- 1Department of Clinical Studies, University of Pennsylvania School of Veterinary Medicine, Kennett Square, PA, United States
- 2Department of Population Medicine and Diagnostic Sciences, Cornell University College of Veterinary Medicine, Ithaca, NY, United States
- 3Department of Anatomy and Physiology, Kansas State University, Manhattan, KS, United States
Globally, humans rely on cattle for food production; however, there is rising societal concern surrounding the welfare of farm animals. From a young age, cattle raised for dairy and beef production experience pain caused by routine management procedures and common disease conditions. The fundamental mechanisms, nociceptive pathways, and central nervous system structures required for pain perception are highly conserved among mammalian species. However, there are limitations to a comparative approach to pain assessment due to interspecies differences in the expression of pain. The stoicism of prey species may impede pain identification and lead to the assumption that cattle lack pain sensitivity. This highlights the importance of establishing validated bovine-specific indicators of pain—a prerequisite for evidence-based pain assessment and mitigation. Our first objective is to provide an overview of pain pathophysiology to illustrate the importance of targeted analgesia in livestock medicine and the negative welfare outcomes associated with unmitigated pain. This is followed by a review of available analgesics, the regulations governing their use, and barriers to implementation of on-farm pain management. We then investigate the current research undertaken to evaluate the pain response in cattle—a critical aspect of the drug approval process. With an emphasis on emerging research in animal cognition and pain pathology, we conclude by discussing the significant influence that pain has on cattle welfare and areas where further research and modified practices are indicated.
1 Introduction
Cows, like humans, live in a sensory environment. Sensory modalities include temperature, pressure, chemicals, light, sound, and movement. Pain, however, is not a sensory modality; it is an affective state that represents a “subjective cerebral response” (1). What we observe in animals—withdrawal, vocalization, inappetence—is the product of nociception, or the neural processing of a noxious stimulus that is transduced from a chemical signal into an action potential (2). Without spoken language to convey the emotional experience occurring within the higher centers of the brain, any reaction to a noxious stimulus must be interpreted as pain (1). Consequently, much of what we understand about the physiology of pain arises from the experience of human subjects. An anthropomorphic approach to pain management in veterinary medicine, however, is misleading due to interspecies differences in the expression of pain. It is therefore imperative to develop a targeted approach to pain detection that acknowledges the unique physiology, behavioral patterns, and evolutionary history of cattle.
Molony and Kent (1997) define pain as “an aversive sensory and emotional experience representing an awareness by the animal of damage or threat to the integrity of its tissues. It changes the animal's physiology and behaviour to reduce or avoid the damage […] and to promote recovery” (3). Although cows cannot communicate the experience of pain through spoken language, these alterations in physiology and behavior facilitate pain detection (4)—a prerequisite for evidence-based pain assessment and mitigation. Causes of pain in cattle raised for dairy and beef include routine management procedures, such as disbudding (5), castration (6), and branding (7), as well as common disease conditions. Studies demonstrate that pain is associated with lameness (8), mastitis (9), gastrointestinal (10) and respiratory disease (11), and conditions surrounding calving, such as dystocia (12) and metritis (13). Although painful conditions are common in cattle, there is a scarcity of validated bovine-specific indicators of pain. This impedes approval of drugs labelled for pain control by the responsible agencies, such as the Food and Drug Administration (FDA) in the United States and contributes to inconsistent and inadequate use of analgesia in cattle.
Our first objective is to provide an overview of pain pathophysiology to illustrate the importance of targeted analgesia in livestock medicine and the negative welfare outcomes associated with unmitigated pain. This is followed by a review of available analgesics, regulations specific to the U.S. that govern their use, and reasons for unsatisfactory implementation of on-farm pain management. We then investigate the current research undertaken to evaluate the pain response in cattle—a critical aspect of the drug approval process. With an emphasis on emerging research in animal cognition and pain pathology, we conclude by discussing the significant influence that pain has on cattle welfare and areas where further research and modified practices are indicated. Although conditions causing pain in cattle are common and widespread, there are minimal established recommendations to guide on-farm pain management protocols, especially for painful disease conditions. Furthermore, the administration of analgesics to safeguard cattle welfare remains voluntary in the U.S., as there are no state or federal regulations enforcing their use. To secure the welfare of cattle under our care, we must prioritize on-farm pain prevention and mitigation through modified management practices and preemptive and multimodal approaches to analgesic intervention.
2 Overview of the physiology and pathophysiology of pain in cattle
2.1 Nociception and pain perception
Nociception—from the Latin “nocere,” or “to harm”—is not exclusive to humans or mammals in general (14, 15). Nociceptors are found in non-mammalian vertebrates including birds, reptiles, amphibians, and fish, as well as in invertebrates, such as nematodes, leeches, and fruit flies (16). Nociceptors are high-threshold sensory receptors that preferentially respond to mechanical, thermal, or chemical noxious stimuli that may result in tissue damage (17). These sensory receptors have free nerve endings and can be found in the skin, muscles, joints, viscera, and vasculature (18). There are two types of nociceptor axons: Aδ and C fibers. Aδ fibers are myelinated and rapidly conduct localizable pain often described as “sharp,” signaling potential tissue damage (17, 19). C fibers are non-myelinated and thus conduct signals more slowly. They are responsible for the perception of non-localizable pain that has been described by humans as dull, throbbing, aching (17), or burning (20). This type of pain can lead to depression and withdrawal, behavioral responses that may encourage healing (17). In healthy, undisturbed tissues, these neurons are quiescent; noxious stimuli must exceed the stimulus receptor threshold for activation to occur (18). Nociception thus serves as an evolutionarily valuable alarm system, alerting an organism to potential tissue damage.
It is important to acknowledge the complexity surrounding nociception and pain. Nociception will not always result in pain, and pain will not always arise from nociception. To connect nociception with the experience of pain, four processes must be considered: transduction, transmission, modulation, and perception. Recognizing these disparate and interconnected processes is critical to understanding pain and targeted analgesia (Figure 1). Transduction occurs when signaling molecules, such as cytokines, bradykinin, and prostaglandins released in response to a noxious stimulus, are converted into an action potential capable of travelling to the central nervous system (18). Transmission follows when this action potential generated during transduction travels to the spinal cord via the dorsal nerve roots. It then synapses within the dorsal horn or may be propagated up the spinal cord via the ascending pathway (17). Pain modulation is a process whereby pain transmission is suppressed or heightened via inhibitory and excitatory mechanisms in both the peripheral and central nervous systems (18).
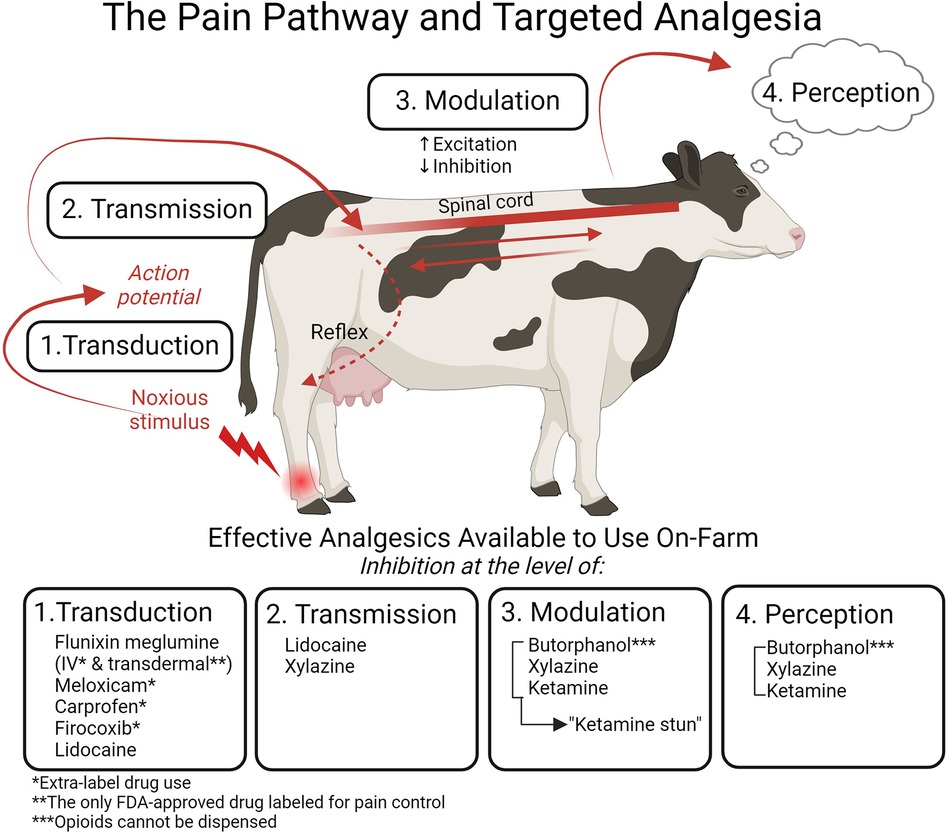
Figure 1. The four processes of nociception targeted by analgesia and the specific drugs available for on-farm use in cattle. Transduction (1) occurs when a noxious stimulus is converted into an action potential. This action potential then travels from the periphery to the central nervous system in the process of transmission (2). Modulation (3) results in enhancement or suppression of the nociceptive signal. Pain is experienced when the signal is perceived (4) in the higher centers of the brain. The nociceptive signal can also result in an immediate reflex response. Analgesics can target each of these processes: the NSAIDs flunixin meglumine, meloxicam, carprofen, and firocoxib inhibit transduction; the local anesthetic lidocaine inhibits transduction and transmission; the α2 agonist xylazine inhibits transmission and, when combined with the NMDA receptor antagonist ketamine and the opioid butorphanol, affects modulation and perception to inhibit pain. Flunixin meglumine transdermal is the only FDA-approved drug for pain control in cattle, and it is labelled only for foot rot; all other NSAIDs must be used in an extra-label manner. Opioids cannot be dispensed on-farm. Figure adapted from (21). Created with BioRender.com.
To experience pain, nociceptive information must lastly be transmitted to higher centers of the brain where the signal is processed, integrated, and recognized (20). In the brainstem, the reticular activating system (RAS) integrates signals received and projects to the thalamus and limbic system, resulting in both behavioral and autonomic pain responses (20). The thalamus projects information to the cerebrum's somatosensory cortex, which communicates with other regions of the brain including the limbic system (20). The cerebral cortex is fundamental to the experience of pain, as it is where conscious perception occurs (20). The electroencephalogram (EEG) detects cortical electrical activity, and electroencephalographic spectral analysis has been used to study pain perception in humans (22, 23) with similar EEG signal variations reported in cattle undergoing painful procedures, such as dehorning (24) and castration (25–28). EEG signal changes in response to pain include “desynchronization,” a phenomenon characterized by an increase in high-frequency activity and a reduction in low-frequency activity (29). These EEG findings signify nociceptive signal processing within the higher centers of the brain, providing evidence of pain perception in cattle.
Pain perception may be modified via cortical communication with subcortical regions, such as the thalamic and limbic systems (30). The amygdala, for example, is involved in mediating the emotional-affective aspects of pain (31). Mammalian perceived pain therefore encompasses not only sensory-discriminatory and evaluative components, but also affective state (20). Attentional and emotional states can modify pain processing, enhancing or diminishing pain perception (32). Studies in humans demonstrate diminished pain perception with distraction, positive expectation, and “positive mood manipulations,” such as playing music for a postoperative patient (32). The influence of all three of these positive contextual elements was demonstrated in a study conducted by Lomb et al. (33) in which researchers used positive reinforcement training and counterconditioning to reduce heifer reactivity to the administration of a subcutaneous injection. Heifers entered a headlock that was either closed (“habituation” heifers) or remained open (“agency” heifers) and received grain upon entrance. This food reward was then paired with gradual exposure to a sham injection. “Naïve” heifers received no treatment area exposure. “Agency” heifers demonstrated reduced approach latency compared to “habituation” and “naïve” heifers and reduced injection reactivity compared to “habituation heifers.” The researchers posited that distraction, positive expectation, and agency attenuated the fear and pain response in study subjects (33). Ede et al. (34) determined that calves receiving an intramuscular injection paired with a milk reward (1 L, 500 mL, 250 mL, and 0 mL) did not demonstrate approach latency when compared to controls until the milk reward was reduced to 250 mL, similarly suggesting that cattle experience pain attenuation in the presence of a positive stimulus.
The principal categories of pain include acute nociceptive, inflammatory, and neuropathic pain (35). Although all categories involve nociceptor activation, they have different pain-generating etiologies (36). Nociceptive pain describes the pain resulting from contact with a noxious stimulus (35); it is typically acute and dissipates upon removal of the inciting cause (36). Inflammatory pain is associated with healing and results from the release of inflammatory mediators following tissue damage that can lower the nociceptor stimulus threshold, enhancing its activation (1). It typically leads to swelling, redness, heat, and loss of function of the affected structure. Lastly, neuropathic pain occurs due to structural damage to neural components responsible for communicating nociceptive information to the central nervous system (CNS) (35). These three categories of pain are not mutually exclusive. As an example, lameness in cattle may derive from acute hoof trauma, resulting in nociceptive pain. Removal of the injurious stimulus may result in pain attenuation and healing; however, a breach in the tissues of the hoof can lead to infection, resulting in inflammatory pain. If the infection is untreated and the pain is unabated, nociceptive sensitivity may be altered, resulting in chronic, neuropathic pain.
Understanding pain etiology and the pain pathway is critical to implementing a targeted and comprehensive analgesic approach. By targeting different levels of the pathway with pharmaceutical agents, we can prevent pain and maximize analgesic efficacy (Figure 1). This preemptive and multimodal analgesic approach can protect an animal from the aversive emotional experience associated with both acute and protracted pain, as well as prevent the development of maladaptive pain—a pathological pain state that significantly impairs animal welfare.
2.2 Central sensitization and maladaptive pain
Adaptive pain, also referred to as acute pain, is protective; it serves to prevent sustained tissue damage by causing physiologic, neuroendocrine, and behavioral changes that promote healing (37). Conversely, maladaptive pain describes a pathological pain state in which pain persists after removal of the inciting cause and results from tissue or nerve damage (37). This pain is considered maladaptive, or “dysfunctional,” because it neither provides protection nor fosters healing (38).
Following tissue injury, neurotransmitters and inflammatory mediators, such as bradykinin and prostaglandins, surround nociceptor terminals, lowering the nociceptor's activating threshold in a process known as peripheral sensitization (1, 18). Growth factors and inflammatory cytokines and chemokines, such as tumor necrosis factor (TNF) and interleukin-1β (IL-1β), may also alter nociceptor properties via enhanced nerve terminal sensitization and nociceptor gene regulation (39). This can lead to hyperalgesia or allodynia, heightened pain sensitivity states in which mildly noxious or innocuous stimulation, respectively, results in nociceptor activation (18) (Figure 2).
