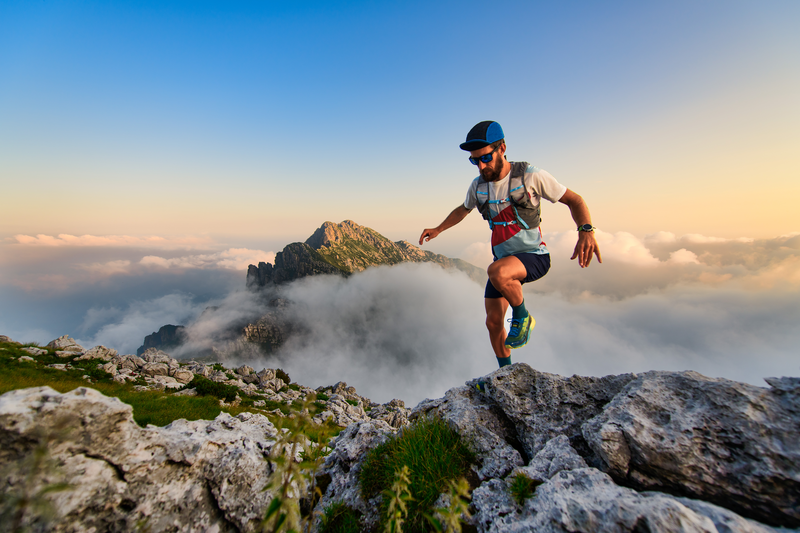
94% of researchers rate our articles as excellent or good
Learn more about the work of our research integrity team to safeguard the quality of each article we publish.
Find out more
MINI REVIEW article
Front. Parasitol. , 26 September 2023
Sec. Parasite Diagnostics
Volume 2 - 2023 | https://doi.org/10.3389/fpara.2023.1260224
This article is part of the Research Topic Polymerase Chain Reaction technique for Chagas Disease: challenges and limitations View all 6 articles
Chagas disease (CD) caused by Trypanosoma cruzi remains a Neglected Tropical Disease with limited access to diagnosis and treatment, particularly for chronically infected patients. Clinical trials are underway to improve treatment using new drugs or different regimens, and Real-Time PCR is used to assess the parasitological response as a surrogate biomarker. However, PCR-based strategies have limitations due to the complex nature of T. cruzi infection. The parasite exhibits asynchronous replication, different strains and clones, and diverse tissue tropism, making it challenging to determine optimal timeline points for monitoring treatment response. This mini-review explores factors that affect PCR-based monitoring and summarizes the endpoints used in clinical trials for detecting treatment failure. Serial sampling and cumulative PCR results may improve sensitivity in detecting parasitemia and treatment failure in these trials.
Chagas disease (CD), caused by the protozoan Trypanosoma cruzi, poses a significant public health threat in many regions worldwide (WHO, 2023). Transmission to humans primarily occurs through triatomine vectors, but other routes such as in utero transmission, contaminated meals, blood transfusions, or organ transplants are also possible. CD remains classified as a Neglected Tropical Disease, with limited access to efficient diagnosis and treatment, hampering efforts to control its impact on affected populations.
Current available therapies, such as benznidazole (BZN) and nifurtimox (NFX) have limitations in efficacy and safety, particularly in chronic adult CD patients (Moraes et al., 2014).
Clinical trials using different drug regimens and exploring new drugs in the pharmaceutical industry portfolio are underway to address this issue (Morillo et al., 2015; Morillo et al., 2017; Villar et al., 2019; Cafferata et al., 2020; Alonso-Vega et al., 2021; Torrico et al., 2021; Altcheh et al., 2023).
One of the challenges in assessing treatment efficacy is the reliance on molecular methods, particularly Real-Time PCR, to detect parasitic loads in peripheral blood (Schijman et al., 2011; Moreira et al., 2013; Ramírez et al., 2015). These methods have a limit of detection, and non-detectable findings cannot confirm parasitological eradication, leading to cure. Only detectable results can indicate treatment failure. This limitation hampers the ability to accurately determine the success of therapeutic interventions.
The lifecycle of T. cruzi further complicates the assessment of treatment effectiveness. Infection begins with motile extracellular trypomastigotes, which invade different cell types and convert into amastigotes, replicating in the host cytoplasm. Amastigotes then convert back to trypomastigotes, breaking the host cell membrane for parasite dissemination to the interstice and bloodstream. Recent studies have highlighted the asynchronous nature of intracellular parasite replication, the occurrence of dormancy and amastigotes’ growth plasticity to adapt and recover from sublethal drug exposure (Dumoulin and Burleigh, 2018; Sanchez-Valdez et al., 2018; Ward et al., 2020).
Another critical factor in treatment response is the genetic diversity and population structure of T. cruzi. CD patients may be infected with multiple parasite strains and clones, each with different tissue tropism (Macedo and Pena, 1998). The presence of parasites in the bloodstream may not necessarily reflect the ones responsible for clinical manifestations and drug susceptibilities. Different subpopulations of the parasite may be responsible for variations in treatment responses observed in multicenter trials.
