- 1Department of Animal Biology Faculty of Science, University of Dschang, Dschang, Cameroon
- 2Laboratory of Tropical and Emerging Infectious Diseases, Dschang, Cameroon
- 3Department of Microbiology, Hematology and Immunology Faculty of Medicine and Pharmaceutical Sciences, University of Dschang, Dschang, Cameroon
- 4Department of Biomedical Sciences, Faculty of Health Sciences, University of Bamenda, Bambili, Cameroon
- 5Department of Animal Organisms, Faculty of Science, University of Douala, Douala, Cameroon
- 6National Engineering Research Center for Modernization of Traditional Chinese Medicine – Hakka Medical Resources Branch, Gannan Medical University, Ganzhou, China
Background: Malaria is one of the leading causes of morbidity and/or mortality in tropical Africa. The spread and development of resistance to chemical antimalarial drugs and the relatively high cost of the latter are problems associated with malaria control and are reasons to promote the use of plants to meet healthcare needs to treat malaria. The aim of this study was to evaluate antiplasmodial activities of extracts of Erythrina sigmoidea (Mah quat), which is traditionally used for the treatment of malaria in the western region of Cameroon.
Material and methods: The ethanol extract of E. sigmoidea stem bark was obtained through the maceration process using 95% ethanol, while the aqueous extract was prepared by infusion. The in vitro antiplasmodial effect of extracts against P. falciparum chloroquine-sensitive (3D7) and chloroquine-resistant (Dd2) strains was determined using the Trager and Jensen method. On the other hand, the in vivo antimalarial activity of the extract was evaluated in mice infected with Plasmodium berghei strain NK65 using the Peters’ 4-day suppressive test and Ryley test (curative test). A total of 36 mice were used, subdivided into six groups of six mice each: one normal control, a negative control, a positive control, and three other groups for the tested product. Blood samples were collected on the 10th day of each test for hematological parameters.
Results: The aqueous extract had an in vitro antiplasmodial activity against the chloroquine-sensitive strain with an IC50 of 29.51 ± 3.63 µg/mL and against the chloroquine-resistant strain with an IC50 of 35.23 ± 3.17 µg/mL. The highest in vitro antiplasmodial activity was observed with the ethanol extract against the chloroquine-sensitive strain with an IC50 of 6.44 ± 0.08 µg/mL and against the chloroquine-resistant strain with an IC50 of 7.53 ± 0.22 µg/mL. The ethanol extract demonstrated suppressive activity in vivo with reduction rates of 87.69%, 86.79%, and 81.08% at doses of 500 mg/kg, 250 mg/kg, and 125 mg/kg, respectively; and curative activity in vivo with reduction rates of 80%, 78.5%, and 77.5% at doses of 500 mg/kg, 250 mg/kg, and 125 mg/kg, respectively. The number of white blood cells in the negative control (44.55 ± 5.02 103/µL) was higher compared to the other groups. As for the red blood cells, we observed a massive destruction of the latter in the infected and untreated group (5.82 ± 1.50 106/µL) compared to the infected and ethanol extract-treated groups (8.74 ± 1.57 106/µL for 500 mg/kg, 7.54 ± 1.77 106/µL for 250 mg/kg, and 8.9 ± 1.50 106/µL for 125 mg/kg).
Conclusion: This study provides scientific data on the use of E. sigmoidea by the local population for the treatment of malaria. It shows that E. sigmoidea has antiplasmodial activity, and we also see that there are differences between the parameters that we have in the treated groups and those of the untreated group. However, toxicity tests are necessary to assess its safety.
1 Introduction
Especially in sub-Saharan Africa, Asia, and Latin America, malaria is a serious tropical illness with a high morbidity and fatality rate (Kolawole et al., 2023). A parasite known as Plasmodium is the cause of malaria and is spread by mosquito bites. There are five species of Plasmodium that people can contract and spread (Sato, 2021). Plasmodium falciparum is mostly responsible for severe illness. The human body’s liver is where parasites first proliferate, followed by erythrocytes. Fever, headaches, and vomiting are among the symptoms of malaria that often manifest 10 to 15 days following the mosquito bite (Balaji et al., 2020). Malaria can soon become fatal if left untreated because it can cut off blood flow to essential organs.
In 2019, the World Health Organization (WHO) estimated that, worldwide, approximately 241 million cases and 627,000 deaths were due to malaria (Liu et al., 2022). These increases are mainly observed in sub-Saharan Africa where there are 12% more deaths than in 2020 (Murray et al., 2007). In sub-Saharan Africa particularly, malaria remains the primary medical concern. The vulnerable groups are mainly children under 5 years old and pregnant women (Gilmartin et al., 2021). The RTS, S/AS01 malaria vaccine implemented in Ghana, Kenya, and Malawi by the WHO in children from the age of 5 months has the specific aim of reducing the parasitic load and not of actually preventing the disease. In Cameroon, malaria constitutes a serious public health problem despite an improvement in certain indicators and the surveillance system (Haidara et al., 2016).
In Cameroon, malaria is also a major health burden for families (Antonio-Nkondjio et al., 2019). Malaria has been managed by an integrated approach that includes medication treatment and vector control (Shiff, 2002). Presently, the main medications used to treat malaria are artemisinin-based combination treatments (ACTs) (Ouji et al., 2018). On the other hand, ACTs are expensive. Regrettably, there have been indications of rising drug resistance to derivatives of artemisinin (Wongsrichanalai and Sibley, 2013). The steady increase in resistance to the majority of available antimalarial drugs and the ability of malaria vectors to resist insecticides are responsible for the re-emergence of malaria in many parts of the world (Na-Bangchang and Karbwang, 2009). The alarming increase in malaria drug resistance coupled with vector resistance has led to the failure of the prevention and eradication program put in place (Sidiki et al., 2023). It is now established that natural products constitute a major and abundant source of anti-infective agents (Mahmoudvand et al., 2023).
In the past, traditional herbs had wide acceptance in the treatment of malaria. As early as the 17th century, infusion of the barks of plant species like Cinchona (Rubiaceae) were effective for the treatment of human malaria (Ceravolo et al., 2021). Over time, quinine has been isolated and characterized, making it an essential drug against malaria (Jones et al., 2015). Therefore, it is crucial to keep up the fight against malaria in Cameroon, and a number of scientists there are using ethnobotanical data to identify effective medicinal plants that could lead to the development of novel antimalarial drugs. In fact, researching medicinal plants is still an effective way to find novel medications. Medicinal herbs are the most cost-effective means of treatment in endemic areas, and substances with antiplasmodial activity include flavonoids, terpenoids, and alkaloids (Narayanan et al., 2019).