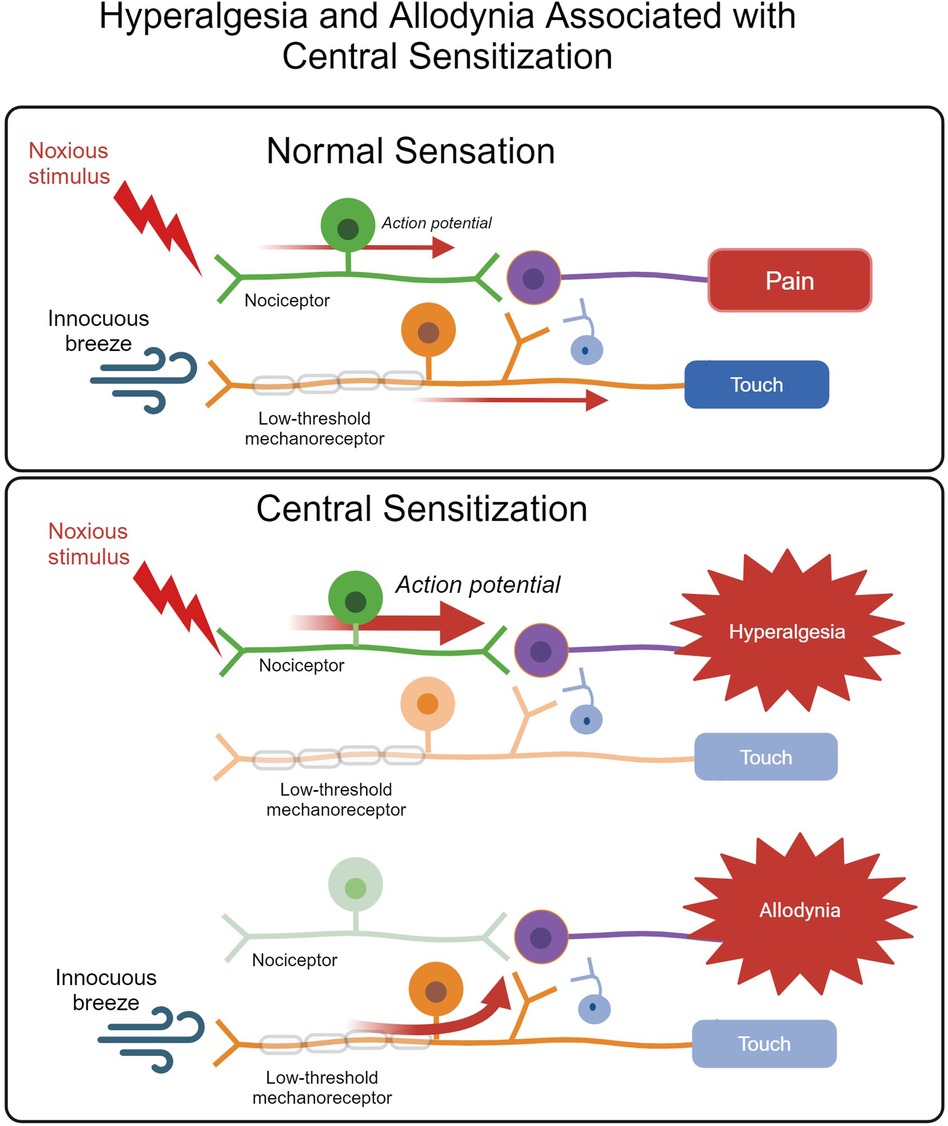
Figure 2. The normal somatosensory pathway for pain and touch versus heightened and abnormal activation of the pain pathway associated with central sensitization resulting in hyperalgesia and allodynia. In an animal experiencing normal sensation, noxious stimuli activate nociceptors, leading to pain. Innocuous stimuli are detected by low-threshold mechanoreceptors, which do not communicate with the pain pathway and result in touch sensation. The pathways do not communicate. Hyperalgesia describes a heightened response to nociceptive input, whereas allodynia occurs when an innocuous stimulus results in pain due to low-threshold mechanoreceptor activation of pain pathways. Figure adapted from (40). Created with BioRender.com.
In the non-diseased state, peripheral sensitization prevents an animal from further aggravating an injured area and will resolve with analgesia and as injured tissues heal (18). Studies demonstrate that cattle alter activity patterns in response to pain; decreased lying time is observed in cases of clinical mastitis due to a painful udder, whereas lameness is often associated with an increase in lying time to avoid applying pressure to a painful hoof (41). Thus, heightened tissue sensitivity leads to behavioral changes that promote healing by minimizing the risk of additional tissue damage. However, prolonged peripheral sensitization due to unrelieved pain can lead to long-term potentiation of neuronal synapses and disinhibition in the process known as central sensitization (42). Central sensitization may enhance neuronal excitability and diminish endogenous pain modulatory systems, leading to exaggerated nociceptor transmission and pain amplification (18). These alterations in the functional properties of neurons persist in the absence of the initiating insult (42), resulting in maladaptive pain. Studies in cattle suffering from chronic lameness demonstrate the development of central sensitization (43–46), leading to pain that is more refractory to treatment. Aversive contextual experiences, such as negative preslaughter conditions, may also predispose cattle to sensitization and heightened pain responses (30).
2.3 Neonatal neuroplasticity
From a young age, calves raised for dairy and beef production are subjected to painful procedures, and neonates may be particularly susceptible to the development of central sensitization and maladaptive pain. Research in human and rodent models suggests that the immature nervous system of neonatal animals is sensitive to somatosensory and nociceptive alterations in response to painful experiences (47). Rodent models demonstrate that neonatal tissue injury alters pain sensitivity; rats that experience injury early in development exhibit generalized hyposensitivity and localized hyperalgesia at the injury site in adulthood, as reviewed by Schwaller and Fitzgerald (48). This increased pain sensitivity at the injury site is likely related to alterations in neurocircuitry at the level of the dorsal horn of the spinal cord (48). Hyperinnervation resulting from the altered distribution of nociceptive fibers (49), microglial cell activation related to the developing immune system (50), and disruption to the descending modulatory system responsible for pain inhibition (47) have also been implicated in sensory disturbances arising from neonatal pain.
Evidence of altered tissue sensitivity in the aftermath of early life painful procedures has been observed in cattle. Calves that undergo both chemical and cautery disbudding may experience chronic sensitization that persists months beyond the post-procedural period (51–53). Although this pain is associated with protracted re-epithelialization, it may lead to central sensitization due to failure to provide analgesia at the onset of tissue trauma and throughout wound healing. Calves disbudded as neonates demonstrate enhanced tissue sensitivity in regions distal to the initial site of trauma (51) and an enhanced cardiac response to vaccine administration (54). Hypersensitivity associated with neuroma formation has been documented in heifers after tail docking (55), as well as other farm animals, including piglets (56) and lambs (57). Although neonatal pain and neuroplasticity in farm animals is understudied, human and rodent models suggest that these nociceptive alterations may persist into adulthood (47). Studies in rats further demonstrate that neonatal inflammation negatively impacts future female reproductive development (58), maternal care, and offspring stress resiliency (59), demonstrating intergenerational repercussions of early life pain. A study conducted by Clark et al. (60) provides evidence that ewes that undergo tail-docking early in life exhibit greater pain responses during parturition as adults, and ewes experimentally induced with an infection during the neonatal period give birth to offspring with reduced pain sensitivity.
3 Overview of livestock anesthesia and analgesia
Although pain control in farm animals is a considerable public concern, there is minimal legislation governing the use of analgesics. Surveys conducted in the U.S. regarding farm animal pain indicate that the general public supports higher welfare standards (61). The Ohio Survey of Food, Agricultural and Environmental Issues (62) reported that 75% of respondents agreed with the statement that “farm animals should be protected from feeling physical pain” and a more recent national online survey conducted by the Animal Welfare Institute (63) revealed that greater than 80% of consumers agreed that painful procedures should be performed with analgesia. This public position, however, has not translated into regulatory expansion regarding the use of analgesics in farm animals. Regulations currently exist for appropriate drug use, such as approved routes of administration and withdrawal intervals for food-producing animals; however, no regulations specifically enforce the use of analgesics to safeguard animal welfare. The American Veterinary Medical Association (AVMA) and the American Association of Bovine Practitioners (AABP) provide recommendations for the use of analgesia for some painful procedures, such as dehorning and castration (64–66). The AVMA acknowledges that these procedures “cause pain and discomfort” and advocates for “procedures and practices that reduce or eliminate these effects” (66). However, a 2021 survey study of U.S. veterinarians (n = 497), producer-veterinarians (n = 569), and producers (n = 121) conducted by Johnstone et al. (67) reported that, in calves less than 2 months old, 43.8% of respondents “never use” local anesthesia for surgical castration and 32.8% “never use” local anesthesia for dehorning. Industry efforts to encourage the use of anesthetics and analgesics are similarly limited. The Farmers Assuring Responsible Management (FARM) Program Version 4.0 standards require the use of pain relieving drugs for disbudding; however, the use of anesthetics and analgesics is not required for castration and branding, although these procedures are characterized as painful in the Animal Care Reference Manual (68). Furthermore, there is a deficiency of recommendations guiding the management of painful disease conditions.
Veterinary drugs are approved by national agencies, and their availability and associated withdrawal times vary widely between countries. In the U.S., the Food and Drug Administration (FDA) Center for Veterinary Medicine (CVM) approves animal drugs. The FDA CVM is an organization designed to secure human and animal health (69). Under the authority of the Federal Food, Drug, and Cosmetic Act (FFDCA), the FDA CVM publishes the Code of Federal Regulations (CFR), defining the regulations governing the introduction of veterinary drugs (69). Drug approval for commercial marketing requires (1) safety for the target species, human consumer, animal handler, and environment; (2) pharmaceutical efficacy for the stated purpose; (3) quality manufacturing; and (4) appropriate labeling, including safety, storage, handling, and withhold information (69). There is a scarcity of drugs labelled for pain control in cattle. Consequently, veterinarians must use medications to control pain in an extra-label manner under the Animal Medicinal Drug Use Clarification Act (AMDUCA) (70). To prescribe and administer extra-label drugs, a veterinarian must comply with AMDUCA regulations, including the establishment of a veterinary-client-patient relationship (VCPR) and strict adherence to withdrawal intervals to avoid violative residues (21). Kleinhenz et al. (71) describe this as a significant “regulatory burden” placed on veterinarians.
In a hospital setting, a multimodal approach to analgesia can be utilized to target transduction, transmission, modulation, and perception to prevent and treat pain in cattle within the confines of extra-label drug use. However, in field settings many of these available drugs are cost-prohibitive or cannot be safely dispensed due to legislation concerning controlled substances, such as the opioids. These limitations underscore the need for more FDA-approved analgesic options to facilitate safe, affordable, and effective methods of pain prevention and mitigation. Currently, the most common drugs used in livestock medicine include local anesthetics and nonsteroidal anti-inflammatory (NSAIDs) agents, although the use of these drugs remains inconsistent even for painful procedures with recommended guidelines (72). Refer to Table 1 for a detailed overview of available anesthetics and analgesics used in cattle medicine.
3.1 Barriers to livestock anesthetic and analgesic use
There are numerous barriers to the use of analgesics in cattle. The scarcity of drugs labelled for pain control and consequent extra-label drug use may discourage producers from implementing pain management protocols due to the requirement for veterinary oversight under AMDUCA (104). Many of the drugs used in an extra-label manner must be administered intravenously and may require repeat doses, further inconveniencing producers, as animal restraint and a degree of skill are needed for this route of administration (21). The animal restraint involved in administration increases labor and may pose a risk to worker safety. The intervening time between analgesic administration and pharmaceutical activity may further discourage pain mitigation efforts by producers, who often have time constraints and many animals to process (21). These inconveniences are compounded by economic considerations, such as high cost and associated milk and meat withdrawal intervals (105). There is also a lack of studies investigating the economic benefits of pain management. Pain is associated with stress, depression, inappetence, and impaired wound healing and immune function (75)—all of which may negatively impact productivity. Studies that demonstrate an association between pain prevention and mitigation and increased animal productivity and longevity may incentivize producers to administer analgesics.
Pain detection and mitigation require shifts in attitude and effective veterinarian-producer communication. In a recent nationwide U.S. survey study of producers and veterinarians, Edwards-Callaway et al. (106) reported a significant association between respondent pain perception and analgesic administration; this is in accordance with previous studies conducted in the UK (107, 108). However, Remnant et al. (107) found that painful management procedures in young calves did not align with this trend. Although respondents recognized the pain associated with procedures such as disbudding and castration, they were less likely to provide postprocedural pain relief. The researchers propose several explanations for producer analgesic hesitancy: firstly, terminology such as “anti-steroidal” and “anti-inflammatory,” in contrast to simpler terms such as “pain killer,” may confuse a producer's understanding of the drug's purpose; secondly, the movement for antibiotic stewardship may encourage producers to avoid all injectable drugs, as they may conflate antimicrobial agents with anti-inflammatory agents. The misconception that neonatal animals do not experience pain may also contribute to inconsistency in analgesic use for these procedures (106). Veterinarians demonstrate a comparatively higher sensitivity to cattle pain than producers (106). Sumner et al. (109) assert that veterinarians, due to their training in pain management and working relationship with clients, are uniquely positioned to challenge the status quo and direct producers towards pain prevention and mitigation protocols.
Inconsistency in the use of pain relief for cattle can lastly be attributed to the difficulty of reliably assessing pain, as pain identification is fundamental to mitigation and required for drug approval as outlined by the CFR (69). A recent systematic review and meta-analysis conducted by Tschoner et al. (110) compared veterinarian and producer pain ratings for a variety of management procedures and disease conditions using the Numerical Rating Scale (NRS) and Visual Analogue Scale (VAS). The researchers concluded that inter-study comparisons were confounded by variations among pain scales, inconsistent terminology, and scorer variables, such as gender, age, and education, that contribute to the subjectivity of pain assessment (110). This underscores the need for sufficiently objective, reliable, and validated pain scales to guide on-farm pain detection.
4 Pain evaluation in cattle
Identifying reliable pain indicators enables us to determine pain intensity and analgesic efficacy, which are required for appropriate therapeutic intervention. Validation of pain indicators, however, is complicated by interspecies differences in the expression of pain. The available research on pain is primarily derived from human subjects with a reliance on self-report (111). Not only are non-human animals incapable of self-report, but findings from one species cannot be reliably extrapolated to another species due to differences in evolutionary history, selective pressures, and learned behaviors, which impact the observable expression of pain (112).
To survive predation, cattle evolved to mask pain. Disguising weakness and focusing on escape are important survival strategies with adaptive benefits (105). In contrast to other social animals, such as primates, dogs, and pigs, that vocalize to signal distress, vocalization may be maladaptive for a cow by signaling vulnerability to attack (112). Although the modern dairy farm does not mirror the predator-prey dynamic of the wild, there remain situations in commercial settings in which a cow benefits from disguising pain. For example, a dominant herd member may impose limits on feed access upon recognition of weakness in another cow (113). A dairy cow's consequent stoicism can obscure clinical pain identification and lead to the perception that cattle lack pain sensitivity (105). This highlights the importance of developing species-specific pain indicators; however, their validation is further complicated by intraspecies differences in pain expression.
