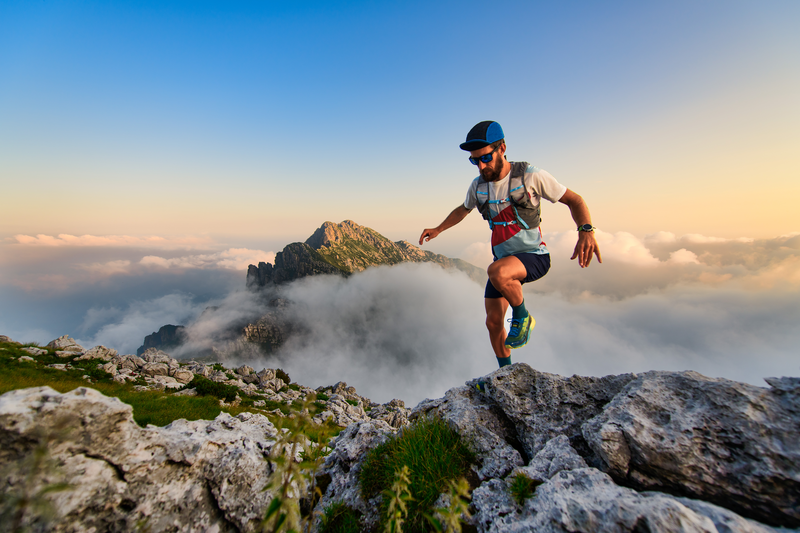
95% of researchers rate our articles as excellent or good
Learn more about the work of our research integrity team to safeguard the quality of each article we publish.
Find out more
MINI REVIEW article
Front. Parasitol. , 01 July 2024
Sec. Parasite Diagnostics
Volume 3 - 2024 | https://doi.org/10.3389/fpara.2024.1394089
This article is part of the Research Topic Advances in Diagnostics for Neglected Parasitic Diseases View all 5 articles
Neurocysticercosis (NCC) is caused by the invasion of Taenia solium larvae in the central nervous system (CNS) and stands as the predominant cause of epilepsy and other neurological disorders in many developing nations. NCC diagnosis is challenging because it relies on brain imaging exams (CT or MRI), which are poorly available in endemic rural or resource-limited areas. Moreover, some NCC cases cannot be easily detected by imaging, leading to inconclusive results. Multiple laboratory assays, principally immunological, have been developed to support the diagnosis and/or monitor the treatment efficacy, but its production can be costly, laborious, and non-globally accessible because they depend on parasite material. Therefore, recent advances have been focused on the implementation of recombinant or synthetic antigens as well as monoclonal antibodies for NCC immunodiagnosis purposes. Similarly, molecular diagnosis has been explored, obtaining promising results. Here we described the recent progress in the development of immunological and molecular diagnostic tools for NCC diagnosis over the past 13 years, discussing their potential application to address important challenges and how to focus future directions to improve NCC diagnosis with emphasis on enhance accessibility and the importance of test validation to provide an adequate support for clinical decisions.
Neurocysticercosis (NCC) is a neglected tropical disease resulting from the ingestion of Taenia solium eggs that can pass through the intestines, enter the bloodstream, and establish in the central nervous system (CNS), forming a cyst or cysticercus (Dixon et al., 2021). Its life cycle included humans and pigs as intermediate hosts, harboring the larvae or cysticercus, and humans as the definitive hosts for the tapeworm. This infection has public health significance, being the main contributor to acquired epilepsy and various others neurological morbidities worldwide (Cárdenas et al., 2021). The prevalence of NCC is not only high in all regions of the developing world but also there is an increasing frequency in industrialized countries due to immigration from endemic zones (Butala et al., 2021; Haddad et al., 2023).
This heterogeneous disease is typically asymptomatic during parasite establishment and when the cysts remain viable; however, the natural disease progression (or induced by anti-helminthic treatment) produces a degeneration process, resulting in an inflammatory reaction (Garcia et al., 2014). Clinical manifestations and host immunological responses are presented based on the stage, number, size, and principally the localization of cysts (Butala et al., 2021; Pineda-Reyes and White, 2022). Parasitic lesions that develop within the brain parenchyma (parenchymal NCC) typically transforms from viable, inflamed cysts to ultimately form calcified scars. Seizures are the primary clinical manifestation due to the focal inflammation of lesions, and prognosis is generally favorable (Del Brutto, 2022). On the other hand, extraparenchymal NCC involves the parasite invading the ventricles or subarachnoid space (subarachnoid NCC, SANCC) and growing massively, causing intracranial hypertension, and being associated to significant mortality rates (Mahale et al., 2015; Abanto et al., 2021).
The confirmation of NCC diagnosis absolutely requires neuroimaging; however, immunodiagnostic tests are accessible diagnostic tools that support imaging findings, especially in rural or resource-limited areas (Del Brutto, 2012). The host immune response has been well studied over the years, identifying a general pattern of immunodominance based on NCC classification. SANCC patients are the most immunodominant (Nash and O’Connell, 2020), followed by parenchymal with viable multiple cysts. Immunodominance decreases depending on the number of cysts (Garcia et al., 2018a; Channel et al., 2021), with single lesions being generally undiagnosed by common immunological tests. Finally, patients with calcified lesions have fewer detectable immune responses (Nash et al., 2015; Nash et al., 2017a; Nash et al., 2017b). In this context, immunodiagnostic tools based on antibody and/or antigen detection could help to discriminate viable and/or severe infections for a confirmatory diagnosis and follow-up (Deckers and Dorny, 2010; Zea-Vera et al., 2013).
Over the years, various diagnostic tools for NCC have been developed and tested, but not all have been properly implemented. As members of the Cysticercosis Working Group in Peru, we have compiled this manuscript to summarize our most recent contributions to the development of laboratory tests and have additionally included some published works from other groups. Although we are aware that the immunological response is not only driven by the parasite’s establishment in the brain but also by muscular cysticercosis, we have focused this review on published advances in diagnostic techniques only tested for NCC to simplify the understanding of this complex disease. The primary aim of this review is to update the current knowledge about new immunological and molecular techniques and propose future applications for them, providing solutions to challenging scenarios in NCC diagnosis.
The definitive diagnostic test for NCC is neuroimaging; however, its use is limited. Immunological tests to detect antibodies and antigens have been widely used as a support for diagnosis (Gilman, 2016; Del Brutto, 2022). They were initially developed for cerebrospinal fluid (CSF) samples and now are commonly used in serum, which is relatively easier to obtain.
Antibody detection using the test of choice, enzyme-linked immunoelectrotransfer blot (EITB) (Tsang et al., 1989) or western blot, is based on a mix of seven glycoproteins purified from T. solium cysts, offering an excellent performance (100% sensitivity and specificity) (Tsang et al., 1991). However, the purification process demands substantial amounts of parasite material, which is difficult to obtain, and the purification technique is costly and laborious. Studies have demonstrated that antibody levels become detectable at 4 weeks after infection and typically persist for many years after the infection has resolved (Garcia et al., 2001; Deckers et al., 2008), making it unsuitable for early diagnosis or as an indicator of an active infection. On the other hand, parasite antigen can be detected as soon as 2 weeks after infection (Arroyo et al., 2022a) and confirms the presence of live parasites, providing an immunological tool for discriminate active infections (Zea-Vera et al., 2013). However, sensitivity is usually lower than antibody test (90% versus 100%), and its detection relies on monoclonal antibodies (moabs) that are only genus-specific and cross-react with other Taenia species (Garcia et al., 1998; Bustos et al., 2019).
Despite these drawbacks, decades of study have positioned these two serological tests as the principal tools to support NCC diagnosis, and the interpretation of results has been studied in detail in different clinical scenarios (Parija and Gireesh, 2011). These serological tests are described in detail below.
Currently, EITB (Tsang et al., 1989) is the serological gold standard for NCC. The EITB detects specific antibodies against seven lentil-lectin purified glycoproteins (LLGP) named according to their molecular weight: GP50, GP42–39, GP24, GP21, GP18, GP14, and GP13. This test has an exceptional performance, nearly 100%, for more than two viable lesions, with reduced sensitivity in single or calcified lesions (Garcia et al., 2018a). Although a positive result can be determined by the response against any of these glycoproteins, diagnostic LLGP antigens used for antibody detection have specific functions and are classified into three protein families: GP50, T24, and 8 kDa. The GP50 family (GP50 band) is a glycosylphosphatidylinositol (GPI) related to membrane-anchoring function. The T24 family (GP42–39 and GP24 bands) is a transmembrane tetraspanin participating in processes of cell proliferation, adhesion, motility, and scaffolding. Lastly, the 8 kDa family (low molecular weight bands GP21, GP18, GP14, and GP13) consists of hydrophilic proteins lacking transmembrane regions, suggesting their involvement in secretion activity and evasion of the host immune response (Hancock et al., 2003; Hancock et al., 2004; Hancock et al., 2006; Rodriguez et al., 2012). Some of these small antigens from the 8 kDa family have demonstrated to share epitopes with antigenic regions of the T24 family (Rodriguez et al., 2012).
The natural progression of NCC infection involves different antibody responses represented as antibody banding patterns in the EITB. These patterns have been identified in clinical patients and animal models and associated to the cyst stage (viable or degenerated) (Arroyo et al., 2018). The study of the order of antibody appearance (or disappearance) has shown that, during the course of infection, the antibody pattern usually appears in a consecutive order, starting with the heaviest protein GP50 band and concluding with the smallest GP13 band (Deckers et al., 2008). The antibody pattern of disappearance during the cyst degeneration process could take years and occurs in the opposite order (from GP13 to GP50) (Garcia et al., 2018a) (Figure 1). When we studied the responses against each family individually, we showed that antibodies against GP50 are the first to appear and last the longest; these are present since exposure or at the initial stages and maintained after cyst resolution. Responses against the T24 family are heterogeneous. A strong immunodominance is characteristic of this protein family, allowing antibodies to persist for many years, as GP50; however, they can also be associated with viable and extraparenchymal infections. Responses against any band from the 8 kDa family are commonly related to active infections or high antigen burden (multiple or extraparenchymal NCC), being the first to disappear after cyst resolution (Garcia et al., 2012; Rodriguez et al., 2012; Arroyo et al., 2018).