Erythrina sigmoidea, family Fabaceae (common name: Mah quat), stem bark is used by the local population of Foumban for the treatment of malaria, typhoid fever, helminthiasis, hepatitis, venereal diseases, conjunctivitis, piles, diarrhea, dysentery, malaria, microbia, bacteria, jaundice, and syphilis, and has many properties such as hallucinatory, aphrodisiac, diuretic, hemostatic, purgative, and tonic. This plant has shown anti-inflammatory, antibacterial, and anticholinergic activities based on phytochemical analysis (Narayanan et al., 2019). Another leguminous plant, Bauhinia variegata, showed marked antileishmanial activity with cell cycle arrest at the sub-G0/G1 phase. B. variegata was found to be more effective at a higher dose in declining parasite concentration in the spleen as compared to the lower dose (Kaur et al., 2021). The aim of this study was to evaluate the antiplasmodial activity of E. sigmoidea, a medicinal plant used for the treatment of malaria in the western region of Cameroon and to provide scientific evidence on its use as an antimalaria agent.
2 Material and methods
2.1 Collection and identification
The stem bark plant was harvested in Foumban West Region of Cameroon on November 2023 in the dry season. The following plant material were sent to the National Herbarium of Cameroon in Yaounde for identification: stem bark, leaves, seeds, and flowers. The plant was identified with Reference Number 8749/SRFCAM by Dr NGANTSOP.
2.2 Preparation of extracts
Ethanol extract was used for the extraction because during the ethnobotanical study, a traditional practitioner used palm wine (ethanol) for maceration or infusion to prepare this remedy. The aqueous extract was prepared by infusion while the ethanol extract was obtained using the procedure described by Wabo et al. (2011). Briefly, 100 g of powder was weighted using an electric balance (SF-400) and introduced into two 3-L containers. One liter of 95% ethanol and heated (100°C) distilled water were introduced in each container and the mixtures were stirred for 5 min. The mixture of ethanol was macerated for 72 h at room temperature, and that of the aqueous solution was left to cool down. The homogenates were first filtered using a 150-μm sieve and then with Whatman paper (No. 1). The filtrates obtained were introduced into an oven at 40°C for evaporation until aqueous and ethanol extracts were obtained.
2.3 In vitro antiplasmodial activity
In summary, fresh human red blood cells of group O+ were used to culture the chloroquine-sensitive Pf3D7 strains and the multi-resistant PfDd2 strains at 4% hematocrit in complete RPMI medium (Gibco, UK). This medium was supplemented with 25 mM HEPES (Gibco, UK), 0.50% Albumax I (Gibco, USA), 1× hypoxanthine (Gibco, USA), and 20 µg/mL gentamicin (Gibco, China). The cells were then incubated at 37°C in a humidified incubator with 92% N2, 5% CO2, and 3% O2. Using the Trager and Jensen method (Trager and Jensen, 1997), the chloroquine-sensitive (3D7) and chloroquine-resistant (Dd2) strains, frequently employed in drug sensitivity experiments, were used. The culture was carried out in Petri dishes and maintained in a humid atmosphere in an incubator. The culture medium in the Petri dishes in culture was renewed daily. The parasitemia of the cultured strain was checked on a May Grunwald-Giemsa-stained thin smear. The differential permeability of the old stages to D-Sorbitol was used to destroy them selectively by osmotic lysis, which makes it possible to obtain cultures enriched in ring stages. Briefly, 90 μL of the parasite solution that was synchronized at the ring stage with 2% parasitemia and 1% hematocrit was incubated with 10 μL of various concentrations of extracts, artemisinin, and chloroquine. The plates were incubated for 48 h. The antiplasmodial activity test of plant extracts, artemisinin, and chloroquine was carried out in 96-well flat-bottomed microplates based on the fluorescence of SYBR green I. The ability of SYBR green I to produce strong fluorescence only in the presence of DNA is the basis for its use in the assessment of cell proliferation. The absence of a cell nucleus inside human red blood cells in which the parasite proliferates allows specific monitoring of plasmodial growth using SYBR green I. One hour prior to the end of incubation, 100 μL of SYBR green was added. The fluorescence was measured using a fluorescence microplate reader at an excitation and emission wavelength of 485 and 538 nm, respectively. The resistance index (RI) was calculated using the formula (Kevin et al., 2023):
2.4 In vivo antiplasmodial activity
2.4.1 Choice of the gavage solution doses
Approximately 650 g of E. sigmoidea plant powder (stem bark), as indicated by the traditional practitioner, was infused in 6 L of water for 15 min. The resulting mixture was cooled and filtered through Whatman No. 1 paper. Three glasses (the practitioner’s daily volume, which is approximately 1 L) were taken from the filtrate and evaporated in a ventilated oven at 40°C, giving a weight of approximately 16.5 g. Assuming that the average weight of a man is 70 kg, the dose of 235.71 mg/kg (16.5/70) = 250 mg/kg was obtained. This dose was divided by 2 and multiplied by 2 to get 125 mg/kg and 500 mg/kg, respectively.
2.4.2 The Peters’ 4-day suppressive test
Mice (Mus musculus) from the Animal Facility of the Center for Schistosomiasis and Parasitology (CSP) in Yaoundé (at the sports stadium) was used in the in vivo tests. Plasmodium berghei NK 65 strain given by the American Type Culture Collection (ATCC) was used. Two weeks were taken for the acclimatization of the mice. The antimalarial activity of the extract was determined using Peters’ 4-day suppressive test (Peters, 1975). A total of 36 mice with an average weight of 25 ± 0.5 g were divided into six groups of six mice each. Mice were randomly inoculated with 1×107 parasitized erythrocytes with P. berghei (except normal control group), stained with picric acid and dispatched into different groups. Three hours after infection, the first three groups received the ethanol extract of E. sigmoidea at doses of 125, 250, and 500 mg/kg of body weight. The three remaining groups each received 1% DMSO (negative control), 5 mg/kg chloroquine (positive control), and distilled water (normal control). All doses were administered orally. These mice were treated for 4 days (D1, D2, D3, and D4). From the 5th to the 10th day (D5 to D10), the parasitemia of each mouse was evaluated. Blood smears were prepared from the tail of each mouse, stained with May Grunwald-Giemsa, and observed under a microscope at 100× objective. From the 11th to the 30th day, mice were observed for the average survival rate. Parameters such as weight and temperature were recorded. The parasitemia, as well as the reduction rate, were determined and calculated as follows:
Parasitemia (P):
The reduction rate:
The average survival rate:
2.4.3 Curative test
The curative activity was evaluated by the method of Ryley (Ryley and Peters, 1970) (Figure 1). A total of 36 albino mice (male and female) with an average weight of 25 ± 0.5 g were divided into six groups of six mice each. Five groups of these mice were infested intraperitoneally on day (D1) with 1×107 parasitized red blood cells contained in 200 μL. Seventy-two hours after infestation, the experimental animals were treated on D4, D5, D6, and D7 at 24-h intervals for 4 days with the ethanol extract (EE). Groups I, II, and III received the extracts at a dose of 500–250–125 mg/kg. Group IV (Negative Control) received 1% DMSO, Group V (Positive Control) received chloroquine (CQ) at a dose of 5 mg/kg, and Group VI (Neutral Control) received distilled water. On D4, the parasitemia of all experimental groups was determined by May-Grünwald-Giemsa staining.