The perception of pain—a subjective emotional experience—is revised by memories of prior experiences with noxious stimuli (114). For example, when pain is associated with a more pleasing environment, the pain intensity may be reduced; conversely, association with a negative environment may increase the pain response (18) (Section 2.1). An animal's degree of vigilance and affective state may impact the experience of pain (1) (Section 2.1), as can their developmental stage and current environment (105). Stress and reproductive factors, such as the estrous cycle, may also confound pain evaluation by altering behavioral and physiological parameters (105). Studies in mammals suggest that genetic differences may contribute to intraspecies variability in the pain response, as evidenced by the identification of more than 300 possible “pain genes” (115). Differences in the type and location of pain further account for variations in observable behavioral responses to pain among individual animals of the same species. Acute pain may present differently than chronic pain; superficial pain may present differently than visceral pain (105). An animal's breed and sex further account for intraspecies differences in pain detection. A study conducted by Martin et al. reported differences in behavioral and physiological indicators of pain between male and female Angus and Hereford calves in response to hot-iron branding (116).
Large scale farming additionally challenges pain detection; herd size within the U.S. dairy sector has increased dramatically with the average number of cows per herd reaching 1,300 in 2017 (117). Without a concomitant increase in worker number or implementation of automated monitoring systems, individual animal observation is compromised. Precision Livestock Farming (PLF) emerged in 2003 (118) and employs technology and machine learning to continuously monitor animals at both the individual and population levels in real-time, enabling the collection of large amounts of data while minimizing associated labor (119). PLF technologies may aid in the advancement of animal welfare, including pain detection and mitigation, by streamlining the detection of parameters associated with positive or negative welfare states. There are concerns that automated systems may encourage further agricultural intensification and negatively impact the human-animal bond (120); however, PLF technologies have the potential to enhance animal welfare if thoughtfully integrated into farm management systems and research initiatives (121). Although PLF technologies have not yet been widely adopted for pain detection, they have the potential to facilitate pain surveillance on large-scale operations. Furthermore, welfare and production parameters associated with pain can be studied in parallel to incentivize higher welfare practices.
Even when aided by automated systems, effective pain detection requires validated pain response measures that are reproducible between studies with maximized sensitivity and specificity. According to Weary et al. (4), the gold standard for validation requires studies that evaluate pain responses in the presence and absence of a painful condition and in the presence and absence of an analgesic drug known to effectively mitigate the pain caused by this condition. Despite the associated challenges, pain assessment can be guided by three categories of pain response: production, physiological, and behavioral indicators (4).
4.1 Evaluating pain in cattle: production indicators
Cattle production indicators include feed intake, average daily gain, milk yield, and fertility measures. Studies investigating performance outcomes are lacking in the available literature, as reviewed by Newton and O'Connor (122). Several studies have examined the influence of disbudding and castration on average daily gain and feed intake with mixed results (123–126), which Newton and O'Connor attribute, in part, to insufficient study periods and small sample sizes. Sufficiently robust studies are similarly lacking for the impact of disease-associated pain on production parameters. Lameness results in herd economic losses due to reduced milk yield (127–129) and impaired reproductive performance (130). Lameness also alters behaviors critical to production performance such as feed intake (131). Clinical metritis has been reported to result in reduced milk yield (132), impaired reproductive performance (133), and premature culling (134). However, these production parameters have not been examined in relation to the specific contribution of pain associated with the disease or management procedure.
Production performance indicators are not as useful for identifying immediate pain to guide treatment, though they may indicate chronic pain. Reduction in average daily gain or body condition score due to decreased feed intake, for example, may suggest protracted pain (4). However, changes in appetite may take several hours to detect, and days are required to detect loss of body condition (4). Thus, this measurement is not necessarily reflective of an animal's current experience. In addition to changes in feeding behavior, pain may increase stress levels and activate the immune system, altering nutrient utilization and negatively impacting body condition (135). Although production performance parameters do not capture the inner experience of the animal and are less important from the animal's point of view, they remain an important consideration, as economic incentive often drives pain mitigation efforts. Further research investigating the impact of pain on production performance is needed, as improved production may offset the cost of analgesics, incentivizing their use.
4.2 Evaluating pain in cattle: physiological indicators
Physiological measures of pain include cardiovascular, respiratory, endocrine, neurophysiological, electrophysiological, and immune responses (136). Nociception may result in physiological stress responses. Nociceptive signals are detected by the hypothalamic-pituitary-adrenal (HPA) axis and the sympathetic division of the autonomic nervous system (ANS) (135). The hypothalamus processes nociceptive signals and releases corticotropin-releasing hormone (CRH) to the anterior pituitary, which in turn releases adrenocorticotropic hormone (ACTH) to the adrenal gland. Once stimulated, the adrenal gland releases cortisol into the bloodstream (137) (Figure 3). Elevated cortisol levels have been detected in calves in response to disbudding (139), castration (140), and branding (141). Cortisol elevations have also been detected in cattle with mastitis (9, 142, 143) and lameness (144, 145). Acute nociception also stimulates sympathetic nerve fibers innervating the adrenal gland, resulting in catecholamine release from the adrenal medulla (146). This release of catecholamines, such as epinephrine and norepinephrine, results in vasoconstriction that directs blood centrally for the “fight or flight” response, increasing cardiac output and respiratory rate (140) (Figure 3). Flight behavior is commonly exhibited by cattle during painful procedures, such as hot-iron branding (147) and elevations in norepinephrine have been reported in lame cattle (148). Many studies examine heart rate, heart rate variability, and respiratory rate as indicators of pain in calves undergoing painful procedures (149) and cattle with lameness (150), mastitis (142), and metritis (13). The autonomic nervous system response to pain can also be captured with infrared thermography (IRT), which detects alterations in temperature distribution related to blood flow, demonstrating sympathetic nervous system activation. Using IRT, ocular temperature changes have been reported in calves undergoing disbudding (151, 152) and castration (27, 140, 149).
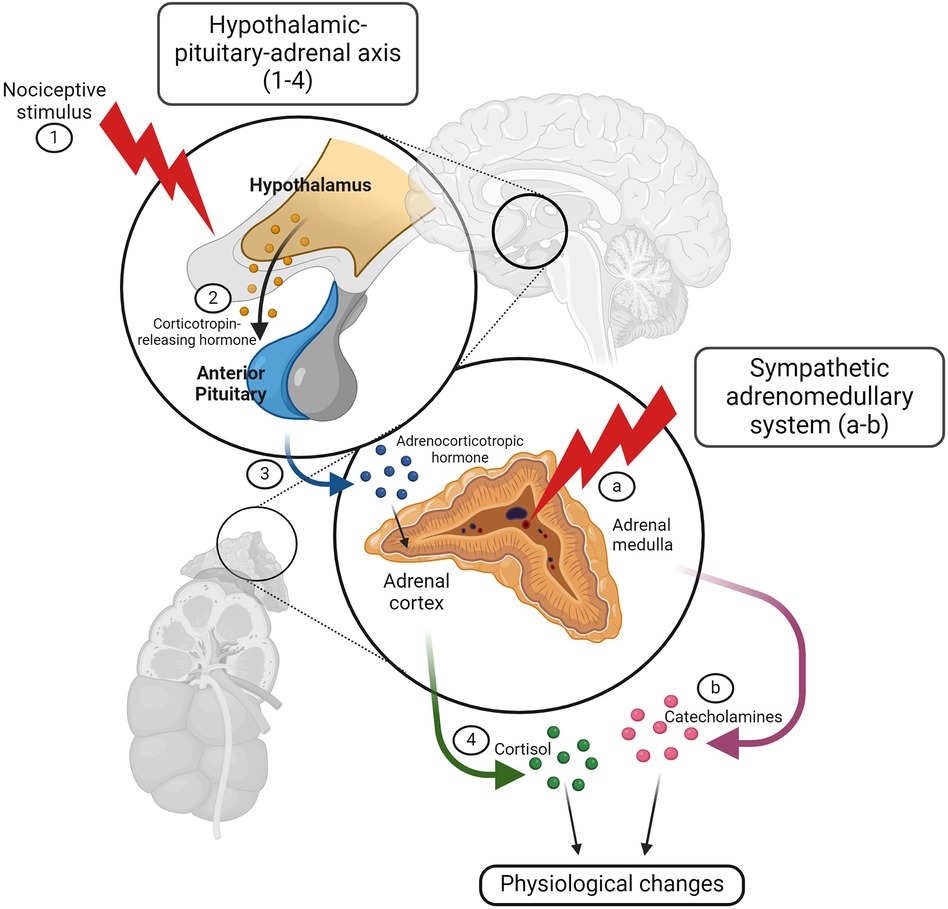
Figure 3. The hypothalamic-pituitary-adrenal axis and autonomic nervous system respond to noxious stimuli resulting in physiologic changes. A noxious stimulus activates the hypothalamic-pituitary-adrenal (HPA) axis and the sympathetic division of the autonomic nervous system (ANS). After processing the nociceptive signal (1), the hypothalamus releases corticotropin-releasing hormone (CRH) to the anterior pituitary (2), which then releases adrenocorticotropic hormone (ACTH) to the adrenal gland (3). Once stimulated, the adrenal cortex releases cortisol into the bloodstream (4), resulting in physiologic changes associated with the stress response. Nociception also stimulates sympathetic nerve fibers innervating the adrenal gland (A) causing catecholamine release from the adrenal medulla (B) Catecholamines, such as epinephrine and norepinephrine, are responsible for the “fight or flight” response. Figure adapted from (138). Created with BioRender.com.
The immune response to tissue damage also provides opportunities for physiological measurements indicative of pain. Tissue damage leads to the release of inflammatory mediators involved in nociception, such as interleukin-1 (IL-1), a proinflammatory cytokine that stimulates the adrenal gland to release ACTH (153). Other inflammatory products, such as fibrinogen and haptoglobin, indirectly suggest pain presence, as the inflammatory process often evokes a state of pain (135). Elevated concentrations of haptoglobin have been reported in cattle with mastitis (9, 142, 143), metritis (154, 155), and lameness (150, 156, 157).
The neurotransmitter substance P has been studied as a pain biomarker in adult cattle and calves as reviewed extensively by Tschoner and Feist (158). Studies have investigated substance P concentrations in cattle suffering from lameness (144, 145, 156), metritis (154), and dystocia (159). Substance P has also been used to evaluate pain in calves that undergo castration (160) and dehorning (161).
Although physiological and neuroendocrine parameters can be easily quantified, their measurements frequently require specialized equipment and advanced skill sets (105). Studies are often more invasive than those analyzing behavioral indicators alone; measurements may require sample collection or fitting the animal with monitoring equipment (135). Physiological changes can also be caused by factors other than pain, such as stress and physical activity (105), minimizing testing specificity. This is exacerbated by collection techniques that may require animal restraint, thus increasing the likelihood that stress may confound data due to sympathetic nervous system activation (162). Lastly, the biological stress response indicates nociceptive pathway activation, which may not be accompanied by conscious pain perception. Animals under anesthesia, for example, demonstrate a physiological response to noxious stimuli and tissue damage in the absence of conscious perception (136). EEG signal variations (Section 2.1) and advanced imaging, such as positron emission tomography (PET) and functional magnetic resonance imaging (fMRI), have been used in human and animal models to provide evidence of central nervous system processing and perception of pain (163); however, these costly techniques remain impractical for farm animal pain assessment. Consequently, physiological parameters are best utilized collectively with parameters that more closely capture the inner experience of the animal, such as behavioral indicators.
4.3 Evaluating pain in cattle: behavioral indicators
Pain-specific behaviors include defensive and avoidance behaviors. When animals encounter a painful stimulus, they demonstrate a “nocifensive withdrawal reflex” (14). An animal may demonstrate an exaggerated withdrawal reflex to an innocuous stimulus due to hyperalgesia of an injured area or may alter body position to avoid stimulating a painful region (4). For example, a cow with mastitis may demonstrate hyperreactivity to udder contact during milking (143) and reduced lying times to avoid pressure applied to a painful quarter (164). To quantify escape behavior evoked by a painful procedure, researchers measure exertion force on a squeeze chute using load cells and strain gauges (147, 165); steers demonstrate greater exertion forces in response to hot iron branding when compared to freeze branding, providing insight into the relative pain associated with different methods of cattle identification (165). Animals may also engage in behaviors directed towards an affected area, such as licking and scratching (135). Calves were observed licking the scrotal region post-castration (166) and scratching their heads following disbudding (167). Head shaking and ear flicking are also used as behavioral indicators of pain in calves post-disbudding (168–170).
Quantitative sensory testing (QST) is a method used to obtain nociceptive threshold measurements based on a behavioral response to determine tissue sensitivity. QST techniques involve the application of a noxious stimulus to a specific body region to evoke a defensive behavior, such as withdrawal (136). Methods of eliciting a reaction include thermal sensitivity tests and mechanical nociception tests, such as pressure algometry (61). The resulting nociceptive threshold measurements, or the lowest magnitude of the applied stimulus needed to induce a response, can be used to determine tissue sensitivity (136). Nociceptor threshold measurements have been used to investigate tissue sensitivity in calves after painful procedures such as disbudding (51, 171–175) and castration (176, 177). They have also been used to evaluate tissue sensitivity associated with painful disease conditions such as mastitis (178), lameness (179–181), and respiratory disease in calves (182).
The goal of a study conducted by Ohlheiser et al. (183) was to create an experimental pain induction model in dairy cows that could be adopted by future researchers to validate pain response measurements and evaluate analgesic efficacy. The researchers experimentally induced pain in cows using an intramuscular injection of oxytetracycline. They measured the mechanical nociceptive thresholds in locations that received the pain inducing drug and those that received a sham injection. They then measured these thresholds in the same locations in treatment animals administered flunixin meglumine (2.2 mg/kg IV) and control animals administered saline. Although this experiment aligned with the gold standard approach of pain assessment in both the presence and absence of analgesia, it is important to assess pharmaceutical efficacy in the presence of pain caused by the targeted disease condition. Furthermore, this study evaluated only mechanical nociceptive thresholds. Assessing multiple pain responses can increase the sensitivity and specificity of evaluation methods (135), which is essential to determining appropriate analgesic intervention.
Changes in vocalization patterns, social interactions, feed consumption and rumination, grooming behavior, and physical activity may be associated with pain (135). Pedometers, accelerometers, and rumen telemetry facilitate data collection of activity pattern alterations due to illness and pain (184). A literature review conducted by Mainau et al. (41) evaluated the available studies on activity pattern variations in dairy cattle with painful disease conditions, such as mastitis, lameness, metritis, and dystocia.