Moreover, certain life-cycle stages may be less sensitive to treatment, and the ability of parasites to reside in metabolically distinct tissue compartments can significantly affect drug susceptibility (Barrett et al., 2019). These complexities highlight the need for more comprehensive approaches when evaluating treatment efficacy and drug response in CD.
Benznidazole and nifurtimox exhibit pleiotropic properties with multiple effects on the target organism. This complexity can give rise to resistance in various ways. It is important to note that T.cruzi resistance observed in laboratory settings where in vitro resistance is generated may differ significantly from the resistance patterns observed in natural populations. This underscores the need of considering the interplay between laboratory studies and real-world observations when addressing drug resistance in T.cruzi.
The intracellular life cycle of T. cruzi in vivo is more intricate than previously assumed, revealing varying degrees of drug susceptibility among different parasite stages.
The research conducted by Moraes et al. (2014) highlights that trypomastigote forms have a higher capacity to withstand the trypanocidal effects of BZN and NFX compared to replicative epimastigotes and amastigotes. On the other hand, epimastigotes and amastigotes are more susceptible to a cumulative, trypanocidal, and trypanostatic impact of these drugs.
Revollo et al. (2019) investigated the susceptibility patterns of T. cruzi stages to BZN and NFX in vitro using a range of twenty-one T. cruzi strains from three different DTUs (Discrete Typing Units) isolated from patients, reservoirs, and triatomines across various geographical origins (Revollo et al., 1998). On the basis of the Epidemiological cut-off value (CO wt) (Kahlmeter, 2014), the authors computed the susceptibility threshold (COwt) of the T. cruzi life cycle forms against BZN and NFX from a panel of previously characterized strains and observed that the trypomastigote form exhibits higher tolerance to the toxic effects of both drugs compared to the other stages.
The study by Dumoulin and Burleigh (2018) revealed that intracellular amastigotes have the ability to adjust their proliferation rates as a survival strategy. They can range from minimal to rapid growth by accumulating within the G1 phase and then being released. The variation in doubling times differed between T.cruzi strains, suggesting that the metabolite levels or their thresholds, needed to initiate the S phase were also different in different strains. Treatment with BZN was found to inhibit amastigote proliferation in a concentration-dependent manner. Interestingly, when the drug was removed, the recovery of amastigotes was inversely proportional to the drug concentration used to treat them (Dumoulin and Burleigh, 2018).
Studies have shown that T. cruzi strains isolated from triatomine vectors and vertebrate hosts exhibit multiclonality (Morel et al., 1986; Oliveira et al., 1998). During the interaction between the parasite and the human host in the chronic infection, certain subpopulations of T. cruzi may be selected and their differential tissue tropism can affect their distribution in the infected host (Vago et al., 2000). This selective role played by the human host can be exemplified by studies done in parasite isolates obtained from clinical samples of the first human CD case reported by Carlos Chagas: a two year-old girl named Berenice (Chagas, 1909). Indeed, two T. cruzi populations were isolated from Berenice Soares de Moura: one when she was a 55 years old- woman and another one when she reached 71 years of age (De Lana et al., 1996). The zymodeme and schizodeme profiles of these isolates diverged, which could be attributed to either a reinfection with a different strain or the presence of a heterogeneous population since the primary infection. Indeed, as T. cruzi is composed of heterogeneous populations, it is possible for the same host to be simultaneously infected by different strains. The clonal histotropic model suggests that the heterogeneity and multiclonality of a strain determine its differential tissue tropism, leading to variations in the clinical presentation of the disease (Macedo and Pena, 1998). It has been observed that clones found in patients’ cardiac tissues differed from those in the esophagus (Vago et al., 2000), and divergent subpopulations were identified in different tissue slices from the same heart explant in patients undergoing heart transplant (Burgos et al., 2010). Additionally, the occasional reactivation of parasites residing in target tissues may contribute to the dynamic behavior of T. cruzi infection, resulting in the appearance of new replication sites in different anatomical locations, as frequently observed in immunosuppressed patients with Chagas reactivation leading to panniculitis, myocarditis, or meningoencephalitis (Burgos et al., 2008; Burgos et al., 2010).
It has been reported that the epimastigote and amastigote forms of TcI strains are significantly less sensitive to BZN or NFX- mediated growth inhibitory effect compared to those belonging to TcII and TcV (Revollo et al., 2019). Strains CL (TcVI) and Colombiana (TcI), known to be susceptible and highly resistant, respectively, to BZN and NFX in vivo (Filardi and Brener, 1987), exhibit similar in vitro susceptibility in the amastigote stage (Canavaci et al., 2010).
In vitro experiments conducted by MacLean et al. (2018) demonstrated that strain PAH179 (TcV) exhibited marked resistance to posaconazole. This reduced sensitivity was attributed to the slow doubling and cycling time of this strain, which resulted in ergosterol biosynthesis inhibition by posaconazole only after multiple rounds of division. The lack of effect of posaconazole on the non-replicative trypomastigote form further supported this observation.