The average survival rate of the treatment groups was determined arithmetically by calculating the average survival time in days from the day of infestation until the 30th day (D1–D30) (Sidiki et al., 2023). The activity of the extract on parasites was expressed as a function of the reduction in parasitemia of treated mice compared to mice in the control group (Singh et al., 2015). Percentage inhibition and parasitemia were also calculated.
For hematological analyses, three mice per group were anesthetized using cotton soaked in chloroform and sacrificed; the blood obtained was introduced into EDTA tubes for hematological analysis using an automated hematology analyzer (Nihonkohden-celltax). The results were expressed as a mean ± standard deviation.
2.5 Quantitative phytochemical screening
The extracts were tested for the presence of flavonoids, alkaloids, triterpenoids, saponins, quinones, phenolic compound, and tannins using standard procedures described by Junaid and Patil (Shaikh and Patil, 2020).
2.6 Ethical approval
All authors hereby declare that the “Principles for the Care of Laboratory Animals” (NIH Publication No. 85-23, revised 1985) have been followed, as well as specific national laws, where applicable. All experiments were reviewed and approved by the Department of Animal Biology, Faculty of Science, University of Dschang.
2.7 Statistical analysis
The data collected were entered on Microsoft Office Excel version 2010 software. Subsequently, the 50% inhibitory concentrations (IC50) were determined using the concentration–response curves obtained by plotting the logarithm of the concentration as a function of the percentage of inhibition using the GraphPad Prism 8 software. The normality test was done using the Shapiro–Wilk test. The Kruskal–Wallis test and one-way ANOVA (analysis of variance) were used to compare the mean developmental inhibition rates of P. berghei. The tests were statistically significant at p ≤ 0.05.
3 Results
3.1 In vitro antiplasmodial activity
Table 1 shows the in vitro antiplasmodial activity of E. sigmoidea. It can be observed that the two extracts—the aqueous and ethanol extracts—inhibited the development of Plasmodium strains (3D7 for the sensitive strain and Dd2 for the resistant strain). For the two strains, the ethanol extract had an IC50 of 6.44 ± 0.08 µg/mL in the chloroquine-sensitive strain and an IC50 of 7.53 ± 0.22 µg/mL in the chloroquine-resistant strain. This ethanol extract had a lower IC50 than the aqueous extract, which had an IC50 of 29.51 ± 3.63 µg/mL in the chloroquine-sensitive strain and an IC50 of 35.23 ± 3.17 µg/mL in the chloroquine-resistant strain. The RI was 1.17 and 1.19 for the ethanol and aqueous extracts, respectively. When the RI is less than 1, this means that the drug acts preferentially on the chloroquine-sensitive strain of Plasmodium, whereas when the RI is greater than 1, the drug acts without distinction of Plasmodium strain.
3.2 In vivo suppressive effect of the ethanol extract on the evolution of parasitemia
Figure 2 shows that the ethanol extract in all doses used inhibited parasitemia in a dose-dependent manner. Parasitemia on the 10th day increased significantly in the negative control group (2.8% to 3.5%) while it decreased in the positive control group (1.2% to 0.2%). As for the groups treated with the extract, we observed that their parasitemia decreased at doses of 500 mg/kg (1.6% to 0.5%), 250 mg/kg (2% to 0.8%), and 125 mg/kg (2.4% to 0.6%) of body weight.
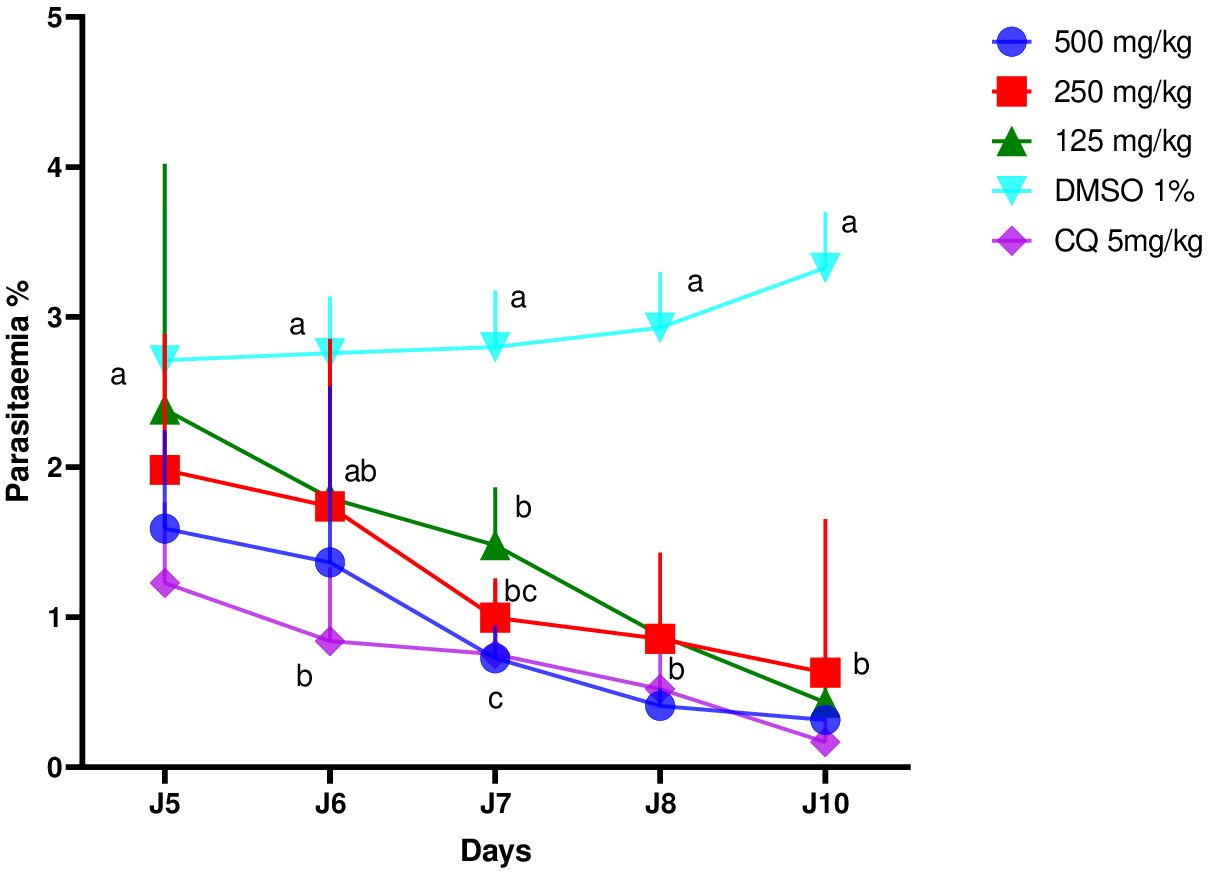
Figure 2 Effects of different doses of the ethanol extract of Erythrina sigmoidea on the evolution of parasitaemia in mice with respect to time.