Figure 1 (A) Enzyme-linked immunoelectrotransfer blot (EITB) appearance antibody pattern obtained using consecutive serum samples from a pig experimentally infected with cysticercosis. The position of the seven antibody bands and the names are shown. “A” indicates the basal EITB result (0b = 0 bands) before the infection, and “B” to “F” are the EITB results during the time that the pig was followed (5 months after infection). (B) EITB disappearance antibody pattern obtained using consecutive serum samples from a neurocysticercosis patient under antiparasitic treatment. “A” indicates the initial EITB result (7b = 7 bands), and “B” to “H” are the EITB results during the time that the patient was under treatment and the infection was finally resolved.
In conclusion, these strong associations between specific antibody responses and clinical aspects of the disease suggest that a careful interpretation of antibody patterns can provide important serological information for the diagnosis, characterization, and follow-up of NCC cases.
Another widely studied immunological test is the direct detection of parasite antigens using the antigen-enzyme-linked immunosorbent assay (Ag-ELISA). The Ag-ELISA represents a valuable tool for NCC diagnosis and follow-up as antigen levels are strongly associated with viability, parasite burden, and decrease rapidly after successful antiparasitic treatment (Deckers and Dorny, 2010; Rodriguez et al., 2012). The first Ag-ELISA based on monoclonal antibodies was initially developed for CSF samples using HP10 which used IgM moabs against a repetitive epitope from an excretory/secretory glycoprotein of Taenia saginata cysts, which cross-reacted with T. solium cysticercosis infection. Later, the performance in serum samples was compared to CSF, demonstrating to be similar by detecting between 96.5% to 80% of viable lesions and 40% of calcified lesions, with specificity of 94% (Fleury et al., 2013; Parkhouse et al., 2018).
Subsequently, the implementation of the B158/B60 Ag-ELISA significantly improved antigen detection. This test also relied on IgM moabs B158/B60 against an excretory/secretory product of 65 kDa from T. saginata metacestode (Draelants et al., 1995; Jansen et al., 2016). The B158/B60 Ag-ELISA was optimized by changing the moabs isotype to IgG, which resulted in overall sensitivity of 90% for living cysts and specificity of 98.7% for NCC (Dorny et al., 2003; Rodriguez et al., 2009; Gómez-Morales et al., 2017). The B158/B60 Ag-ELISA quickly emerged as the preferred and most widely used assay for antigen detection diagnosis in NCC, leading to the development of a commercial version [Cysticercosis Ag ELISA, ApDia, Belgium) with sensitivity of 98% (ELISA cysticercosis ag kit (REF.650501)]. Although these moabs are only genus-specific and cross-reacted with other Taenia species, it is a useful tool for the diagnosis of NCC since T. solium is the only species causing human cysticercosis (Castillo et al., 2023).
Many studies have focused on the association of antigen levels in NCC patients with different characteristics and clinical spectrums. Elevated antigen levels are associated with extraparenchymal (SANCC), viable multiple NCC, and patients with epilepsy, while negative or low antigen levels are found in calcified or single lesions (Gabriël et al., 2012; Garcia et al., 2012). Additionally, another important use of Ag-ELISA is for monitoring of treatment efficacy. The short longevity of released antigens results in a gradual decrease in antigen levels after antiparasitic treatment, serving as a predictor for successful therapy (Fleury et al., 2013; Rodríguez-Hidalgo et al., 2019). Therefore, measuring antigen levels by Ag-ELISA has been crucial in support diagnosis when the imaging results are unclear and has shown as a promising candidate for monitoring treatment efficacy.
EITB glycoproteins have been fully characterized, but their limited availability restricts their use. We have characterized and cloned homologous proteins and synthetic peptides of the three EITB diagnostic protein families (rGP50, rT24H, and peptides sTsRS1, sTs18var1, sTsRS2var1, and sTs14) (Greene et al., 2000; Hancock et al., 2003; Hancock et al., 2004; Hancock et al., 2006), providing advantages in accessibility and reproducibility for measuring specific antibody responses. These have been evaluated to demonstrate comparable performance to their native counterparts in the EITB (sensitivity 90%–100% and specificity 93%–100% for more than two viable cysts) (Handali et al., 2004; Bueno et al., 2005; Handali et al., 2010a; Lee et al., 2011a). A detailed review of the production and application of these glycoproteins was conducted in 2012 (Rodriguez et al., 2012), and since then, additional studies have developed new tools focusing on antigen rT24H. An ACEK POC immunosensor test (Liang et al., 2019), rT24H EITB (Dermauw et al., 2018; Stelzle et al., 2023), ELISA (Lee et al., 2011b), and the validation of an rT24H lateral flow assay (Handali et al., 2010b; Corstjens et al., 2014) have been reported.
As previously mentioned, the study of antibody patterns may enhance the understanding of the immune responses during NCC infection (Praet et al., 2010; Arroyo et al., 2022b). Therefore, simultaneous antibody response tests have also been developed, such as an immunoblot based on rGP50, rT24H, and sTsRS1 (Noh et al., 2014) and two multiantigen printing immunoassays (MAPIA) (Handali et al., 2010c; Toribio et al., 2023b), obtaining acceptable performance in viable and extraparenchymal NCC (over 90% of sensitivity). The easy handling and assembling of MAPIA represents an important advantage for large-scale assay production. The first MAPIA (Handali et al., 2010c) compared the performance of all six recombinant proteins, reaching 97% and 99% of sensitivity and specificity for patients with intra- and extra-parenchymal NCC. However, it was concluded that using all six recombinant antigens did not improve its sensitivity. More recently and highlighting the advantage that these proteins are now released from patent rights, an optimized MAPIA using only three antigens (rGP50, rT24H, and sTs14) of each protein diagnostic family was developed (Toribio et al., 2023b). It detected 100% of SANCC and 97.5% of parenchymal cases. Another approach based on the quantitative measurement of antibody responses is the development of a multiple triplex ELISA using rGP50, rT24H, and sTs18var3 (Tang et al., 2023), with an excellent concordance with the EITB (over 98% agreement), and a multiplex bead assay (MBA) using two forms of rT24H and recombinant Ts8B2 that showed high sensitivity in cases with two or more viable cysts (sensitivity of 96.1% and specificity of 96.5%) (Hernández-González et al., 2017; Moss et al., 2018).
A detailed study of the antibody responses against recombinant antigens in different NCC scenarios is needed to prove their potential contribution in diagnosis. In fact, measuring different antibody responses simultaneously and quantifying them should contribute enormously to the understanding of antibody pattern interpretation.
Some commercial kits for serum antibody detection have been developed (DRG™, RIDASCREEN™, NOVATECH™, CYPRESS™, and IVD™, among others) using native or less purified versions of total and vesicular antigen of metacestodes. Although they offer quantitative and rapid results, they have suboptimal sensitivity (under 72%) and specificity (~60%) (Gómez-Morales et al., 2017; Zammarchi et al., 2018; Hernández et al., 2019). One study that tested RIDASCREEN™ and NOVATECH™ showed a sensitivity rate lower than 44% in viable parenchymal lesions and <6.7% for calcified NCC and a specificity rate between 52.2% and 71.1%. Additionally, cross-reaction with hydatic disease and hymenolepiasis was confirmed (84.4% and 11.1%, respectively) (Chuang et al., 2017; Garcia et al., 2018b).
Various other purified and recombinant antigens have been developed in the past 13 years. These include crude soluble extract (Arora et al., 2020), purified antigens [others LLGP version (Ayala-Sulca and Miranda-Ulloa, 2015; Davelois et al., 2016), ag 52–53 kDa (Piña et al., 2011), cathepsin L (León-Janampa et al., 2019), TsAg5 (Rueda et al., 2011), LLa-Gp (Suzuki and Rossi, 2011), and Jacalin purified (MaChado et al., 2013)], excretory/secretory antigens, and lower molecular mass (10–30 kDa) (Atluri et al., 2009; Sako et al., 2015) using ELISA, Dot ELISA, and immunoblot techniques, but which have not been validated for its use in diagnosis. One of the most promising tests uses the recombinant Tsol-p27 with sensitivity above 85% and specificity of ~96% (Salazar-Anton et al., 2012; Nhancupe et al., 2016; Nhancupe et al., 2019). However, its initial description was not followed by further performance assessments. A detailed description of the developed immunological tests is shown in Table 1A.
Table 1A Principal immunological tests assessed in neurocysticercosis (NCC) patients for antibody and antigen detection in the last 13 years.
Table 1B Principal molecular tests assessed in neurocysticercosis (NCC) patients in the last 13 years.
In recent years, the reference test B158/B60 Ag-ELISA has been further studied and validated (Jansen et al., 2016) and extensively employed for routine clinical diagnosis and epidemiological studies (Moyano et al., 2014; Carod et al., 2021). Antigen detection with B158/B60 moabs has been improved using urine samples, providing a significant advantage as it is non-invasive.
One of the first reports in urine was conducted by our group in 2009 (Castillo et al., 2009), obtaining a sensitivity of 92% for viable intra- and extraparenchymal NCC. As expected, sensitivity decreased to 62.5% for patients with a single lesion and to 16.7% for calcified NCC. Since then, B158/B60 Ag-ELISA in urine has been used as a screening test, principally for SANCC detection in endemic communities. The positive predictive value for this ELISA was assessed as being 62% for SANCC and patients with high antigen levels (Ag OD ratio >3), also demonstrating that low antigen levels are poor predictors of SANCC (Mwape et al., 2011; Garvey et al., 2018; McCleery et al., 2020). The usefulness of antigen detection in urine for the early identification of NCC cases needs to be further studied (Sahu et al., 2014).