Characterization of transcriptomic profiles of BZN-resistant T. cruzi clones has revealed a wide array of genes from different metabolic pathways associated with the BZ-resistant phenotype. This indicates that parasite resistance mechanisms are multifactorial and complex (García-Huertas et al., 2017; Lima et al., 2023). Functional analysis has enabled the identification of relevant biological processes linked to the resistance phenotype, including changes in RNA processing and translation, antioxidant defence, as well as inter- and intracellular molecular transport (Lima et al., 2023).
Parasite genotyping can aid in understanding the differences in outcomes observed across different trials conducted in various geographical regions, as well as in detecting naturally resistant populations (Parrado et al., 2019; Muñoz-Calderón et al., 2023). Biorepositories containing well-conserved blood samples and extracted DNAs can be valuable for typing parasite populations from previous trials. The DNDi-CH-E1224-001 (Ramírez et al., 2022) and TESEO trials (Alonso-Vega et al., 2021) have incorporated DTU genotyping into their study designs.
Sanchez-Valdez et al. (2018) made a ground breaking discovery by identifying drug-resistant persister subpopulations in T. cruzi. These dormant parasites have the unique characteristic of either not replicating or displaying slow replication rates. Moreover, they undergo metabolic changes, including reduced DNA synthesis and widespread downregulation of protein translation, in comparison to actively replicating cells (as shown in the studies by Van den Bergh et al., 2017, and Barrett et al., 2019).
There is a need to consider the potential role of dormancy mechanisms in the context of chronic infections. As the infection progresses to its chronic stage the parasite may encounter conditions that reduce its replication rate. For instance, certain types of DNA damage in T.cruzi such as double-strand breaks can trigger cell cycle arrest followed by repair without leading to parasite cell death. This repair process might inadvertently contribute to a dormancy-like state, where the parasite remains metabolically active but exhibits limited replication (Repolês et al, 2020).Dormant forms can arise spontaneously, independent of any drug pressure. Additionally, they possess a remarkable resilience to compounds that are highly effective against the actively replicating parasites.
The inability of trypanocidal drugs to completely eradicate replicative amastigote nests suggests the occurrence of transient dormancy within a specific subpopulation among the intracellular amastigote forms. These dormant cells can enter a state of quiescence during drug treatment and have the potential to resume replication once the treatment is stopped. Taylor and coworkers (2020) have hypothesized that the presence of such dormant nests may function as a form of intracellular “herd-protection,” where those parasite cells situated in an inner core of an amastigote nest would be less exposed to the drug compared to those amastigotes located at the periphery. As a result, the drug concentration within the host cell may be depleted before it can effectively target the core amastigotes, contributing to the survival and persistence of the dormant parasites. Such a phenomenon would pose challenges in completely eradicating T. cruzi infections and underscore the need for novel therapeutic approaches targeting these drug-resistant persister subpopulations.
In vitro studies using TUNEL assays and 5-ethynyl-2’-deoxyuridine (EdU) labeling have shown that replicating amastigotes, amastigote-to-trypomastigote differentiating forms and non-replicative trypomastigotes can coexist concurrently in a same host cell. The dynamics of parasite replication in each host cell does not follow a predictable or tightly regulated pattern, either in vitro or in vivo, at any phase of the disease or in specific infected tissues (Taylor et al., 2020).
Barrett et al. (2019) conducted a review of mechanisms used by protozoan parasite stages to enter a dormant state and establish persistent infections. To study the proliferation of T. cruzi in the colon of chronically infected mice, they incorporated EdU into DNA to track the replication status. By imaging infection foci at the single-cell level, they discovered that T. cruzi parasites were three times more likely to be in the S-phase during the acute stage of murine infection in the colon compared to the chronic stage. This finding suggested that a lower rate of parasite replication was associated with chronic colonic infections. Interestingly, the majority of infected murine cells did not survive T. cruzi infection for more than 14 days, indicating that persistence involved regular cycles of replication, host cell lysis, and reinfection (as mentioned in the study by Ward et al., 2020). This insight sheds light on the dynamics of T. cruzi infection and the potential mechanisms of persistence within the host.
Real-Time PCR remains the main laboratory tool for assessing treatment efficacy in clinical trials for Chagas disease. It is crucial to have well-standardized DNA extraction methods and commercially available Real-Time PCR kits, accompanied by internal and external quality controls (Ramírez et al., 2015; Ramírez et al., 2017) and reliable standard curves for quantification for expressing parasitic loads or target gene copies per unit of sample volume (Ramírez et al, 2015; Muñoz-Calderón et al., 2021). Quantitative PCR would permit establishing a minimal difference in parasitic loads between subsequent samples of a same patient under follow-up to interpret it as a true decrease or increase in parasitemia and accordingly treatment response improvement or failure.