3.2.1 Mortality rate of experimental animals after treatments with E. sigmoidea of the suppressive test
Table 2 shows that the groups that received 5 mg/kg of chloroquine and extract of E. sigmoidea with doses of 250 mg/kg and 500 mg/kg of body weight had no mortality on the 10th day, whereas the untreated group and the group receiving the extract with a dose of 125 mg/kg had a mortality rate of 16.67%. It is only after the 10th day that we recorded mortality in the groups treated with doses of 250 mg/kg and 500 mg/kg, with mortality rates of 83.33% and 66.67%, respectively, on the 30th day. We recorded mortality of 83.33% in the untreated group, and the group receiving the extract with a dose of 125 mg/kg had a mortality rate of 100% on the 30th day. The survival rate was 100% in the group treated with chloroquine on the 30th day.
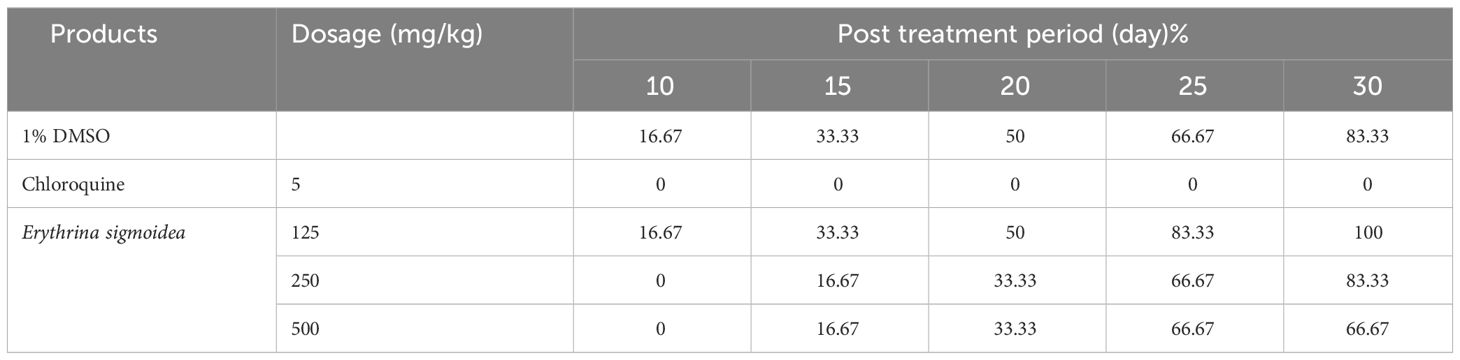
Table 2 Mortality rate (%) of experimental animals after treatment with different doses of extracts during the suppressive test.
3.2.2 Parasitemia reduction rate during post-treatment period of the suppressive test
Table 3 shows that the reference drug (chloroquine) used in this study exerts a suppressive activity of 94.89% with a dose of 5 mg/kg of body weight from the 10th day. The extract administered at doses of 500 mg/kg, 250 mg/kg, and 125 mg/kg/day inhibited growth of P. berghei by 87.69%, 86.79%, and 81.08%, respectively, in mice on the 10th day.
3.2.3 Effect of E. sigmoidea extract on hematological parameters during the suppressive test
Table 4 presents the effects of ethanol extract on hematological parameters. In the groups treated with plant extracts and chloroquine, parameters such as hemoglobin, red blood cells, and hematocrit increased compared to the group that received DMSO (negative control) and, on the other hand, decreased compared to the neutral control group. The white blood cell value increased while the other measured parameters rather decreased when compared to the normal control. The values of the other parameters of the groups treated with the extract and the chloroquine showed a statistically significant difference (p < 0.05) when compared with those of the normal and negative control groups.
3.2.4 Effect of E. sigmoidea ethanol extract on body temperature during the suppressive test
Table 5 shows the effect of treatment on body temperature. It follows from the analysis of this table that temperature did not really have an impact on the evolution of the suppressive test.
3.2.5 Effect of ethanol extract of Erythrina sigmoidea on body weight during the suppressive test
Table 6 shows the evolution of weight with respect to time during the suppressive test. It can be observed that on day 8, values of the different masses were slightly modified with a statistically significant difference (p < 0.05). However, there is an increase in weight in the positive control group, unlike the other groups.
3.3 Curative effect of the ethanol extract on the development of Plasmodium berghei
Figure 3 shows the evolution of parasitemia in mice treated with the ethanol extract of E. sigmoidea at doses of 125 mg/kg, 250 mg/kg, and 500 mg/kg. In general, these curves showed a growth phase that corresponded to the multiplication of the Plasmodium after the infection, then an inhibition phase of the development of Plasmodium after the mice had started assimilating the treatments (extracts and chloroquine). On the 10th day, the negative control group had its parasitemia increase (1.2% to 3.8%), while the other groups’ parasitemia dropped considerably.
