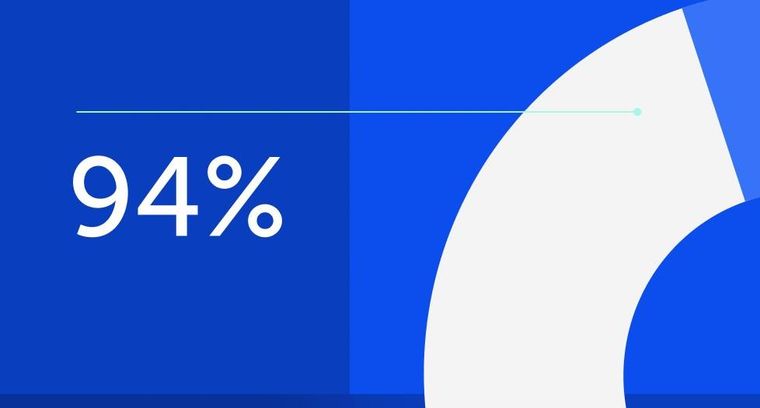
94% of researchers rate our articles as excellent or good
Learn more about the work of our research integrity team to safeguard the quality of each article we publish.
Find out more
REVIEW article
Front. Pain Res., 24 May 2023
Sec. Musculoskeletal Pain
Volume 4 - 2023 | https://doi.org/10.3389/fpain.2023.1150749
Chronic primary orofacial pain (OFP) conditions such as painful temporomandibular disorders (pTMDs; i.e., myofascial pain and arthralgia), idiopathic trigeminal neuralgia (TN), and burning mouth syndrome (BMS) are seemingly idiopathic, but evidence support complex and multifactorial etiology and pathophysiology. Important fragments of this complex array of factors have been identified over the years largely with the help of preclinical studies. However, findings have yet to translate into better pain care for chronic OFP patients. The need to develop preclinical assays that better simulate the etiology, pathophysiology, and clinical symptoms of OFP patients and to assess OFP measures consistent with their clinical symptoms is a challenge that needs to be overcome to support this translation process. In this review, we describe rodent assays and OFP pain measures that can be used in support of chronic primary OFP research, in specific pTMDs, TN, and BMS. We discuss their suitability and limitations considering the current knowledge of the etiology and pathophysiology of these conditions and suggest possible future directions. Our goal is to foster the development of innovative animal models with greater translatability and potential to lead to better care for patients living with chronic primary OFP.
Orofacial pain (OFP) is that localized below the orbito-meatal line, above the neck and anterior to the ears, including pain in structures of the oral cavity. According to the latest OFP classification, OFP can be acute, when it lasts for less than 3 months, episodic, when it occurs on fewer than 15 days per month whether or not for more than 3 months, or chronic, when it persists for more than 3 months and is present on at least 15 days per month (1). This distinction is important because chronic OFP is often accompanied by reduced quality of life, sleep and psychological disturbances and disability (2–6) that require different management and it has less favorable prognosis. The estimated prevalence of chronic OFP in the general population is of 10% (7–9). OFP can be additionally classified as primary, when its etiology is unknown (e.g., chronic primary temporomandibular joint pain and idiopathic trigeminal neuralgia), or secondary, when it has an identifiable cause (e.g., temporomandibular joint pain attributed to arthritis and trigeminal neuralgia attributed to multiple sclerosis). In other words, chronic primary OFP conditions can be understood as their own disease, while chronic secondary pain syndromes represent symptoms of other underlying conditions or diseases (10).
Some of the most challenging types of chronic primary OFP to manage include painful temporomandibular disorders (pTMDs), idiopathic trigeminal neuralgia (TN), and burning mouth syndrome (BMS). These seemingly idiopathic conditions have no cure and treatment is mostly palliative, geared toward pain management and coping strategies. Often, patients living chronic pain, including pTMDs, TN, and BMS, must endure significant biological, psychosocial, and economic burdens that also affect their families, and society as a whole (11).
The most common types of primary pTMDs are myofascial pain and temporomandibular joint pain (12). These conditions have a prevalence of about 5% that is greater in women than in men (13, 14) and their hallmark is spontaneous masticatory muscle and/or temporomandibular joint pain that is exacerbated upon jaw function. TN has a lifetime prevalence of 0.16%–0.3% and also affects more women than men (1.5:1) (15). TN is characterized by recurrent severe paroxysmal pain restricted to the territory of the trigeminal nerves (which innervate the orofacial region) lasting from a fraction of a second up to 2 min. TN pain is described as electric shock-like, stabbing, or sharp, and is triggered by innocuous stimuli (e.g., washing the face, eating, and brushing the teeth). BMS has a prevalence of 2.5%–5% in the general population that rises to 14% among post-menopausal women. BMS is characterized by a spontaneous burning sensation that most commonly affects the tongue, lips, and hard and soft palates and may be accompanied by an alteration of taste, a stinging sensation, dryness, and atypical odontalgia (16).
Despite considerable scientific advances over the past decades (17, 18), the need for greater understanding of the pathophysiology underlying these primary OFP conditions persists because treatment remains suboptimal. In this scenario, pre-clinical research has been and will continue to be an important and irreplaceable means of facilitating scientific advancement. Furthermore, we are currently experiencing a boom of both pain neuroimaging and genetic studies that find crucial complementation in animal research (19, 20).