In clinical practice, the concept of the “minimum clinically significant difference” (MCID) is often used to determine whether a change in a measured parameter, such as parasitic load, is meaningful from a clinical perspective. The MCID is the smallest change in a measurement that is considered to be clinically relevant and not just due to random variability. This depends on several factors, including the specific parasite population, DTU or strain being studied, the testing method used, and the clinical context. It is essential to consider the sensitivity and precision of the testing method, the variability in the measurement, and the natural course of the disease. However, this has not been done in the context of Chagas disease trials, yet.
Harmonization and agreement on criteria for using PCR in clinical trials for Chagas disease can be challenging due to various factors, including scientific, practical, and logistical considerations. Some debatable points that may arise during discussions and negotiations for harmonization are presented in Table 1.
Table 1 Debatable points regarding harmonization and agreement on criteria for using Polymerase Chain Reaction (PCR) in clinical trials for Chagas disease.
The minimum set of PCR timepoints required to detect treatment failure early needs to be agreed upon by the community involved in Chagas disease trials. Some trials have included a positive PCR result at baseline as an inclusion criterion for patients in a treatment arm. The clinical sensitivity of PCR in the chronic stage varies between 50% and 70% and is dependent on the geographical region. This has been observed by comparing PCR positivity and parasitic loads in baseline samples from patients enrolled in multicentric trials. For example, in the BENEFIT trial, treatment with benznidazole (BZN) was effective for patients from Argentina and Brazil (80% of the study population) but not for participants from Colombia and El Salvador (Morillo et al., 2015). This difference could be related to the distribution of parasite strains and DTUs (Discrete Typing Units), which can have different gene dosage for the molecular targets used in PCR. Furthermore, in certain regions, such as Minas Gerais, Brazil, primary resistant strains may prevail.
Serial sampling studies have been conducted in an attempt to increase the clinical sensitivity of PCR for detecting parasitic loads in pre-treated chronic Chagas disease patients (Parrado et al., 2019). In the DNDi-CH-E1224-001 trial, up to three samples were collected at each timepoint. Samples 1 and 2 were collected on the same day, with the former containing 10 ml and the second one containing 5 ml of blood in both cases mixed with one volume of the stabilizing agent. A third sample (10 ml) was collected seven days later if the PCR results from the previous two ones were non-detectable. The timing of the third sample collection was based on the assumption that T. cruzi intracellular replication generally lasts for 4-5 days, although it can be slower for certain isolates (Dumoulin and Burleigh, 2018).
Comparison of PCR positivity between individual samples did not show significant differences, indicating that collecting 5 ml or 10 ml of blood did not impact PCR results in that cohort of chronically infected patients. However, when cumulative results from the combination of the first two samples were computed, higher PCR positivity rates were observed in both cohorts. In cases where samples 1 and 2 were PCR negative, the inclusion of the third sample collected one week later increased sensitivity. Indeed, increasing the number of samples during screening allows enrolling a higher number of patients within the same project period.
In the MSF-DNDi PCR Sampling Optimization Study (Parrado et al., 2019), three samples were taken from all patients regardless of the PCR results in the first two. No statistical differences were observed when testing individual samples. However, when the cumulative PCR positivity of sample 1 plus 2 was compared to the positivity of either of them as a single test, an increase in sensitivity was observed. This was also true when considering the combination of all three samples, resulting in higher PCR positivity rates.
The fluctuation of PCR positivity and non-detectable results can be attributed to the low parasitic burden in chronic Chagas disease patients, which often falls below the limits of detection and quantification of available PCR techniques. In the DNDi-CH-E1224-001 trial, quantitative PCR results showed fluctuations in parasitic loads among positive PCR samples from the same patient, with some samples falling below the limit of quantification. The decision on the optimal opportunity to take an additional sample for PCR testing is currently based on operational and logistical factors rather than in the probability of detecting a particular percentage of additional positives based on the replicative dynamics of the parasite population. This is also applicable to the detection of treatment failure, as conducting more PCR determinations increases the chances of detecting failures.
In reported clinical trials, the measurement of DNAemia at the end of treatment (EOT) regimens was not useful for assessing treatment response. In the DNDi-CH-E1224-001 trial, there was a rapid response in all treatment arms at EOT, but differences in the degree of treatment failure became clear approximately 100 days after treatment initiation. Parasitemia appeared to remain dynamic for up to 6 months after treatment and became more stable afterward. This is likely because some time was needed for refractory or resistant parasite populations to reach a detectable parasitic load threshold in the bloodstream using available PCR procedures.
Different clinical trials have used PCR as an endpoint to evaluate treatment efficacy. In the BENDITA trial, sustained parasitological clearance at six months, defined as persistent negative qualitative PCR results since EOT, was the primary endpoint (Torrico et al., 2018).