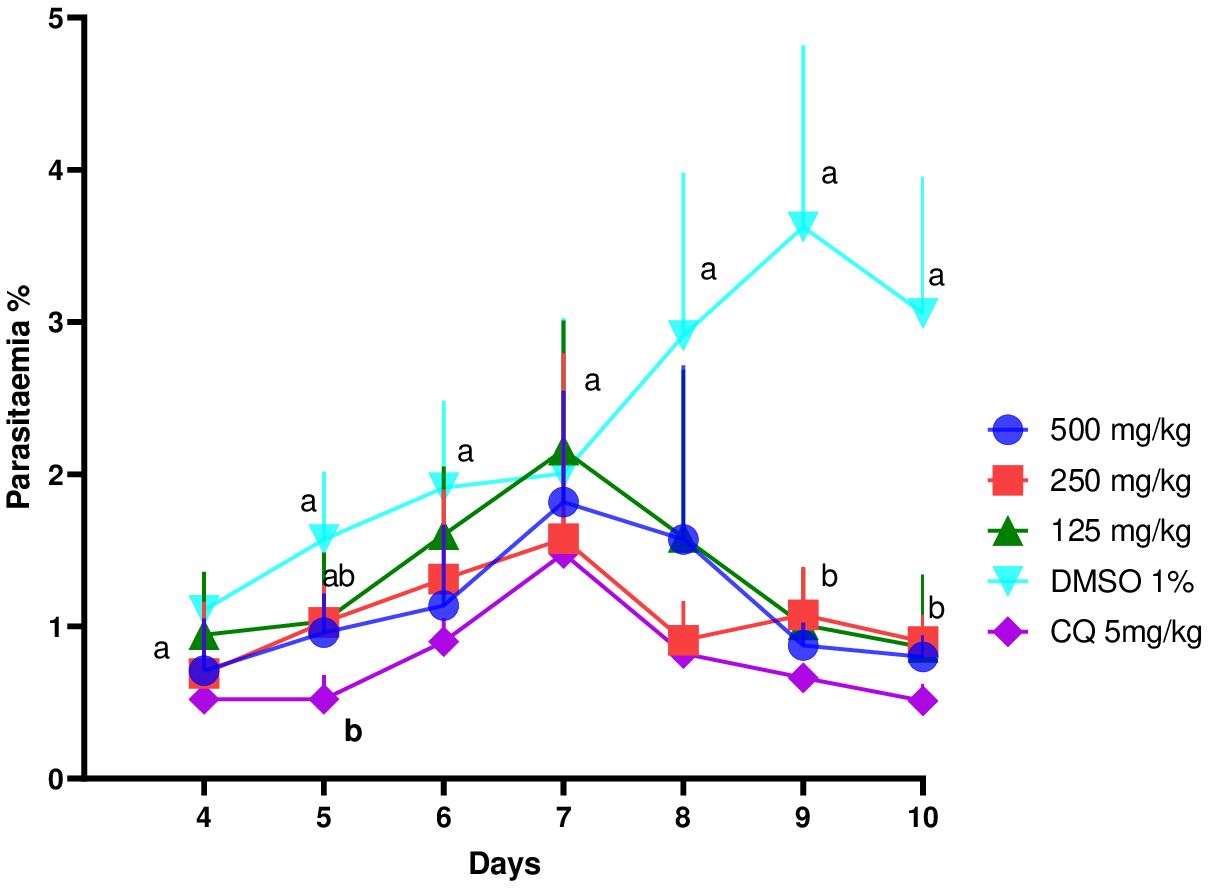
Figure 3 Effect of ethanol extract of Erythrina sigmoidea on the evolution of Plasmodium berghei parasitaemia in mice with respect to time.
3.3.1 Mortality rate during post treatment period for the curative test
Table 7 shows the mortality rate (%) during the post-treatment period of the curative test. It follows from the analysis of this table that the groups receiving chloroquine at 5 mg/kg and the extract at a dose of 500 mg/kg of body weight had no mortality on the 10th day, whereas in the untreated group and the group receiving the extract at doses of 125 mg/kg and 250 mg/kg, the mortality was 83.33%, 50%, and 33.33%, respectively, on day 20. On the 30th day, the mortality rates in the groups treated with chloroquine 5 mg/kg and the extract at 125 mg/kg and 500 mg/kg doses were 33.33%, 100%, and 66.67%, respectively.
3.3.2 Parasitemia reduction rate during the curative test
Table 8 shows that the inhibition rate was lower when compared to the inhibition of the suppressive test. The reference drug (chloroquine) used in this study had an inhibition rate of 87.5% from the 10th day. The ethanol extract of E. sigmoidea administered at doses of 500 mg/kg, 250 mg/kg, and 125 mg/kg/day inhibited the growth of P. berghei at 80%, 78.5%, and 77.5%, respectively, in mice.
3.3.3 Effect of E. sigmoidea on hematological parameters during the curative test
Table 9 shows the effect of E. sigmoidea on hematological parameters. It appears from this table that hemoglobin, RBCs, and hematocrit levels were higher in the groups treated with plant extracts and chloroquine than in the DMSO-treated (negative control) group, but lower than in the normal control group. When compared to the normal control, the white blood cell value increased while the other evaluated parameters actually declined. When compared to those of the normal and negative control groups, the values of the other parameters in the groups treated with the extract and the chloroquine revealed a statistically significant difference (p ≤ 0.05).
3.3.4 Effect of E. sigmoidea ethanol extract on body temperature during the curative test
Table 10 shows that the values for temperature were slightly modified with a statistically significant difference (p < 0.05). However, animals with the highest temperatures were those of the normal control groups and those of the doses of 250 and 500 mg/kg of body weight on day 8.
3.3.5 Effect of ethanol extract of Erythrina sigmoidea on body weight during the curative test
Table 11 shows that on day 8, the values of the different weights were slightly modified with a statistically significant difference (p < 0.05). However, there was an increase in weight in the positive control group, unlike the other groups.
3.4 Qualitative phytochemical screening
Table 12 shows the phytochemical screening of the ethanol extract of E. sigmoidea stem bark. It follows from the analysis of this table that this extract contains flavonoid, phenolic compound, tannin, and saponins.