Changes in posture and facial expression may also indicate experienced pain. Postural changes represent an attempt to mitigate discomfort and have been detected in cows with metritis (13), lameness (185, 186), and disorders of the gastrointestinal tract (187). Facial expression studies have gained recent traction with scales developed for farm animals, including sheep, pigs, and cows (188–192). In a study conducted by Gleerup et al. (192), researchers evaluated pain in dairy cattle using a variety of pain indicators, including facial expression. The “Cow Pain Face” includes tense or low ears, tense or withdrawn eyes, dilated nostrils, and muscle tension above the eyes, nostrils, and along either side of the head (192). Although the researchers reported higher scores in cows with a painful clinical diagnosis, additional studies are needed to evaluate the diagnostic ability of this scoring system. A study conducted by Yamada et al. (193) used facial expression to evaluate the pain associated with different castration methods in bulls. Automated systems that analyze facial expressions have been developed for sheep to enhance objectivity and avoid inter-observer variability (194).
Behavioral parameters have also been used to develop pain scales. Validated pain scales exist for horses (195), dogs (196–199), and cats (200, 201); however, there is a lack of validated pain scales developed specifically for cattle. The UNESP-Botucatu unidimensional composite pain scale was refined and validated in 2014 to evaluate postoperative pain in cattle that undergo an orchiectomy (202). With this scale, readily observable behaviors, such as activity level, eating frequency, posture, and behaviors directed towards the source of pain, provide a non-invasive method of assessment to guide analgesic intervention.
A primary advantage of behavioral studies is that they can be conducted on-farm with direct observation and minimal subject manipulation. Additionally, pain-specific behaviors can be used to localize a painful region, guiding targeted therapy (135). Behavioral indicators are also rapidly observable and sensitive, as changes in behavior occur as cattle experience pain in real time, enabling early detection for timely analgesic intervention (135). This sensitivity, however, is compromised by the subjective nature of behavioral observations, which rely on individual interpretation (203). Studies that evaluate observer identification of cattle pain indicate variability based on education, age, and gender (107, 110). To increase testing sensitivity, observers must be experienced and trained in bias avoidance. Testing specificity is undermined by alterations in behavior that may be due to sickness, stress, or discomfort rather than nociception (135). Lastly, as previously discussed, a cow's evolutionary predisposition to stoicism challenges pain identification based on behavioral indicators (4). However, behavioral studies bring us closer to the inner experience of the animal.
4.4 Evaluating pain in cattle: affective state
Although many physiological parameters, such as cortisol and heart rate, reflect emotional arousal, few approaches to pain evaluation capture emotional valence, or whether an emotional experience is positive or negative (204). Although behavioral measures may bring us closer to the inner experience of an animal, many of these measurements simply indicate nociceptive activation and tissue damage avoidance without providing insight into the animal's psychology. Emerging studies in animal cognition, however, promise access to this inner experience that is difficult to quantify. Cognitive bias tests attempt to illuminate emotional valence by evaluating an animal's response to an ambiguous stimulus. Anticipation of a negative outcome demonstrates a “pessimistic” or negative affective state (136). Studies demonstrate a negative cognitive bias in calves following hot iron disbudding (205, 206), providing evidence that pain impacts emotional state. In a study conducted by Neave et al. (205), calves were trained to nose-touch a red and white screen; activation of the red screen resulted in a milk reward, whereas activation of the white screen resulted in a milk “time out.” Researchers found that within the 22 h following cautery disbudding, calves were less likely to approach ambiguously colored screens, indicating a negative cognitive bias. Reduction in play behavior (207–209) and intake of a sweetened solution (210) post-disbudding suggest that calves experience anhedonia—or disinterest in activities they once found pleasurable—as a result of experienced pain. Gingerich et al. (211) reported calves exhibiting “social withdrawal” following disbudding, as evidenced by a greater tendency to retreat to a secluded, sheltered area after this painful event. Anhedonia is associated with depression and anxiety in humans (212) and may include social isolation (213).
Conditioned place preference and avoidance tests further seek to illustrate an animal's perception of pain by demonstrating that, when provided the choice, animals will engage in behaviors that minimize the risk of repeated encounters with a painful experience. This was demonstrated by Ede et al. (214) in which calves chose to spend less time in the disbudding pen than the control pen (214). Providing animals with the agency to mitigate pain is demonstrated by self-medication trials in which animals are presented with the opportunity to consume feed containing analgesics. Danbury et al. (215) demonstrated that lame broiler chickens consumed more treated feed compared to control animals, and the degree of consumption correlated with lameness severity. Adcock and Tucker (168) studied disbudded versus control calves' motivation to obtain analgesia by pairing different visual stimuli with either a lidocaine injection or a saline injection. The researchers found that, 3 weeks after disbudding, the control calves demonstrated conditioned place aversion to the lidocaine-paired stimulus, whereas disbudded calves did not avoid the lidocaine-paired stimulus. The researchers concluded that disbudded calves were willing to absorb the aversive costs associated with a lidocaine injection to obtain relief for the more persistent pain resulting from disbudding. A study conducted by Colston et al. (216) provides evidence that calves are motivated to obtain cold therapy post-disbudding. Prior to disbudding, researchers exposed calves to a milk reward paired with habituation to cold and ambient packs applied to their horn buds. Following disbudding, calves more quickly approached and remained in contact with the milk reward associated with cold pack application, demonstrating motivation to obtain cold therapy for pain relief. According to Sneddon et al., to demonstrate the experience of pain rather than simply nociception, an animal must exhibit a “change in future behavioral decisions and motivational changes” (14). Tests that illuminate an animal's emotional state, memory-based avoidance behavior, and the decision to self-medicate thus provide evidence of the conscious perception of pain in animals.
5 Discussion: cattle pain and welfare
The fundamental mechanisms, nociceptive pathways, and central nervous system structures that lead to pain perception are highly conserved among mammalian species (217). We can therefore infer that cattle experience an aversion to pain based on commonalities in our underlying neuroanatomy and physiology. However, we must draw caution when relying on humans as a comparative model due to interspecies differences in pain expression—most notably a cow's inherent stoicism. Using an anthropomorphic paradigm to shape our understanding of pain expression can lead to the assumption that cattle experience a blunted pain response or fail to perceive pain altogether. Stoicism is a behavioral state deeply rooted in a cow's evolutionary history, and we must acknowledge this predisposition to conceal vulnerability when investigating appropriate measurements to identify pain and assess analgesic efficacy.
There is empirical evidence that cattle respond to pain as demonstrated by alterations in physiological, neuroendocrine, and behavioral parameters. These measurements bring us closer to understanding the experience of an animal in pain. Emerging studies in animal cognition promise greater access to an animal's inner experience. Studies that aim to assess preference and motivation, such as conditioned place aversion and cognitive bias tests, are gaining momentum. Available data illuminate the significant influence of pain on animal welfare as indicated by lasting memories of painful experiences (214), anhedonia (207, 210), and behaviors indicative of a negative affective state in animals following a pain-inducing event (205). Although these approaches require continued investigation and validation, available data reveal that, like humans, cattle experience psychological distress associated with pain.
5.1 Unabated pain, central sensitization, and cattle welfare
The impact of pain on welfare is further revealed by studies demonstrating the development of pathological pain states when pain is left untreated. Acute pain does not always dissipate with time; it may become chronic and pathologic when protracted. The mechanisms that cause and maintain central sensitization are complex; they encompass multiple molecular pathways and mechanisms, as well as a multitude of both neuronal and non-neuronal contributors. Although the causes and mechanisms of central sensitization have not been fully elucidated, current evidence revises historical notions that pain results only from pathology or noxious stimuli arising from the periphery. Pain can manifest from central neuronal plasticity due to prolonged and unabated pain, leading to fundamental functional alterations to the properties of neurons (218). These functional alterations may result in spontaneous nociceptor activity, reduced nociceptor thresholds, and expansion of nociceptor receptivity to both noxious and innocuous stimuli (19), amplifying pain. The available research provides evidence of chronic pain in cattle suffering from lameness (45, 219) and that prolonged lameness may lead to central nervous system alterations (43).
Further research investigating pain chronicity and the development of central sensitization in farm animals is needed to guide analgesic intervention and ensure adequate pain relief duration to prevent the development of pathological pain. There is a scarcity of studies evaluating neuropathic pain, and analgesic options for these conditions are severely limited. In human medicine, self-report of pain has enabled evidence-based treatment of neuropathic pain, which includes anticonvulsant, antidepressant, opioid, and cannabinoid pharmaceutical agents (220). There is minimal research investigating the use of these drugs in farm animals. Cross-disciplinary and transspecies engagement may advance pharmaceutical approaches to chronic pain management in cattle; however, novel pain relief investigation is hindered by the lack of established pain evaluation methods. Studies in humans demonstrate that neuropathic pain is associated with depression (221) and anxiety (222), and available research in cattle suggest similar negative emotional outcomes (Section 4.4), highlighting the need for additional research in this area to secure welfare.
5.2 Early life painful procedures and cattle welfare
Although it is important to perform painful management procedures at the “earliest age practicable” to minimize tissue damage (66), preemptive and multimodal analgesic approaches are still required early in life to safeguard welfare. Not only are these procedures painful in young animals, but the neonate may be particularly susceptible to long-term negative welfare outcomes due to the plasticity of the developing nervous system (48). When exposed to noxious stimuli, the immature nervous system may experience detrimental somatosensory and pain processing alterations (47). In addition to altered pain sensitivity, rodent models demonstrate that inflammation experienced in early life can have adverse effects on fear, anxiety, and stress responses, as well as cognitive and social development (223). More studies investigating farm animal neonatal neuroplasticity in the context of painful early life procedures are needed. Where research is lacking in these species, a comparative approach to understanding the negative long-term consequences of unrelieved pain using other animal models should be applied to prioritize pain management on farms.
The long-term welfare implications of neonatal pain underscore the need for alternative methods of management and genetic advances to preclude the need for painful procedures. This includes the use of sexed semen (224) and immunocastration (225) to minimize the need for castration, and the advancement of polled genetics (226) to eliminate the need for disbudding. When these procedures are required for animal and human safety and welfare, they must be performed as early as appropriate to minimize tissue damage, the least painful method should be employed, and a preemptive and multimodal analgesic approach implemented. Although the combined use of a local anesthetic and NSAID are considered standard practice for painful procedures such as disbudding due to veterinary and industry-driven guidelines (64, 65, 227), ensuring adequate duration analgesic relief based on pain chronicity is needed. Adcock and Tucker (51) reported that, following cautery disbudding, calves experience “evoked pain,” or increased sensitivity in response to a stimulus, for 9 weeks and “ongoing pain,” or pain experienced in the absence of a stimulus, for at least 3 weeks. This suggests that a single NSAID dose is insufficient in mitigating post-procedural pain following disbudding. It is also important to reduce situational stress through appropriate handling and restraint, as fear and anxiety may enhance the perception of pain (Section 2.1). Practical means of implementing positive reinforcement in farm settings should also be investigated, as pairing a painful stimulus with a reward may promote pain attenuation. However, we consider these methods of situational stress reduction supplemental and not as a replacement for appropriate analgesic intervention.
It is also important to note that the pain associated with disbudding and castration is well-documented in the literature; however, there are other understudied painful procedures that we must examine to enhance cattle welfare. Additional studies are needed to evaluate the pain associated with branding, ear tagging, and electroejaculation, as well as effective analgesic options to mitigate this pain. Although there are studies demonstrating that these procedures are painful (141, 228, 229), there is relatively less public awareness about them, minimizing efforts to prioritize alternative methods of management and analgesic intervention.
5.3 Painful disease conditions and cattle welfare
When approaching infectious disease treatment on farms, priority is given to disease resolution via the use of antimicrobial agents while the pain associated with disease receives less attention in clinical decision making. Although veterinary and industry recommendations exist to guide pain mitigation for painful procedures (64, 65, 227), similar guidelines are not widely available to guide the management of painful disease conditions. There is also minimal research investigating the pain associated with disease in cattle, especially diseases such as metritis and respiratory illness. Consequently, the pain associated with disease is frequently undertreated or untreated. Research that investigates the pain associated with cattle disease is needed to implement evidence-based analgesic protocols. Improved management practices are also required to reduce the risks associated with disease development. For example, we can minimize the risk of lameness through environmental management, scheduled hoof trimmings, and appropriate nutrition (230). Genetic selection for traits that prioritize health and resilience may also reduce the incidence of production-associated diseases.
The importance of pain prevention and mitigation applies to all animals raised for food (71). Although this review focuses on pain in cattle, we need to draw attention to pain in all species, especially those domesticated for human use. Similar challenges to analgesic protocol implementation exist among operations rearing different farm animal species; these challenges include constraints related to pain detection, attitudes towards an animal's experience of pain, insufficient pain management guidelines, and the scarcity of FDA-approved drugs labelled for pain control (71). The barriers to pain prevention and mitigation are not insurmountable; further research investigating and validating bovine indicators of pain, veterinarian-driven initiatives to communicate the importance of pain management, and public awareness of the detrimental impact of pain on cattle welfare together may shift society towards farm animal pain prioritization and incentivize drug manufacturers to pursue drug approval. The available literature indicates that we must implement preemptive and multimodal pain management protocols to address the welfare of animals under our care; however, pain management prioritization—even in the presence of regulatory requirements—hinges on shifts in attitude toward pain and available, convenient, and effective analgesic options.
Author contributions
AZ: Conceptualization, Writing – original draft, Writing – review & editing, Methodology. SM: Funding acquisition, Methodology, Supervision, Writing – review & editing. JC: Supervision, Writing – review & editing.
Funding
The author(s) declare financial support was received for the research, authorship, and/or publication of this article.
This manuscript was funded by the Cornell University College of Veterinary Medicine Veterinary Investigator Program and unrestricted funds of Cornell University College of Veterinary Medicine made available to SM.
Acknowledgments
The authors are grateful to the Cornell Veterinary Investigator Program for providing the resources to support the work involved in writing this manuscript. Elements of this manuscript were presented in poster format at the Pain in Animals Workshop hosted by the National Institutes of Health September 26–27, 2023, and in webinar format for the Veterinary Association for Farm Animal Welfare on May 8, 2024.
Conflict of interest
The authors declare that the research was conducted in the absence of any commercial or financial relationships that could be construed as a potential conflict of interest.
Publisher's note
All claims expressed in this article are solely those of the authors and do not necessarily represent those of their affiliated organizations, or those of the publisher, the editors and the reviewers. Any product that may be evaluated in this article, or claim that may be made by its manufacturer, is not guaranteed or endorsed by the publisher.
References
1. de Lahunta A, Glass E, Kent M. General sensory systems: general proprioception and general somatic afferent. In: de Lahunta A, Glass E, Kent M, editors. Veterinary Neuroanatomy and Clinical Neurology. 4th ed. St. Louis. MO: Saunders Elsevier (2015). p. 237–56.
2. Purves D, Augustine GJ, Fitzpatrick CE, Katz LC, LaMantia AS, McNamara JO, et al. Nociceptors. In: Purves D, Augustine GJ, Fitzpatrick CE, Katz LC, LaMantia A-S JOM, editors. Neuroscience. Sunderland, MA: Sinauer Associates, Inc. (2001). p. 209–22.
