- 1Lucine, Bordeaux, France
- 2Faculté de Médecine et des Sciences de la Santé, Centre de Recherche Clinique du Centre Hospitalier Universitaire de Sherbrooke, Université de Sherbrooke, Sherbrooke, QC, Canada
For the past two decades, using Digital Therapeutics (DTx) to counter painful symptoms has emerged as a novel pain relief strategy. Several studies report that DTx significantly diminish pain while compensating for the limitations of pharmacological analgesics (e.g., addiction, side effects). Virtual reality (VR) is a major component of the most effective DTx for pain reduction. Notably, various stimuli (e.g., auditory, visual) appear to be frequently associated with VR in DTx. This review aims to compare the hypoalgesic power of specific stimuli with or without a VR environment. First, this review will briefly describe VR technology and known elements related to its hypoalgesic effect. Second, it will non-exhaustively list various stimuli known to have a hypoalgesic effect on pain independent of the immersive environment. Finally, this review will focus on studies that investigate a possible potentialized effect on pain reduction of these stimuli in a VR environment.
1. Virtual reality
Many virtual reality (VR) definitions have been proposed in the past few decades, from short straightforward to more complex explanations. Honzel et al. elegantly summarized it as follows: an immersive computer-generated environment designed to be perceived as real by the user (56). Meanwhile, Digital Therapeutics (DTx) has been defined as an “evidence-based therapeutic interventions that are driven by high-quality software to treat, manage, or prevent a disease or disorder […] used independently or in concert with medications, devices, or other therapies to optimize patient care and health outcomes” (138). Interestingly, DTx benefit from VR technologies in the healthcare system (21), particularly since the COVID-19 pandemic, which led to a more digitalized model (22). Thus, VR has been increasingly studied, notably in acute or chronic pain analgesia situations (128).
The hypoalgesic power of VR has been extensively highlighted in recent meta-analysis and reviews not only in the adult population (1, 9, 16, 17, 25, 47, 72, 76, 78, 102) but also in pediatric patients (28). In addition, benefits affecting several modalities of quality of life have been reported (e.g., stress, anxiety), suggesting VR as a good non-pharmacological therapeutic tool (95, 76, 150). The goal of this review is to verify if the addition of different auditory and visual stimulations frequencies [e.g., binaural beats (BBs), hypnosis] have additive effects on VR efficacy. The first part of this review will exclusively focus on pain studies that investigated the hypoalgesic effects of VR in acute or chronic pain conditions, followed by the physiological evidence supporting this effect. The second part will non-exhaustively list several sensory stimuli used to promote analgesia as stand-alone treatments. Finally, the third part of this review will aim to investigate studies combining one or many of the sensory stimuli previously described in a VR environment (Table 1).
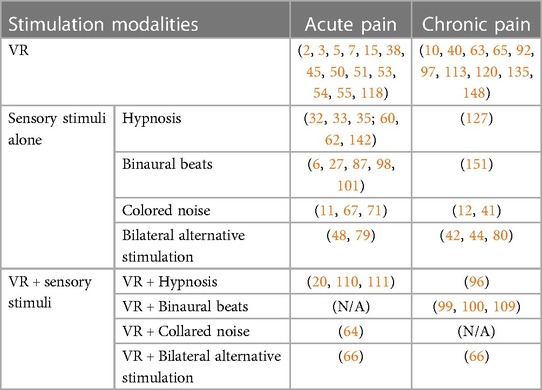
Table 1. List of the articles that refer to the different stimulations with and without VR for acute and chronic pain.
1.1. Analgesic power of virtual reality
1.1.1. Acute pain
Pain is a perception mechanism aiming to alert the organism of nociceptive stimuli potentially compromising its survival. Since 2020, its definition has been revised by the International Association for the Study of Pain (IASP) as follows: “An unpleasant sensory and emotional experience associated with, or resembling that associated with, actual or potential tissue damage” (106).
To our knowledge, the first evidence of VR analgesia on acute pain came from the work of Hoffman et al. in the early 2000s. First, they reported a decrease of the perceived pain following a VR session in two adolescent patients during burn wound care (Figure 1) (50). Second, using magnetic resonance imaging (MRI), they highlighted that VR effectively lowered brain activity in areas related to pain (i.e., anterior cingulate cortex, primary and secondary cortex, insula, and thalamus) in 14 healthy participants (51). Third, they reported the importance of choosing a good quality VR headset to improve the efficiency of the device (52). Fourth, they have shown that VR significantly reduces pain compared to opioids, with a potentialized effect when both are being coupled (53). Following these results, they continued to provide significant evidence concerning the hypoalgesic power of VR through pain measurements and cerebral imaging, mainly in burn victims or children receiving painful procedures (2, 3, 5, 7, 54, 55). Following the release of these pioneer studies performed by Hoffman et al., many teams have now shown a hypoalgesic effect of VR in acute pain situations, such as venipuncture, lumbar puncture, women during labor, or dental surgery (15, 45, 38, 118).
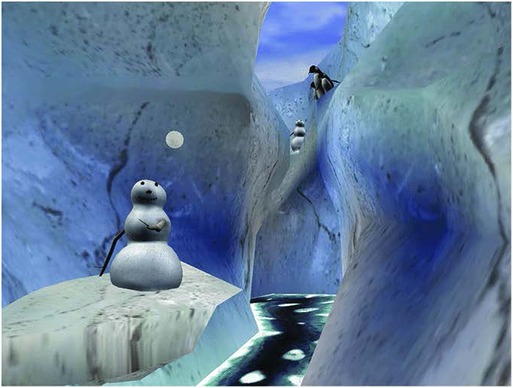
Figure 1. SnowWorld, a 3D virtual reality environment designed in the early 2000s for analgesia purposes in burnt victims [adapted from Honzel et al. (56)].
In accordance with these results, recent reviews are increasingly reporting the benefits of VR for acute pain analgesia (1, 25, 47, 58, 72, 76, 78, 102). For instance, it has been shown that VR is able to significantly increase thermal pain tolerance (46) or significantly decrease experimental pain intensity using electrical- and thermal-induced stimuli (119). Moreover, it has been highlighted that three sessions a day of 30 min of VR is also efficient to reduce pain during a rehabilitation protocol after knee surgery (63). Interestingly, the addition of VR with local anesthesia in patients undergoing dental surgery can significantly reduce oxygen saturation, intraoperative pulse rate, and postoperative visual analog pain scale results (126).
However, it is important to notice that some studies did not find significant results regarding a hypoalgesic effect of VR on some acute pain stimulation. Indeed, Walker et al. investigated the efficacy of VR distraction during a cystoscopy, without a significant decrease of pain questionnaire scores between the VR and control groups (145). The authors suggested that these results could likely be due to a lack of immersion, which is the main mechanism of action during a VR experience (see Section 1.2). In addition, Smith et al. tested the pressure pain during various contextual conditions and did not show statistical differences on participants’ pain thresholds (121). However, the authors mentioned that they investigated pain sensitivity instead of pain intensity, which could explain their results. Importantly, these disparate results also highlight the difficulty for VR studies to properly choose the best methods (e.g., protocol of administration, VR apparatus), thus leading to difficulties to compare the results between studies.
Interestingly, the VR hypoalgesic effects observed on acute pain are not limited to the duration of the application. It has been reported that this effect can last up to 1 h post-VR application following either a cardiac surgical or an episiotomy repair procedure (59, 86). Further investigations are required to evaluate this lasting hypoalgesic effect of VR on acute pain (Table 1).
1.1.2. Chronic pain
Chronic pain can be defined as a persistent or recurrent pain, lasting for at least 3 months (136, 137). Chronic pain can lead to critical dysfunctions in both peripheral and central nervous systems, such as gray and white matter loss, increase or decrease of the activity in major cerebral areas, or alterations of synaptic neurotransmission (124, 108). In addition, chronic pain may severely affect the quality of life of patients living with it, from nutrition and physical activity to sleep disorders and mental wellbeing (91, 85). Importantly, chronic pain can appear through aging and in patients presenting specific diseases (77).
While current results are converging toward the efficacy of VR to diminish acute pain, reviews are also looking to the potential hypoalgesic power of VR on chronic pain (47, 78, 102). Interestingly, major significant VR benefits on pain ratings or pain relief have been reported in various chronic conditions, such as musculoskeletal pain, neuropathic pain, cervical/thoracic/lumbar spine pain, hip pain, pervasive pain, or interstitial cystitis (63, 65).
A study on six fibromyalgia patients has shown that pain reduction was significantly greater in the VR group compared to the control group (10). Another study has highlighted that VR immersion can significantly reduce pain perceived in patients living with chronic migraines in a hospital waiting room (135). Similarly, VR benefits have been found in children with chronic headaches (120). Another one has shown a major decrease of pain intensity in patients suffering from chronic pain (148). Also, an improvement of pain intensity, frequency, duration, and intrusion in patients living with phantom limb pain after 12 VR sessions has been reported (92). In addition, pain intensity was significantly decreased after a 6-week VR session in patients with subacromial impingement syndrome (97). Recently, a 56-day study has highlighted a significant decrease of pain intensity in patients living with chronic low back pain (40).
