Byrne PM, Boulet L-P, Wang Y, Cockcroft D, Bigler J, et al. Effects of an anti-TSLP antibody on allergen-induced asthmatic responses. N Engl J Med (2014) 370:2102–10. doi:10.1056/NEJMoa1402895
151. MedImmune LLC, Amgen. A Study to Evaluate the Pharmacokinetics of MEDI9929 (AMG 157) in Adolescents with Mild to Moderate Asthma. (2017). Available from: https://clinicaltrials.gov/ct2/show/NCT02512900?term=NCT02512900&rank=1
152. MedImmune LLC, Amgen. Study to Evaluate the Efficacy and Safety of MEDI9929 (AMG 157) in Adult Subjects with Inadequately Controlled, Severe Asthma. (2017). Available at: https://clinicaltrials.gov/ct2/show/NCT02054130?term=NCT02054130&rank=1
Keywords: severe therapy-resistant asthma, mechanisms, eosinophils, innate cytokines, therapies, remodeling, children
Citation: Martin Alonso A and Saglani S (2017) Mechanisms Mediating Pediatric Severe Asthma and Potential Novel Therapies. Front. Pediatr. 5:154. doi: 10.3389/fped.2017.00154
Received: 11 April 2017; Accepted: 20 June 2017;
Published: 05 July 2017
Edited by:
Luis Garcia-Marcos, Universidad de Murcia, SpainReviewed by:
Michael B. Anthracopoulos, University of Patras, GreeceKostas N. Priftis, National and Kapodistrian University of Athens, Greece
Kelechi Benjamin Ugonna, Sheffield Children’s Hospital, United Kingdom
Copyright: © 2017 Martin Alonso and Saglani. This is an open-access article distributed under the terms of the Creative Commons Attribution License (CC BY). The use, distribution or reproduction in other forums is permitted, provided the original author(s) or licensor are credited and that the original publication in this journal is cited, in accordance with accepted academic practice. No use, distribution or reproduction is permitted which does not comply with these terms.
*Correspondence: Sejal Saglani, s.saglani@imperial.ac.uk