3. Molony V, Kent JE. Assessment of acute pain in farm animals using behavioral and physiological measurements. J Anim Sci. (1997) 75(1):266–72. doi: 10.2527/1997.751266x
4. Weary DM, Niel L, Flower FC, Fraser D. Identifying and preventing pain in animals. Appl Anim Behav Sci. (2006) 100(1–2):64–76. doi: 10.1016/j.applanim.2006.04.013
5. Marquette GA, Ronan S, Earley B. Calf disbudding—animal welfare considerations. J Appl Anim Res. (2023) 51(1):616–23. doi: 10.1080/09712119.2023.2264912
6. Coetzee JF. A review of pain assessment techniques and pharmacological approaches to pain relief after bovine castration: practical implications for cattle production within the United States. Appl Anim Behav Sci. (2011) 135(3):192–213. doi: 10.1016/j.applanim.2011.10.016
7. Tucker CB, Mintline EM, Banuelos J, Walker KA, Hoar B, Varga A, et al. Pain sensitivity and healing of hot-iron cattle brands. J Anim Sci. (2014) 92(12):5674–82. doi: 10.2527/jas.2014-7887
8. Coetzee JF, Shearer JK, Stock ML, Kleinhenz MD, van Amstel SR. An update on the assessment and management of pain associated with lameness in cattle. Vet Clin North Am Food Anim Pract. (2017) 33(2):389–411. doi: 10.1016/j.cvfa.2017.02.009
9. de Boyer des Roches A, Faure M, Lussert A, Herry V, Rainard P, Durand D, et al. Behavioral and patho-physiological response as possible signs of pain in dairy cows during Escherichia coli mastitis: a pilot study. J Dairy Sci. (2017) 100(10):8385–97. doi: 10.3168/jds.2017-12796
10. Braun U, Gerspach C, Ohlerth S, Warislohner S, Nuss K. Aetiology, diagnosis, treatment and outcome of traumatic reticuloperitonitis in cattle. Vet J. (2020) 255:105424. doi: 10.1016/j.tvjl.2020.105424
11. Martin MS, Kleinhenz MD, White BJ, Johnson BT, Montgomery SR, Curtis AK, et al. Assessment of pain associated with bovine respiratory disease and its mitigation with flunixin meglumine in cattle with induced bacterial pneumonia. J Anim Sci. (2022) 100(2):skab373. doi: 10.1093/jas/skab373
12. Gladden N, Ellis K, Martin J, McKeegan D. Administration of ketoprofen affects post-partum lying behaviours of Holstein dairy cows regardless of whether parturition is assisted. Vet Rec. (2021) 189(6):245-+. doi: 10.1002/vetr.300
13. Stojkov J, von Keyserlingk MA, Marchant JN, Weary DM. Assessment of visceral pain associated with metritis in dairy cows. J Dairy Sci. (2015) 98(8):5352–61. doi: 10.3168/jds.2014-9296
14. Sneddon LU. Evolution of nociception and pain: evidence from fish models. Philos Trans R Soc Lond B Biol Sci. (2019) 374(1785):20190290. doi: 10.1098/rstb.2019.0290
15. Im SH, Galko MJ. Pokes, sunburn, and hot sauce: drosophila as an emerging model for the biology of nociception. Dev Dyn. (2012) 241(1):16–26. doi: 10.1002/dvdy.22737
16. Sneddon LU. Comparative physiology of nociception and pain. Physiology. (2018)33(1):63–73. doi: 10.1152/physiol.00022.2017
17. Thomson C, Hahn C. Ascending Somatic Sensory Tracts and Conscious Sensory Systems. Veterinary Neuroanatomy. Edinburgh: Saunders Ltd. (2012). p. 59–66.
18. Stoelting RK, Hillier SC. Pain. In: Flood P, Rathmell JP, Shafer S, editors. Pharmacology and Physiology in Anesthetic Practice. 4th ed. Philadelphia, PA: Wolters Kluwer (2006). p. 707–17.
19. Ringkamp M, Raja SN, Campbell JN, Meyer RA. Neurobiology of pain: peripheral mechanisms of cutaneous nociception. In: McMahon SB, Koltzenburg M, Tracey I, Turk DC, editors. Wall and Melzack’s Textbook of Pain. 6th ed. Philadelphia, PA: Elsevier Saunders (2013). p. 1–30.
20. McCune CM, Murrell JC, Nolan AM, White KL, Wright BD. Nociception and pain. In: Grimm KA, Lamont LA, Tranquili WJ, Greene SA, Robertson SA, editors. Veterinary Anesthesia and Analgesia Fifth ed. Ames, Iowa: Wiley & Sons, Inc. (2015). p. 584–623.
21. Coetzee JF. A review of analgesic compounds used in food animals in the United States. Vet Clin North Am Food Anim Pract. (2013) 29(1):11–28.
22. Zhao Z-F, Wan Y. Electrophysiological Signature of Pain. Advances in Pain Research: Mechanisms and Modulation of Chronic Pain. Singapore: Springer (2018). p. 167–77.
23. Nir R-R, Sinai A, Raz E, Sprecher E, Yarnitsky D. Pain assessment by continuous EEG: association between subjective perception of tonic pain and peak frequency of alpha oscillations during stimulation and at rest. Brain Res. (2010) 1344:77–86. doi: 10.1016/j.brainres.2010.05.004
24. Gibson TJ, Johnson CB, Stafford KJ, Mitchinson SL, Mellor DJ. Validation of the acute electroencephalographic responses of calves to noxious stimulus with scoop dehorning. N Z Vet J. (2007) 55(4):152–7. doi: 10.1080/00480169.2007.36760
25. Bergamasco L, Edwards-Callaway LN, Bello NM, Mijares S, Cull CA, Mosher RA, et al. Unmitigated surgical castration in calves of different ages: electroencephalographic and neurohormonal findings. Animals (Basel). (2021) 11(6):1791. doi: 10.3390/ani11061791
26. Bergamasco L, Coetzee JF, Gehring R, Murray L, Song T, Mosher RA. Effect of intravenous sodium salicylate administration prior to castration on plasma cortisol and electroencephalography parameters in calves. J Vet Pharmacol Ther. (2011) 34(6):565–76. doi: 10.1111/j.1365-2885.2011.01269.x
27. Dockweiler J, Coetzee J, Edwards-Callaway L, Bello N, Glynn H, Allen K, et al. Effect of castration method on neurohormonal and electroencephalographic stress indicators in Holstein calves of different ages. J Dairy Sci. (2013) 96(7):4340–54. doi: 10.3168/jds.2012-6274
28. Haga HA, Ranheim B. Castration of piglets: the analgesic effects of intratesticular and intrafunicular lidocaine injection. Vet Anaesth Analg. (2005) 32(1):1–9. doi: 10.1111/j.1467-2995.2004.00225.x
29. Murrell J, Johnson C. Neurophysiological techniques to assess pain in animals. J Vet Pharmacol Ther. (2006) 29(5):325–35. doi: 10.1111/j.1365-2885.2006.00758.x
30. Mota-Rojas D, Napolitano F, Strappini A, Orihuela A, Ghezzi MD, Hernández-Ávalos I, et al. Pain at the slaughterhouse in ruminants with a focus on the neurobiology of sensitisation. Animals (Basel). (2021) 11(4):1085. doi: 10.3390/ani11041085
31. Neugebauer V, Galhardo V, Maione S, Mackey SC. Forebrain pain mechanisms. Brain Res Rev. (2009) 60(1):226–42. doi: 10.1016/j.brainresrev.2008.12.014
32. Villemure C, Bushnell MC. Cognitive modulation of pain: how do attention and emotion influence pain processing? Pain. (2002) 95(3):195–9. doi: 10.1016/S0304-3959(02)00007-6
33. Lomb J, Mauger A, von Keyserlingk MAG, Weary DM. Effects of positive reinforcement training for heifers on responses to a subcutaneous injection. J Dairy Sci. (2021) 104(5):6146–58. doi: 10.3168/jds.2020-19463
34. Ede T, von Keyserlingk MA, Weary DM. Approach-aversion in calves following injections. Sci Rep. (2018) 8(1):9443. doi: 10.1038/s41598-018-27669-7
35. McEntire DM, Kirkpatrick DR, Dueck NP, Kerfeld MJ, Smith TA, Nelson TJ, et al. Pain transduction: a pharmacologic perspective. Expert Rev Clin Pharmacol. (2016) 9(8):1069–80. doi: 10.1080/17512433.2016.1183481
36. Xu Q, Yaksh TL. A brief comparison of the pathophysiology of inflammatory versus neuropathic pain. Curr Opin Anaesthesiol. (2011) 24(4):400–7. doi: 10.1097/ACO.0b013e32834871df
37. Anderson DE, Muir WW. Pain management in cattle. Vet Clin North Am Food Anim Pract. (2005) 21(3):623–35. doi: 10.1016/j.cvfa.2005.07.002
38. Costigan M, Scholz J, Woolf CJ. Neuropathic pain: a maladaptive response of the nervous system to damage. Annu Rev Neurosci. (2009) 32:1–32. doi: 10.1146/annurev.neuro.051508.135531
39. Dawes JM, Andersson DA, Bennett DLH, Bevan S, McMahon SB. Neurobiology of pain: inflammatory mediators and modulators of pain. In: McMahon SB, Koltzenburg M, Tracey I, Turk DC, editors. Wall and Melzack’s Textbook of Pain. 6th ed. Philadelphia, PA: Elsevier Saunders (2013). p. 48–67.
40. Woolf CJ. Central sensitization: implications for the diagnosis and treatment of pain. Pain. (2011) 152(3 Suppl):S2–S15. doi: 10.1016/j.pain.2010.09.030
41. Mainau E, Llonch P, Temple D, Goby L, Manteca X. Alteration in activity patterns of cows as a result of pain due to health conditions. Animals. (2022) 12(2):176. doi: 10.3390/ani12020176
42. Sandküler J. Neurobiology of pain: spinal cord plasticity and pain. In: McMahon SB, Koltzenburg M, Tracey I, Turk DC, editors. Wall and Melzack’s Textbook of Pain. 6th ed. Philadelphia, PA: Elsevier Saunders (2013). p. 94–110.
43. Herzberg D, Strobel P, Chihuailaf R, Ramirez-Reveco A, Muller H, Werner M, et al. Spinal reactive oxygen species and oxidative damage mediate chronic pain in lame dairy cows. Animals (Basel). (2019) 9(9):693. doi: 10.3390/ani9090693
44. Herzberg D, Strobel P, Ramirez-Reveco A, Werner M, Bustamante H. Chronic inflammatory lameness increases cytokine concentration in the spinal cord of dairy cows. Front Vet Sci. (2020) 7:125. doi: 10.3389/fvets.2020.00125
45. Whay H, Waterman A, Webster A, O'brien J. The influence of lesion type on the duration ofhyperalgesia associated with hindlimb lameness in dairy cattle. Vet J. (1998) 156(1):23–9. doi: 10.1016/S1090-0233(98)80058-0
46. Herzberg D, Strobel P, Müller H, Meneses C, Werner M, Bustamante H. Proteomic profiling of proteins in the dorsal horn of the spinal cord in dairy cows with chronic lameness. PloS One. (2020) 15(1):e0228134. doi: 10.1371/journal.pone.0228134
47. Williams MD, Lascelles BDX. Early neonatal pain-A review of clinical and experimental implications on painful conditions later in life. Front Pediatr. (2020) 8:30. doi: 10.3389/fped.2020.00030
48. Schwaller F, Fitzgerald M. The consequences of pain in early life: injury-induced plasticity in developing pain pathways. Eur J Neurosci. (2014) 39(3):344–52. doi: 10.1111/ejn.12414
49. Zouikr I, Karshikoff B. Lifetime modulation of the pain system via neuroimmune and neuroendocrine interactions. Front immunol. (2017) 8:276. doi: 10.3389/fimmu.2017.00276
50. Burke NN, Fan CY, Trang T. Microglia in health and pain: impact of noxious early life events. Exp Physiol. (2016) 101(8):1003–21. doi: 10.1113/EP085714
51. Adcock SJ, Tucker CB. The effect of disbudding age on healing and pain sensitivity in dairy calves. J Dairy Sci. (2018) 101(11):10361–73. doi: 10.3168/jds.2018-14987
52. Adcock SJJ, Tucker CB. Conditioned place preference reveals ongoing pain in calves 3 weeks after disbudding. Sci Rep. (2020) 10(1):3849. doi: 10.1038/s41598-020-60260-7
53. Casoni D, Mirra A, Suter M, Gutzwiller A, Spadavecchia C. Can disbudding of calves (one versus four weeks of age) induce chronic pain? Physiol Behav. (2019) 199:47–55. doi: 10.1016/j.physbeh.2018.11.010
54. Adcock SJ, Tucker CB. The effect of early burn injury on sensitivity to future painful stimuli in dairy heifers. PloS One. (2020) 15(6):e0233711. doi: 10.1371/journal.pone.0233711
55. Eicher S, Cheng HW, Sorrells A, Schutz M. Behavioral and physiological indicators of sensitivity or chronic pain following tail docking. J Dairy Sci. (2006) 89(8):3047–51. doi: 10.3168/jds.S0022-0302(06)72578-4
56. Sandercock DA, Barnett MW, Coe JE, Downing AC, Nirmal AJ, Di Giminiani P, et al. Transcriptomics analysis of porcine caudal dorsal root ganglia in tail amputated pigs shows long-term effects on many pain-associated genes. Front Vet Sci. (2019) 6:314. doi: 10.3389/fvets.2019.00314
57. French N, Morgan K. Neuromata in docked lambs’ tails. Res Vet Sci. (1992) 52(3):389–90. doi: 10.1016/0034-5288(92)90045-4
58. Sominsky L, Meehan CL, Walker AK, Bobrovskaya L, McLaughlin EA, Hodgson DM. Neonatal immune challenge alters reproductive development in the female rat. Horm Behav. (2012) 62(3):345–55. doi: 10.1016/j.yhbeh.2012.02.005
59. Walker AK, Hawkins G, Sominsky L, Hodgson DM. Transgenerational transmission of anxiety induced by neonatal exposure to lipopolysaccharide: implications for male and female germ lines. Psychoneuroendocrinology. (2012) 37(8):1320–35. doi: 10.1016/j.psyneuen.2012.01.005
60. Clark C, Murrell J, Fernyhough M, O'Rourke T, Mendl M. Long-term and trans-generational effects of neonatal experience on sheep behaviour. Biol Lett. (2014) 10(7):20140273. doi: 10.1098/rsbl.2014.0273
61. Millman ST. Behavioral responses of cattle to pain and implications for diagnosis, management, and animal welfare. Vet Clin North Am Food Anim Pract. (2013) 29(1):47–58.23438399
62. Rauch A, Sharp JS. Ohians’ Attitudes About Animal Welfare: A Topical Report from the 2004 Ohio Survey of Food, Agricultural, and Environmental Issues. Columbus, OH: The Ohio State University (2005).