The duration of the hypoalgesic effect of VR on chronic pain varies across the studies (78). The benefits have been demonstrated to last up to (i) 1 month (97); (ii) 1 to 3 months (120, 135); (iii) 1, 3, and 6 months (92); or even (iv) 6 months (10) post-treatment, potentially suggesting a long-term efficacy of VR on chronic pain. Finally, it is important to notice that some studies did not find significant results regarding the hypoalgesic effects of VR, or at least sustainable ones (113), on unspecified chronic pain conditions (63, 148).
In conclusion, ample evidence attests to the many benefits of VR on acute and chronic pain analgesia, while some pointed out the lack of an effect. Nevertheless, the study of this technology in these pain conditions is still under investigation in various pathologies, allowing us to better understand the limits of its hypoalgesic power (Table 1).
1.2. Physiological evidence supporting the analgesic efficacy of VR
The exact mechanisms through which VR is procuring the hypoalgesic effects previously mentioned on acute and chronic pain are still unclear, although it seems that the main one is immersion (18, 74). This concept needs to be differentiated from distraction. Indeed, it has recently been shown that immersive VR significantly increases heat-pain tolerance limits, as well as improves mood, situation anxiety, and pain unpleasantness, while a distraction control only increased the pain tolerance limits without affecting the other modalities (18). In addition, the authors highlighted that the increase of the heat-pain tolerance limits by VR was related to an increase of sympathetic and parasympathetic responses (e.g., heart rate variability standard deviation from normal to normal, galvanic skin responses). Interestingly, it has also been shown that VR cues related to “virtual water temperature” (i.e., color red for hot and blue for cold) can significantly influence the pain perception of thermal stimuli (68). Using the same nociceptive thermal stimulation, a virtual hot water signal was perceived as significantly more painful than a virtual cold signal, likely through top-down endogenous mechanisms.
This hypoalgesic ability of VR leads us to compare it to traditional medication. To date, the most common analgesics prescribed for pain are opioids (89). Notably, repetitive opioid use can lead to an increase of postoperative acute pain episodes (37) as well as major aversive effects, such as addiction or overdose death (112). Thus, the current worldwide opioid crisis has led to an urgency to find new non-pharmacological hypoalgesic solutions, in which VR appears to be effective (132). As previously mentioned, VR can be as efficient as opioids to reduce pain, with a potentialized efficiency when used adjunctively (53). In addition, VR can effectively reduce opioid administration during painful wound care procedures (82). Thus, the current emergence of various DTx using VR is very interesting in terms of novel hypoalgesic strategies.
One of the main counter-indications of VR is the adverse effect known as “cybersickness,” mainly causing nausea symptoms in VR users (70, 81, 144, 146). It seems that this motion sickness could be due to a conflict between the sensory stimuli or autonomic responses (e.g., visual system, vestibular system) (70). Interestingly, a recent review highlighted a close relationship between cybersickness and the feeling of presence in a VR environment: the more the cybersickness experienced, the less immersed the VR user will be (146), which could prevent any hypoalgesic effects of DTx using VR.
Several sensory (i.e., auditory, visual, olfactory, gustatory, tactile) stimuli can be transmitted to users through a VR apparatus in order to increase immersion within the virtual environment (1, 68), allowing the transmission of various stimulations [e.g., hypnosis, binaural beats, colored noise, bilateral alternative stimulation (BAS)], thus potentially being able to increase the VR hypoalgesic power.
2. Sensory stimuli
Among the various sensory stimuli that DTx may use to induce a hypoalgesic effect, VR technologies for pain treatment frequently include therapeutic scripts as well as sound and light frequencies in the virtual environment (83). It is difficult to separate the efficacy of VR from the effects of some visual or auditory stimuli since they are presented together in most of the studies. We will introduce a non-exhaustive description of psychological (i.e., hypnosis), auditory (i.e., binaural beats, colored noise), and visual (i.e., bilateral alternative stimulation) stimuli that are the most used in VR, but can be used as a stand-alone treatment (Table 1).
2.1. Hypnosis
The definition of hypnosis has frequently evolved throughout the years, mainly to increase its comprehension, in order to allow its use, notably, in scientific studies (29). One of the more recent is the following: “A state of consciousness involving focused attention and reduced peripheral awareness characterized by an enhanced capacity for response to suggestions” (29). Several clinical studies have highlighted the benefits of hypnosis in several situations (e.g., pain, depression, motor paralysis, phobia) (8, 103, 105, 129, 142).
Analgesia through hypnosis has majorly been investigated during surgeries for “hypnosedation” (35, 69, 142). A pioneer retrospective study has shown that adding hypnosedation to a conscious intravenous sedation coupled with a local anesthesia improves both perioperative pain and anxiety relief during various plastic surgery (e.g., breast augmentation, correction of mammary ptosis, liposuction) compared to medical sedation alone (32). These results were confirmed a few years later during a prospective study (33). More recently, a critical review of nearly 30 randomized controlled clinical trials (RCTs) highlighted that hypnosis was consistently able to decrease pain in acute painful conditions compared to standard care and attention control groups (69). The authors also mentioned that hypnosis was at least as efficient to comparable adjunct psychological or behavioral therapies in the same context. Importantly, it has been shown that hypnosis was able to significantly reduce pain intensity in patients suffering from chronic pain following a spinal cord injury, compared to non-invasive electrical stimulation (60, 62). Hypnosis has been reported to more effectively reduce the pain intensity in chronic low back pain participants using self-hypnosis training compared to biofeedback (127). Finally, a recent review and meta-analysis reported that hypnosis was moderately able to manage pain in musculoskeletal and neuropathic pain patients, suggesting that deeper investigations were necessary to conclude on the hypoalgesic power of hypnosis on chronic pain conditions (73).
In addition to its hypoalgesic power, hypnosis can effectively impact the patient's quality of life, as well as multiple medical spheres (e.g., pain, medication consumption, physiological parameters, recovery and professional activity restart latency, emotional distress) (8, 61, 73, 110, 111, 125, 129, 134, 142), suggesting a cerebral effect across various brain areas. Importantly, it has been reported that hypnosedation was able to reduce both the affective and sensory components of pain (i.e., unpleasantness, intensity) compared to control conditions (33, 34, 36, 103, 105). Several studies focusing on brain electrical signals have highlighted changes in brain activity due to hypnosis-induced states (60, 62, 104, 149). Functional MRI (fMRI), positron emission tomography (PET), and laser-evoked potential (LEP) studies reported various changes in functional brain connectivity in participants during ongoing hypnosis (8). Interestingly, these changes mainly occurred in brain areas related to consciousness (e.g., anterior and posterior cingulate cortex, medial frontal cortex, precuneus) (8, 61, 140). Another study using hypnosis suggested that experimental pain will become less unpleasant even if the intensity stayed the same and was able to demonstrate the possibility to reduce brain imaging activity in regions related to affective components of pain (e.g., cingulate cortex and insula) without affecting the sensory-discriminative regions (e.g., somatosensory cortices) (103).
However, we must notice that there are some limits surrounding the clinical use of hypnosis. First, protocols using hypnosis frequently take a long time to administer to participants, and measurements are often limited to solely behavioral responses, without considering the subjective experience of the participants, especially to assess their hypnotizability (61, 141). This limit can lead to another constraint, which is that the number of participants often quite small (141, 143). In addition, methods can be subject to limitations, as some variations can occur across most studies, notably on the number of hypnosis sessions and intervention length or timing (especially regarding the induction phase), thus complicating interpretations and comparisons between the studies of this field (69). Although hypoalgesic benefits previously reported are promising, further studies will help expand these results in various acute and chronic pain conditions (Table 1).
2.2. Binaural beats
BBs can be defined as a perceived third frequency resulting from the difference between two different frequencies applied in each ear (e.g., a frequency of 253 Hz in the right ear and a 250 Hz one in the left ear will result in a third frequency of 3 Hz perceived in the brain) (19). Interestingly, it has been shown that this auditory stimulus can elicit an evoked synchronous response reproducing the same frequency and waveform of the stimulus entering the central auditory pathway as a brain oscillation (19). Thus, five different types of BBs have been listed, depending on their frequency: delta (i.e., 0.1–4 Hz), theta (i.e., 4–8 Hz), alpha (i.e., 8–13 Hz), beta (i.e., 13–30 Hz), and gamma (i.e., >30 Hz). To our knowledge, only theta- and alpha-BBs have been reported being able to elicit a hypoalgesic effect.
Theta-BB (i.e., 4–8 Hz) can significantly decrease the severity of perceived chronic pain compared to neutral sound (151). In this study, 36 patients suffering from different types of chronic pain had to listen to a 6 Hz BB tone for 20 min during 14 successive days, while a sham intervention group listened to a non-BB tone of 300 Hz. The results indicated reduced perceived pain severity exclusively in the theta-BB group. Although the pain scores were reduced in both groups, the authors observed a 77% larger reduction of the mean pain scores in favor of the theta-BB group (151).
Concerning alpha rhythms (i.e., 8–13 Hz), several studies indicate that a global suppression of alpha oscillations in somatosensory, motor, and visual areas are observed in response to both transient and tonic painful stimuli (98, 101). In some studies, pain intensity ratings were correlated with a decrease in alpha power (6, 87). More recently, a study has shown that listening to alpha-BB for 10 min significantly decreased the ratings of experimentally induced pain, compared to a control group (27). The authors also discovered that this effect was maximized for 10 Hz frequencies, compared to 8 and 12 Hz. However, the same study found no statistically significant differences with the control group for several aspects of the quality of life (e.g., anxiety, wellbeing, drowsiness), although these results contradict another study that found that alpha rhythm stimulation reduces both stress and anxiety (13). Interestingly, they also noted an improvement in heart rate variability via parasympathetic reinforcement, highlighting the ability of BBs to act on physiological variables as well. Thus, by reducing stress, anxiety, and physiological parameters, alpha rhythm stimulation could potentially decrease pain perception. In addition to their involvement in pain, a recent study pointed out the link between alpha rhythm and memory, by showing that listening to alpha-BB for 15 min can enhance memory recall (88).
