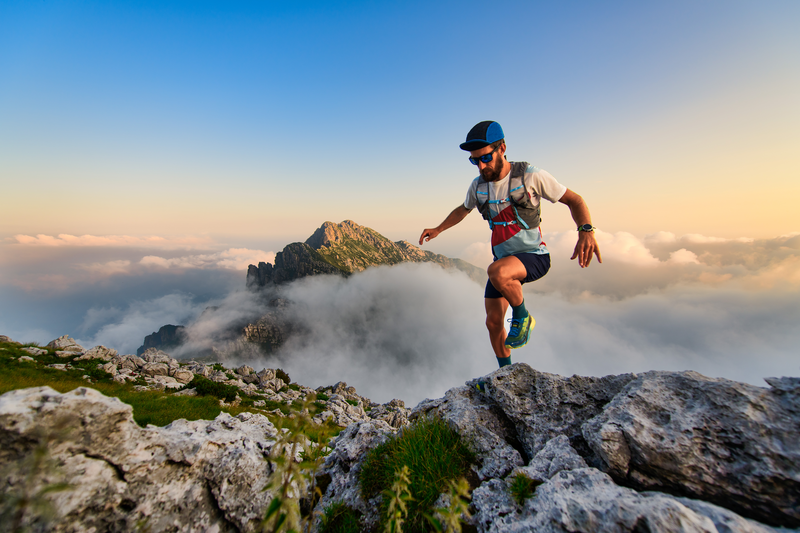
94% of researchers rate our articles as excellent or good
Learn more about the work of our research integrity team to safeguard the quality of each article we publish.
Find out more
ORIGINAL RESEARCH article
Front. Pain Res.
Sec. Veterinary and Comparative Pain
Volume 6 - 2025 | doi: 10.3389/fpain.2025.1496607
The final, formatted version of the article will be published soon.
You have multiple emails registered with Frontiers:
Please enter your email address:
If you already have an account, please login
You don't have a Frontiers account ? You can register here
Acute paraspinal hyperesthesia could result in a mixture of nociceptive and neuropathic pain that requires drug treatment but it is suggested that could also benefit from a non-pharmacologic approach such as 2-channel transcutaneous electrical nerve stimulation (TENS). From a health perspective that can be transferred from human to veterinary medicine, conventional TENS is suggested to reduce pain score and a possible reduction of pain medication. This prospective, blinded, controlled cohort study was conducted in a clinical setting. The dogs were randomized into the study group (SG) (standard pharmacological protocol (PSP) + TENS) and the control group (CG) (PSP only), with observers unaware of the treatment administered. The aim was to observe the efficacy of TENS in relieving thoracolumbar paraspinal hyperesthesia in dogs. 818 dogs with paraspinal hyperesthesia, classified as grade 4 or 5 in the modified Frankel Scale (MFS) and with a Dynamic Interactive Visual Analog Scale (DIVAS) score ≥14, were enrolled in the study. Two blinded observers were responsible for scoring the video recording results for all checklist parameters. All results were assessed every 24 hours from T0 (admission) to T8, always five minutes before TENS for SG. 74% of dogs (605/818) with PSP with TENS and 26% (213/818) with PSP only. In the first 48 hours, SG showed a faster improvement in the reduction of muscle tone. All were hyperesthetic and became non-painful, but in SG there was a faster recovery from T2 (48h) to T4. There was a statistical difference between groups in terms of DIVAS score (p<0.001) and in terms of treatment duration, SG took on average 2.14 days to discharge, whereas CG took twice as long (p<0.001). TENS may help to improve paraspinal hyperesthesia and promote early recovery by reducing pain, the need for medication and also recovery time. However, these results should be interpreted cautiously as there are no objective measures in a clinical trial, which may lead to potential bias. This modality could be considered in clinical practice as an adjunctive treatment for acute thoracolumbar hyperesthesia in dogs, although further studies are needed.
Keywords: TENS, neuropathic pain, IVDD, Paraspinal hyperesthesia, Divas
Received: 14 Sep 2024; Accepted: 03 Mar 2025.
Copyright: © 2025 Gouveia, Cardoso, Carvalho, Moisés, Monteiro Coelho, Balça, Alvites, Maurício, Ferreira and Martins. This is an open-access article distributed under the terms of the Creative Commons Attribution License (CC BY). The use, distribution or reproduction in other forums is permitted, provided the original author(s) or licensor are credited and that the original publication in this journal is cited, in accordance with accepted academic practice. No use, distribution or reproduction is permitted which does not comply with these terms.
* Correspondence:
Ana Colette Maurício, Institute of Biomedical Sciences Abel Salazar, University of Porto, Porto, 4050-343, Portugal
Disclaimer: All claims expressed in this article are solely those of the authors and do not necessarily represent those of their affiliated organizations, or those of the publisher, the editors and the reviewers. Any product that may be evaluated in this article or claim that may be made by its manufacturer is not guaranteed or endorsed by the publisher.
Research integrity at Frontiers
Learn more about the work of our research integrity team to safeguard the quality of each article we publish.