The STOP CHAGAS trial evaluated the proportion of subjects with persistent negative PCR by day 180 as the primary efficacy outcome, with negative PCR at 360 days as a secondary outcome (Morillo et al., 2015; Morillo et al., 2017). The EQUITY trial assessed the proportion of enrolled patients testing positive at least once in up to three independent PCR assays, separated by seven days between each, carried out between 12 and 18 months after randomization (Villar et al., 2019).
Currently, clinical trials include PCR measurements at six and twelve months after EOT as common timepoints for post-treatment PCR assessments (Table 2).
Table 2 PCR timeline points in current clinical trials evaluating regimens of BZN or NFX in chronic CD adult patients.
In recent years, the landscape of DNA amplification and detection techniques has expanded to included highly sensitive isothermal amplification methods such as Loop mediated isothermal amplification (LAMP) and CRISPR-Cas12 technology. Cas12 is a a RNA-guided endonuclease enzyme found in CRISPR-Cas systems, which has been harnessed for its ability to target specific DNA sequences (You et al., 2022). When paired with a guide RNA complementary to the target DNA, Cas12 can be activated upon binding to the target resulting in a collateral cleavage activity that generates detectable signals, such as fluorescence. LAMP employs a set of four to six primers designed to recognize multiple distinct regions on the target DNA enabling a strand-displacement DNA synthesis process. A T.cruzi LAMP prototype kit (Besuschio et al., 2017; Besuschio et al., 2020) has been already tested in blood samples from CD patients under treatment, with promising sensitivity and specificity and high agreement with Real Time PCR used as a comparator test (Muñoz-Calderon et al., 2022).
In conclusion, the complexity of T.cruzi infection, including the coexistence of multiple clones, strains, and discrete typing units in a specific geographic region and patient cohort, as well as the differential histotropism and lack of synchrony among life-stage cycles, poses challenges in predicting an optimal timeline for Real-Time PCR monitoring and assessing the overall parasitological response to treatment. Given these complexities, it is reasonable to maintain PCR tests at baseline, as well as at six and twelve months post-treatment, in order to facilitate the comparison of parasitological responses among different drugs and treatment regimens. This approach allows for a more comprehensive evaluation of treatment efficacy and response in CD clinical trials.
AS: Conceptualization, Funding acquisition, Writing – original draft, Investigation, Writing – review & editing.
The author declares that financial support was received for the research, authorship, and/or publication of this article from PICT2020-00862, MinCyT, Argentina and LEG ID39002 Small Grant Programme from PAHO.
The author declares that the research was conducted in the absence of any commercial or financial relationships that could be construed as a potential conflict of interest.
All claims expressed in this article are solely those of the authors and do not necessarily represent those of their affiliated organizations, or those of the publisher, the editors and the reviewers. Any product that may be evaluated in this article, or claim that may be made by its manufacturer, is not guaranteed or endorsed by the publisher.
Alonso-Vega C., Urbina J. A., Sanz S., Pinazo M. J., Pinto J. J., Gonzalez V. R., et al. (2021). New chemotherapy regimens and biomarkers for Chagas disease: the rationale and design of the TESEO study, an open-label, randomised, prospective, phase-2 clinical trial in the Plurinational State of Bolivia. BMJ Open 11 (12), e052897. doi: 10.1136/bmjopen-2021-052897
Altcheh J., Sierra V., Ramirez T., Pinto Rocha J. J., Grossmann U., Huang E., et al. (2023). Efficacy and safety of nifurtimox in pediatric patients with chagas disease: results at 4-year follow-up in a prospective, historically controlled study (CHICO SECURE). Antimicrob. Agents Chemother. 67 (4), e0119322.
Barrett M. P., Kyle D. E., Sibley L. D., Radke J. B., Tarleton R. L. (2019). Protozoan persister-like cells and drug treatment failure. Nat. Rev. Microbiol. 17 (10), 607–620. doi: 10.1038/s41579-019-0238-x
Besuschio S. A., Llano Murcia M., Benatar A. F., Monnerat S., Cruz I., Picado A., et al. (2017). Analytical sensitivity and specificity of a loop-mediated isothermal amplification (LAMP) kit prototype for detection of trypanosoma cruzi DNA in human blood samples. PloS Negl. Trop. Dis. 11 (7), e0005779. doi: 10.1371/journal.pntd.0005779
Besuschio S. A., Picado A., Muñoz-Calderón A., Wehrendt D. P., Fernández M., Benatar A., et al. (2020). Trypanosoma cruzi loop-mediated isothermal amplification (Trypanosoma cruzi loopamp) kit for detection of congenital, acute and chagas disease reactivation. PloS Negl. Trop. Dis. 14 (8), e0008402. doi: 10.1371/journal.pntd.0008402
Burgos J. M., Begher S., Silva H. M., Bisio M., Duffy T., Levin M. J., et al. (2008). Molecular identification of Trypanosoma cruzi I tropism for central nervous system in Chagas reactivation due to AIDS. Am. J. Trop. Med. Hyg. 78 (2), 294–297. doi: 10.4269/ajtmh.2008.78.294
Burgos J. M., Diez M., Vigliano C., Bisio M., Risso M., Duffy T., et al. (2010). Molecular identification of Trypanosoma cruzi discrete typing units in end-stage chronic Chagas heart disease and reactivation after heart transplantation. Clin. Infect. Dis. 51 (5), 485–495. doi: 10.1086/655680
Cafferata M. L., Toscani M. A., Althabe F., Belizán J. M., Bergel E., Berrueta M., et al. (2020). Short-course Benznidazole treatment to reduce Trypanosoma cruzi parasitic load in women of reproductive age (BETTY): a non-inferiority randomized controlled trial study protocol. Reprod. Health 17 (1), 128. doi: 10.1186/s12978-020-00972-1
Canavaci A. M. C., Bustamante J. M., Padilla A. M., Perez Brandan C. M., Simpson L. J., Xu D., et al. (2010). In vitro and in vivo high-throughput assays for the testing of anti-Trypanosoma cruzi compounds. PloS Negl. Trop. Dis. 4, e740. doi: 10.1371/journal.pntd.0000740
Chagas C. (1909). Nova Tripanomíase humana. Estudos sobre morfologia e o ciclo evolutivo do Schizotrypanum cruzi, n. gen., n. sp., agente etiolójico da nova entidade morbida do homem. Mem. Inst. Oswaldo Cruz 1 (2).