However, it is important to notice that we found three studies that failed to promote brain oscillations following theta-, alpha-, beta-, or gamma-BBs, listening for durations of (i) 2 min (43), (ii) 3 min (75), or (iii) 5 min (39). Based on these findings, more studies are needed to investigate the potential hypoalgesic power of all types of BB in order to choose protocols adequately for brain oscillation-induced states (Table 1).
2.3. Colored noise
The “color” of a noise is a terminology used to classify different noises according to their power spectrum density (i.e., frequency of a sound), similar to light waves classification (26). For instance, if we decide to draw the sound wave diagram for “pink noise” by transposing it into a diagram of light waves, it would correspond to a pink light. As such, warm colors are assigned to low-frequency sounds, while cold colors are related to high-frequency sounds. To our knowledge, only white and pink noises have been related to pain analgesia studies (Table 1).
White noise has been extensively studied on sleep, mainly in infants and children (122), as well as intensive care unit patients (31, 123), although, at high intensity, it has been revealed in rodents that white noises can be harmful to the organism, creating anxiety-like behaviors as well as inducing apoptosis, chromatolysis, cytoplasmic organelle destruction, and glial activation brain structures (153). Concerning studies on pain, a team compared the effect of an MRI scanner noise to white noises on the sensory-discriminative (i.e., intensity, localization) and the motivational-affective (i.e., unpleasantness) components of pain (11). They showed that both the MRI scanner noise and white noises significantly reduced unpleasantness ratings, whereas the ability to locate pain was not significantly affected. Interestingly, they assume that, by acting solely on the motivational-affective component of pain, without affecting the sensory-discriminative one, noises may have therapeutic implications by diminishing the distress associated with the pain unpleasantness, while maintaining the capacity to localize the pain to avoid further injury. Another study demonstrated that the pain score was lowered with white noises in newborns (i.e., 38–42 weeks old) during an acute painful procedure (i.e., blood draw), compared to control conditions (67). Similar results were discovered to relieve procedural pain caused by vaccination in premature infants compared to a control group (71). Unfortunately, the impact of white noise in adults has mostly been studied regarding higher cognitive functions, such as semantic priming (4) or recognition memory tasks (107), and not pain.
Pink noise has been studied extensively in relation to sleep (12, 90, 93, 94, 152). A study recently investigated the effects of pink noise on pain in a rat model of chronic pain (12). They measured mechanical allodynia responses before and after exposure to pink noise and observed a statistically significant decrease in behavioral pain response in rats exposed to pink noise. It is important to notice that pink noise has also been used as a control stimulation, compared to music, in a study aiming to investigate the hypoalgesic effect of music on fibromyalgia pain (41). The results indicated a reduction in pain and an increased functional mobility in the music group, whereas there was no change in the pink noise control group. Consecutively, it is difficult to conclude the hypoalgesic power of pink noises.
To our knowledge, no scientific data have been published in order to highlight the potential hypoalgesic power on acute or chronic pain for other types of noise, including red, black, gray, green, blue, and purple noise (26). Concerning brown noise, we found only one study that has been published with stimulation close enough to brown noise, but relative to consciousness and not pain (115). They showed high stability of a Ganzfeld-induced state (i.e., altered state of consciousness through visual and auditory perceptual field homogenization following an unstructured sensory input), white noise displaying the highest overall global scores. Interestingly, the authors suggest that, based on their results, white noise could be very effective to homogenize the auditory field while ignoring potential environment distraction (115).
2.4. Bilateral alternative stimulation
Bilateral alternative stimulation (BAS) is a visual technique mainly applied during a psychotherapeutic approach called eye movement desensitization and reprocessing (EMDR) for post-traumatic stress disorder (PTSD) treatment (139, 147), although growing evidence tends to highlight possible applications of EMDR to relieve pain in patients living with acute or chronic painful conditions (44, 80, 130, 131, 42, 114).
We found a recent review of two RCTs related to acute pain and EMDR (114). The first one found that one session of EMDR was efficient to diminish acute experimental pain intensity (i.e., cold pain pressor), as well as to improve the pain threshold, bettering pain tolerance and reducing anxiety (48). The second one highlighted that one 60-min session 2 h post-abdominal surgery in an emergency service effectively decreased pain intensity (79).
A systematic review reported that EMDR has been efficient in decreasing pain intensity in several studies with patients suffering from various chronic pain conditions (e.g., phantom limb pain, headache, musculoskeletal pain, fibromyalgia) (130). An RCT pilot study also highlighted that EMDR effectively diminished pain intensity, sometimes for up to 6 months, in patients suffering from non-specific chronic back pain after 10 sessions of EMDR (42). In addition, nine weekly sessions of 1 h of EMDR can effectively decrease pain levels and their affect, as well as increase pain control in patients suffering from chronic pain for at least 6 months (44). In 38 patients living with chronic pain, it has been shown that 12 weekly EMDR sessions of 90 min were able to significantly decrease the amount of medication needed and to improve the quality of life (e.g., pain, physical activity, vitality, social interaction, emotional management) (80).
Little is known concerning the possible mechanisms through which EMDR might diminish pain. Pioneer EMDR protocols from Shapiro highlight eight phases to optimize the efficacy of the technique: (i) history and treatment plan, (ii) introduction to EMDR protocol and development of coping strategies, (iii) evaluation of the treatment targets, (iv) desensitization and reprocessing, (v) incorporation of positive cognitions, (vi) body scan (and the reprocessing of any remaining negative bodily sensations), (vii) relaxation (i.e., re-establishing emotional stability if distress has been experienced, and for use between sessions), and, finally, (viii) re-evaluation (116, 117, 114, 139). Interestingly, Grant and Threlfo specified that to facilitate relaxation and to change pain sensations, EMDR was usually accompanied by suggestions to ask the patient to shift their attention from the pain to the BAS (44). An fMRI study reported that BAS can either increase or decrease the activation of limbic structures (i.e., right amygdala, left dorsolateral prefrontal), thus highlighting the effect of EMDR on emotion processing in healthy participants (49). Interestingly, it seems that visual BAS are more effective than auditive BAS on memory tasks (57).
Finally, it is important to note that, despite an increased amount of evidence showing the benefits of EMDR on pain, studies usually do not use an active control group to address the results, and their monocentric design leads to small sample sizes (131). These results thus need to be extended in future investigations to confirm the potential hypoalgesic power of BAS (Table 1).
3. Sensory stimuli associated with virtual reality
In this review, we previously highlighted how VR or isolated sensory stimuli (i.e., hypnosis, binaural beats, colored noise, bilateral alternative stimulation) can elicit a hypoalgesic effect. This section aims to investigate the hypoalgesic power of combining these stimuli in a VR environment (Table 1).
3.1. Virtual reality and hypnosis
As hypnosis has been used for many decades, since the 21st century, its use in a 3D environment following the emergence of VR was rapidly tested in the early 2000s (78, 110, 111, 134). In 2010, Patterson et al. investigated how a hypnotic induction and hypoalgesic suggestions delivered by a customized VR hardware/software would be able to assess analgesia in patients with physical trauma at the hospital (96). They showed that pain intensity ratings, as well as pain unpleasantness, were significantly lowered in the group with VR and hypnosis compared to the groups with only VR or standard care alone, up to 8 hours post-treatment. Interestingly, a recent review and meta-analysis on hypnosis suggests that VR could potentiate the efficacy of hypnosis, especially in low hypnotic suggestibility patients (73). This observation is partially based on an RCT that highlighted that hypnosis with 3D VR animation can improve the user's mood, as well as reduce both the tiredness and the level of cortisol, measured through a salivary test (133). Meanwhile, it has recently been shown that hypnosis added with VR can effectively reduce pain, as well as anxiety and fatigue, in patients undergoing cardiac surgery (110, 111). However, it is important to notice that a study recently showed that human care was preferable to hypnosis through VR in patients undergoing electrophysiology and pacing procedures to improve their comfort (20).
Nevertheless, authors have highlighted some limits surrounding VR hypnosis induction, notably in case-series designs, such as an absence of a randomized distribution and a control group as well as possibly a small sample size (30). The methods can also be affected, since choosing the proper amount of VR sessions to induce hypnosis can often be limited across studies (110, 111). Further studies are needed to clarify all the possibilities of VR hypnotic induction on pain analgesia, but recent findings tend to encourage its use for alleviating patient's pain (14) (Table 1).
3.2. Virtual reality and binaural beats
To our knowledge, only a few studies recently investigated the potentializing effect of BB through VR (Table 1). In 2019, Perales et al. reported that some BBs (i.e., delta, theta, alpha) coupled with a VR environment can act on the sympathetic nervous system modalities (e.g., electrodermal activity) in healthy participants, in addition to other physiological parameters (e.g., temperature, heart rate) for children living with chronic pain (99, 100). The authors mention that these changes could introduce the user into an effective relaxation mood, potentially leading to an improvement in the perception of pain. More recently, they confirmed these results by showing a potentialized effect of VR with BB for chronic pain in children, but surprisingly not on the physiological modalities (i.e., heart rate, galvanic skin response), possibly due to study design limitations (109). Interestingly, it has recently been highlighted that BB in a VR environment can also drastically decrease the main aversive event of VR use, cybersickness, suggesting a potentially better efficacy of DTx using VR and BB (23).