The use of monoclonal antibodies (moabs) for antigen detection was initially performed using HP10 moabs, followed by B158/B60 moabs, both produced against T. saginata. After decades, new T. solium moabs have been developed by our group. A set of 21 clones of moabs against whole cyst (TsW1–13), vesicular fluid (TsV1–5), and excretory/secretory antigens (TsE1–3) was developed, and eight of them were able to detect antigens in body fluids (CSF, serum, and urine). A novel Ag-ELISA was later standardized using moabs TsW8 as the capture antibody and TsW5 as the detection antibody, both directed against whole cyst antigens. The initial report showed a cross-reaction of these moabs with T. saginata antigens (Paredes et al., 2016). Although none of them were reactive against Fasciola hepatica, Echinococcus granulosus, and Taenia hydatigena, a recent study reported the cross-reaction of T. hydatigena antigens in pigs (Paredes et al., 2016; Arroyo et al., 2024).
Recently, one study (Castillo et al., 2023) compared TsW8/TsW5 Ag-ELISA performance against the B158/B60 Ag-ELISA. Both assays demonstrated high levels of agreement (over 97%) in serum from patients with subarachnoid, parenchymal, and calcified NCC, suggesting a new role for TsW8/TsW5 in accurately detecting antigen levels in serum samples. Moreover, ongoing studies are currently assessing the utility of TsW8/TsW5 Ag-ELISA to accurately detect antigen in serum and in urine.
Nanobodies have been established as promising tools for antigen detection due to their easy handling and high stability. However, only one report (2009) about nanobodies against an 8-kDa antigen (sTs14) was published (Deckers et al., 2009), and no further evaluations have been conducted since then. Single-chain variable fragment (scFv), produced by phage display against T. solium total saline extraction, has been reported (Ribeiro Vda et al., 2013). The three scFv antibodies (G10, A4, and B6) presented a sensitivity rate of 97% for NCC patients (NCC type was not mentioned) and a specificity rate of 95%. Similarly, a novel recombinant moab (TsG10) has been developed from previous IgM-secreting hybridomas (Paredes et al., 2016); its use could decrease the cost of moabs production (Corda et al., 2022). Performance was assessed in serum, plasma, and CSF from patients with extraparenchymal NCC, achieving a sensitivity rate of 98% and a specificity rate of 100%. These promising results may encourage the use of these more affordable antibody options to detect circulating antigen.
Finally, the use of TsW8/TsW5 moabs in urine in a point-of-care (POC) test format detected 97% of extraparenchymal NCC patients with a specificity rate of 100% (Toribio et al., 2023a). This rapid test can be performed in 15 min in urine samples and constitutes a valuable tool for rural settings. Studies are being carried out to determine the performance of this rapid test and to validate its use as a screening tool in low-resource areas.
Since the introduction of the PCR assay, molecular diagnosis has assumed a crucial role in diagnosing diseases where conventional immunological tests fall short. PCR offers significant advantages over immunological tests, increasing its sensitivity and specificity by amplifying very low quantities of specific parasite DNA sequences (Li et al., 2011). Several PCR tests have been developed for the detection of T. solium DNA, primarily for phylogeny and taxonomy analysis or its direct use for confirming T. solium infection in pig carcasses (Ramahefarisoa et al., 2010; Sreedevi et al., 2012; Gauci et al., 2019; Satyaprakash et al., 2023).
More recently, the detection of circulating cell-free parasite DNA fragments (cfDNA) has been explored. cfDNA comprises small nuclear or mitochondrial DNA fragments released from cells into body fluids; the origin and mechanisms of DNA release are not fully understood but are associated with cell death pathways and active secretion. cfDNA molecules are released as small fragments from 50 to 400 bp in urine, after they have been cleared in the kidney by the glomerular barrier (Toribio et al., 2020; Diao et al., 2022; Lozano et al., 2023). Detection of cfDNA offers a convenient sample handling due to its stability and, more importantly, eliminates the dependence on parasite material for its development by using commercially synthetic primers.
In NCC diagnosis, cfDNA was first detected in CSF, recognizing a repetitive sequence of the T. solium genome (pTsol9) in 29/30 NCC cases (95.9%), including parenchymal, subarachnoid, and calcified lesions (Almeida et al., 2006). Subsequent studies developed other PCR assays targeting the same (Toribio et al., 2019; Goyal et al., 2020a) or different sequences (Michelet et al., 2011) in CSF, blood, and urine samples. Besides pTsol9 sequence, another promising target is a repetitive sequence (TsolR13), which was tested in a quantitative assay (qPCR) obtaining a sensitivity rate of 100% for extraparenchymal NCC diagnosis (Michelet et al., 2011; O’Connell et al., 2020). Moreover, one POC-test based on loop-isothermal amplification (LAMP) directed against mitochondrial gene COX1 was also developed, obtaining a sensitivity rate of 71.8% and 86.7% for parenchymal and extraparenchymal NCC, respectively (see Table 1B) (Goyal et al., 2020b).
This literature review of new immunological and molecular diagnostic tools is focused only on NCC diagnosis. As members of one of the most productive cysticercosis research groups, we have explained in detail our contributions, as well as some others, to the NCC diagnosis. Major advances in clinical laboratory diagnostic technologies (development of recombinant antigens, new monoclonal antibodies, and the exploration of the molecular diagnosis) have resulted in high-quality, safe, and effective clinical decision tools. However, NCC diagnosis continues to face challenges, including its limited accessibility in many scenarios (Mlowe et al., 2022). In this final section, we propose possible solutions for diagnostic challenges using new tests and future lines of research.
NCC diagnosis is challenging due to the clinical heterogeneity of the immunological response and the variability of antigen/antibody expression during different stages of the disease (Ito, 2013; Ostoa-Saloma et al., 2013; Hossain et al., 2023). Additionally, available diagnostic tests are limited by their low sensitivity in some NCC forms and difficult accessibility (Mlowe et al., 2022; Takayanagui and Haes, 2022). Here we propose the use of new laboratory tests to address these specific scenarios of NCC:
These lesions represent a major challenge in diagnosis due to two principal reasons: neuroimaging commonly confuses them with other pathologies, and they are underdiagnosed by the traditional immunological assays because of their low levels of antigen/antibody responses. More sensitive assays can contribute to improve single lesion detection. One strategy to address this challenge is the simultaneous detection of various antibodies to enhance the sensitivity using our new MAPIA with higher antigen concentrations of recombinant antigens to capture lower levels of antibody responses or by taking advantage of the high capacity of beads to capture antibodies in their spherical surface used in the triplex ELISA or the multiplex bead assay (Hernández-González et al., 2022).
Another alternative recently explored is the use of gene expression from monocytes to differentiate NCC single parenchymal lesion from brain tuberculomas. The expression of NCC-associated monocyte genes (TAX1BP1, RAP1A, PLCG2, TOR3A, GBP1P1, LRRFIP2, and FEZ2) has a sensitivity rate of 87.2% and a specificity rate of 100% (combination of TAX1BP1-RAP1A and GBP1P1 genes) in single lesions (Pamela et al., 2021).
A prompt detection of extraparenchymal NCC cases, especially SANCC, is crucial, as it is the most severe form of NCC and the one with the worst prognosis. Recent studies in endemic areas of Peru have detected an unexpectedly higher prevalence of SANCC in asymptomatic villagers (Mahale et al., 2015). Priority should be its rapid identification through practical immunological tests, followed by imaging diagnosis for confirmation to provide an adequate clinical management. Since these patients release great quantities of antigens into circulation, the approach would be the use of a reliable and practical test with high specificity, capable of discriminating active forms of this severe case of NCC to be applied as a screening test especially in rural settings. The Ag-ELISA in urine can be useful (Gabriël et al., 2012) to determine antigen levels in a quantitative manner; however, for mass screening, interventions may result as impractical and costly. Our new POC assay, based on moabs TsW8/TsW5, could also serve as an affordable and practical tool to quickly identify active SANCC cases in non-invasive samples to be later referred for imaging confirmation and specialized care.
Post-treatment follow-up is essential to assess the effectiveness of antiparasitic treatment and make clinical decisions. Ideally, neuroimaging studies would be employed for this purpose, but they are expensive and logistically impractical (Hamamoto Filho et al., 2022). The strategy here should be the use of quantitative tests, such as Triplex ELISA, Ag-ELISA (Zea-Vera et al., 2013; Rodríguez-Hidalgo et al., 2019) or multiplex beads assay to evaluate antigen/antibody levels in samples collected from patients undergoing antiparasitic treatment. Urine collection is non-invasive and painless to collect, providing an easy-to-access sample. Studying the antigen/antibody dynamics in response to treatment and a simultaneous identification of one or a set of biomarkers that rapidly change in response to alterations in parasite viability could define an earlier time point for confirming treatment success, reducing costs and the period of uncertainty for patients under treatment (Hamamoto Filho et al., 2022). Lastly, quantitative PCR has been reported for the detection and follow-up of NCC patients, constituting a possible new tool for monitoring treatment efficacy (Rottbeck et al., 2013).
Regarding new molecular diagnostic tests, exploring the potential of cfDNA detection could be promising to support NCC diagnosis in specific scenarios and for monitoring treatment efficacy. DNA amplification should increase the sensitivity, making it a good candidate to detect single lesions with lower antibody/antigen. Moreover, detection in non-invasive samples, the easy handling of cfDNA, and accessible synthesis for its development could benefit a screening application. The limitations of DNA assays need to be further explored; we anticipate that could be the same as immunological assays, being the difficulty to differentiate muscular cysticercosis from NCC since cfDNA is actively released from cysts in any location. DNA technologies are usually expensive, limiting its application in a large scale. However, some rapid and affordable techniques have been mentioned (such as LAMP). In addition, some reports have demonstrated the possibility to detect DNA in a significant proportion of calcified NCC cases using PCR (around 40%), not allowing an adequate discrimination of active and inactive NCC cases.