63. Animal Welfare Institute (AWI). Survey of Consumer Attitudes About Pain Relief for Physical Alterations of Farm Animals. Animal Welfare Institute (AWI) (2022). Available online at: https://awionline.org/sites/default/files/uploads/documents/Survey-Consumer-Attitudes-Pain-Relief.pdf
64. American Association of Bovine Practitioners (AABP). Castration Guidelines. Ashland, OH: American Association of Bovine Practitioners (AABP) (2019). Available online at: https://www.aabp.org/Resources/AABP_Guidelines/Castration_Guidelines-2019.pdf
65. American Association of Bovine Practitioners (AABP). Dehorning Guidelines. Ashland, OH: American Association of Bovine Practitioners (AABP) (2019). Available online at: https://aabp.org/Resources/AABP_Guidelines/Dehorning-2019.pdf
66. American Veterinary Medical Association (AVMA). Bovine Disbudding and Dehorning. American Veterinary Medical Association (AVMA) (2024). Available online at: https://www.avma.org/KB/Policies/Pages/Castration-and-Dehorning-of-Cattle.aspx
67. Johnstone ECS, Coetzee JF, Pinedo PJ, Edwards-Callaway L. Current attitudes of veterinarians and producers regarding the use of local and systemic analgesia in beef and dairy cattle in the United States. J Am Vet Med Assoc. (2021) 258(2):197–209. doi: 10.2460/javma.258.2.197
68. Program NDF. Animal Care Reference Manual 2020. (2020). Available online at: https://nationaldairyfarm.com/wp-content/uploads/2020/02/Animal-Care-V4-Manual-Print-Friendly.pdf (Accessed March 4, 2024).
69. Smith ER, Modric S. Regulatory considerations for the approval of analgesic drugs for cattle in the United States. In: Coetzee JF, editor. Veterinary Clinics of North America: Food Animal Practice. 29. 1st ed. Philadelphia, PA: Elsevier (2013). p. 1–10.
70. Food and Drug Administration (FDA). Animal Medicinal Drug Use Clarification Act of 1994. (1994). Available online at: https://www.fda.gov/animal-veterinary/guidance-regulations/animal-medicinal-drug-use-clarification-act-1994-amduca (updated April 19, 2022).
71. Kleinhenz MD, Viscardi AV, Coetzee JF. INVITED REVIEW: on-farm pain management of food production animals. Applied Animal Science. (2021) 37(1):77–87. doi: 10.15232/aas.2020-02106
72. Fajt VR, Wagner SA, Norby B. Analgesic drug administration and attitudes about analgesia in cattle among bovine practitioners in the United States. J Am Vet Med Assoc. (2011) 238(6):755–67. doi: 10.2460/javma.238.6.755
73. Ritter JM, Flower R, Henderson G, Loke YK, MacEwan D, Rang HP. Local anaesthetics and other drugs affecting sodium channels. In: Ritter JM, Flower R, Henderson G, Loke YK, MacEwan D, Rang HP, editors. Rang and Dalés Pharmacology. Ninth ed. Edinburgh: Elsevier; 2019. p. 563–8.
74. Sellers G, Lin H, Riddell M, Ravis W, Duran S, Givens M. Pharmacokinetics of lidocaine in serum and milk of mature Holstein cows. J Vet Pharmacol Ther. (2009) 32(5):446–50. doi: 10.1111/j.1365-2885.2009.01061.x
75. Lin H. Pain Management for Farm Animals. In: Lin H, Passler T, Clark-Price S, editors. Farm Animal Anesthesia: Cattle, Small Ruminants, Camelids, and Pigs. 2nd ed. Hoboken, NJ: John Wiley & Sons, Inc (2022). p. 207–46. doi: 10.1002/9781119672661.ch10
76. McKay W, Morris R, Mushlin P. Sodium bicarbonate attenuates pain on skin infiltration with lidocaine, with or without epinephrine. Anesth Analg. (1987) 66(6):572–4. doi: 10.1213/00000539-198706000-00015
77. Curatolo M, Petersen-Felix S, Arendt-Nielsen L, Lauber R, Hogstrom H, Scaramozzino P, et al. Adding sodium bicarbonate to lidocaine enhances the depth of epidural blockade. Anesth Analg. (1998) 86(2):341–7. doi: 10.1213/00000539-199802000-00024
78. Sinnott CJ, Garfield JM, Thalhammer JG, Strichartz GR. Addition of sodium bicarbonate to lidocaine decreases the duration of peripheral nerve block in the rat. J Am Soc Anesth. (2000) 93(4):1045–52. doi: 10.1097/00000542-200010000-00028
79. Dehghani SN, Bigham AS. Comparison of caudal epidural anesthesia by use of lidocaine versus a lidocaine–magnesium sulfate combination in cattle. Am J Vet Res. (2009) 70(2):194–7. doi: 10.2460/ajvr.70.2.194
80. Ritter JM, Flower R, Henderson G, Loke YK, MacEwan D, Rang HP. Analgesic drugs. In: Ritter JM, Flower R, Henderson G, Loke YK, MacEwan D, Rang HP, editors. Rang and Dalés Pharmacology. 9th ed. Edinburgh: Elsevier (2019). p. 542–62.
81. Odensvik K, Johansson IM. High-performance liquid chromatography method for determination of fhinixin in bovine plasma and pharmacokinetics after single and repeated doses of the drug. Am J Vet Res. (1995) 56(4):489–95. doi: 10.2460/ajvr.1995.56.04.489
82. Anderson K, Neff-Davis C, Davis L, Bass V. Pharmacokinetics of flunixin meglumine in lactating cattle after single and multiple intramuscular and intravenous administrations. Am J Vet Res. (1990) 51(9):1464–7. doi: 10.2460/ajvr.1990.51.09.1464
83. Odensvik K. Pharmacokinetics of flunixin and its effect on prostaglandin F2α metabolite concentrations after oral and intravenous administration in heifers. J Vet Pharmacol Ther. (1995) 18(4):254–9. doi: 10.1111/j.1365-2885.1995.tb00589.x
84. Hardee G, Smith J, Harris S. Pharmacokinetics of flunixin meglumine in the cow. Res Vet Sci. (1985) 39(1):110–2. doi: 10.1016/S0034-5288(18)31783-1
85. Kleinhenz MD, Van Engen N, Gorden PJ, KuKanich B, Rajewski SM, Walsh P, et al. The pharmacokinetics of transdermal flunixin meglumine in Holstein calves. J Vet Pharmacol Ther. (2016) 39(6):612–5. doi: 10.1111/jvp.12314
86. Merck Animal Health USA. Merck Animal Health Receives U.S. FDA Approval of Expanded Indication for Banamine Transdermal (flunixin transdermal solution). (2023). Available online at: https://www.merck-animal-health-usa.com/newsroom/merck-animal-health-receives-u-s-fda-approval-of-expanded-indication-for-banamine-transdermal-flunixin-transdermal-solution (Accessed January 22, 2024).
87. Lohuis J, Van Werven T, Brand A, Van Miert A, Rohde E, Ludwig B, et al. Phmacodynamics and pharmacokinetics of carprofen, a non-steroidal anti-inflammatory drug, in healthy cows and cows with Escherichia coli endotoxin-induced mastitis. J Vet Pharmacol Ther. (1991) 14(3):219–29. doi: 10.1111/j.1365-2885.1991.tb00830.x
88. Landoni M, Cunningham F, Lees P. Pharmacokinetics and pharmacodynamics of ketoprofen in calves applying PK/PD modelling. J Vet Pharmacol Ther. (1995) 18(5):315–24.8587147
89. Coetzee JF, KuKanich B, Mosher R, Allen PS. Pharmacokinetics of intravenous and oral meloxicam in ruminant calves. Vet Ther. (2009) 10(4):E1–8.20425727
90. Warner R, Ydstie JA, Wulf LW, Gehring R, Coetzee JF, Mochel JP, et al. Comparative pharmacokinetics of meloxicam between healthy post-partum vs. Mid-lactation dairy cattle. Front Vet Sci. (2020) 7:548. doi: 10.3389/fvets.2020.00548
91. Merck Veterinary Manual. Nonsteroidal Anti-inflammatory Drugs. (2014). Available online at: https://www.merckvetmanual.com/pharmacology/anti-inflammatory-agents/nonsteroidal-anti-inflammatory-drugs (Accessed November 10, 2023).
92. Stock ML, Coetzee JF. Clinical pharmacology of analgesic drugs in cattle. Vet Clin North Am Food Anim Pract. (2015) 31(1):113–38., vi-vii. doi: 10.1016/j.cvfa.2014.11.002
93. De Veau I, Pedersoli W, Cullison R, Baker J. Pharmacokinetics of phenylbutazone in beef steers. J Vet Pharmacol Ther. (2002) 25(3):195–200. doi: 10.1046/j.1365-2885.2002.00406.x
94. De Veau EJ, Pedersoli W, Cullison R. Pharmacokinetics of phenylbutazone in plasma and milk of lactating dairy cows. J Vet Pharmacol Ther. (1998) 21(6):437–43. doi: 10.1046/j.1365-2885.1998.00167.x
95. Arifah A, Lees P. Pharmacodynamics and pharmacokinetics of phenylbutazone in calves. J Vet Pharmacol Ther. (2002) 25(4):299–309. doi: 10.1046/j.1365-2885.2002.00421.x
96. Stock ML, Gehring R, Barth LA, Wulf LW, Coetzee JF. Pharmacokinetics of firocoxib in preweaned calves after oral and intravenous administration. J Vet Pharmacol Ther. (2014) 37(5):457–63. doi: 10.1111/jvp.12124
97. Wagner SA, Fajt VR, Lo CP, Byrd CJ. Pharmacokinetics of oral firocoxib in un-weaned calves. J Vet Pharmacol Ther. (2021) 44(5):793–8. doi: 10.1111/jvp.12971
98. Gingerich D, Baggot J, Yeary R. Pharmacokinetics and dosage of aspirin in cattle. J Am Vet Med Assoc. (1975) 167(10):945–8.1184427
99. Fritz BR, Kleinhenz MD, Montgomery SR, Magnin G, Martin MS, Weeder M, et al. Determination of milk concentrations and pharmacokinetics of salicylic acid following acetylsalicylic acid (aspirin) administration in postpartum dairy cows. J Dairy Sci. (2022) 105(12):9869–81. doi: 10.3168/jds.2021-21507
100. Baldridge SL, Coetzee JF, Dritz SS, Reinbold JB, Gehring R, Havel J, et al. Pharmacokinetics and physiologic effects of intramuscularly administered xylazine hydrochloride-ketamine hydrochloride-butorphanol tartrate alone or in combination with orally administered sodium salicylate on biomarkers of pain in Holstein calves following castration and dehorning. Am J Vet Res. (2011) 72(10):1305–17. doi: 10.2460/ajvr.72.10.1305
101. Seddighi R, Doherty TJ. Field sedation and anesthesia of ruminants. Vet Clin Food Anim Pract. (2016) 32(3):553–70. doi: 10.1016/j.cvfa.2016.05.002
102. Annetta MG, Iemma D, Garisto C, Tafani C, Proietti R. Ketamine: new indications for an old drug. Curr Drug Targets. (2005) 6(7):789–94. doi: 10.2174/138945005774574533
103. Coetzee JF, Mosher RA, Kohake LE, Cull CA, Kelly LL, Mueting SL, et al. Pharmacokinetics of oral gabapentin alone or co-administered with meloxicam in ruminant beef calves. Vet J. (2011) 190(1):98–102. doi: 10.1016/j.tvjl.2010.08.008
104. American Veterinary Medical Association (AVMA). American Veterinary Medical Association: Extralabel Drug Use and AMDUCA: FAQ 2020. Available online at: https://www.avma.org/extralabel-drug-use-and-amduca-faq (Accessed December 3, 2023).
105. Anil L, Anil SS, Deen J. Pain detection and amelioration in animals on the farm: issues and options. J Appl Anim Welf Sci. (2005) 8(4):261–78. doi: 10.1207/s15327604jaws0804_3
106. Edwards-Callaway LN, Keller KP, Oselinsky K, Johnstone E, Cramer C, Roman-Muniz N, et al. A nationwide survey on producer and veterinarian perceptions of the painfulness of procedures and disease states in dairy and beef cattle. Front Pain Res (Lausanne). (2023) 4:1059224. doi: 10.3389/fpain.2023.1059224
107. Remnant JG, Tremlett A, Huxley JN, Hudson CD. Clinician attitudes to pain and use of analgesia in cattle: where are we 10 years on? Veterinary Record. (2017) 181(15):400-. doi: 10.1136/vr.104428
108. Huxley JN, Whay HR. Current attitudes of cattle practitioners to pain and the use of analgesics in cattle. Vet Rec. (2006) 159(20):662–8. doi: 10.1136/vr.159.20.662
109. Sumner CL, von Keyserlingk MAG, Weary DM. Perspectives of farmers and veterinarians concerning dairy cattle welfare. Anim Front. (2018) 8(1):8–13. doi: 10.1093/af/vfx006
110. Tschoner T, Mueller KR, Zablotski Y, Feist M. Pain assessment in cattle by use of numerical rating and visual analogue scales-A systematic review and meta-analysis. Animals (Basel). (2024) 14(2):351. doi: 10.3390/ani14020351
111. Livingston A. Ethical issues regarding pain in animals. J Am Vet Med Assoc. (2002) 221(2):229–33. doi: 10.2460/javma.2002.221.229
112. Broom DM. Welfare assessment. In: Broom DM, editor. Broom and Fraser’s Domestic Animal Behaviour and Welfare. 6th ed. Wallingford, UK: CABI (2022). p. 83–100.
113. Leslie KE, Petersson-Wolfe CS. Assessment and management of pain in dairy cows with clinical mastitis. Vet Clin North Am Food Anim Pract. (2012) 28(2):289–305. doi: 10.1016/j.cvfa.2012.04.002
114. Khera T, Rangasamy V. Cognition and pain: a review. Front Psychol. (2021) 12:673962. doi: 10.3389/fpsyg.2021.673962
115. Muralidharan A, Smith MT. Pain, analgesia and genetics. J Pharm Pharmacol. (2011) 63(11):1387–400. doi: 10.1111/j.2042-7158.2011.01340.x
116. Martin MS, Kleinhenz MD, Edwards-Callaway LN, Engle TE, Guimaraes O, Schafer DW, et al. The effect of breed, sex, and oral meloxicam administration on pain biomarkers following hot-iron branding in Hereford and Angus calves. J Anim Sci. (2022) 100(3):skac038. doi: 10.1093/jas/skac038
117. McDonald JM, Law J, Mosheim R. Report Number 274. Consolidation in US Dairy Farming. Economic Research Report. Washington, DC: United States Department of Agriculture (USDA) (2020).