3.3. Virtual reality and colored noise
As mentioned above, VR greatly benefits from immersion to generate its efficacy (18, 74). To our knowledge, studies that specifically investigated a potentialized effect of colored noise on VR analgesia are quite rare (Table 1), although we found one study that showed that adding sounds to a VR game can significantly increase pain tolerance for experimental thermal pain compared to the sounds or the VR game separately (64). However, the authors specified that “sounds” cited referred to the game's music, thus preventing us from concluding the specific hypoalgesic effect of white or pink noise when incorporated into a VR environment. Interestingly, it has recently been shown that shifting a music volume to the same frequency (i.e., 0.1 Hz) as the VR environment motion does not influence the body sway assessed by position measurements, suggesting a lack of effect of colored noise on cybersickness (24).
3.4. Virtual reality and bilateral alternative stimulation
To our knowledge, only one study has investigated more specifically the effect of transmitting EMDR techniques through a VR environment (Table 1). Kaminska et al. reported in 2020 that BAS in VR can significantly reduce the acute stress level as well as mood improvements in healthy adult volunteers, leading to be considered a great tool when added to a relaxation training program (66). Even if it is difficult to conclude with only one study, it seems that BAS through VR could benefit analgesia.
4. Conclusion
In this review, we highlighted (i) the hypoalgesic power of VR only, (ii) the hypoalgesic power of various sensory stimuli only (i.e., hypnosis, binaural beats, colored noise, bilateral alternative stimulation), and (iii) the potentialized hypoalgesic power of these sensory stimuli in a VR environment. In the first part, we have summarized many studies that showed with self-reported scales scores and cerebral imaging that VR can effectively reduce pain perception, both in healthy participants and during acute and chronic pain conditions, likely through the immersive capacity of VR. In addition, we found that the hypoalgesic effect of VR is sometimes as powerful as strong pharmacological analgesics (i.e., opioids). In the second part, we highlighted (i) how hypnosis can elicit an hypoalgesic effect as well as an improvement of the quality of life of participants, (ii) that some BB (i.e., theta, alpha) can effectively produce an hypoalgesic effect, likely by acting on cerebral oscillations, (iii) the low but existing hypoalgesic power of some colored noise (i.e., white, pink), and (iv) how an EMDR technique (i.e., BAS) may both decrease pain and improve the quality of life of some patients suffering from acute or chronic pain. In the final part, we reported the short but emerging scientific literature investigating the potentialized hypoalgesic effect of combining previous sensory stimuli evoked with a VR environment.
A potential limit to our review is the difficulty to compare all these modalities (i.e., VR only, stimuli only, VR and stimuli) as the methods are different across studies, even in the same fields of research. Moreover, the small sample size and the lack of information on the effect size in several studies are limiting a final conclusion on the clinical relevance of these studies. Another limit could be the non-exhaustivity of the sensory stimuli chosen in this review. Further reviews should investigate the hypoalgesic power of a plethora of other sensory stimuli (e.g., odors, textures, biofeedback) or cognitive approaches (e.g., cardiac coherence, mindfulness breathing) and their probable potentialized effect while being coupled with VR technologies. The fact that some studies report a hypoalgesic effect of the VR session outlasting hours and even months may be explainable by several mechanisms. For instance, the activation of endogenous pain modulation may outlast the effect by minutes or even hours. However, the longer effects may be explained by some life habit changes such as moving more freely and more frequently after the positive effect of VR. More studies are needed to characterize the different variables that may contribute to the long-term effect of VR.
We recently published an RCT (NCT04650516) where we highlighted that a VR treatment comprised of some sensory stimuli mentioned above (e.g., BB, BAS) effectively diminished the mean pain intensity in 45 patients diagnosed with moderate-to-severe endometriosis-related chronic pelvic pain, up to 4 h post-treatment, as well as reducing the mean perceived pain, compared to a 2D digital control (84). These results encourage us to conclude that VR with added sensory stimuli can be a good addition to an arsenal for alleviating pain. However, since the control was with the same 2D environment without the additional stimuli, we can only conclude the potential effects of the combination of these stimuli. Future studies are needed to better characterize the potentializing effect of adding BB, BAS, EMDR, or different sound frequencies on the hypoalgesic effect of VR.
In conclusion, our review suggests that adding sensory stimuli to VR can be a great opportunity for a plethora of DTx in order to alleviate patients from painful symptoms. It suggests that we can increase the efficacy of DTx analgesia with the addition of different sensory stimuli combined with VR.
Author contributions
MF, JV, and SM wrote the paper. MF, JV, CA, FA, VE, CL, and SM reviewed the paper. All authors contributed to the article and approved the submitted version.
Funding
This work was funded, in part, by SM research grants from the Natural Sciences and Engineering Research Council of Canada (NSERC) Discovery Grant (grant number: 323767).
Conflict of interest
The authors declare that the research was conducted in the absence of any commercial or financial relationships that could be construed as a potential conflict of interest.
Publisher's note
All claims expressed in this article are solely those of the authors and do not necessarily represent those of their affiliated organizations, or those of the publisher, the editors and the reviewers. Any product that may be evaluated in this article, or claim that may be made by its manufacturer, is not guaranteed or endorsed by the publisher.
References
1. Ahmadpour N, Randall H, Choksi H, Gao A, Vaughan C, Poronnik P. Virtual reality interventions for acute and chronic pain management. Int J Biochem Cell Biol. (2019) 114:105568. 10/gg7h9231306747
2. Al-Ghamdi NA, Meyer WJ 3rd, Atzori B, Alhalabi W, Seibel CC, Ullman D, et al. Virtual reality analgesia with interactive eye tracking during brief thermal pain stimuli: a randomized controlled trial (crossover design). Front Hum Neurosci. (2020) 13:467. doi: 10.3389/fnhum.2019.00467
3. Alrimy T, Alhalabi W, Malibari AA, Alzahrani FS, Alrajhi S, Alhalabi M, et al. Virtual reality animal rescue world: pediatric virtual reality analgesia during just noticeable pressure pain in children aged 2–10 years old (crossover design). Front Psychol. (2022) 13:963765. doi: 10.3389/fpsyg.2022.963765
4. Angwin AJ, Wilson WJ, Copland DA, Barry RJ, Myatt G, Arnott WL. The impact of auditory white noise on semantic priming. Brain Lang. (2018) 180–182:1–7. doi: 10.1016/j.bandl.2018.04.001
5. Atzori B, Vagnoli L, Graziani D, Hoffman HG, Sampaio M, Alhalabi W, et al. An exploratory study on the effectiveness of virtual reality analgesia for children and adolescents with kidney diseases undergoing venipuncture. Int J Environ Res Public Health. (2022) 19(4):2291. doi: 10.3390/ijerph19042291
6. Babiloni C, Brancucci A, Percio CD, Capotosto P, Arendt-Nielsen L, Chen ACN, et al. Anticipatory electroencephalography alpha rhythm predicts subjective perception of pain intensity. J Pain. (2006) 7(10):709–17. doi: 10.1016/j.jpain.2006.03.005
7. Bermo MS, Patterson D, Sharar SR, Hoffman H, Lewis DH. Virtual reality to relieve pain in burn patients undergoing imaging and treatment. Top Magn Reson Imaging (2020) 29(4):203–8. doi: 10.1097/RMR.0000000000000248
8. Bicego A, Rousseaux F, Faymonville ME, Nyssen AS, Vanhaudenhuyse A. Neurophysiology of hypnosis in chronic pain: a review of recent literature. Am J Clin Hypn. (2021) 64(1):62–80. doi: 10.1080/00029157.2020.1869517