4 Discussion
The IC50 values obtained with extracts of E. sigmoidea against P. falciparum strain using the Trager and Jensen (1997) method show that the extracts have promising activity with IC50 6.44 ± 0.08 µg/mL on the sensitive strain and 7.53 ± 0.22 µg/mL on the resistant strain of the ethanol extract and moderate activity with IC50 29.51 ± 3.63 µg/mL on the sensitive strain and 35.23 ± 3.17 µg/mL on the resistant strain for the aqueous extract. According to WHO guidelines, antiplasmodial activity is classified as follows: highly active for IC50 values of 5 g/mL; promising for values of 5–15 g/mL; moderate for values of 15–50 g/mL; and inactive for values greater than 50 g/mL (Philippe et al., 2005). The highest activity was observed with the chloroquine-sensitive strain with an IC50 of 0.029 ± 0.0004 µg/mL and 0.64 ± 0.08 µg/mL on the resistant strain. These findings are consistent with those of Nadia et al. (2020), who tested Bidens pilosa and found it to be highly active against the chloroquine resistant strain. This difference observed between the antiplasmodial activity of the two extracts could be linked to the difference in the Plasmodium strains on which the extracts were evaluated and also to the chemical composition of the plant, which may vary according to certain characteristics such as climatic and edaphic factors (Wabo et al., 2011). This could also be explained by the nature of the solvent used for the extraction. According to Koagne et al. (Houari, 2006), flavonoids could lead to more useful derivatives for the development of an antiplasmodial agent and have antiplasmodial properties.
The level of parasitemia was reduced in a dose-dependent manner, which is an indicator of the antiplasmodial activity of E. sigmoidea. These percentages of inhibition were considered very active according to the classification of Munoz et al. (2000), which stipulates that an extract is considered active if its inhibition is ≥30%. This observation was also reported by Zaruwa et al. (2018) when evaluating the suppressive activity of the ethanol extract of Achyranthes aspera and Ficus thoningii on the development of P. berghei on “Swiss” mice. The reduction of parasitemia was also reported by Kaur et al. (2021) on the effect of the hydroethanolic extract of B. variegata on Leishmania donovani. Similarly, Olasehinde et al. (2016) in Nigeria evaluated the suppressive activity of the ethanol extract of Moringa oleifera on the model of mouse M. musculus. On the other hand, Camara (2020) presented a weak inhibition of development of P. berghei with the ethanol extracts of Terminalia albida from Kankan and Rourea minor. Inhibition was 13% for R. minor at 65 mg/kg and 14% for T. albida Kankan at 100 mg/kg. This low inhibition could be due to the diversity of molecules present in plants. The phytochemical constituents might have acted by destroying the parasite cytosol, membrane, mitochondria, and digestive vacuole of the parasites.
The negative control recorded the highest mortality rate (83.33%). Our results are in line with those obtained by Camara (2020) when evaluating the suppressive activity of D. velutinum extracts and R. minor on P. berghei with greater mortality in the negative control with 100% death on the 8th day. Similarly, Adebajo et al. (2014) in the suppressive test also recorded greater mortality in the untreated group. This could be justified by the fact that the negative control group is the infected and untreated group.
A significant difference (p < 0.05) was seen in weight between the treatment groups and the control group. When compared to the untreated group, the treated groups lost less weight. These findings support those made by Sulaiman et al. (Rukayyah et al., 2015). A little weight gain was observed in the groups that got the extract at various doses during the post-treatment period. This shows that this plant might have compounds that are useful for treating malnutrition in children and preventing vitamin deficits. Our results are similar to those of Camara (2020) when evaluating the weight of animals after treatment with the Desmodium velutinum extract and R. minor during the suppressive test as well as the work of Ma et al. (2011) who also presented D. velutinum (Fabaceae) as a plant fighting against nutritional deficiencies.
Hematological parameters are reliable parameters for assessing the state of health. White blood cells play a role in immune defense against foreign bodies typically through leukocytosis and antibody production in infected and untreated animals. This observation was made by Noumedem et al. (Nadia et al., 2020) with B. pilosa where the average white blood cell count was higher in the untreated group compared to the other treated groups. The anemia observed in infected and untreated animals could be due to the destruction of red blood cells, itself caused by the multiplication of the parasite. The rate of hematocrit is directly related to anemia. This result corroborates with those of Okombé (Okombe et al., 2011) obtained with the powder of Vitex thomasii and indicates that the products help animals withstand the effects of parasitism.
During the curative test, the inhibitions were lower compared to the inhibitions obtained in the suppressive test. During the 3 days without treatment, the plasmodia may have developed and adapted better to their environment. No inhibition was obtained in the untreated group. Also, Omagha et al. (2021) also demonstrated inhibition of the parasitemia with a mixture of two cocktails of plants commonly used in southwestern Nigeria. This justifies their use in the treatment of malaria in Western Cameroon. This inhibition may be due to the diversity of molecules present in plants.
The mortalities recorded in the groups receiving the treatments are not totally linked to the hyperparasitemia but to an immunopathology that promotes the accumulation of immune cells in the brain and the dysfunction of the organ and leads to symptoms such as paralysis and head deviation; this is in line with the results obtained by Musila et al. (2013), who recorded 100% death in the untreated group on the 10th day. On the 25th day, we recorded 100% mortality in the untreated group and the group that received the extract at a dose of 125 mg/kg. This is due to the resurgence of the parasite due to a low dose of the extract administered. This result corroborates those found by Haidara et al. (2016). This would be justified by the fact that the negative control group is the infected and untreated group.
5 Conclusion
The present study substantiates local claims about the efficacy of E. sigmoidea in the treatment of malarial infection. The ethanol extract of E. sigmoidea has showed in vitro and in vivo antimalarial properties and have a higher reduction rate of parasitemia on the inhibition of the development of P. berghei NK65 strain, which justifies its use in traditional medicine for the treatment of malaria. However, it will be necessary to evaluate the safety of E. sigmoidea in order to assess its toxicity.
Data availability statement
The raw data supporting the conclusions of this article will be made available by the authors, without undue reservation.
Ethics statement
All authors hereby declare that the "Principles for the Care of Laboratory Animals" (NIH Publication No. 85-23, revised 1985) have been followed, as well as specific national laws, where applicable. All experiments were reviewed and approved by the Department of Animal Biology, Faculty of Science, University of Dschang.
Author contributions
TS: Conceptualization, Investigation, Writing – original draft. NN: Conceptualization, Supervision, Writing – review & editing. YC: Conceptualization, Supervision, Writing – review & editing. GG-A: Data curation, Investigation, Writing – original draft. MA: Conceptualization, Investigation, Writing – original draft. NS: Data curation, Investigation, Methodology, Writing – original draft, Writing – review & editing. TK: Conceptualization, Data curation, Investigation, Writing – original draft. VP: Methodology, Supervision, Writing – review & editing. HH: Data curation, Methodology, Supervision, Conceptualization, Writing – original draft, Writing – review & editing.