Future directions for NCC diagnosis should focus on enhancing the accessibility, reliability, and efficiency of current and new diagnostic tools. An adequate validation of methods is critical to ensure their effectiveness in different scenarios and to help the clinical practice (Gjorup, 1997; Ito, 2013; Cardona-Arias et al., 2017).
Accessibility to immunological tests was improved with the use of recombinant forms of the EITB glycoproteins (rGP50 and rT24, sTSRS1, sTS18var1, sTS18var1, sTSRS2var1, and sTs14); their easier production reduces costs and improves assay reproducibility. Molecular diagnosis based on synthetic primers can also be accessible for any laboratory. Hence, its use should be exploited and applied to more studies (Esquivel-Velázquez et al., 2011).
The reliability of an assay is determined by a combination of factors, including accuracy, precision, consistency, sensitivity, specificity, and reproducibility (Bolarinwa, 2015; Gómez-Morales et al., 2017; Ramachandran and Wilson, 2018). Methods for evaluating this depend on the assay type and the analyte, but general guidelines can be followed. To evaluate accuracy, the use of reference standards and controls with known values (or concentrations) must be implemented. Precision can be assessed by measuring inter- and intra-assay variations (Manterola et al., 2018). In the same way, consistency and robustness are evaluated by determining the tolerance of the test to environmental variations (temperature, pH, and others). Finally, reproducibility can be ensured through participation in collaborative studies or ring trials with other institutions (Jansen et al., 2016; Gómez-Morales et al., 2021).
Assessing sensitivity and specificity is crucial to define test performance and for an adequate validation. The best way to determine them is evaluating a well-defined set of samples in a ROC curve analysis (Jayashi et al., 2014). Here we proposed a practical manner for defining NCC groups based on its immunological response (Garcia et al., 2014), including extraparenchymal (SANCC), multiple parenchymal with severe (>10 cysts), moderate (five to 10 cysts), mild (three to five cysts), low infection (one to three cysts), and single lesions. On the other hand, for specificity, a large group of appropriate negative controls must be tested. It is also important to mention that the establishment of the cutoff point should be flexible and aligned with the study aim—for example, for screening tests focused on the detection of severe cases with a high immunological response, specificity should be maximized, while for identifying single brain lesions, sensitivity must be prioritized over specificity.
With the diagnostic tools currently available and their standardized validation, it will be possible to implement one or more rapid, accurate, and worldwide-accessible diagnostic tests for the early and accurate detection of NCC, both in clinical and in field conditions, as well as tools for monitoring treatment success. It is clear that diagnosis cannot rely only on laboratory tests but must adequately support the imaging findings and guide the clinician for appropriate clinical decisions. A rigorous validation of new tests could facilitate the decision-making regarding the implementation (or not) of these tests in the laboratory, and a careful interpretation of results should be implemented to update the global scheme of diagnosis criteria.
LT: Formal analysis, Investigation, Methodology, Project administration, Writing – original draft, Writing – review & editing. JB: Data curation, Resources, Supervision, Writing – original draft, Writing – review & editing. HG: Conceptualization, Data curation, Funding acquisition, Resources, Supervision, Writing – original draft, Writing – review & editing.
The author(s) declare financial support was received for the research, authorship, and/or publication of this article. All these years of research for improving NCC diagnosis were possible through the funding provided by NIAID-NIH grants U19AI129909, R01AI150544, and R01AI143553 and FIC-NIH grant D43TW001140.
The authors would like to express their sincere appreciation to the laboratory and clinical personnel of the Cysticercosis Unit (Erika Perez, Karen Arteaga, Monica Vera, Nancy Puchuri, Dr. Isidro Gonzales, Dr. Herbert Saavedra, and Dr. Carolina Guzman) at the Instituto de Ciencias Neurologicas, the Laboratory of Parasite Immunology (Yesenia Castillo and Cindy Espinoza), the Laboratory of Neurocysticercosis at the Universidad Peruana Cayetano Heredia for their hard work during all these years in processing EITB and Ag-ELISA and to the Center for Global Health in Tumbes (Dr. Seth O'Neal and staff). We are grateful to the Center for Disease Control and Prevention (CDC) staff (Dr. Patricia Wilkins, Dr. Sukwan Handali, and Dr. John Noh) as well as the MMI department of Johns Hopkins University (Dr. Clive Shiff and Dr. Alan Scott) and to Dr. Theodore Nash from the National Institute of Allergy and Infectious Diseases, for their collaboration and guidance in the development of new diagnostic tests. This review is a tribute to the lasting influence of Dr. Silvia Rodriguez, whose studies in improvements on NCC immunodiagnosis laid the foundation for this study. Finally, special thanks to all the members of the Cysticercosis Group in Peru (CWGP).
The authors declare that the research was conducted in the absence of any commercial or financial relationships that could be construed as a potential conflict of interest.
The author(s) declared that they were an editorial board member of Frontiers, at the time of submission. This had no impact on the peer review process and the final decision.
All claims expressed in this article are solely those of the authors and do not necessarily represent those of their affiliated organizations, or those of the publisher, the editors and the reviewers. Any product that may be evaluated in this article, or claim that may be made by its manufacturer, is not guaranteed or endorsed by the publisher.
Abanto J., Blanco D., Saavedra H., Gonzales I., Siu D., Pretell E. J., et al. (2021). Mortality in parenchymal and subarachnoid neurocysticercosis. Am. J. Trop. Med. Hyg 105 (1), 176–180. doi: 10.4269/ajtmh.20-1330
Almeida C. R., Ojopi E. P., Nunes C. M., Machado L. R., Takayanagui O. M., Livramento J. A., et al. (2006). Taenia solium DNA is present in the cerebrospinal fluid of neurocysticercosis patients and can be used for diagnosis. Eur. Arch. Psychiatry Clin. Neurosci. 256 (5), 307–310. doi: 10.1007/s00406-006-0612-3 doi: 10.1016/j.actatropica.2017.11.005
Arora N., Kaur R., Rawat S. S., Kumar A., Singh A. K., Tripathi S., et al. (2020). Evaluation of taenia solium cyst fluid-based enzyme linked immunoelectro transfer blot for neurocysticercosis diagnosis in urban and highly endemic rural population of north india. Clin. Chim. Acta 508, 16–21. doi: 10.1186/s13071-023-05989-6 doi: 10.1016/j.cca.2020.05.006
Arroyo G., Bustos J. A., Lescano A. G., Gonzales I., Saavedra H., Pretell E. J., et al. (2022b). Improved diagnosis of viable parenchymal neurocysticercosis by combining antibody banding patterns on enzyme-linked immunoelectrotransfer blot (EITB) with antigen enzyme-linked immunosorbent assay (ELISA). J. Clin. Microbiol. 60 (2), e0155021. doi: 10.3389/fneur.2023.1173909 doi: 10.1128/jcm.01550-21
Arroyo G., Rodriguez S., Lescano A. G., Alroy K. A., Bustos J. A., Santivanez S., et al. (2018). Antibody banding patterns of the enzyme-linked immunoelectrotransfer blot and brain imaging findings in patients with neurocysticercosis. Clin. Infect. Dis. 66 (2), 282–288. doi: 10.1093/cid/cix774
Arroyo G., Toribio L., Garrido S., Chile N., Lopez-Urbina T., Gomez-Puerta L. A., et al. (2024). Concordance between two monoclonal antibody-based antigen detection enzyme-linked immunosorbent assays for measuring cysticercal antigen levels in sera from pigs experimentally infected with taenia solium and taenia hydatigena. Parasit Vectors 17 (1), 172. doi: 10.1016/j.pt.2016.09.003 doi: 10.1186/s13071-024-06197-6
Arroyo G., Toribio L., Vargas-Calla A., Calcina J. F., Bernal E., Chile N., et al. (2022a). Porcine model of neurocysticercosis by intracarotid injection of taenia solium oncospheres: Dose assessment, infection outcomes and serological responses. PloS Negl. Trop. Dis. 16 (6), e0010449. doi: 10.1371/journal.pntd.0010449 doi: 10.1371/journal.pntd.0010442
Atluri S. R., Singhi P., Khandelwal N., Malla N. (2009). Neurocysticercosis immunodiagnosis using taenia solium cysticerci crude soluble extract, excretory secretory and lower molecular mass antigens in serum and urine samples of indian children. Acta Trop. 110 (1), 22–27. doi: 10.1016/j.actatropica.2008.12.004
(2023). ELISA cysticercosis ag kit (REF.650501) (ApDia Group). Available at: https://apdiagroup.com/we-sell/elisa/elisa-apdia/cysticercosis-antigen/.