9. Bordeleau M, Stamenkovic A, Tardif P-A, Thomas J. The use of virtual reality in back pain rehabilitation: a systematic review and meta-analysis. J Pain. (2022) 23(2):175–95. doi: 10.1016/j.jpain.2021.08.001
10. Botella C, Garcia-Palacios A, Vizcaíno Y, Herrero R, Baños RM, Belmonte MA. Virtual reality in the treatment of fibromyalgia: a pilot study. Cyberpsychol Behav Soc Netw. (2013) 16(3):215–23. doi: 10.1089/cyber.2012.1572
11. Boyle Y, Bentley DE, Watson A, Jones AK. Acoustic noise in functional magnetic resonance imaging reduces pain unpleasantness ratings. NeuroImage. (2006) 31(3):1278–83. 10/fdbhhs16517183
12. Caravan B, Hu L, Veyg D, Kulkarni P, Zhang Q, Chen ZS, et al. Sleep spindles as a diagnostic and therapeutic target for chronic pain. Mol Pain. (2020) 16:174480692090235. doi: 10.1177/1744806920902350
13. Casciaro F, Laterza V, Conte S, Pieralice M, Federici A, Todarello O, et al. Alpha-rhythm stimulation using brain entrainment enhances heart rate variability in subjects with reduced HRV. World J Neurosci. (2013) 03(04):213–20. doi: 10.4236/wjns.2013.34028
14. Célestin-Lhopiteau I, Bioy A, Bernard C. Chapitre 33. Réalité virtuelle thérapeutique et hypnose: Une médecine digitale à notre portée !, in Hypnoanalgésie et hypnosédation. Paris: Dunod (Aide-Mémoire) (2020). p. 256–61. doi: 10.3917/dunod.celes.2020.01.0256
15. Chan E, Foster S, Sambell R, Leong P. (2018) Clinical efficacy of virtual reality for acute procedural pain management: a systematic review and meta-analysis. PLoS One. 13(7):e0200987. doi: 10.1371/journal.pone.0200987
16. Chow H, Hon J, Chua W, Chuan A. Effect of virtual reality therapy in reducing pain and anxiety for cancer-related medical procedures: a systematic narrative review. J Pain Symptom Manage. (2021) 61(2):384–94. 10/ghdvk8 32822755
17. Chuan A, Zhou JJ, Hou RM, Stevens CJ, Bogdanovych A. Virtual reality for acute and chronic pain management in adult patients: a narrative review. Anaesthesia. (2021) 76(5):695–704. doi: 10.1111/anae.15202
18. Colloca L, Raghuraman N, Wang Y, Akintola T, Brawn-Cinani B, Colloca G, et al. Virtual reality: physiological and behavioral mechanisms to increase individual pain tolerance limits. Pain. (2020) 161(9):2010–21. doi: 10.1097/j.pain.0000000000001900
19. Corona-González CE, Alonso-Valerdi LM, Ibarra-Zarate DI. Personalized theta and beta binaural beats for brain entrainment: an electroencephalographic analysis. Front Psychol. (2021) 12:764068. doi: 10.3389/fpsyg.2021.764068
20. Coulibaly I, Cardelli LS, Duflos C, Moulis L, Mandoorah B, Nicoleau J, et al. Virtual reality hypnosis in the electrophysiology lab: when human treatments are better than virtual ones. J Clin Med. (2022) 11(13):3913. doi: 10.3390/jcm11133913
21. Dang A, Arora D, Rane P. Role of digital therapeutics and the changing future of healthcare. J Family Med Prim Care. (2020) 9(5):2207. doi: 10.4103/jfmpc.jfmpc_105_20
22. Dang A, Dang D, Rane P. The expanding role of digital therapeutics in the post-COVID-19 era. Open COVID J. (2021) 1(1):32–7. doi: 10.2174/2666958702101010032
23. Bukhari AAS, Deng X, Huang J, Liu K, Durrani M, Zaman MH. Evaluating binaural beats as a therapeutic tool for intervening visually-induced motion sickness in virtual reality environments. J Rehman Med Inst. (2022) 8(1):3–11. doi: 10.52442/jrmi.v8i1.397
24. Dent S, Burger K, Stevens S, Smith BD, Streepey JW. The effect of music on body sway when standing in a moving virtual environment. PLoS One. (2021) 16(9):e0258000. doi: 10.1371/journal.pone.0258000
25. Ding L, Hua H, Zhu H, Zhu S, Lu J, Zhao K, et al. Effects of virtual reality on relieving postoperative pain in surgical patients: a systematic review and meta-analysis. Int J Surg. (2020) 82:87–94. 10/gpdnvv 32882400
26. Doyle J.A. and Evans A.C. What colour is neural noise? arXiv:1806.03704 [q-bio] [Preprint]. (2018) Available at: http://arxiv.org/abs/1806.03704 (Accessed 9 March, 2020).
27. Ecsy K, Jones AKP, Brown CA. Alpha-range visual and auditory stimulation reduces the perception of pain. Eur J Pain. (2017) 21(3):562–72. 10/f9sppf 27807916
28. Eijlers R, Utens EM, Staals LM, de Nijs PF, Berghmans JM, Wijnen RM, et al. Systematic review and meta-analysis of virtual reality in pediatrics: effects on pain and anxiety. Anesth Analg. (2019) 129(5):1344–53. doi: 10.1213/ANE.0000000000004165
29. Elkins GR, Barabasz AF, Council JR, Spiegel D. Advancing research and practice: the revised APA division 30 definition of hypnosis. Am J Clin Hypn. (2015) 57(4):378–85. doi: 10.1080/00029157.2015.1011465
30. Enea V, Dafinoiu I, Opriş D, David D. Effects of hypnotic analgesia and virtual reality on the reduction of experimental pain among high and low hypnotizables. Int J Clin Exp Hypn. (2014) 62(3):360–77. doi: 10.1080/00207144.2014.901087
31. Farokhnezhad Afshar P, Bahramnezhad F, Asgari P, Shiri M. Effect of white noise on sleep in patients admitted to a coronary care. J Caring Sci. (2016) 5(2):103–9. doi: 10.15171/jcs.2016.011
32. Faymonville ME, Fissette J, Mambourg PH, Roediger L, Joris J, Lamy M. Hypnosis as adjunct therapy in conscious sedation for plastic surgery. Reg Anesth. (1995) 20(2):145–51. PMID: 7605762.7605762
33. Faymonville EM, Mambourg HP, Joris J, Vrijens B, Fissette J, et al. Psychological approaches during conscious sedation. Hypnosis versus stress reducing strategies: a prospective randomized study. Pain. (1997) 73(3):361–7. 10/fsm5cc 9469526
34. Faymonville ME, Roediger L, Del Fiore G, Delgueldre C, Phillips C, Lamy M, et al. Increased cerebral functional connectivity underlying the antinociceptive effects of hypnosis. Brain Res Cogn Brain Res. (2003) 17(2):255–62. 10/b3mqnx 12880897
35. Faymonville M-E, Boly M, Laureys S. Functional neuroanatomy of the hypnotic state. J Physiol Paris. (2006) 99(4–6):463–9. 10/cpw7v4 16750615
36. Faymonville ME, Fiore GD, Maquet P. Neural mechanisms of antinociceptive effects of hypnosis. Anesthesiology. (2000) 92(5):11. doi: 10.1097/00000542-200005000-00013
37. Fletcher D, Martinez V. Opioid-induced hyperalgesia in patients after surgery: a systematic review and a meta-analysis. Br J Anaesth. (2014) 112(6):991–1004. 10/f545rw 24829420
38. Frey DP, Bauer ME, Bell CL, Low LK, Hassett AL, Cassidy RB, et al. Virtual reality analgesia in labor: the VRAIL pilot study—a preliminary randomized controlled trial suggesting benefit of immersive virtual reality analgesia in unmedicated laboring women. Anesth Analg. (2019) 128(6):e93–6. 10/ggmc7g 31094789
39. Gao X, Cao H, Ming D, Qi H, Wang X, Wang X, et al. Analysis of EEG activity in response to binaural beats with different frequencies. Int J Psychophysiol. (2014) 94(3):399–406. 10/f6whqg 25448376
40. Garcia LM, Birckhead BJ, Krishnamurthy P, Sackman J, Mackey IG, Louis RG, et al. An 8-week self-administered at-home behavioral skills-based virtual reality program for chronic low back pain: double-blind, randomized, placebo-controlled trial conducted during COVID-19. J Med Internet Res. (2021) 23(2):e26292. 10/gngz7m 33484240
41. Garza-Villarreal EA, Wilson AD, Vase L, Brattico E, Barrios FA, Jensen TS, et al. Music reduces pain and increases functional mobility in fibromyalgia. Front Psychol. (2014) 5:90. doi: 10.3389/fpsyg.2014.00090
42. Gerhardt A, Leisner S, Hartmann M, Janke S, Seidler GH, Eich W, et al. Eye movement desensitization and reprocessing vs. Treatment-as-usual for non-specific chronic back pain patients with psychological trauma: a randomized controlled pilot study. Front Psychiatry. (2016) 7:201. Available at: https://www.frontiersin.org/article/10.3389/fpsyt.2016.00201 (Accessed: 1 February, 2022). doi: 10.3389/fpsyt.2016.00201
43. Goodin P, Ciorciari J, Baker K, Carey AM, Harper M, Kaufman J. A high-density EEG investigation into steady state binaural beat stimulation. PLoS One. (2012) 7(4):e34789. doi: 10.1371/journal.pone.0034789
44. Grant M, Threlfo C. EMDR in the treatment of chronic pain. J Clin Psychol. (2002) 58(12):1505–20. 10/frhrsx 12455018
45. Guo C, Deng H, Yang J. Effect of virtual reality distraction on pain among patients with hand injury undergoing dressing change. J Clin Nurs. (2015) 24(1–2):115–20. 10/f63zq3 24899241
46. Gutiérrez-Maldonado J, Gutiérrez-Martínez O, Loreto-Quijada D, Nieto-Luna R. The use of virtual reality for coping with pain with healthy participants. Psicothema. (2012) 24(4):516–22. PMID: 23079345.