De Lana M., Chiari C. A., Chiari E., Morel C. M., Gonçalves A. M., Romanha A. J. (1996). Characterization of two isolates of Trypanosoma cruzi obtained from the patient Berenice, the first human case of Chagas’ disease described by Carlos Chagas in 1909. Parasitol. Res. 82 (3), 257–260. doi: 10.1007/s004360050106
Dumoulin P. C., Burleigh B. A. (2018). Stress-induced proliferation and cell cycle plasticity of intracellular trypanosoma cruzi amastigotes. mBio 9 (4), e00673–e00618. doi: 10.1128/mBio.00673-18
Filardi L. S., Brener Z. (1987). Susceptibility and natural resistance of Trypanosoma cruzi strains to drugs used clinically in Chagas disease. Trans. R. Soc Trop. Med. Hyg. 81, 755–759. doi: 10.1016/0035-9203(87)90020-4
García-Huertas P., Mejía-Jaramillo A. M., González L., Triana−Chávez O. (2017). Transcriptome and functional genomics reveal the participation of adenine phosphoribosyltransferase in Trypanosoma cruzi resistance to benznidazole. J. Cell Biochem. 118, 1936–1945. doi: 10.1002/jcb.25978
Kahlmeter G. (2014). Defining antibiotic resistance-towards international harmonization. Upsala J. Med. Sci. 119, 78–86. doi: 10.1186/s13071-023-05775-4
Lima D. A., Gonçalves L. O., Reis-Cunha J. L., Guimarães P. A. S., Ruiz J. C., Liarte D. B., et al. (2023). Transcriptomic analysis of benznidazole-resistant and susceptible Trypanosoma cruzi populations. Parasitol. Vectors 16 (1), 167. doi: 10.1186/s13071-023-05775-4
Macedo A. M., Pena S. D. (1998). Genetic variability of trypanosoma cruzi: implications for the pathogenesis of chagas disease. Parasitol. Today 14 (3), 119–124. doi: 10.1016/s0169-4758(97)01179-4
MacLean L. M., Thomas J., Lewis M. D., Cotillo I., Gray D. W., De Rycker M. (2018). Development of Trypanosoma cruzi in vitro assays to identify compounds suitable for progression in Chagas’ disease drug discovery. PloS Negl. Trop. Dis. 12 (7), e0006612. doi: 10.1371/journal.pntd.0006612
Molina-Morant D., Fernández M. L., Bosch-Nicolau P., Sulleiro E., Bangher M., Salvador F., et al. (2020). Efficacy and safety assessment of different dosage of benznidazol for the treatment of chagas disease in chronic phase in adults (MULTIBENZ study): study protocol for a multicenter randomized phase II non-inferiority clinical trial. Trials 21 (1), 328. doi: 10.1186/s13063-020-4226-2
Moraes C. B., Giardini M. A., Kim H., Franco C. H., Araujo-Junior A. M., Schenkman S., et al. (2014). Nitroheterocyclic compounds are more efficacious than CYP51 inhibitors against Trypanosoma cruzi: Implications for Chagas disease drug discovery and development. Sci. Rep. 4, 4703. doi: 10.1038/srep04703
Moreira O. C., Ramírez J. D., Velázquez E., Melo M. F., Lima-Ferreira C., Guhl F., et al. (2013). Towards the establishment of a consensus real-time qPCR to monitor Trypanosoma cruzi parasitemia in patients with chronic Chagas disease cardiomyopathy: a substudy from the BENEFIT trial. Acta Trop. 125 (1), 23–31. doi: 10.1016/j.actatropica.2012.08.020
Morel C. M., Deane M. P., Gonçalves A. M. (1986). The complexity of Trypanosoma cruzi populations revealed by schizodeme analysis. Parasitol. Today 2 (4), 97–101. doi: 10.1016/0169-4758(86)90038-4
Morillo C. A., Marin-Neto J. A., Avezum A., Sosa-Estani S., Rassi A., Rosas F., et al. (2015). Randomized trial of benznidazole for chronic Chagas’ cardiomyopathy. N Engl. J. Med. 373, 1295–1306. doi: 10.1056/NEJMoa1507574
Morillo C. A., Waskin H., Sosa-Estani S., Del Carmen Bangher M., Cuneo C., Milesi R., et al. (2017). Benznidazole and posaconazole in eliminating parasites in asymptomatic T. Cruzi carriers: the STOP-CHAGAS trial. J. Am. Coll. Cardiol. 69 (8), 939–947. doi: 10.1016/j.jacc.2016.12.023