Ayala-Sulca E., Miranda-Ulloa E. (2015). [Evaluation of enzyme-linked immunoelectrotransfer blot test using purified native antigen mix from cisticercus fluid of taenia solium for diagnosis of human cysticercosis]. Rev. Peru Med. Exp. Salud Publica 32 (3), 485–491. doi: 10.1016/j.vetpar.2016.07.027 doi: 10.17843/rpmesp.2015.323.1678
Bolarinwa O. A. (2015). Principles and methods of validity and reliability testing of questionnaires used in social and health science researches. Niger Postgrad Med. J. 22 (4), 195–201. doi: 10.4103/1117-1936.173959
Bueno E. C., Scheel C. M., Vaz A. J., Machado L. R., Livramento J. A., Takayanagui O. M., et al. (2005). Application of synthetic 8-kD and recombinant GP50 antigens in the diagnosis of neurocysticercosis by enzyme-linked immunosorbent assay. Am. J. Trop. Med. Hyg 72 (3), 278–283. doi: 10.4269/ajtmh.2005.72.278
Bustos J. A., Ninaquispe B. E., Rodriguez S., Castillo Y., Yang S. Y., Gilman R. H., et al. (2019). Performance of a sandwich antigen-detection ELISA for the diagnosis of porcine taenia solium cysticercosis. Am. J. Trop. Med. Hyg 100 (3), 604–608. doi: 10.4269/ajtmh.18-0697
Butala C., Brook T. M., Majekodunmi A. O., Welburn S. C. (2021). Neurocysticercosis: Current perspectives on diagnosis and management. Front. Vet. Sci. 8, 615703. doi: 10.3389/fvets.2021.615703
Cárdenas G., Salgado P., Laura-Foronda E., Popoca-Rodriguez I., Delgado-Hernández R. D., Rojas R., et al. (2021). Neglected and (re-)emergent infections of the CNS i n low-/middle-income countries. Infez Med. 29 (4), 513–525.
Cardona-Arias J. A., Carrasquilla-Agudelo Y. E., Restrepo-Posada D. C. (2017). [Validity of three methods for inmuno-diagnostic of neurocysticercosis: systematic review of the literature with meta-analysis 1960-2014]. Rev. Chil. Infectol 34 (1), 33–44. doi: 10.4067/S0716-10182017000100005
Carod J. F., Mauny F., Parmentier A. L., Desmarets M., Rakotondrazaka M., Brembilla A., et al. (2021). Hyperendemicity of cysticercosis in madagascar: Novel insights from school children population-based antigen prevalence study. PloS One 16 (10), e0258035. doi: 10.1371/journal.pone.0258035
Carrara G. M. P., Silva G. B., Faria L. S., Nunes D. S., Ribeiro V. S., Lopes C. A., et al. (2020). IgY antibody and human neurocysticercosis: a novel approach on immunodiagnosis using taenia crassiceps hydrophobic antigens. Parasitology 147 (2), 240–247. doi: 10.1017/S0031182019001446
Carvalho Junior R. M., Costa D. L., Soares S. C., Costa C. H. (2010). Evaluation of an enzyme immunoassay for clinical diagnosis of neurocysticercosis in symptomatic patients. Rev. Soc. Bras. Med. Trop. 43 (6), 647–650. doi: 10.1590/S0037-86822010000600009
Castillo Y., Rodriguez S., García H. H., Brandt J., Van Hul A., Silva M., et al. (2009). Urine antigen detection for the diagnosis of human neurocysticercosis. Am. J. Trop. Med. Hyg 80 (3), 379–383. doi: 10.4269/ajtmh.2009.80.379
Castillo Y., Toribio L. M., Guzman C., Arroyo G., Espinoza C., Saavedra H., et al. (2023). Consistent measurement of parasite-specific antigen levels in sera of patients with neurocysticercosis using two different monoclonal antibody (mAb)-based enzyme-linked immunosorbent assays. Pathogens 12 (4). doi: 10.3390/pathogens12040566
Channel I. C., Damara F. A., Ramdhani A. N., Anton A. (2021). Letter to the editor. diagnosis of subarachnoid neurocysticercosis. Neurosurg. Focus 50 (5), E24.
Chuang C., Xing-Wang C., Jian-Zhong D., Tiao-Ying L. (2017). [Evaluation of ELISA kit for detection of serum specific IgG antibodies against taenia solium in diagnosis of human cysticercosis]. Zhongguo Xue Xi Chong Bing Fang Zhi Za Zhi 29 (2), 228–230.
Corda M., Sciurba J., Blaha J., Mahanty S., Paredes A., Garcia H. H., et al. (2022). A recombinant monoclonal-based taenia antigen assay that reflects disease activity in extra-parenchymal neurocysticercosis. PloS Negl. Trop. Dis. 16 (5), e0010442. doi: 10.1371/journal.pntd.0010449 doi: 10.1371/journal.pntd.0010442
Corstjens P. L., de Dood C. J., Priest J. W., Tanke H. J., Handali S. (2014). Feasibility of a lateral flow test for neurocysticercosis using novel up-converting nanomaterials and a lightweight strip analyzer. PloS Negl. Trop. Dis. 8 (7), e2944. doi: 10.1371/journal.pntd.0002944
Davelois K., Escalante H., Jara C. (2016). [Western blot diagnostic yield for simultaneous antibody-detection in patients with human cysticercosis, hydatidosis, and human fascioliasis]. Rev. Peru Med. Exp. Salud Publica 33 (4), 616–624. doi: 10.17843/rpmesp.2016.334.2543
Deckers N., Dorny P. (2010). Immunodiagnosis of taenia solium taeniosis/cysticercosis. Trends Parasitol. 26 (3), 137–144. doi: 10.1016/j.pt.2009.12.008
Deckers N., Kanobana K., Silva M., Gonzalez A. E., Garcia H. H., Gilman R. H., et al. (2008). Serological responses in porcine cysticercosis: a link with the parasitological outcome of infection. Int. J. Parasitol. 38 (10), 1191–1198. doi: 10.1016/j.ijpara.2008.01.005
Deckers N., Saerens D., Kanobana K., Conrath K., Victor B., Wernery U., et al. (2009). Nanobodies, a promising tool for species-specific diagnosis of taenia solium cysticercosis. Int. J. Parasitol. 39 (5), 625–633. doi: 10.1016/j.ijpara.2008.10.012
Del Brutto O. H. (2012). Diagnostic criteria for neurocysticercosis, revisited. Pathog. Glob Health 106 (5), 299–304. doi: 10.1179/2047773212Y.0000000025
Del Brutto O. H. (2022). Human neurocysticercosis: An overview. Pathogens 11 (10). doi: 10.3390/pathogens11101212
Dermauw V., Carabin H., Cissé A., Millogo A., Tarnagda Z., Ganaba R., et al. (2018). Evaluating the recombinant T24H enzyme-linked immunoelectrotransfer blot assay for the diagnosis of neurocysticercosis in a panel of samples from a large community-based randomized control trial in 60 villages in burkina faso. Am. J. Trop. Med. Hyg 98 (2), 565–569. doi: 10.4269/ajtmh.17-0541
Diao Z. L., Zhang R., Li J. M. (2022). [Analysis of the methods and quality assurance of metagenomic next-generation sequencing to detect the microbial cfDNA from blood samples in china]. Zhonghua Yi Xue Za Zhi 102 (15), 1114–1118. doi: 10.1093/cid/cix774
Dixon M. A., Winskill P., Harrison W. E., Basáñez M. G. (2021). Taenia solium taeniasis/cysticercosis: From parasite biology and immunology to diagnosis and control. Adv. Parasitol. 112, 133–217. doi: 10.1016/bs.apar.2021.03.003
Dorny P., Brandt J., Zoli A., Geerts S. (2003). Immunodiagnostic tools for human and porcine cysticercosis. Acta Trop. 87 (1), 79–86. doi: 10.1016/S0001-706X(03)00058-5
Draelants E., Hofkens E., Harding E., Brandt J., Geerts S. (1995). Development of a dot-enzyme immunoassay for the detection of circulating antigen in cattle infected with taenia saginata cysticerci. Res. Vet. Sci. 58 (1), 99–100. doi: 10.1016/0034-5288(95)90098-5
Esquivel-Velázquez M., Ostoa-Saloma P., Morales-Montor J., Hernández-Bello R., Larralde C. (2011). Immunodiagnosis of neurocysticercosis: ways to focus on the challenge. J. BioMed. Biotechnol. 2011, 516042. doi: 10.1155/2011/516042
Fleury A., Garcia E., Hernández M., Carrillo R., Govezensky T., Fragoso G., et al. (2013). Neurocysticercosis: HP10 antigen detection is useful for the follow-up of the severe patients. PloS Negl. Trop. Dis. 7 (3), e2096. doi: 10.1371/journal.pntd.0002096
Gabriël S., Blocher J., Dorny P., Abatih E. N., Schmutzhard E., Ombay M., et al. (2012). Added value of antigen ELISA in the diagnosis of neurocysticercosis in resource poor settings. PloS Negl. Trop. Dis. 6 (10), e1851. doi: 10.1371/journal.pntd.0001851
Garcia H. H., Castillo Y., Gonzales I., Bustos J. A., Saavedra H., Jacob L., et al. (2018b). Low sensitivity and frequent cross-reactions in commercially available antibody detection ELISA assays for taenia solium cysticercosis. Trop. Med. Int. Health 23 (1), 101–105. doi: 10.1111/tmi.13010
Garcia H. H., Gonzalez A. E., Gilman R. H., Palacios L. G., Jimenez I., Rodriguez S., et al. (2001). Short report: transient antibody response in taenia solium infection in field conditions-a major contributor to high seroprevalence. Am. J. Trop. Med. Hyg 65 (1), 31–32. doi: 10.4269/ajtmh.2001.65.31
Garcia H. H., O'Neal S. E., Noh J., Handali S., Cysticercosis Working Group in, P. (2018a). Laboratory diagnosis of neurocysticercosis (Taenia solium). J. Clin. Microbiol. 56 (9). doi: 10.1128/JCM.00424-18
Garcia H. H., Rodriguez S., Friedland J. S. (2014). Immunology of taenia solium taeniasis and human cysticercosis. Parasite Immunol. 36 (8), 388–396. doi: 10.1111/pim.12126
Garcia H. H., Rodriguez S., Gilman R. H., Gonzalez A. E., Tsang V. C., Cysticercosis Working Group in, P. Neurocysticercosis: is serology useful in the absence of brain imaging? Trop. Med. Int. Health 17 (8), 1014–1018. doi: 10.1111/j.1365-3156.2012.03037.x
Garcia H. H., Tsang V. C., Gilman R. H. (1998). A high degree of cross reactivity between the antigens of taenia solium and taenia crassiceps, potentially useful for the diagnosis of human neurocysticercosis (NCC). Am. J. Trop. Med. Hyg 58 (6), 693–694. doi: 10.4269/ajtmh.1998.58.693
Garvey B. T., Moyano L. M., Ayvar V., Rodriguez S., Gilman R. H., Gonzalez A. E., et al. (2018). Neurocysticercosis among people living near pigs heavily infected with cysticercosis in rural endemic peru. Am. J. Trop. Med. Hyg 98 (2), 558–564. doi: 10.4269/ajtmh.17-0443
Gauci C. G., Ayebazibwe C., Nsadha Z., Rutebarika C., Poudel I., Sah K., et al. (2019). Accurate diagnosis of lesions suspected of being caused by taenia solium in body organs of pigs with naturally acquired porcine cysticercosis. PloS Negl. Trop. Dis. 13 (6), e0007408. doi: 10.1371/journal.pntd.0007408
Gilman R. H. (2016). Infectious disease: Diagnostic criteria for neurocysticercosis - a difficult update. Nat. Rev. Neurol. 12 (10), 560–561. doi: 10.1038/nrneurol.2016.145
Gómez-Morales M. A., Gárate T., Blocher J., Devleesschauwer B., Smit G. S. A., Schmidt V., et al. (2017). Present status of laboratory diagnosis of human taeniosis/cysticercosis in europe. Eur. J. Clin. Microbiol. Infect. Dis. 36 (11), 2029–2040. doi: 10.1007/s10096-017-3029-1
Gómez-Morales M., Pezzotti P., Ludovisi A., Boufana B., Dorny P., Kortbeek T., et al. (2021). Collaborative studies for the detection of taenia spp. infections in humans within CYSTINET, the european network on Taeniosis/Cysticercosis. Microorganisms 9 (6).