118. Morrone S, Dimauro C, Gambella F, Cappai MG. Industry 4.0 and precision livestock farming (PLF): an up to date overview across animal productions. Sensors. (2022) 22(12):4319. doi: 10.3390/s22124319
119. Neethirajan S, Kemp B. Digital livestock farming. Sens Biosensing Res. (2021) 32:100408. doi: 10.1016/j.sbsr.2021.100408
120. Tuyttens FA, Molento CF, Benaissa S. Twelve threats of precision livestock farming (PLF) for animal welfare. Front Vet Sci. (2022) 9:889623. doi: 10.3389/fvets.2022.889623
121. Lovarelli D, Bacenetti J, Guarino M. A review on dairy cattle farming: is precision livestock farming the compromise for an environmental, economic and social sustainable production? J Cleaner Prod. (2020) 262:121409. doi: 10.1016/j.jclepro.2020.121409
122. Newton HP, O’Connor AM. The economics of pain management. Vet Clin Food Anim Pract. (2013) 29(1):229–50. doi: 10.1016/j.cvfa.2012.11.010
123. Coetzee JF, Edwards L, Mosher R, Bello N, O'connor A, Wang B, et al. Effect of oral meloxicam on health and performance of beef steers relative to bulls castrated on arrival at the feedlot. J Anim Sci. (2012) 90(3):1026–39. doi: 10.2527/jas.2011-4068
124. Ting S, Earley B, Crowe M. Effect of repeated ketoprofen administration during surgical castration of bulls on cortisol, immunological function, feed intake, growth, and behavior. J Anim Sci. (2003) 81(5):1253–64. doi: 10.2527/2003.8151253x
125. Ting S, Earley B, Hughes J, Crowe M. Effect of ketoprofen, lidocaine local anesthesia, and combined xylazine and lidocaine caudal epidural anesthesia during castration of beef cattle on stress responses, immunity, growth, and behavior. J Anim Sci. (2003) 81(5):1281–93. doi: 10.2527/2003.8151281x
126. Fisher A, Crowe M, De La Varga MA, Enright W. Effect of castration method and the provision of local anesthesia on plasma cortisol, scrotal circumference, growth, and feed intake of bull calves. J Anim Sci. (1996) 74(10):2336–43. doi: 10.2527/1996.74102336x
127. Green LE, Hedges VJ, Schukken YH, Blowey RW, Packington AJ. The impact of clinical lameness on the milk yield of dairy cows. J Dairy Sci. (2002) 85(9):2250–6. doi: 10.3168/jds.S0022-0302(02)74304-X
128. Sulayeman M, Fromsa A. Lameness in dairy cattle: prevalence, risk factors and impact on milk production. Glob Veterin. (2012) 8(1):1–7.
129. Bicalho R, Warnick L, Guard C. Strategies to analyze milk losses caused by diseases with potential incidence throughout the lactation: a lameness example. J Dairy Sci. (2008) 91(7):2653–61. doi: 10.3168/jds.2007-0744
130. Tsousis G, Boscos C, Praxitelous A. The negative impact of lameness on dairy cow reproduction. Reprod Domest Anim. (2022) 57:33–9. doi: 10.1111/rda.14210
131. Bach A, Dinarés M, Devant M, Carré X. Associations between lameness and production, feeding and milking attendance of Holstein cows milked with an automatic milking system. J Dairy Res. (2007) 74(1):40–6. doi: 10.1017/S0022029906002184
132. Rajala P, Gröhn Y. Effects of dystocia, retained placenta, and metritis on milk yield in dairy cows. J Dairy Sci. (1998) 81(12):3172–81. doi: 10.3168/jds.S0022-0302(98)75883-7
133. Fourichon C, Seegers H, Malher X. Effect of disease on reproduction in the dairy cow: a meta-analysis. Theriogenology. (2000) 53(9):1729–59. doi: 10.1016/S0093-691X(00)00311-3
134. Gröhn Y, Rajala-Schultz P, Allore H, DeLorenzo M, Hertl J, Galligan D. Optimizing replacement of dairy cows: modeling the effects of diseases. Prev Vet Med. (2003) 61(1):27–43. doi: 10.1016/S0167-5877(03)00158-2
135. Prunier A, Mounier L, Le Neindre P, Leterrier C, Mormede P, Paulmier V, et al. Identifying and monitoring pain in farm animals: a review. Animal. (2013) 7(6):998–1010. doi: 10.1017/S1751731112002406
136. Viñuela-Fernández I, Weary DM, Flecknell PA. Pain. In: Appleby MC, Olsson IAS, Galindo F, editors. Animal Welfare. 3rd ed. Wallingford, UK: CABI (2018). p. 76–91.
137. Eller-Smith OC, Nicol AL, Christianson JA. Potential mechanisms underlying centralized pain and emerging therapeutic interventions. Front Cell Neurosci. (2018) 12:35. doi: 10.3389/fncel.2018.00035
138. Sjörs A. I Feel Terrible! Can you Measure That? Exploring Psychophysiological Stress Responses and Their Interactions with Performance, Subjective Reports and Health status. Linköpink, Sweden: Linköping University (2010).
139. Mellor D, Stafford K, Todd S, Lowe T, Gregory N, Bruce R, et al. A comparison of catecholamine and cortisol responses of young lambs and calves to painful husbandry procedures. Aust Vet J. (2002) 80(4):228–33. doi: 10.1111/j.1751-0813.2002.tb10820.x
140. Stewart M, Verkerk GA, Stafford KJ, Schaefer AL, Webster JR. Noninvasive assessment of autonomic activity for evaluation of pain in calves, using surgical castration as a model. J Dairy Sci. (2010) 93(8):3602–9. doi: 10.3168/jds.2010-3114
141. Lay DC Jr, Friend TH, Grissom KK, Bowers CL, Mal ME. Effects of freeze or hot-iron branding of angus calves on some physiological and behavioral indicators of stress. Appl Anim Behav Sci. (1992) 33(2-3):137–47. doi: 10.1016/S0168-1591(05)80003-6
142. Fitzpatrick JL, Nolan AM, Young FJ, Hogarth C, McDonald T, Weber A, et al. Objective measurement of pain and inflammation in dairy cows with clinical mastitis. 9th ISVEE Symposium (2000). p. 1171–3
143. Ginger L, Ledoux D, Bouchon M, Rautenbach I, Bagnard C, Lurier T, et al. Using behavioral observations in freestalls and at milking to improve pain detection in dairy cows after lipopolysaccharide-induced clinical mastitis. J Dairy Sci. (2023) 106(8):5606–25. doi: 10.3168/jds.2022-22533
144. Kleinhenz MD, Gorden PJ, Smith JS, Schleining JA, Kleinhenz KE, Juarez JR, et al. Effects of transdermal flunixin meglumine on experimentally induced lameness in adult dairy cattle. J Dairy Sci. (2019) 102(7):6418–30. doi: 10.3168/jds.2018-15091
145. Warner R, Kleinhenz MD, Ydstie JA, Schleining JA, Wulf LW, Coetzee JF, et al. Randomized controlled trial comparison of analgesic drugs for control of pain associated with induced lameness in lactating dairy cattle. J Dairy Sci. (2021) 104(2):2040–55. doi: 10.3168/jds.2020-18563
146. Godoy LD, Rossignoli MT, Delfino-Pereira P, Garcia-Cairasco N, de Lima Umeoka EH. A comprehensive overview on stress neurobiology: basic concepts and clinical implications. Front Behav Neurosci. (2018) 12:127. doi: 10.3389/fnbeh.2018.00127
147. Schwartzkopf-Genswein KS, Stookey JM, Welford R. Behavior of cattle during hot-iron and freeze branding and the effects on subsequent handling ease. J Anim Sci. (1997) 75(8):2064–72. doi: 10.2527/1997.7582064x
148. Rodriguez AR, Herzberg DE, Werner MP, Muller HY, Bustamante HA. Plasma concentration of norepinephrine, beta-endorphin, and substance P in lame dairy cows. J Vet Res. (2018) 62(2):193–7. doi: 10.2478/jvetres-2018-0029
149. Bergamasco L, Edwards-Callaway LN, Bello NM, Mijares SH, Cull CA, Rugan S, et al. Unmitigated surgical castration in calves of different ages: cortisol concentrations, heart rate variability, and infrared thermography findings. Animals (Basel). (2021) 11(9):2719. doi: 10.3390/ani11092719
150. Tadich N, Tejeda C, Bastias S, Rosenfeld C, Green LE. Nociceptive threshold, blood constituents and physiological values in 213 cows with locomotion scores ranging from normal to severely lame. Vet J. (2013) 197(2):401–5. doi: 10.1016/j.tvjl.2013.01.029
151. Stewart M, Stafford KJ, Dowling SK, Schaefer AL, Webster JR. Eye temperature and heart rate variability of calves disbudded with or without local anaesthetic. Physiol Behav. (2008) 93(4-5):789–97. doi: 10.1016/j.physbeh.2007.11.044
152. Stewart M, Stookey J, Stafford K, Tucker C, Rogers A, Dowling S, et al. Effects of local anesthetic and a nonsteroidal antiinflammatory drug on pain responses of dairy calves to hot-iron dehorning. J Dairy Sci. (2009) 92(4):1512–9. doi: 10.3168/jds.2008-1578
153. Ren K, Torres R. Role of interleukin-1beta during pain and inflammation. Brain Res Rev. (2009) 60(1):57–64. doi: 10.1016/j.brainresrev.2008.12.020
154. Barragan AA, Pineiro JM, Schuenemann GM, Rajala-Schultz PJ, Sanders DE, Lakritz J, et al. Assessment of daily activity patterns and biomarkers of pain, inflammation, and stress in lactating dairy cows diagnosed with clinical metritis. J Dairy Sci. (2018) 101(9):8248–58. doi: 10.3168/jds.2018-14510
155. Schmitt R, Pieper L, Borchardt S, Swinkels JM, Gelfert CC, Staufenbiel R. Effects of a single transdermal administration of flunixin meglumine in early postpartum Holstein Friesian dairy cows: part 1. Inflammatory and metabolic markers, uterine health, and indicators of pain. J Dairy Sci. (2023) 106(1):624–40. doi: 10.3168/jds.2021-20555
156. Bustamante HA, Rodriguez AR, Herzberg DE, Werner MP. Stress and pain response after oligofructose induced-lameness in dairy heifers. J Vet Sci. (2015) 16(4):405–11. doi: 10.4142/jvs.2015.16.4.405
157. Ilievska K, Atanasov B, Dovenski T, Smolac O, Stojanov B, Trojachanec P. Acute phase proteins–as indicators of claw diseases in dairy cattle. Macedonian Vet Rev. (2019) 42(1):95–100. doi: 10.2478/macvetrev-2019-0011
158. Tschoner T, Feist M. Substance P concentrations in the blood plasma and serum of adult cattle and calves during different painful procedures and conditions—a systematic review. BMC Vet Res. (2022) 18(1):232. doi: 10.1186/s12917-022-03304-6
159. Barragan AA, Bauman LM, Schuenemann GM, Velez J, Lakritz J, Coetzee JF, et al. Administration of acetylsalicylic acid after parturition in lactating dairy cows under certified organic management: part II. Biomarkers of nociception, inflammation, and stress. J Dairy Sci. (2020) 103(12):11713–22. doi: 10.3168/jds.2020-18389
160. Coetzee JF, Lubbers BV, Toerber SE, Gehring R, Thomson DU, White BJ, et al. Plasma concentrations of substance P and cortisol in beef calves after castration or simulated castration. Am J Vet Res. (2008) 69(6):751–62. doi: 10.2460/ajvr.69.6.751
161. Coetzee JF, Mosher RA, KuKanich B, Gehring R, Robert B, Reinbold JB, et al. Pharmacokinetics and effect of intravenous meloxicam in weaned Holstein calves following scoop dehorning without local anesthesia. BMC Vet Res. (2012) 8:1–15. doi: 10.1186/1746-6148-8-153
162. Chen Y, Arsenault R, Napper S, Griebel P. Models and methods to investigate acute stress responses in cattle. Animals (Basel). (2015) 5(4):1268–95. doi: 10.3390/ani5040411
163. Thompson SJ, Bushnell MC. Rodent functional and anatomical imaging of pain. Neurosci Lett. (2012) 520(2):131–9. doi: 10.1016/j.neulet.2012.03.015
164. Siivonen J, Taponen S, Hovinen M, Pastell M, Lensink BJ, Pyörälä S, et al. Impact of acute clinical mastitis on cow behaviour. Appl Anim Behav Sci. (2011) 132(3-4):101–6. doi: 10.1016/j.applanim.2011.04.005
165. Schwartzkopf-Genswein KS, Stookey JM, Crowe TG, Genswein BM. Comparison of image analysis, exertion force, and behavior measurements for use in the assessment of beef cattle responses to hot-iron and freeze branding. J Anim Sci. (1998) 76(4):972–9. doi: 10.2527/1998.764972x
166. Molony V, Kent JE, Robertson IS. Assessment of acute and chronic pain after different methods of castration of calves. Appl Anim Behav Sci. (1995) 46(1-2):33–48. doi: 10.1016/0168-1591(95)00635-4
167. Morisse JP, Cotte JP, Huonnic D. Effect of dehorning on behavior and plasma-cortisol responses in young calves. Appl Anim Behav Sci. (1995) 43(4):239–47. doi: 10.1016/0168-1591(95)00569-E
168. Adcock SJ, Cruz DM, Tucker CB. Behavioral changes in calves 11 days after cautery disbudding: effect of local anesthesia. J Dairy Sci. (2020) 103(9):8518–25. doi: 10.3168/jds.2020-18337
169. Stilwell G, Lima M, Carvalho R, Broom D. Effects of hot-iron disbudding, using regional anaesthesia with and without carprofen, on cortisol and behaviour of calves. Res Vet Sci. (2012) 92(2):338–41. doi: 10.1016/j.rvsc.2011.02.005
170. Faulkner P, Weary D. Reducing pain after dehorning in dairy calves. J Dairy Sci. (2000) 83(9):2037–41. doi: 10.3168/jds.S0022-0302(00)75084-3
171. Heinrich A, Duffield TF, Lissemore KD, Millman ST. The effect of meloxicam on behavior and pain sensitivity of dairy calves following cautery dehorning with a local anesthetic. J Dairy Sci. (2010) 93(6):2450–7. doi: 10.3168/jds.2009-2813
172. Allen K, Coetzee J, Edwards-Callaway L, Glynn H, Dockweiler J, KuKanich B, et al. The effect of timing of oral meloxicam administration on physiological responses in calves after cautery dehorning with local anesthesia. J Dairy Sci. (2013) 96(8):5194–205. doi: 10.3168/jds.2012-6251
173. Stock ML, Millman ST, Barth LA, Van Engen NK, Hsu WH, Wang C, et al. The effects of firocoxib on cautery disbudding pain and stress responses in preweaned dairy calves. J Dairy Sci. (2015) 98(9):6058–69. doi: 10.3168/jds.2014-8877
174. Karlen KJ, Baier FS, Odegard SL, Baumann RM, Coetzee JF, Kehoe SI, et al. Efficacy of oral meloxicam as primary pain mitigation following caustic paste disbudding of three day old Holstein calves. Transl Anim Sci. (2021) 5(2):txz151. doi: 10.1093/tas/txz151
175. Drwencke AM, Adcock SJ, Tucker CB. Wound healing and pain sensitivity following caustic paste disbudding in dairy calves. J Dairy Sci. (2023) 106(9):6375–87. doi: 10.3168/jds.2023-23238
176. Musk GC, Laurence M, Collins T, Tuke J, Hyndman TH. Mechanical nociceptive threshold testing in bos indicus bull calves. Anim Prod Sci. (2016) 57(3):576–82. doi: 10.1071/AN15441
177. Ting ST, Earley B, Veissier I, Gupta S, Crowe MA. Effects of Burdizzo castration on CO2 laser induced thermal nociception of Holstein–Friesian calves of different ages. Appl Anim Behav Sci. (2010) 126(1-2):12–8. doi: 10.1016/j.applanim.2010.05.005
178. Fitzpatrick CE, Chapinal N, Petersson-Wolfe CS, DeVries TJ, Kelton DF, Duffield TF, et al. The effect of meloxicam on pain sensitivity, rumination time, and clinical signs in dairy cows with endotoxin-induced clinical mastitis. J Dairy Sci. (2013) 96(5):2847–56. doi: 10.3168/jds.2012-5855
179. Dyer RM, Neerchal NK, Tasch U, Wu Y, Dyer P, Rajkondawar PG. Objective determination of claw pain and its relationship to limb locomotion score in dairy cattle. J Dairy Sci. (2007) 90(10):4592–602. doi: 10.3168/jds.2007-0006
180. Ley SJ, Waterman AE, Livingston A. Measurement of mechanical thresholds, plasma cortisol and catecholamines in control and lame cattle: a preliminary study. Res Vet Sci. (1996) 61(2):172–3. doi: 10.1016/S0034-5288(96)90096-X
181. Cutler JH, Cramer G, Walter J, Millman S, Kelton D. Randomized clinical trial of tetracycline hydrochloride bandage and paste treatments for resolution of lesions and pain associated with digital dermatitis in dairy cattle. J Dairy Sci. (2013) 96(12):7550–7. doi: 10.3168/jds.2012-6384
182. Williams HJ, Duncan JS, Grove-White DH, Mahen PJ, Gillespie AV. Repeatability and feasibility of pressure algometry for quantifying mechanical nociceptive threshold in the thoracic region of calves. Front Vet Sci. (2020) 7:442. doi: 10.3389/fvets.2020.00442
183. Ohlheiser AL, Ahola JK, Baier FS, Callan RJ, Lear AS, Byers SR, et al. Evaluation of intramuscular injection of oxytetracycline for use as an experimental model to induce pain and assess the efficacy of pain mitigation strategies in dairy cows. Am J Vet Res. (2020) 81(6):471–8. doi: 10.2460/ajvr.81.6.471
184. Theurer ME, Amrine DE, White BJ. Remote noninvasive assessment of pain and health status in cattle. In: Coetzee JF, editor. Veterinary Clinics of North America: Food Animal Practice. 29. 1st ed. Philadelphia, PA: Elsevier (2013). p. 59–74.