47. Hadjiat Y, Marchand S. Virtual reality and the mediation of acute and chronic pain in adult and pediatric populations: research developments. Front Pain Res. (2022) 3:840921. doi: 10.3389/fpain.2022.840921
48. Hekmat H, Groth S, Rogers D. Pain ameliorating effect of eye movement desensitization. J Behav Ther Exp Psychiatry. (1994) 25(2):121–9. 10/b5c7pk 7983221
49. Herkt D, Tumani V, Grön G, Kammer T, Hofmann A, Abler B. Facilitating access to emotions: neural signature of EMDR stimulation. PLoS One. (2014) 9(8):e106350. doi: 10.1371/journal.pone.0106350
50. Hoffman HG, Doctor JN, Patterson DR, Carrougher GJ, Furness TA 3rd. Virtual reality as an adjunctive pain control during burn wound care in adolescent patients. Pain. (2000) 85(1–2):305–9. doi: 10.1016/s0304-3959(99)00275-4
51. Hoffman HG, Richards TL, Coda B, Bills AR, Blough D, Richards AL, et al. Modulation of thermal pain-related brain activity with virtual reality: evidence from fMRI. NeuroReport. (2004) 15(8):1245–8. 10/d6q43p 15167542
52. Hoffman HG, Seibel EJ, Richards TL, Furness TA, Patterson DR, Sharar SR. Virtual reality helmet display quality influences the magnitude of virtual reality analgesia. J Pain. (2006) 7(11):843–50. 10/dkndds 17074626
53. Hoffman HG, Richards TL, Van Oostrom T, Coda BA, Jensen MP, Blough DK, et al. The analgesic effects of opioids and immersive virtual reality distraction: evidence from subjective and functional brain imaging assessments. Anesth Analg. (2007) 105(6):1776–83; table of contents. doi: 10.1213/01.ane.0000270205.45146.db
54. Hoffman HG, Chambers GT, Meyer WJ 3rd, Arceneaux LL, Russell WJ, Seibel EJ, et al. Virtual reality as an adjunctive non-pharmacologic analgesic for acute burn pain during medical procedures. Ann Behav Med. (2011) 41(2):183–91. 10/fjwzqq 21264690
55. Hoffmann J, May A. Diagnosis, pathophysiology, and management of cluster headache. Lancet Neurol. (2018) 17(1):75–83. doi: 10.1016/S1474-4422(17)30405-2
56. Honzel E, Murthi S, Brawn-Cinani B, Colloca G, Kier C, Varshney A, et al. Virtual reality, music, and pain: developing the premise for an interdisciplinary approach to pain management. Pain. (2019) 160(9):1909–19. doi: 10.1097/j.pain.0000000000001539
57. van den Hout MA, Engelhard IM, Rijkeboer MM, Koekebakker J, Hornsveld H, Leer A, et al. EMDR: eye movements superior to beeps in taxing working memory and reducing vividness of recollections. Behav Res Ther. (2011) 49(2):92–8. 10/b28mgt 21147478
58. Indovina P, Barone D, Gallo L, Chirico A, De Pietro G, Giordano A. Virtual reality as a distraction intervention to relieve pain and distress during medical procedures: a comprehensive literature review. Clin J Pain. (2018) 34(9):858–77. doi: 10.1097/AJP.0000000000000599
59. JahaniShoorab N, Ebrahimzadeh Zagami S, Nahvi A, Mazluom SR, Golmakani N, Talebi M, et al. The effect of virtual reality on pain in primiparity women during episiotomy repair: a randomize clinical trial. Iran J Med Sci. (2015) 40(3):6. PMID: 25999621.
60. Jensen MP, Sherlin LH, Gertz KJ, Braden AL, Kupper AE, Gianas A, et al. Brain EEG activity correlates of chronic pain in persons with spinal cord injury: clinical implications. Spinal Cord. (2013) 51(1):55–8. doi: 10.1038/sc.2012.84
61. Jensen MP, Jamieson GA, Lutz A, Mazzoni G, McGeown WJ, Santarcangelo EL, et al. New directions in hypnosis research: strategies for advancing the cognitive and clinical neuroscience of hypnosis. Neurosci Conscious. (2017) 2017:1. doi: 10.1093/nc/nix004
62. Jensen MP, Day MA, Miró J. Neuromodulatory treatments for chronic pain: efficacy and mechanisms. Nat Rev Neurol. (2014) 10(3):167–78. 10/f5vm6w 24535464
63. Jin W, Choo A, Gromala D, Shaw C, Squire P. A virtual reality game for chronic pain management: a randomized, controlled clinical study. Stud Health Technol Inform. (2016) 220:154–60. PMID: 27046570.27046570
64. Johnson S, Coxon M. Sound can enhance the analgesic effect of virtual reality. R Soc Open Sci. (2016) 3(3):150567. doi: 10.1098/rsos.150567
65. Jones T, Moore T, Choo J. The impact of virtual reality on chronic pain. PLoS One. (2016) 11:12. doi: 10.1371/journal.pone.0167523
66. Kaminska D, Smolka K, Zwolinski G, Wiak S, Merecz-Kot D, Anbarjafari G. Stress reduction using bilateral stimulation in virtual reality. IEEE Access. (2020) 8:200351–66. doi: 10.1109/ACCESS.2020.3035540
67. Karakoç A, Türker F. Effects of white noise and holding on pain perception in newborns. Pain Manag Nurs. (2014) 15(4):864–70. doi: 10.1016/j.pmn.2014.01.002
68. Käthner I, Bader T, Pauli P. Heat pain modulation with virtual water during a virtual hand illusion. Sci Rep. (2019) 9(1):1–12. 10/gg7rq4
69. Kendrick C, Sliwinski J, Yu Y, Johnson A, Fisher W, Kekecs Z, et al. Hypnosis for acute procedural pain: a critical review. Int J Clin Exp Hypn. (2016) 64(1):75–115. doi: 10.1080/00207144.2015.1099405
70. Kiryu T, So RH. Sensation of presence and cybersickness in applications of virtual reality for advanced rehabilitation. J Neuroeng Rehabil. (2007) 4:34. doi: 10.1186/1743-0003-4-34
71. Kucukoglu S, Aytekin A, Celebioglu A, Celebi A, Caner I, Maden R. Effect of white noise in relieving vaccination pain in premature infants. Pain Manag Nurs. (2016) 17(6):392–400. doi: 10.1016/j.pmn.2016.08.006
72. Lambert V, Boylan P, Boran L, Hicks P, Kirubakaran R, Devane D, et al. Virtual reality distraction for acute pain in children. Cochrane Database Syst Rev. (2020) 10(10):CD010686. doi: 10.1002/14651858.CD010686.pub2
73. Langlois P, Perrochon A, David R, Rainville P, Wood C, Vanhaudenhuyse A, et al. Hypnosis to manage musculoskeletal and neuropathic chronic pain: a systematic review and meta-analysis. Neurosci Biobehav Rev. (2022) 135:104591. doi: 10.1016/j.neubiorev.2022.104591
74. Lewis GN, Rosie JA. Virtual reality games for movement rehabilitation in neurological conditions: how do we meet the needs and expectations of the users? Disabil Rehabil. (2012) 34(22):1880–6. doi: 10.3109/09638288.2012.670036
75. López-Caballero F, Escera C. Binaural beat: a failure to enhance EEG power and emotional arousal. Front Hum Neurosci. (2017) 11:557. doi: 10.3389/fnhum.2017.00557
76. López-Valverde N, Muriel-Fernández J, López-Valverde A, Valero-Juan LF, Ramírez JM, Flores-Fraile J, et al. Use of virtual reality for the management of anxiety and pain in dental treatments: systematic review and meta-analysis. J Clin Med. (2020) 9(10):3086. doi: 10.3390/jcm9103086
77. Maddern J, Grundy L, Castro J, Brierley SM. Pain in endometriosis. Front Cell Neurosci. (2020) 14:590823. 10/gpc2g233132854
78. Mallari B, Spaeth EK, Goh H, Boyd BS. Virtual reality as an analgesic for acute and chronic pain in adults: a systematic review and meta-analysis. J Pain Res. (2019) 12:2053–85. 10/gg7rtr 31308733
79. Maroufi M, Zamani S, Izadikhah Z, Marofi M, O'Connor P. Investigating the effect of eye movement desensitization and reprocessing (EMDR) on postoperative pain intensity in adolescents undergoing surgery: a randomized controlled trial. J Adv Nurs. (2016) 72(9):2207–17. doi: 10.1111/jan.12985
80. Mazzola A, Calcagno ML, Goicochea MT, Pueyrredòn H, Leston J, Salvat F. L’EMDR dans le traitement de la douleur chronique. J EMDR Pract Res. (2010) 4(3):31–44. 10/dnrqwd
81. McCauley ME, Sharkey TJ. Cybersickness: perception of self-motion in virtual environments. Presence. (1992) 1(3):311–8. doi: 10.1162/pres.1992.1.3.311
82. McSherry T, Atterbury M, Gartner S, Helmold E, Searles DM, Schulman C. Randomized, crossover study of immersive virtual reality to decrease opioid use during painful wound care procedures in adults. J Burn Care Res. (2017) 1:278–85. doi: 10.1097/BCR.0000000000000589
83. Melo M, Goncalves G, Monteiro P, Coelho H, Vasconcelos-Raposo J, Bessa M. Do multisensory stimuli benefit the virtual reality experience? A systematic review. IEEE Trans Visualiz Comp Graph. (2022) 28(2):1428–42. doi: 10.1109/TVCG.2020.3010088