Goyal G., Phukan A. C., Hussain M., Lal V., Modi M., Goyal M. K., et al. (2020a). Identification of taenia solium DNA by PCR in blood and urine samples from a tertiary care center in north india. J. Neurol. Sci. 417, 117057. doi: 10.1016/j.jns.2020.117057
Goyal G., Phukan A. C., Hussain M., Lal V., Modi M., Goyal M. K., et al. (2020b). Sorting out difficulties in immunological diagnosis of neurocysticercosis: Development and assessment of real time loop mediated isothermal amplification of cysticercal DNA in blood. J. Neurol. Sci. 408, 116544. doi: 10.1016/j.jns.2019.116544
Greene R. M., Hancock K., Wilkins P. P., Tsang V. C. (2000). Taenia solium: molecular cloning and serologic evaluation of 14- and 18-kDa related, diagnostic antigens. J. Parasitol. 86 (5), 1001–1007. doi: 10.1645/0022-3395(2000)086[1001:TSMCAS]2.0.CO;2
Haddad N., Shaheen Y., Abunaib M., Melikyan G., El Sotouhy A., Wahbeh F., et al. (2023). Neurocysticercosis in non-endemic regions: The experience of qatar. Front. Neurol. 14, 1173909. doi: 10.3389/fneur.2023.1173909 doi: 10.1128/jcm.01550-21
Hamamoto Filho P. T., Rodríguez-Rivas R., Fleury A. (2022). Neurocysticercosis: A review into treatment options, indications, and their efficacy. Res. Rep. Trop. Med. 13, 67–79.
Hancock K., Khan A., Williams F. B., Yushak M. L., Pattabhi S., Noh J., et al. (2003). Characterization of the 8-kilodalton antigens of taenia solium metacestodes and evaluation of their use in an enzyme-linked immunosorbent assay for serodiagnosis. J. Clin. Microbiol. 41 (6), 2577–2586. doi: 10.1128/JCM.41.6.2577-2586.2003
Hancock K., Pattabhi S., Greene R. M., Yushak M. L., Williams F., Khan A., et al. (2004). Characterization and cloning of GP50, a taenia solium antigen diagnostic for cysticercosis. Mol. Biochem. Parasitol. 133 (1), 115–124. doi: 10.1016/j.molbiopara.2003.10.001
Hancock K., Pattabhi S., Whitfield F. W., Yushak M. L., Lane W. S., Garcia H. H., et al. (2006). Characterization and cloning of T24, a taenia solium antigen diagnostic for cysticercosis. Mol. Biochem. Parasitol. 147 (1), 109–117. doi: 10.1016/j.molbiopara.2006.02.004
Handali S., Gonzalez A. E., Hancock K., Garcia H. H., Roberts J. M., Gilman R. H., et al. (2004). Porcine antibody responses to taenia solium antigens rGp50 and sTs18var1. Am. J. Trop. Med. Hyg 71 (3), 322–326. doi: 10.4269/ajtmh.2004.71.322
Handali S., Klarman M., Gaspard A. N., Dong X. F., Laborde R., Noh J., et al. (2010b). Development and evaluation of a magnetic immunochromatographic test to detect taenia solium, which causes taeniasis and neurocysticercosis in humans. Clin. Vaccine Immunol. 17 (4), 631–637. doi: 10.1128/CVI.00511-09
Handali S., Klarman M., Gaspard A. N., Noh J., Lee Y. M., Rodriguez S., et al. (2010c). Multiantigen print immunoassay for comparison of diagnostic antigens for taenia solium cysticercosis and taeniasis. Clin. Vaccine Immunol. 17 (1), 68–72. doi: 10.1128/CVI.00339-09
Handali S., Pattabhi S., Lee Y. M., Silva-Ibanez M., Kovalenko V. A., Levin A. E., et al. (2010a). Development and evaluation of porcine cysticercosis QuickELISA in triturus EIA analyzer. J. Immunoassay Immunochem 31 (1), 60–70.
Hernández M., Astudillo O. G., Diego G., de-la-Rosa-Arana J. L., Meza-Lucas A., García-Rodea R., et al. (2019). Immunodiagnosis of human neurocysticercosis: comparative performance of serum diagnostic tests in mexico. Parasitol. Res. 118 (10), 2891–2899. doi: 10.1007/s00436-019-06425-4
Hernández-González A., González-Bertolín B., Urrea L., Fleury A., Ferrer E., Siles-Lucas M., et al. (2022). Multiple-bead assay for the differential serodiagnosis of neglected human cestodiases: Neurocysticercosis and cystic echinococcosis. PloS Negl. Trop. Dis. 16 (1), e0010109. doi: 10.1371/journal.pntd.0010109
Hernández-González A., Noh J., Perteguer M. J., Gárate T., Handali S. (2017). Comparison of T24H-his, GST-T24H and GST-Ts8B2 recombinant antigens in western blot, ELISA and multiplex bead-based assay for diagnosis of neurocysticercosis. Parasit Vectors 10 (1), 237. doi: 10.1186/s13071-017-2160-2
Hossain M. S., Shabir S., Toye P., Thomas L. F., Falcone F. H. (2023). Insights into the diagnosis, vaccines, and control of taenia solium, a zoonotic, neglected parasite. Parasit Vectors 16 (1), 380. doi: 10.1186/s13071-023-05989-6 doi: 10.1016/j.cca.2020.05.006
Ito A. (2013). Nothing is perfect! trouble-shooting in immunological and molecular studies of cestode infections. Parasitology 140 (13), 1551–1565. doi: 10.1017/S0031182013000966
Jansen F., Dorny P., Berkvens D., Van Hul A., Van den Broeck N., Makay C., et al. (2016). Assessment of the repeatability and border-plate effects of the B158/B60 enzyme-linked-immunosorbent assay for the detection of circulating antigens (Ag-ELISA) of taenia saginata. Vet. Parasitol. 227, 69–72. doi: 10.1016/j.vetpar.2016.07.027 doi: 10.17843/rpmesp.2015.323.1678
Jayashi C. M., Gonzalez A. E., Castillo Neyra R., Rodríguez S., García H. H., Lightowlers M. W. (2014). Validity of the enzyme-linked immunoelectrotransfer blot (EITB) for naturally acquired porcine cysticercosis. Vet. Parasitol. 199 (1-2), 42–49. doi: 10.1016/j.vetpar.2013.10.004
Lee Y. M., Handali S., Hancock K., Pattabhi S., Kovalenko V. A., Levin A., et al. (2011a). Serologic diagnosis of human taenia solium cysticercosis by using recombinant and synthetic antigens in QuickELISA. Am. J. Trop. Med. Hyg 84 (4), 587–593. doi: 10.4269/ajtmh.2011.10-0079
Lee Y. M., Handali S., Hancock K., Pattabhi S., Kovalenko V. A., Levin A., et al. (2011b). Serologic diagnosis of human taenia solium cysticercosis by using recombinant and synthetic antigens in QuickELISA™. Am. J. Trop. Med. Hyg 84 (4), 587–593. doi: 10.4269/ajtmh.2011.10-0079
Lee C., Noh J., O'Neal S. E., Gonzalez A. E., Garcia H. H., Handali S. (2019). Feasibility of a point-of-care test based on quantum dots with a mobile phone reader for detection of antibody responses. PloS Negl. Trop. Dis. 13 (10), e0007746. doi: 10.1371/journal.pntd.0007746
León-Janampa N., Liendo R., Gilman R. H., Padilla C., García H. H., Gonzales A., et al. (2019). Characterization of a novel cathepsin l-like protease from taenia solium metacestodes for the immunodiagnosis of porcine cysticercosis. Vet. Parasitol. 267, 9–16. doi: 10.1016/j.vetpar.2019.01.004
Liang J., Wang F., Lin X., Qi H., Wu J. (2019). Serologic diagnosis of taenia solium cysticercosis through linear unmixing analysis of biosignals from ACEK capacitive sensing method. Annu. Int. Conf IEEE Eng. Med. Biol. Soc. 2019, 2261–2264.