Muñoz-Calderón A. A., Besuschio S. A., Wong S., Fernández M., García Cáceres L. J., Giorgio P., et al. (2022). Loop-mediated isothermal amplification of Trypanosoma cruzi DNA for point-of-care follow-up of anti-parasitic treatment of chagas disease. Microorganisms 10 (5), 909. doi: 10.3390/microorganisms10050909
Muñoz-Calderón A., Ramírez J. L., Díaz-Bello Z., Alarcón de Noya B., Noya O., Schijman A. G. (2023). Genetic characterization of trypanosoma cruzi I populations from an oral chagas disease outbreak in Venezuela: natural resistance to nitroheterocyclic drugs. ACS Infect. Dis. 9 (3), 582–592. doi: 10.1021/acsinfecdis.2c00569
Muñoz-Calderón A., Silva-Gomes N. L., Apodaca S., Alarcón de Noya B., Díaz-Bello Z., Souza L. R. Q., et al. (2021). Toward the establishment of a single standard curve for quantification of trypanosoma cruzi natural populations using a synthetic satellite unit DNA sequence. J. Mol. Diagn. 23 (5), 521–531. doi: 10.1016/j.jmoldx.2021.01.007
Oliveira R. P., Broude N. E., Macedo A. M., Cantor C. R., Smith C. L., Pena S. D. (1998). Probing the genetic population structure of Trypanosoma cruzi with polymorphic microsatellites. Proc. Natl. Acad. Sci. U S A. 95 (7), 3776–3780. doi: 10.1073/pnas.95.7.3776
Parrado R., Ramírez J. C., de la Barra A., Alonso-Vega C., Juiz N., Ortiz L., et al. (2019). Usefulness of serial blood sampling and PCR replicates for treatment monitoring of patients with chronic chagas disease. Antimicrob. Agents Chemother. 63 (2), e01191–e01118. doi: 10.1128/AAC.01191-18
Ramírez J. C., Acevedo G. R., Torres C., Parrado R., de la Barra A., Villarroel S., et al. (2022). Genetic polymorphism of Trypanosoma cruzi bloodstream populations in adult chronic indeterminate Chagas disease patients from the E1224 clinical trial. J. Antimicrob. Chemother. 77 (3), 578–584. doi: 10.1093/jac/dkab446
Ramírez J. C., Cura C. I., da Cruz Moreira O., Lages-Silva E., Juiz N., Velázquez E., et al. (2015). Analytical validation of quantitative real-time PCR methods for quantification of trypanosoma cruzi DNA in blood samples from chagas disease patients. J. Mol. Diagn. 17 (5), 605–615. doi: 10.1016/j.jmoldx.2015.04.010
Ramírez J. C., Parrado R., Sulleiro E., de la Barra A., Rodríguez M., Villarroel S., et al. (2017). First external quality assurance program for bloodstream Real-Time PCR monitoring of treatment response in clinical trials of Chagas disease. PloS One 12 (11), e0188550. doi: 10.1371/journal.pone.0188550
Repolês B. M., MaChado C. R., Florentino P. T. V. (2020). DNA lesions and repair in trypanosomatids infection. Genet. Mol. Biol. 43 (1 suppl. 1), e20190163. doi: 10.1590/1678-4685-GMB-2019-0163
Revollo S., Oury B., Laurent J. P., Barnabé C., Quesney V., Carrière V., et al. (1998). Trypanosoma cruzi: Impact of clonal evolution of the parasite on its biological and medical properties. Exp. Parasitol. 89, 30–39. doi: 10.1006/expr.1998.4216
Revollo S., Oury B., Vela A., Tibayrenc M., Sereno D. (2019). In vitro benznidazole and nifurtimox susceptibility profile of trypanosoma cruzi strains belonging to discrete typing units tcI, tcII, and tcv. Pathogens 8 (4), 197. doi: 10.3390/pathogens8040197
Sanchez-Valdez F. J., Padilla A., Wang W., Orr D. (2018). Tarleton RL Spontaneous dormancy protects Trypanosoma cruzi during extended drug exposure. Elife 7, e34039. doi: 10.7554/eLife.34039
Schijman A. G., Bisio M., Orellana L., Sued M., Duffy T., Mejia Jaramillo A. M., et al. (2011). International study to evaluate PCR methods for detection of Trypanosoma cruzi DNA in blood samples from chagas disease patients. PloS Negl. Trop. Dis. 5 (1), e931. doi: 10.1371/journal.pntd.0000931
Taylor M. C., Ward A., Olmo F., Jayawardhana S., Francisco A. F., Lewis M. D., et al. (2020). Intracellular DNA replication and differentiation of Trypanosoma cruzi is asynchronous within individual host cells in vivo at all stages of infection. PloS Negl. Trop. Dis. 14 (3), e0008007. doi: 10.1371/journal.pntd.0008007