84. Merlot B, Dispersyn G, Husson Z, Chanavaz-Lacheray I, Dennis T, Greco-Vuilloud J, et al. Pain reduction with an immersive digital therapeutic tool in women living with endometriosis-related pelvic pain: randomized controlled trial. J Med Internet Res. (2022) 24(9):e39531. doi: 10.2196/39531
85. Mills SEE, Nicolson KP, Smith BH. Chronic pain: a review of its epidemiology and associated factors in population-based studies. Br J Anaesth. (2019) 123(2):e273–83. doi: 10.1016/j.bja.2019.03.023
86. Mosso-Vázquez JL, Gao K, Wiederhold BK, Wiederhold MD. Virtual reality for pain management in cardiac surgery. Cyberpsychol Behav Soc Netw. (2014) 17(6):371–8. 10/f56c9w
87. Mouraux A, Guérit JM, Plaghki L. Non-phase locked electroencephalogram (EEG) responses to CO2 laser skin stimulations may reflect central interactions between A∂- and C-fibre afferent volleys. Clin Neurophysiol. (2003) 114(4):710–22. doi: 10.1016/S1388-2457(03)00027-0
88. Mujib MD, Hasan MA, Qazi SA, Vuckovic A. Understanding the neurological mechanism involved in enhanced memory recall task following binaural beat: a pilot study. Exp Brain Res. (2021) 239(9):2741–54. 10/gpj89m 34232346
89. Nadeau SE, Wu JK, Lawhern RA. Opioids and chronic pain: an analytic review of the clinical evidence. Front Pain Res. (2021) 2:721357. doi: 10.3389/fpain.2021.721357
90. Ngo HV, Claussen JC, Born J, Mölle M. Induction of slow oscillations by rhythmic acoustic stimulation. J Sleep Res. (2013) 22(1):22–31. doi: 10.1111/j.1365-2869.2012.01039.x
91. Nicholson B, Verma S. Comorbidities in chronic neuropathic pain. Pain Med. (2004) 5(suppl 1):S9–27. doi: 10.1111/j.1526-4637.2004.04019.x
92. Ortiz-Catalan M, Guðmundsdóttir RA, Kristoffersen MB, Zepeda-Echavarria A, Caine-Winterberger K, Kulbacka-Ortiz K, et al. Phantom motor execution facilitated by machine learning and augmented reality as treatment for phantom limb pain: a single group, clinical trial in patients with chronic intractable phantom limb pain. Lancet. (2016) 388(10062):2885–94. doi: 10.1016/S0140-6736(16)31598-7
93. Papalambros NA, Santostasi G, Malkani RG, Braun R, Weintraub S, Paller KA, et al. Acoustic enhancement of sleep slow oscillations and concomitant memory improvement in older adults. Front Hum Neurosci. (2017) 11:109. 10/ggsw9k 28337134
94. Papalambros NA, Weintraub S, Chen T, Grimaldi D, Santostasi G, Paller KA, et al. Acoustic enhancement of sleep slow oscillations in mild cognitive impairment. Ann Clin Transl Neurol. (2019) 6(7):1191–201. doi: 10.1002/acn3.796
95. Park MJ, Kim DJ, Lee U, Na EJ, Jeon HJ. A literature overview of virtual reality (VR) in treatment of psychiatric disorders: recent advances and limitations. Front Psychiatry. (2019) 10:505. doi: 10.3389/fpsyt.2019.00505
96. Patterson DR, Jensen MP, Wiechman SA, Sharar SR. Virtual reality hypnosis for pain associated with recovery from physical trauma. Int J Clin Exp Hypn. (2010) 58(3):288–300. 10/chxv3d 20509069
97. Pekyavas NO, Ergun N. Comparison of virtual reality exergaming and home exercise programs in patients with subacromial impingement syndrome and scapular dyskinesis: short term effect. Acta Orthop Traumatol Turc. (2017) 51(3):238–42. doi: 10.1016/j.aott.2017.03.008
98. Peng W, Hu L, Zhang Z, Hu Y. Changes of spontaneous oscillatory activity to tonic heat pain. PLoS One. (2014) 9(3):e91052. doi: 10.1371/journal.pone.0091052
99. Perales FJ, Riera L, Ramis S, Guerrero A. Evaluation of a VR system for pain management using binaural acoustic stimulation. Multimed Tools Appl. (2019) 78(23):32869–90. doi: 10.1007/s11042-019-07953-y
100. Perales FJ, Sanchez M, Ramis S. A mood modulation using virtual reality and binaural sounds. Proceedings of the XX international conference on human computer interaction—interacción ‘19. The XX international conference, June 2019. Donostia, Spain. New York, NY: Association for Computing Machinery (2019). pp. 1–2. 10/gg7rhd
101. Ploner M, Gross J, Timmermann L, Pollok B, Schnitzler A. Pain suppresses spontaneous brain rhythms. Cerebral Cortex. (2006) 16(4):537–40. doi: 10.1093/cercor/bhj001
102. Pourmand A, Davis S, Marchak A, Whiteside T, Sikka N. Virtual reality as a clinical tool for pain management. Curr Pain Headache Rep. (2018) 22(8):53. 10/gdr7hs 29904806
103. Rainville P, Duncan GH, Price DD, Carrier B, Bushnell MC. Pain affect encoded in human anterior cingulate but not somatosensory cortex. Science. (1997) 277(5328):968–71. doi: 10.1126/science.277.5328.968
104. Rainville P, Hofbauer RK, Paus T, Duncan GH, Bushnell MC, Price DD. Cerebral mechanisms of hypnotic induction and suggestion. J Cogn Neurosci. (1999) 11(1):110–25. 10/bfbkzg 9950718
105. Rainville P. Hypnosis and the analgesic effect of suggestions. Pain. (2008) 134(1):1–2. doi: 10.1016/j.pain.2007.10.030
106. Raja SN, Carr DB, Cohen M, Finnerup NB, Flor H, Gibson S, et al. The revised international association for the study of pain definition of pain: concepts, challenges, and compromises. Pain. (2020) 161(9):1976–82. doi: 10.1097/j.pain.0000000000001939
107. Rausch VH, Bauch EM, Bunzeck N. White noise improves learning by modulating activity in dopaminergic midbrain regions and right superior temporal sulcus. J Cogn Neurosci. (2014) 26(7):1469–80. 10/gg7rtd 24345178
108. Rauschecker JP, May ES, Maudoux A, Ploner M. Frontostriatal gating of tinnitus and chronic pain. Trends Cogn Sci (Regul Ed). (2015) 19(10):567–78. doi: 10.1016/j.tics.2015.08.002
109. Riera L, Verger S, Montoya PJ, Perales FJ. Advances in the cognitive management of chronic pain in children through the use of virtual reality combined with binaural beats: a pilot study. Adv Hum Comput Interact. (2022) 2022:2495182. doi: 10.1155/2022/2495182
110. Rousseaux F, Faymonville ME, Nyssen AS, Dardenne N, Ledoux D, Massion PB, et al. Can hypnosis and virtual reality reduce anxiety, pain and fatigue among patients who undergo cardiac surgery: a randomised controlled trial. Trials. (2020) 21(1):330. doi: 10.1186/s13063-020-4222-6
111. Rousseaux F, Bicego A, Ledoux D, Massion P, Nyssen AS, Faymonville ME, et al. Hypnosis associated with 3D immersive virtual reality technology in the management of pain: a review of the literature. J Pain Res. (2020) 13:1129–38. doi: 10.2147/JPR.S231737
112. Salsitz EA. Chronic pain, chronic opioid addiction: a complex nexus. J Med Toxicol. (2016) 12(1):54–7. 10/gn46xm 26602212
113. Sarig Bahat H, Takasaki H, Chen X, Bet-Or Y, Treleaven J. Cervical kinematic training with and without interactive VR training for chronic neck pain—a randomized clinical trial. Man Ther. (2015) 20(1):68–78. doi: 10.1016/j.math.2014.06.008
114. Scelles C, Bulnes LC. EMDR as treatment option for conditions other than PTSD: a systematic review. Front Psychol. (2021) 12:644369. doi: 10.3389/fpsyg.2021.644369
115. Schmidt TT, Prein JC. The Ganzfeld experience—a stably inducible altered state of consciousness: effects of different auditory homogenizations. Psych J. (2019) 8(1):66–81. doi: 10.1002/pchj.262
116. Shapiro F. Eye movement desensitization: a new treatment for post-traumatic stress disorder. J Behav Ther Exp Psychiatry. (1989) 20(3):211–7. 10/cbthcv 2576656
117. Shapiro F. EMDR, adaptive information processing, and case conceptualization. J EMDR Pract Res. (2007) 1(2):68–87. doi: 10.1891/1933-3196.1.2.68
118. Sharar SR, Carrougher GJ, Nakamura D, Hoffman HG, Blough DK, Patterson DR. Factors influencing the efficacy of virtual reality distraction analgesia during postburn physical therapy: preliminary results from 3 ongoing studies. Arch Phys Med Rehabil. (2007) 88(12):S43–9. doi: 10.1016/j.apmr.2007.09.004
119. Sharar SR, Alamdari A, Hoffer C, Hoffman HG, Jensen MP, Patterson DR. Circumplex model of affect: a measure of pleasure and arousal during virtual reality distraction analgesia. Games Health J. (2016) 5(3):197–202. doi: 10.1089/g4h.2015.0046
120. Shiri S, Feintuch U, Weiss N, Pustilnik A, Geffen T, Kay B, et al. A virtual reality system combined with biofeedback for treating pediatric chronic headache—a pilot study. Pain Med. (2013) 14(5):621–7. doi: 10.1111/pme.12083