Li Y., Liu H., Yang Y. M. (2011). [Application of molecular biological techniques in taenia identification]. Zhongguo Ji Sheng Chong Xue Yu Ji Sheng Chong Bing Za Zhi 29 (5), 385–388. doi: 10.1016/j.actatropica.2008.12.004
Lozano N., Samblas M. G., Calabuig E., Gimenez Marti M. J., Gomez Ruiz M. D., Arce J. M. S., et al. (2023). Use of sera cell free DNA (cfDNA) and exovesicle-DNA for the molecular diagnosis of chronic chagas disease. PloS One 18 (9), e0282814. doi: 10.1371/journal.pone.0282814
Machado G. A., Oliveira H. B., Gennari-Cardoso M. L., Mineo J. R., Costa-Cruz J. M. (2013). Serodiagnosis of human neurocysticercosis using antigenic components of taenia solium metacestodes derived from the unbound fraction from jacalin affinity chromatography. Mem Inst Oswaldo Cruz 108 (3), 368–375. doi: 10.1590/S0074-02762013000300016
Mahale R. R., Mehta A., Rangasetty S. (2015). Extraparenchymal (Racemose) neurocysticercosis and its multitude manifestations: A comprehensive review. J. Clin. Neurol. 11 (3), 203–211. doi: 10.3988/jcn.2015.11.3.203
Manterola C., Grande L., Otzen T., Garcia N., Salazar P., Quiroz G. (2018). [Reliability, precision or reproducibility of the measurements. methods of assessment, utility and applications in clinical practice]. Rev. Chil. Infectol 35 (6), 680–688.
McCleery E., Allen S. E., Moyano L. M., Gamboa R., Vilchez P., Muro C., et al. (2020). Population screening for urine antigens to detect asymptomatic subarachnoid neurocysticercosis: A pilot study in northern peru. Am. J. Trop. Med. Hyg 103 (3), 1125–1128. doi: 10.4269/ajtmh.20-0196
Michelet L., Fleury A., Sciutto E., Kendjo E., Fragoso G., Paris L., et al. (2011). Human neurocysticercosis: comparison of different diagnostic tests using cerebrospinal fluid. J. Clin. Microbiol. 49 (1), 195–200. doi: 10.1128/JCM.01554-10
Mlowe F., Karimuribo E., Mkupasi E., Churi A., Nyerere A. D., Schmidt V., et al. (2022). Challenges in the diagnosis of taenia solium cysticercosis and taeniosis in medical and veterinary settings in selected regions of tanzania: A cross-sectional study. Vet. Med. Int. 2022, 7472051. doi: 10.1155/2022/7472051
Moss D. M., Handali S., Chard A. N., Trinies V., Bullard S., Wiegand R. E., et al. (2018). Detection of immunoglobulin g antibodies to taenia solium cysticercosis antigen glutathione-S-Transferase-rT24H in malian children using multiplex bead assay. Am. J. Trop. Med. Hyg 98 (5), 1408–1412. doi: 10.4269/ajtmh.17-0310
Moyano L. M., Saito M., Montano S. M., Gonzalvez G., Olaya S., Ayvar V., et al. (2014). Neurocysticercosis as a cause of epilepsy and seizures in two community-based studies in a cysticercosis-endemic region in peru. PloS Negl. Trop. Dis. 8 (2), e2692. doi: 10.1371/journal.pntd.0002692
Mubanga C., Van Damme I., Trevisan C., Schmidt V., Phiri I. K., Zulu G., et al. (2021). Evaluation of an antibody detecting point of care test for diagnosis of taenia solium cysticercosis in a zambian rural community: A prospective diagnostic accuracy study. Diagnostics (Basel) 11 (11). doi: 10.3390/diagnostics11112121
Mwape K. E., Praet N., Benitez-Ortiz W., Muma J. B., Zulu G., Celi-Erazo M., et al. (2011). Field evaluation of urine antigen detection for diagnosis of taenia solium cysticercosis. Trans. R Soc. Trop. Med. Hyg 105 (10), 574–578. doi: 10.1016/j.trstmh.2011.05.010
Nash T. E., Bustos J. A., Garcia H. H., Cysticercosis Working Group in, P. (2017). Disease centered around calcified taenia solium granuloma. Trends Parasitol. 33 (1), 65–73. doi: 10.1016/j.pt.2016.09.003 doi: 10.1186/s13071-024-06197-6
Nash T. E., Mahanty S., Loeb J. A., Theodore W. H., Friedman A., Sander J. W., et al. (2015). Neurocysticercosis: A natural human model of epileptogenesis. Epilepsia 56 (2), 177–183. doi: 10.1111/epi.12849
Nash T. E., O'Connell E. M. (2020). Subarachnoid neurocysticercosis: emerging concepts and treatment. Curr. Opin. Infect. Dis. 33 (5), 339–346. doi: 10.1097/QCO.0000000000000669
Nash T. E., Ware J. M., Mahanty S. (2017b). Natural history of patients with perilesional edema around taenia solium calcified granulomas. J. Infect. Dis. 215 (7), 1141–1147. doi: 10.1093/infdis/jix027
Nhancupe N., Noormahomed E. V., Afonso S., Falk K. I., Lindh J. (2016). Performance of tsol-p27 antigen for the serological diagnosis of cysticercosis in mozambique. J. Helminthol 90 (5), 630–633. doi: 10.1017/S0022149X15000747
Nhancupe N., Noormahomed E. V., Afonso S., Svard S., Lindh J. (2019). Further evaluation of recombinant tsol-p27 by enzyme-linked immunoelectrotransfer blot for the serodiagnosis of cysticercosis in pigs from mozambique. Parasit Vectors 12 (1), 564. doi: 10.1186/s13071-019-3816-x
Noh J., Rodriguez S., Lee Y. M., Handali S., Gonzalez A. E., Gilman R. H., et al. (2014). Recombinant protein- and synthetic peptide-based immunoblot test for diagnosis of neurocysticercosis. J. Clin. Microbiol. 52 (5), 1429–1434. doi: 10.1128/JCM.03260-13
O'Connell E. M., Harrison S., Dahlstrom E., Nash T., Nutman T. B. (2020). A novel, highly sensitive quantitative polymerase chain reaction assay for the diagnosis of subarachnoid and ventricular neurocysticercosis and for assessing responses to treatment. Clin. Infect. Dis. 70 (9), 1875–1881. doi: 10.1093/cid/ciz541
Ostoa-Saloma P., Esquivel-Velázquez M., Larralde C. (2013). Immunological variation in taenia solium porcine cysticercosis: measurement on the variation of the antibody immune response of naturally infected pigs against antigens extracted from their own cysticerci and from those of different pigs. Vet. Parasitol. 197 (1-2), 130–140. doi: 10.1016/j.vetpar.2013.07.010
Pamela B. E., Vasudevan P., Thamizhmaran S., Moorthy R. K., Oommen A., Manoj J., et al. (2021). Monocyte gene expression distinguishes enhancing brain parenchymal cysticercal granulomas from tuberculomas. Open Forum Infect. Dis. 8 (9), ofab427. doi: 10.1093/ofid/ofab427
Pappala B. C. S., Indugula J. P., Shrivastava A. K., Kumar S., Talabhatula S. K., Kolli R. S., et al. (2017). Comparative evaluation of indigenous ELISAs for detection of anti-cysticercus IgG antibodies in serum from clinically and radiologically suspected cases of neurocysticercosis. Trop. BioMed. 34 (3), 622–635.
Paredes A., Sáenz P., Marzal M. W., Orrego M. A., Castillo Y., Rivera A., et al. (2016). Anti-taenia solium monoclonal antibodies for the detection of parasite antigens in body fluids from patients with neurocysticercosis. Exp. Parasitol. 166, 37–43. doi: 10.1016/j.exppara.2016.03.025
Parija S. C., Gireesh A. (2011). Cysticercus cellulosae antigens in the serodiagnosis of neurocysticercosis. Trop. Parasitol. 1 (2), 64–72. doi: 10.4103/2229-5070.86932
Parija S. C., Raman G. A. (2011). Anti-taenia solium larval stage ig g antibodies in patients with epileptic seizures. Trop. Parasitol. 1 (1), 20–25. doi: 10.4103/2229-5070.72113
Parkhouse R. M. E., Carpio A., Campoverde A., Sastre P., Rojas G., Cortez M. M. (2018). Reciprocal contribution of clinical studies and the HP10 antigen ELISA for the diagnosis of extraparenchymal neurocysticercosis. Acta Trop. 178, 119–123. doi: 10.1007/s00406-006-0612-3 doi: 10.1016/j.actatropica.2017.11.005
Piña R., Gutiérrez A. H., Gilman R. H., Rueda D., Sifuentes C., Flores M., et al. (2011). A dot-ELISA using a partially purified cathepsin-l-like protein fraction from taenia solium cysticerci, for the diagnosis of human neurocysticercosis. Ann. Trop. Med. Parasitol. 105 (4), 311–318.
Pineda-Reyes R., White A. C. Jr. (2022). Neurocysticercosis: an update on diagnosis, treatment, and prevention. Curr. Opin. Infect. Dis. 35 (3), 246–254. doi: 10.1097/QCO.0000000000000831
Praet N., Rodriguez-Hidalgo R., Speybroeck N., Ahounou S., Benitez-Ortiz W., Berkvens D., et al. (2010). Infection with versus exposure to taenia solium: what do serological test results tell us? Am. J. Trop. Med. Hyg 83 (2), 413–415. doi: 10.4269/ajtmh.2010.10-0121
Ramachandran P. S., Wilson M. R. (2018). Diagnostic testing of neurologic infections. Neurol. Clin. 36 (4), 687–703. doi: 10.1016/j.ncl.2018.07.004
Ramahefarisoa R. M., Rakotondrazaka M., Jambou R., Carod J. F. (2010). Comparison of ELISA and PCR assays for the diagnosis of porcine cysticercosis. Vet. Parasitol. 173 (3-4), 336–339. doi: 10.1016/j.vetpar.2010.05.002
Ribeiro Vda S., Araújo T. G., Gonzaga H. T., Nascimento R., Goulart L. R., Costa-Cruz J. M. (2013). Development of specific scFv antibodies to detect neurocysticercosis antigens and potential applications in immunodiagnosis. Immunol. Lett. 156 (1-2), 59–67.