Torrico F., Gascón J., Barreira F., Blum B., Almeida I. C., Alonso-Vega C., et al. (2021). New regimens of benznidazole monotherapy and in combination with fosravuconazole for treatment of Chagas disease (BENDITA): a phase 2, double-blind, randomised trial. Lancet Infect. Dis. 21 (8), 1129–1140. doi: 10.1016/S1473-3099(20)30844-6
Torrico F., Gascon J., Ortiz L., Alonso-Vega C., Pinazo M. J., Schijman A., et al. (2018). Treatment of adult chronic indeterminate chagas disease with benznidazole and three E1224 dosing regimens: a proof-of-concept, randomised, placebo-controlled trial. Lancet Infect. Dis. 18 (4), 419–430. doi: 10.1016/S1473-3099(17)30538-8
Vago A. R., Andrade L. O., Leite A. A., d’Avila Reis D., Macedo A. M., Adad S. J., et al. (2000). Genetic characterization of Trypanosoma cruzi directly from tissues of patients with chronic Chagas disease: differential distribution of genetic types into diverse organs. Am. J. Pathol. 156 (5), 1805–1809. doi: 10.1016/s0002-9440(10)65052-3
Van den Bergh B., Fauvart M., Michiels J. (2017). Formation, physiology, ecology, evolution and clinical importance of bacterial persisters. FEMS Microbiol. Rev. 41, 219–251. doi: 10.1093/femsre/fux001
Villar J. C., Herrera V. M., Pérez Carreño J. G., Váquiro Herrera E., Castellanos Domínguez Y. Z., Vásquez S. M., et al. (2019). Nifurtimox versus benznidazole or placebo for asymptomatic Trypanosoma cruzi infection (Equivalence of Usual Interventions for Trypanosomiasis - EQUITY): study protocol for a randomised controlled trial. Trials 20 (1), 431. doi: 10.1186/s13063-019-3423-3
Ward A. I., Olmo F., Atherton R. L., Taylor M. C., Kelly J. M. (2020). Trypanosoma cruzi amastigotes that persist in the colon during chronic stage murine infections have a reduced replication rate. Open Biol. 10 (12), 200261. doi: 10.1098/rsob.200261
You H., Gordon C. A., MacGregor S. R., Cai P., McManus D. P. (2022). Potential of the CRISPR-Cas system for improved parasite diagnosis: CRISPR-Cas mediated diagnosis in parasitic infections: CRISPR-Cas mediated diagnosis in parasitic infections. Bioessays 44 (4), e2100286. doi: 10.1002/bies.202100286
Keywords: polymerase chain reaction, Chagas disease, drug resistance, benznidazole, nifurtimox, dormancy, treatment failure, Trypanosoma cruzi
Citation: Schijman AG (2023) Unveiling challenges in real-time PCR strategies for detecting treatment failure: observations from clinical trials on chronic Chagas disease. Front. Parasitol. 2:1260224. doi: 10.3389/fpara.2023.1260224
Received: 17 July 2023; Accepted: 04 September 2023;
Published: 26 September 2023.
Edited by:
Eric Chatelain, Drugs for Neglected Diseases Initiative, SwitzerlandReviewed by:
Sergio Schenkman, Federal University of São Paulo, BrazilCopyright © 2023 Schijman. This is an open-access article distributed under the terms of the Creative Commons Attribution License (CC BY). The use, distribution or reproduction in other forums is permitted, provided the original author(s) and the copyright owner(s) are credited and that the original publication in this journal is cited, in accordance with accepted academic practice. No use, distribution or reproduction is permitted which does not comply with these terms.
*Correspondence: Alejandro G. Schijman, YXNjaGlqbWFuQGluZ2ViaS1jb25pY2V0Lmdvdi5hcg==
Disclaimer: All claims expressed in this article are solely those of the authors and do not necessarily represent those of their affiliated organizations, or those of the publisher, the editors and the reviewers. Any product that may be evaluated in this article or claim that may be made by its manufacturer is not guaranteed or endorsed by the publisher.
Research integrity at Frontiers
Learn more about the work of our research integrity team to safeguard the quality of each article we publish.