Funding
The author(s) declare financial support was received for the research, authorship, and/or publication of this article. This work was supported by the Laboratory of Tropical and Emerging Infectious Diseases (LTEID) in terms of chemicals and equipment’s put in place for this research. The study received funding from the Scientific Research Key Project of the Jiangxi Education Department (No. GJJ211501) for chemical reagents.
Acknowledgments
We would like to thank the BEI Resources for the donation of the Plasmodium berghei NK 65 strain used in this work. We also want to express our gratitude to Prof. Walter Luyten of Belgium, who gave us the tools and chemicals we needed to do this research.
Conflict of interest
The authors declare that the research was conducted in the absence of any commercial or financial relationships that could be construed as a potential conflict of interest.
Publisher’s note
All claims expressed in this article are solely those of the authors and do not necessarily represent those of their affiliated organizations, or those of the publisher, the editors and the reviewers. Any product that may be evaluated in this article, or claim that may be made by its manufacturer, is not guaranteed or endorsed by the publisher.
References
Adebajo A. C., Odediran S. A., Aliyu F. A., Nwafor P. A., Nwoko N. T., Umana U. S. (2014). In vivo antiplasmodial potentials of the combinations of four Nigerian antimalarial plants. Molecules 19, 13136–13146. doi: 10.3390/molecules190913136
Antonio-Nkondjio C., Ndo C., Njiokou F., Bigoga J. D., Awono-Ambene P., Etang J., et al. (2019). Review of malaria situation in Cameroon: technical viewpoint on challenges and prospects for disease elimination. Parasites Vectors 12, 1–23. doi: 10.1186/s13071-019-3753-8
Balaji S., Deshmukh R., Trivedi V. (2020). Severe malaria: Biology, clinical manifestation, pathogenesis and consequences. J. Vector. Borne. Dis. 57, 1–1. doi: 10.4103/0972-9062.308793
Camara A. (2020). Plantes médicinales guinéennes: validation de l’effet antipaludique et impact sur la modulation de l’immunité (Conakry: Université Paul Sabatier-Toulouse III; Université Gamal Abdel Nasser).
Ceravolo I. P., Aguiar A. C., Adebayo J. O., Krettli A. U. (2021). Studies on activities and chemical characterization of medicinal plants in search for new Antimalarials: a ten year review on Ethnopharmacology. Front. Pharmacol. 12, 734263. doi: 10.3389/fphar.2021.734263
Gilmartin C., Nonvignon J., Cairns M., Milligan P., Bocoum F., Winskill P., et al. (2021). Seasonal malaria chemoprevention in the Sahel subregion of Africa: a cost-effectiveness and cost-savings analysis. Lancet Global Health 9, e199–e208. doi: 10.1016/S2214-109X(20)30475-7
Haidara M., Bourdy G., De Tommasi N., Braca A., Traore K., Giani S., et al. (2016). Medicinal plants used in Mali for the treatment of malaria and liver diseases. Natural Product. Commun. 11, 1934578X1601100309. doi: 10.1177/1934578X1601100309
Houari K. D. (2006). Impact de la nature des sols sahariens sur la composition chimique de quelques plantes de la région de Ouargla (Ouargla: Université de Kasdi Merbah. Faculté des Sciences et Sciences de l …).
Jones R. A., Panda S. S., Hall C. D. (2015). Quinine conjugates and quinine analogues as potential antimalarial agents. Eur. J. Med. Chem. 97, 335–355. doi: 10.1016/j.ejmech.2015.02.002
Kaur G., Chauhan K., Anand N., Kaur S. (2021). Evaluation of in vitro and in vivo protective efficacy of Bauhinia variegata against Leishmania donovani in Murine Model. Acta Parasitol. p, 1–15. doi: 10.1007/s11686-020-00326-8
Kevin T. D. A., Cedric Y., Nadia N. A. C., Sidiki N. N. A., Azizi M. A., Guy-Armand G. N., et al. (2023). Antiplasmodial, antioxidant, and cytotoxic activity of bridelia micrantha a Cameroonian medicinal plant used for the treatment of malaria. BioMed. Res. Int. 2023.
Kolawole E. O., Ayeni E. T., Abolade S. A., Ugwu S. E., Awoyinka T. B., Ofeh A. S., et al. (2023). Malaria endemicity in Sub-Saharan Africa: Past and present issues in public health. Microbes Infect. Dis. 4, 242–251.
Liu Q., Yan W., Qin C., Du M., Liu M., Liu J. (2022). Millions of excess cases and thousands of excess deaths of malaria occurred globally in 2020 during the COVID-19 pandemic. J. Global Health 12. doi: 10.7189/jogh.12.05045
Ma X., Zheng C., Hu C., Rahman K., Qin L. (2011). The genus Desmodium (Fabaceae)-traditional uses in Chinese medicine, phytochemistry and pharmacology. J. Ethnopharmacol. 138, 314–332. doi: 10.1016/j.jep.2011.09.053
Mahmoudvand H., Khalaf A. K., Rajabi P. Z., Karbasian N., Ghasemian Yadegari J. (2023). Leishmanicidal and immunomodulatory activities of the formononetin (a natural isoflavone) against Leishmania tropica. BMC Res. Notes 16, 1–6. doi: 10.1186/s13104-023-06403-1
Munoz V., Sauvain M., Bourdy G., Callapa J., Rojas I., Vargas L., et al. (2000). The search for natural bioactive compounds through a multidisciplinary approach in Bolivia. Part II. Antimalarial activity of some plants used by Mosetene Indians. J. Ethnopharmacol. 69, 139–155. doi: 10.1016/S0378-8741(99)00096-3
Murray C. J., Laakso T., Shibuya K., Hill K., Lopez A. D. (2007). Can we achieve Millennium Development Goal 4? New analysis of country trends and forecasts of under-5 mortality to 2015. Lancet 370, 1040–1054. doi: 10.1016/S0140-6736(07)61478-0
Musila M., Dossaji S., Nguta J., Lukhoba C., Munyao J. (2013). In vivo antimalarial activity, toxicity and phytochemical screening of selected antimalarial plants. J. Ethnopharmacol. 146, 557–561. doi: 10.1016/j.jep.2013.01.023
Na-Bangchang K., Karbwang J. (2009). Current status of malaria chemotherapy and the role of pharmacology in antimalarial drug research and development. Fundam. Clin. Pharmacol. 23, 387–409. doi: 10.1111/j.1472-8206.2009.00709.x
Nadia N. A. C., Cédric Y., Raoul S. N. S., Christian N. O., Azizi M. A., Diane G. D. C., et al. (2020). Antimalarial activity of ethyl acetate extract and fraction of Bidens pilosa against Plasmodium berghei (ANKA). J. Parasitol. Res. 2020. doi: 10.1155/2020/8832724
Narayanan A., Sindhe K. M., Rai L. S. (2019). Drug Resistance in Plasmodium sp. and Novel Antimalarial Natural Products-Emerging Trends. Phytochemistry: An in-silico and in-vitro Update: Advances in Phytochemical Research. 95–108.