Ribeiro Vda S., Nunes Dda S., Gonzaga H. T., da Cunha-Junior J. P., Costa-Cruz J. M. (2014). Diethylaminoethyl (DEAE) binding fraction from taenia solium metacestode improves the neurocysticercosis serodiagnosis. Parasitol. Res. 113 (7), 2569–2575.
Rodriguez S., Dorny P., Tsang V. C., Pretell E. J., Brandt J., Lescano A. G., et al. (2009). Detection of taenia solium antigens and anti-t. solium antibodies in paired serum and cerebrospinal fluid samples from patients with intraparenchymal or extraparenchymal neurocysticercosis. J. Infect. Dis. 199 (9), 1345–1352.
Rodríguez-Hidalgo R., Carpio A., Van den Enden E., Benítez-Ortiz W. (2019). Monitoring treatment of taenia solium- neurocysticercosis by detection of circulating antigens: a case report. BMC Neurol. 19 (1), 52. doi: 10.1186/s12883-019-1282-x
Rodriguez S., Wilkins P., Dorny P. (2012). Immunological and molecular diagnosis of cysticercosis. Pathog. Glob Health 106 (5), 286–298. doi: 10.1179/2047773212Y.0000000048
Roelfsema J. H., Nozari N., Pinelli E., Kortbeek L. M. (2016). Novel PCRs for differential diagnosis of cestodes. Exp. Parasitol. 161, 20–26. doi: 10.1016/j.exppara.2015.12.010
Rottbeck R., Nshimiyimana J. F., Tugirimana P., Düll U. E., Sattler J., Hategekimana J. C., et al. (2013). High prevalence of cysticercosis in people with epilepsy in southern rwanda. PloS Negl. Trop. Dis. 7 (11), e2558. doi: 10.1371/journal.pntd.0002558
Rueda A., Sifuentes C., Gilman R. H., Gutiérrez A. H., Piña R., Chile N., et al. (2011). TsAg5, a taenia solium cysticercus protein with a marginal trypsin-like activity in the diagnosis of human neurocysticercosis. Mol. Biochem. Parasitol. 180 (2), 115–119. doi: 10.1016/j.molbiopara.2011.08.003
Sadaow L., Boonroumkaew P., Rodpai R., Janwan P., Sanpool O., Thanchomnang T., et al. (2023). Development and evaluation of an immunochromatography-based point-of-care test kit for a rapid diagnosis of human cysticercosis. Food Waterborne Parasitol. 33, e00211. doi: 10.1016/j.fawpar.2023.e00211
Sahu P. S., Parija S., Kumar D., Jayachandran S., Narayan S. (2014). Comparative profile of circulating antigenic peptides in CSF, serum & urine from patients with neurocysticercosis diagnosed by immunoblotting. Parasite Immunol. 36 (10), 509–521. doi: 10.1111/pim.12124
Sako Y., Takayanagui O. M., Odashima N. S., Ito A. (2015). Comparative study of paired serum and cerebrospinal fluid samples from neurocysticercosis patients for the detection of specific antibody to taenia solium immunodiagnostic antigen. Trop. Med. Health 43 (3), 171–176. doi: 10.2149/tmh.2015-04
Salazar-Anton F., Tellez A., Lindh J. (2012). Evaluation of an immunodot blot technique for the detection of antibodies against taenia solium larval antigens. Parasitol. Res. 110 (6), 2187–2191. doi: 10.1007/s00436-011-2747-z
Satyaprakash K., Khan W. A., Chaudhari S. P., Shinde S. V., Kolte S. W., Pansare N. R., et al. (2023). Optimization of in-house indirect-ELISA & EITB assays employing cysticercus cellulosae antigens for serological detection and PCR assays for molecular detection of porcine cysticercosis. Iran J. Parasitol. 18 (1), 19–29. doi: 10.18502/ijpa.v18i1.12376
Sreedevi C., Hafeez M., Kumar P. A., Rayulu V. C., Subramanyam K. V., Sudhakar K. (2012). PCR test for detecting taenia solium cysticercosis in pig carcasses. Trop. Anim. Health Prod 44 (1), 95–99. doi: 10.1007/s11250-011-9893-2
Stelzle D., Makasi C. E., Schmidt V., Van Damme I., Trevisan C., Ruether C., et al. (2023). Evaluation of a point-of-care test for the diagnosis of taenia solium neurocysticercosis in rural southern tanzania: a diagnostic accuracy study. Lancet Infect. Dis.
Suzuki L. A., Rossi C. L. (2011). Evaluation of two taenia solium cysticercal antigenic preparations (vesicular fluid and a glycoprotein fraction with affinity for lentil lectin) for the immunodiagnosis of neurocysticercosis by enzyme-linked immunosorbent assay (ELISA). Arq Neuropsiquiatr 69 (3), 470–474. doi: 10.1590/S0004-282X2011000400012
Takayanagui O. M., Haes T. M. (2022). Update on the diagnosis and management of neurocysticercosis. Arq Neuropsiquiatr 80 (5 Suppl 1), 296–306. doi: 10.1590/0004-282x-anp-2022-s115
Tang N. L., Nash T. E., Corda M., Nutman T. B., O'Connell E. M. (2023). Triplex ELISA for assessing durability of taenia solium seropositivity after neurocysticercosis cure. Emerg. Infect. Dis. 29 (7), 1340–1348. doi: 10.3201/eid2907.230364
Toribio L., Guzman C., Noazin S., Zimic-Sheen A., Zimic M., Gonzales I., et al. (2023b). Multiantigen print immunoassay (MAPIA) for the diagnosis of neurocysticercosis: a single-center diagnostic optimization and accuracy study in Lima, Peru. J. Clin. Microbiol., e0076023. doi: 10.1128/jcm.00760-23
Toribio L., Handali S., Marin Y., Perez E., Castillo Y., Bustos J. A., et al. (2023a). A rapid point-of-Care assay for cysticercosis antigen detection in urine samples. Am. J. Trop. Med. Hyg 108 (3), 578–580. doi: 10.4269/ajtmh.22-0598
Toribio L., Romano M., Scott A. L., Gonzales I., Saavedra H., Garcia H. H., et al. (2019). Detection of taenia solium DNA in the urine of neurocysticercosis patients. Am. J. Trop. Med. Hyg 100 (2), 327–329. doi: 10.4269/ajtmh.18-0706
Toribio L., Santivanez S., Scott A. L., Enriquez R., Sedano C., Soto-Becerra P., et al. (2020). Diagnostic urinary cfDNA detected in human cystic echinococcosis. Mol. Biochem. Parasitol. 239, 111314. doi: 10.1016/j.molbiopara.2020.111314
Tsang V. C., Brand J. A., Boyer A. E. (1989). An enzyme-linked immunoelectrotransfer blot assay and glycoprotein antigens for diagnosing human cysticercosis (Taenia solium). J. Infect. Dis. 159 (1), 50–59. doi: 10.1093/infdis/159.1.50
Tsang V. C., Pilcher J. A., Zhou W., Boyer A. E., Kamango-Sollo E. I., Rhoads M. L., et al. (1991). Efficacy of the immunoblot assay for cysticercosis in pigs and modulated expression of distinct IgM/IgG activities to taenia solium antigens in experimental infections. Vet. Immunol. Immunopathol. 29 (1-2), 69–78. doi: 10.1016/0165-2427(91)90053-F
Zammarchi L., Angheben A., Fantoni T., Chiappini E., Mantella A., Galli L., et al. (2018). Screening for neurocysticercosis in internationally adopted children: yield, cost and performance of serological tests, ital To 2016. Euro Surveill 23 (40). doi: 10.2807/1560-7917.ES.2018.23.40.1700709
Keywords: neurocysticercosis, immunodiagnosis, molecular diagnosis, Taenia solium, Peru
Citation: Toribio LM, Bustos JA and Garcia HH (2024) From laboratory to clinical practice: an update of the immunological and molecular tools for neurocysticercosis diagnosis. Front. Parasitol. 3:1394089. doi: 10.3389/fpara.2024.1394089
Received: 01 March 2024; Accepted: 04 June 2024;
Published: 01 July 2024.
Edited by:
Vitaliano Cama, Centers for Disease Control and Prevention (CDC), United StatesReviewed by:
Alejandro Castellanos-Gonzalez, University of Texas Medical Branch at Galveston, United StatesCopyright © 2024 Toribio, Bustos and Garcia. This is an open-access article distributed under the terms of the Creative Commons Attribution License (CC BY). The use, distribution or reproduction in other forums is permitted, provided the original author(s) and the copyright owner(s) are credited and that the original publication in this journal is cited, in accordance with accepted academic practice. No use, distribution or reproduction is permitted which does not comply with these terms.
*Correspondence: Luz M. Toribio, bHRvcmliaW9Ac2d1bC5hYy51aw==; bHV6LnRvcmliaW8uc2FsYXphckB1cGNoLnBl
Disclaimer: All claims expressed in this article are solely those of the authors and do not necessarily represent those of their affiliated organizations, or those of the publisher, the editors and the reviewers. Any product that may be evaluated in this article or claim that may be made by its manufacturer is not guaranteed or endorsed by the publisher.
Research integrity at Frontiers
Learn more about the work of our research integrity team to safeguard the quality of each article we publish.