121. Smith A, Carlow K, Biddulph T, Murray B, Paton M, Harvie DS. Contextual modulation of pain sensitivity utilising virtual environments. Br J Pain. (2017) 11(2):71–80. doi: 10.1177/2049463717698349
122. Spencer JA, Moran DJ, Lee A, Talbert D. White noise and sleep induction. Arch Dis Child. (1990) 65(1):135–7. doi: 10.1136/adc.65.1.135
123. Stanchina ML, Abu-Hijleh M, Chaudhry BK, Carlisle CC, Millman RP. The influence of white noise on sleep in subjects exposed to ICU noise. Sleep Med. (2005) 6(5):423–8. doi: 10.1016/j.sleep.2004.12.004
124. Staud R. Abnormal endogenous pain modulation is a shared characteristic of many chronic pain conditions. Expert Rev Neurother. (2012) 12(5):577–85. doi: 10.1586/ern.12.41
125. Stoelb BL, Molton IR, Jensen MP, Patterson DR. The efficacy of hypnotic analgesia in adults: a review of the literature. Contemp Hypn. (2009) 26(1):24–39. doi: 10.1002/ch.370
126. Sweta V, Abhinav R, Ramesh A. Role of virtual reality in pain perception of patients following the administration of local anesthesia. Ann Maxillofac Surg. (2019) 9(1):110. doi: 10.4103/ams.ams_263_18
127. Tan G, Rintala DH, Jensen MP, Fukui T, Smith D, Williams W. A randomized controlled trial of hypnosis compared with biofeedback for adults with chronic low back pain. Eur J Pain. (2015) 19(2):271–80. doi: 10.1002/ejp.545
128. Tarrant J, Viczko J, Cope H. Virtual reality for anxiety reduction demonstrated by quantitative EEG: a pilot study. Front Psychol. (2018) 9:1280. doi: 10.3389/fpsyg.2018.01280
129. Tefikow S, Barth J, Maichrowitz S, Beelmann A, Strauss B, Rosendahl J. Efficacy of hypnosis in adults undergoing surgery or medical procedures: a meta-analysis of randomized controlled trials. Clin Psychol Rev. (2013) 33(5):623–36. doi: 10.1016/j.cpr.2013.03.005
130. Tesarz J, Leisner S, Gerhardt A, Janke S, Seidler GH, Eich W, et al. Effects of eye movement desensitization and reprocessing (EMDR) treatment in chronic pain patients: a systematic review: systematic review: EMDR in chronic pain. Pain Med. (2014) 15(2):247–63. 10/f6sb9q 24308821
131. Tesarz J, Wicking M, Bernardy K, Seidler GH. EMDR therapy's efficacy in the treatment of pain. J EMDR Pract Res. (2019) 13(4):337–44. doi: 10.1891/1933-3196.13.4.337
132. Theingi S, Leopold I, Ola T, Cohen GS, Maresky HS. Virtual reality as a non-pharmacological adjunct to reduce the use of analgesics in hospitals. J Cogn Enhanc. (2022) 6(1):108–13. doi: 10.1007/s41465-021-00212-9
133. Thompson T, Steffert T, Steed A, Gruzelier J. A randomized controlled trial of the effects of hypnosis with 3-D virtual reality animation on tiredness, mood, and salivary cortisol. Int J Clin Exp Hypn. (2010) 59(1):122–42. doi: 10.1080/00207144.2011.522917
134. Thompson T, Terhune DB, Oram C, Sharangparni J, Rouf R, Solmi M, et al. The effectiveness of hypnosis for pain relief: a systematic review and meta-analysis of 85 controlled experimental trials. Neurosci Biobehav Rev. (2019) 99:298–310. doi: 10.1016/j.neubiorev.2019.02.013
135. de Tommaso M, Ricci K, Laneve L, Savino N, Antonaci V, Livrea P. Virtual visual effect of hospital waiting room on pain modulation in healthy subjects and patients with chronic migraine. Pain Res Treat. (2013) 2013:1–8. doi: 10.1155/2013/515730
136. Treede RD, Rief W, Barke A, Aziz Q, Bennett MI, Benoliel R, et al. A classification of chronic pain for ICD-11. Pain. (2015) 156(6):1003–7. 10/f3ph69 25844555
137. Treede RD, Rief W, Barke A, Aziz Q, Bennett MI, Benoliel R, et al. Chronic pain as a symptom or a disease: the IASP classification of chronic pain for the international classification of diseases (ICD-11). Pain. (2019) 160(1):19–27. 10/gfvp3z 30586067
138. Understanding DTx. Digital therapeutics definition and core principles (2019). Available at: https://dtxalliance.org/understanding-dtx/ (Accessed January 2023).
139. Valiente-Gómez A, Moreno-Alcázar A, Treen D, Cedrón C, Colom F, Pérez V, et al. EMDR beyond PTSD: a systematic literature review. Front Psychol. (2017) 8:1668. 10/gb2zwx
140. Vanhaudenhuyse A, Demertzi A, Schabus M, Noirhomme Q, Bredart S, Boly M, et al. Two distinct neuronal networks mediate the awareness of environment and of self. J Cogn Neurosci. (2011) 23(3):570–8. doi: 10.1162/jocn.2010.21488
141. Vanhaudenhuyse A, Ledoux D, Gosseries O, Demertzi A, Laureys S, Faymonville M-E. Can subjective ratings of absorption, dissociation, and time perception during “neutral hypnosis” predict hypnotizability?: an exploratory study. Int J Clin Exp Hypn. (2019) 67(1):28–38. doi: 10.1080/00207144.2019.1553765
142. Vanhaudenhuyse A, Laureys S, Faymonville M-E. Neurophysiology of hypnosis. Neurophysiol Clin. (2014) 44(4):343–53. doi: 10.1016/j.neucli.2013.09.006
143. Vanhaudenhuyse A, Nyssen A-S, Faymonville M-E. Recent insight on how the neuroscientific approach helps clinicians. OBM Integr Complement Med. (2020) 5(2):1–20. doi: 10.21926/obm.icm.2002028
144. Veličković P, Milovanović M. Improvement of the interaction model aimed to reduce the negative effects of cybersickness in VR rehab applications. Sensors (Basel). (2021) 21(2):321. doi: 10.3390/s21020321
145. Walker MR, Kallingal GJ, Musser JE, Folen R, Stetz MC, Clark JY. Treatment efficacy of virtual reality distraction in the reduction of pain and anxiety during cystoscopy. Mil Med. (2014) 179(8):891–6. 10/f6d4fm 25102532
146. Weech S, Kenny S, Barnett-Cowan M. Presence and cybersickness in virtual reality are negatively related: a review. Front Psychol. (2019) 10:158. doi: 10.3389/fpsyg.2019.00158
147. Welch KL, Beere DB. Eye movement desensitization and reprocessing: a treatment efficacy model. Clin Psychol Psychother. (2002) 9(3):165–76. 10/c2qqjv
148. Wiederhold BK, Gao K, Sulea C, Wiederhold MD. Virtual reality as a distraction technique in chronic pain patients. Cyberpsychol Behav Soc Netw. (2014) 17(6):346–52. 10/f56fv924892196
149. Williams JD, Gruzelier JH. Differentiation of hypnosis and relaxation by analysis of narrow band theta and alpha frequencies. Int J Clin Exp Hypn. (2001) 49(3):185–206. doi: 10.1080/00207140108410070
150. Yang JH, Ryu JJ, Nam E, Lee HS, Lee JK. Effects of preoperative virtual reality magnetic resonance imaging on preoperative anxiety in patients undergoing arthroscopic knee surgery: a randomized controlled study. Arthroscopy. (2019) 35(8):2394–9. doi: 10.1016/j.arthro.2019.02.037
151. Zampi DD. Efficacy of theta binaural beats for the treatment of chronic pain. Altern Ther Health Med. (2016) 22(1):32–8. PMID: 26773319.26773319
152. Zhou J, Liu D, Li X, Ma J, Zhang J, Fang J. Pink noise: effect on complexity synchronization of brain activity and sleep consolidation. J Theor Biol. (2012) 306:68–72. doi: 10.1016/j.jtbi.2012.04.006
Keywords: digital therapeutics, pain, virtual reality, analgesia, hypnosis, binaural beats, colored noise, bilateral alternative stimulation
Citation: Fougère M, Greco-Vuilloud J, Arnous C, Abel F, Lowe C, Elie V and Marchand S (2023) Sensory stimulations potentializing digital therapeutics pain control. Front. Pain Res. 4:1168377. doi: 10.3389/fpain.2023.1168377
Received: 17 February 2023; Accepted: 14 August 2023;
Published: 6 September 2023.
Edited by:
Pierrick Poisbeau, Université de Strasbourg, FranceReviewed by:
Mathieu Roy, McGill University, CanadaEugenio Santoro, Mario Negri Institute for Pharmacological Research (IRCCS), Italy
© 2023 Fougère, Greco-Vuilloud, Arnous, Abel, Lowe, Elie and Marchand. This is an open-access article distributed under the terms of the Creative Commons Attribution License (CC BY). The use, distribution or reproduction in other forums is permitted, provided the original author(s) and the copyright owner(s) are credited and that the original publication in this journal is cited, in accordance with accepted academic practice. No use, distribution or reproduction is permitted which does not comply with these terms.
*Correspondence: Serge Marchand c2VyZ2UubWFyY2hhbmRAdXNoZXJicm9va2UuY2E=
†These authors have contributed equally to this work