Okombe E., Pongombo S., Duez P. (2011). “Anthelmintic activity of Vitex thomasii de Wild (Verbenaceae) root bark powder on Haemonchus contortus in goats,” in HERBAN (Herbal products in animal health and nutrition). (University of Lubumbashi: PhD thesis)
Olasehinde G., Ayanda O., Egwari L., Ajayi A., Awofeso T. (2016). In vivo antiplasmodial activity of crude ethanolic and N-hexane extracts of Moringa oleifera leaves. Int. J. Agric. Biol. 18, 906–910. doi: 10.17957/IJAB
Omagha R., Idowu E. T., Alimba C. G., Otubanjo O. A., Oyibo W. A., Agbaje E. O. (2021). In vivo antiplasmodial activities and acute toxicity assessment of two plant cocktail extracts commonly used among Southwestern Nigerians. J. Parasitic. Dis. p, 1–11. doi: 10.21203/rs.3.rs-366502/v1
Ouji M., Augereau J.-M., Paloque L., Benoit-Vical F. (2018). Plasmodium falciparum resistance to artemisinin-based combination therapies: A sword of Damocles in the path toward malaria elimination. Parasite 25. doi: 10.1051/parasite/2018021
Peters W. (1975). The four-day suppressive in vivo antimalarial test. Ann. Trop. Med. Parasitol. 69, 155–171.
Philippe G., Angenot L., De Mol P., Goffin E., Hayette M.-P., Tits M., et al. (2005). In vitro screening of some Strychnos species for antiplasmodial activity. J. Ethnopharmacol. 97, 535–539. doi: 10.1016/j.jep.2004.12.011
Rukayyah S. S., Jigam A. A., Aisha M. T. (2015). In vivo antiplasmodial and effects of subchronic administration of Trichilia emetica Leaves Extracts. Int. J. Natural Sci. Res. 3, 1–15.
Ryley J., Peters W. (1970). The antimalarial activity of some quinolone esters. Ann. Trop. Med. Parasitol. 64, 209–222. doi: 10.1080/00034983.1970.11686683
Sato S. (2021). Plasmodium—a brief introduction to the parasites causing human malaria and their basic biology. J. Physiol. Anthropol. 40, 1–13. doi: 10.1186/s40101-020-00251-9
Shaikh J. R., Patil M. (2020). Qualitative tests for preliminary phytochemical screening: An overview. Int. J. Chem. Stud. 8, 603–608. doi: 10.22271/chemi
Shiff C. (2002). Integrated approach to malaria control. Clin. Microbiol. Rev. 15, 278–293. doi: 10.1128/CMR.15.2.278-293.2002
Sidiki N. N. A., Nadia N. A. C., Cedric Y., Guy-Armand G. N., Sandra T. N. J., Kevin T. D. A., et al. (2023). Antimalarial and antioxidant activities of ethanolic stem bark extract of Terminalia macroptera in Swiss albino mice infected with Plasmodium berghei. J. Parasitol. Res. 2023. doi: 10.1155/2023/3350293
Singh N., Kaushik N. K., Mohanakrishnan D., Tiwari S. K., Sahal D. (2015). Antiplasmodial activity of medicinal plants from Chhotanagpur plateau, Jharkhand, India. J. Ethnopharmacol. 165, 152–162. doi: 10.1016/j.jep.2015.02.038
Trager W., Jensen J. B. (1997). Continuous culture of Plasmodium falciparum: its impact on malaria research. Int. J. Parasitol. 27, 989–1006. doi: 10.1016/S0020-7519(97)00080-5
Wabo P. J., Ngankam N. J., Bilong B. C., Mpoame M. (2011). A comparative study of the ovicidal and larvicidal activities of aqueous and ethanolic extracts of pawpaw seeds Carica papaya (Caricaceae) on Heligmosomoides bakeri. Asian Pacific. J. Trop. Med. 4, 447–450. doi: 10.1016/S1995-7645(11)60123-5
Wongsrichanalai C., Sibley C. (2013). Fighting drug-resistant Plasmodium falciparum: the challenge of artemisinin resistance. Clin. Microbiol. Infect. 19, 908–916. doi: 10.1111/1469-0691.12316
Keywords: antiplasmodial, plasmodium, Erythrina sigmoidea, in vivo, in vitro, Cameroon
Citation: Jemimah Sandra TN, Christelle Nadia NA, Cedric Y, Guy-Armand GN, Azizi MA, Aboubakar Sidiki NN, Alex Kevin TD, Payne VK and Hu H (2024) In vitro and in vivo antimalarial activities of the ethanol extract of Erythrina sigmoidea stem bark used for the treatment of malaria in the Western Region of Cameroon. Front. Parasitol. 3:1359442. doi: 10.3389/fpara.2024.1359442
Received: 21 December 2023; Accepted: 12 March 2024;
Published: 09 April 2024.
Edited by:
Josue De Moraes, Guarulhos University, BrazilReviewed by:
Namrata Anand, University of Kentucky, United StatesDrissa Konate, Université des Sciences, des Techniques et des Technologies de Bamako, Mali
Copyright © 2024 Jemimah Sandra, Christelle Nadia, Cedric, Guy-Armand, Azizi, Aboubakar Sidiki, Alex Kevin, Payne and Hu. This is an open-access article distributed under the terms of the Creative Commons Attribution License (CC BY). The use, distribution or reproduction in other forums is permitted, provided the original author(s) and the copyright owner(s) are credited and that the original publication in this journal is cited, in accordance with accepted academic practice. No use, distribution or reproduction is permitted which does not comply with these terms.
*Correspondence: Yamssi Cedric, Y3lhbXNzaUB5YWhvby5jb20=; Noumedem Anangmo Christelle Nadia, bm91bXMxMUB5YWhvby5mcg==; Haibo Hu, aGhiMjAxN0BnbXUuZWR1LmNu; Vincent Khan Payne, dmsucGF5bmVAeWFob28uY29t