185. Whay H. Locomotion scoring and lameness detection in dairy cattle. In Pract. (2002) 24(8):444–9. doi: 10.1136/inpract.24.8.444
186. Sprecher D, Hostetler DE, Kaneene J. A lameness scoring system that uses posture and gait to predict dairy cattle reproductive performance. Theriogenology. (1997) 47(6):1179–87. doi: 10.1016/S0093-691X(97)00098-8
187. Radostits OM, Gay CC, Hinchcliff KW, Constable PD. Diseases of the alimentary tract- I. In: Radostits OM, Gay CC, Hinchcliff KW, Constable PD, editors. Veterinary Medicine: A Textbook of the Diseases of Cattle, Horses, Sheep, Pigs and Goats. 10th ed. Philadelphia: Saunders Elsevier (2007). p. 189–382.
188. McLennan KM. Why pain is still a welfare issue for farm animals, and how facial expression could be the answer. Agri. (2018) 8(8):127. doi: 10.3390/agriculture8080127
189. Hager C, Biernot S, Buettner M, Glage S, Keubler LM, Held N, et al. The sheep grimace scale as an indicator of post-operative distress and pain in laboratory sheep. PLoS One. (2017) 12(4):e0175839. doi: 10.1371/journal.pone.0175839
190. Viscardi AV, Hunniford M, Lawlis P, Leach M, Turner PV. Development of a piglet grimace scale to evaluate piglet pain using facial expressions following castration and tail docking: a pilot study. Front Vet Sci. (2017) 4:51. doi: 10.3389/fvets.2017.00051
191. Navarro E, Mainau E, Manteca X. Development of a facial expression scale using farrowing as a model of pain in sows. Animals. (2020) 10(11):2113. doi: 10.3390/ani10112113
192. Gleerup KB, Andersen PH, Munksgaard L, Forkman B. Pain evaluation in dairy cattle. Appl Anim Behav Sci. (2015) 171(October):25–32. doi: 10.1016/j.applanim.2015.08.023
193. Yamada PH, Codognoto VM, de Ruediger FR, Trindade PH, da Silva KM, Rizzoto G, et al. Pain assessment based on facial expression of bulls during castration. Appl Anim Behav Sci. (2021) 236:105258.
194. Lu Y, Mahmoud M, Robinson P. Estimating sheep pain level using facial action unit detection. 2017 12th IEEE International Conference on Automatic Face & Gesture Recognition (FG 2017) (2017) 30 May-3 June 2017.
195. Sutton GA, Paltiel O, Soffer M, Turner D. Validation of two behaviour-based pain scales for horses with acute colic. Veterinary Journal (London, England: 1997). (2013) 197(3):646–50. doi: 10.1016/j.tvjl.2013.04.007
196. Firth AM, Haldane SL. Development of a scale to evaluate postoperative pain in dogs. J Am Vet Med Assoc. (1999) 214(5):651–9. doi: 10.2460/javma.1999.214.05.651
197. Holton L, Reid J, Scott EM, Pawson P, Nolan A. Development of a behaviour-based scale to measure acute pain in dogs. Vet Rec. (2001) 148(17):525–31. doi: 10.1136/vr.148.17.525
198. Morton CM, Reid J, Scott EM, Holton LL, Nolan AM. Application of a scaling model to establish and validate an interval level pain scale for assessment of acute pain in dogs. Am J Vet Res. (2005) 66(12):2154–66. doi: 10.2460/ajvr.2005.66.2154
199. Testa B, Reid J, Scott ME, Murison PJ, Bell AM. The short form of the glasgow composite measure pain scale in post-operative analgesia studies in dogs: a scoping review. Front Vet Sci. (2021) 8:751949. doi: 10.3389/fvets.2021.751949
200. Brondani JT, Luna SPL, Minto BW, Santos BPR, Beier SL, Matsubara LM, et al. Validity and responsiveness of a multidimensional composite scale to assess postoperative pain in cats. Arq Bras Med Vet Zootec. (2012) 64(6):1529–38. doi: 10.1590/S0102-09352012000600019
201. Reid J, Scott EM, Calvo G, Nolan AM. Definitive Glasgow acute pain scale for cats: validation and intervention level. Vet Rec. (2017) 180(18):449. doi: 10.1136/vr.104208
202. de Oliveira FA, Luna SP, do Amaral JB, Rodrigues KA, Sant'Anna AC, Daolio M, et al. Validation of the UNESP-botucatu unidimensional composite pain scale for assessing postoperative pain in cattle. BMC Vet Res. (2014) 10:200. doi: 10.1186/s12917-014-0200-0
203. Dobromylskyj P. Pain assessment. In: Flecknell PA, Waterman-Pearson A, editors. Pain Management in Animals. Oxford: W.B. Saunders (2000). p. 53–79.
204. Horback KM, Parsons TD. Judgement bias testing in group-housed gestating sows. Behav Processes. (2019) 159:86–92. doi: 10.1016/j.beproc.2018.12.021
205. Neave HW, Daros RR, Costa JH, von Keyserlingk MA, Weary DM. Pain and pessimism: dairy calves exhibit negative judgement bias following hot-iron disbudding. PLoS One. (2013) 8(12):e80556. doi: 10.1371/journal.pone.0080556
206. Lecorps B, Ludwig BR, von Keyserlingk MAG, Weary DM. Pain-Induced pessimism and anhedonia: evidence from a novel probability-based judgment bias test. Front Behav Neurosci. (2019) 13:54. doi: 10.3389/fnbeh.2019.00054
207. Mintline EM, Stewart M, Rogers AR, Cox NR, Verkerk GA, Stookey JM, et al. Play behavior as an indicator of animal welfare: disbudding in dairy calves. Appl Anim Behav Sci. (2013) 144(1-2):22–30. doi: 10.1016/j.applanim.2012.12.008
208. Rushen J, de Passillé AM. Automated measurement of acceleration can detect effects of age, dehorning and weaning on locomotor play of calves. Appl Anim Behav Sci. (2012) 139(3-4):169–74. doi: 10.1016/j.applanim.2012.04.011
209. Winder CB, LeBlanc SJ, Haley DB, Lissemore KD, Godkin MA, Duffield TF. Clinical trial of local anesthetic protocols for acute pain associated with caustic paste disbudding in dairy calves. J Dairy Sci. (2017) 100(8):6429–41. doi: 10.3168/jds.2017-12724
210. Lecorps B, Nogues E, von Keyserlingk MAG, Weary DM. Pessimistic dairy calves are more vulnerable to pain-induced anhedonia. PLoS One. (2020) 15(11):e0242100. doi: 10.1371/journal.pone.0242100
211. Gingerich K, Choulet V, Miller-Cushon E. Disbudding affects use of a shelter provided to group-housed dairy calves. J Dairy Sci. (2020) 103(11):10519–29. doi: 10.3168/jds.2020-18267
212. Craske MG, Meuret AE, Ritz T, Treanor M, Dour HJ. Treatment for anhedonia: a neuroscience driven approach. Depress Anxiety. (2016) 33(10):927–38. doi: 10.1002/da.22490
213. Barkus E. The effects of anhedonia in social context. Curr Behav Neurosci Rep. (2021) 8:77–89. doi: 10.1007/s40473-021-00232-x
214. Ede T, Lecorps B, von Keyserlingk MAG, Weary DM. Calf aversion to hot-iron disbudding. Sci Rep. (2019) 9(1):5344. doi: 10.1038/s41598-019-41798-7
215. Danbury TC, Weeks CA, Chambers JP, Waterman-Pearson AE, Kestin SC. Self-selection of the analgesic drug carprofen by lame broiler chickens. Vet Rec. (2000) 146(11):307–11. doi: 10.1136/vr.146.11.307
216. Colston KPJ, Ede T, Mendl MT, Lecorps B. Cold therapy and pain relief after hot-iron disbudding in dairy calves. PloS One. (2024) 19(7):e0306889. doi: 10.1371/journal.pone.0306889
217. Sneddon LU, Elwood RW, Adamo SA, Leach MC. Defining and assessing animal pain. Anim Behav. (2014) 97:201–12. doi: 10.1016/j.anbehav.2014.09.007
218. Latremoliere A, Woolf CJ. Central sensitization: a generator of pain hypersensitivity by central neural plasticity. J Pain. (2009) 10(9):895–926. doi: 10.1016/j.jpain.2009.06.012
219. Laven RA, Lawrence KE, Weston JF, Dowson KR, Stafford KJ. Assessment of the duration of the pain response associated with lameness in dairy cows, and the influence of treatment. N Z Vet J. (2008) 56(5):210–7. doi: 10.1080/00480169.2008.36835
220. Adcock SJJ, Tucker CB. Painful procedures: when and what should we be measuring in cattle? In: Tucker CB, editor. Advances in Cattle Welfare. Duxford, UK: Woodhead Publishing (2018):157–98. doi: 10.1016/B978-0-08-100938-3.00008-5
221. Dickens C, McGowan L, Clark-Carter D, Creed F. Depression in rheumatoid arthritis: a systematic review of the literature with meta-analysis. Psychosom Med. (2002) 64(1):52–60. doi: 10.1097/00006842-200201000-00008
222. McWilliams LA, Goodwin RD, Cox BJ. Depression and anxiety associated with three pain conditions: results from a nationally representative sample. Pain. (2004) 111(1-2):77–83. doi: 10.1016/j.pain.2004.06.002
223. Adcock SJJ. Early life painful procedures: long-term consequences and implications for farm animal welfare. Front Anim Sci. (2021) 2:759522. doi: 10.3389/fanim.2021.759522
224. Holden SA, Butler ST. Applications and benefits of sexed semen in dairy and beef herds. Animal. (2018) 12(s1):s97–s103. doi: 10.1017/S1751731118000721
225. Needham T, Lambrechts H, Hoffman L. Castration of male livestock and the potential of immunocastration to improve animal welfare and production traits: invited review. S Afr J Anim Sci. (2017) 47(6):731–42. doi: 10.4314/sajas.v47i6.1
226. Spurlock D, Stock M, Coetzee J. The impact of 3 strategies for incorporating polled genetics into a dairy cattle breeding program on the overall herd genetic merit. J Dairy Sci. (2014) 97(8):5265–74. doi: 10.3168/jds.2013-7746
227. National Dairy FARM Program (Farmers Assuring Responsible Management). FARM Animal Care Evaluation Preparation Guide Version 4. (2020). Available online at: https://nationaldairyfarm.com/wp-content/uploads/2021/11/FARM_Prep-Guide_Animal-Care-Evaluation_1021_FINAL_HR.pdf (Accessed March 4, 2024).
228. Schnaider MA, Heidemann MS, Silva AHP, Taconeli CA, Molento CFM. Vocalization and other behaviors indicating pain in beef calves during the ear tagging procedure. J Vet Behav. (2022) 47:93–8. doi: 10.1016/j.jveb.2021.10.005
229. Palmer CW. Welfare aspects of theriogenology: investigating alternatives to electroejaculation of bulls. Theriogenology. (2005) 64(3):469–79. doi: 10.1016/j.theriogenology.2005.05.032
Keywords: analgesia, pain, cow, cattle, animal welfare, pain management
Citation: Zoltick AH, Mann S and Coetzee JF (2024) Pain pathophysiology and pharmacology of cattle: how improved understanding can enhance pain prevention, mitigation, and welfare. Front. Pain Res. 5:1396992. doi: 10.3389/fpain.2024.1396992
Received: 6 March 2024; Accepted: 29 July 2024;
Published: 27 August 2024.
Edited by:
Daniel Mota-Rojas, Metropolitan Autonomous University, MexicoReviewed by:
Marcelo Ghezzi, Universidad Nacional del Centro de Buenos Aires, ArgentinaAdriana Domínguez-Oliva, Metropolitan Autonomous University, Mexico
Pamela Lendez, Universidad Nacional del Centro de Buenos Aires, Argentina
Copyright: © 2024 Zoltick, Mann and Coetzee. This is an open-access article distributed under the terms of the Creative Commons Attribution License (CC BY). The use, distribution or reproduction in other forums is permitted, provided the original author(s) and the copyright owner(s) are credited and that the original publication in this journal is cited, in accordance with accepted academic practice. No use, distribution or reproduction is permitted which does not comply with these terms.
*Correspondence: Abigale H. Zoltick, YXpvbHRpY2tAdmV0LnVwZW5uLmVkdQ==