The goal of this review is to describe the currently available rodent assays (injury models) and OFP measures (behavior) that can be used in support of chronic primary OFP research, in specific pTMDs, TN, and BMS. We will discuss their applicability and challenges considering our current knowledge of the etiology and pathophysiology of these conditions and possible future directions. Previous reviews have focused on other conditions linked to OFP, including migraine/headaches (20, 21) and oral cancer (22).
Chronic primary pTMDs have multifactorial etiology and complex pathophysiology that involve the biological [e.g., genetics (23), central sensitization (24)], psychosocial [e.g., stress and somatic symptoms (25)] and environmental [e.g., trauma (26)] realms (27). Mimicking this complex array of factors in animal models to enable studying the mechanisms leading to and sustaining pTMDs is clearly challenging. Hence, pre-clinical pTMD research has been mainly based on assays that induce pain to the orofacial region using the chemical, surgical, and jaw trauma assays described next (Table 1). This review does not include assays that modify occlusion (i.e., the way upper and lower teeth fit together) as a means of inducing pain the orofacial region because there is sufficient and consistent scientific evidence of the absence of causal relationship between occlusal factors and pTMDs (28).
Here we briefly describe and discuss the most frequently used assays involving the injection of inflammatory and pain-inducing agents into the TMJ or masseter muscle to induce TMD-like pain in rodents. These assays are typically complemented with experiments assessing the OFP measure(s) of choice, which often include nocifensive behaviors, mechanical and thermal pain sensitivity tests, but can also include bite force and orofacial operant tests. Often developed and used to study mechanisms underlying pTMDs (29, 57, 79–82), the main limitation of chemical assays is their inability to sufficiently simulate the etiological and/or pathophysiological mechanisms of pTMDs. Moreover, except for Complete Freund's Adjuvant (CFA), these chemical agents induce pain that last for short periods of time. Nonetheless, the use of these assays has allowed the accumulation of critical evidence on the mechanisms of trigeminal nerve pain transmission (17). This knowledge forms the basis required to advance the methods employed in developing more clinically similar assays.
Introduced in 1989 (83), this is a reliable assay for inflicting OFP in rodents (29, 30). In this model, formalin (recommended concentration up to 1.5%) (31) is injected subcutaneously into the TMJ or masseter region (29, 32, 33). The reliability of this assay lies on its consistent elicitation of two phases of behavioral responses considered as OFP surrogate measures (e.g., face rubbing): an early phase lasting from 0 to 5 min post-injection and a late phase lasting from 10 to 50 min post-injection [times may vary according to strain (34)]. The duration of each phase may vary with the animal strain. At each phase, the duration of face rubbing is annotated and compared to that of saline-injected animals: prolonged face rubbing has a positive correlation with formalin concentration. Pain in the early phase is attributed to direct stimulation of sensory afferent neurons, while pain in the last phase has been proposed to reflect the combined effects of afferent input, central sensitization and inflammatory response (35). While the mechanisms underlying pain in the early phase can arguably parallel acute OFP in humans, as the latter is often the result of jaw trauma (36) (as opposed to the injection of a chemical), the mechanisms in the late phase may partly parallel those involved in chronic primary pTMDs, as central sensitization and pathways of immune and inflammatory response seem to contribute to these conditions (37, 38).
CFA is a valid irritating agent composed of desiccated mycobacterium in paraffin oil and mannide monooleate that induces a potent inflammatory response in rodents following local injection (84) that is accompanied by long-lasting hyperalgesia (i.e., exacerbated pain response to a painful stimulus) and allodynia (i.e., pain response to a non-painful stimulus) (79, 85). CFA can be injected directly into the masseter muscle (39, 40) or into the TMJ (41) to cause OFP: in addition to leading to mechanical (42, 43) and thermal orofacial hypersensitivity (44), it also leads to reduced biting force (45) and signs of spontaneous OFP as assessed using the Grimace Scale (46).
The long-lasting effects (>30 days) (47) of the CFA assay comprise its main advantage, and it is considered one of the few assays that allow the investigation of the “chronic” phase of pain. Nonetheless, the underlying immune and inflammatory processes sustaining OFP post-CFA injection may or may not partly reflect those of chronic OFP in humans, as subcutaneous accumulation of dead bacteria is hardly an OFP trigger in patients.
To quantify the CFA-induced hyperalgesia and/or allodynia, this assay is paired with experiments assessing the OFP measure(s) of choice (48, 49), which often include mechanical and thermal pain sensitivity tests (50), but can also include bite force(52, 51), spontaneous pain assessments (46), and orofacial operant tests (52).
Carrageenan is a seaweed extract used to induce inflammation in rodents since 1962 (86). Its inflammatory and pain-inducing effects may be strain-dependent (87), but generally lead to acute swelling and behavioral nociceptive responses (i.e., face grooming). The injection of carrageenan (usually 1%–1.5%) into the TMJ of rats has been used to investigate the inflammatory aspects of TMJ hyperalgesia, under the assumption that TMJ pain may result from an inflammatory episode (88). These studies have shown the involvement of P2X receptors (57, 58, 89), nerve growth factor (59), β-adrenoceptors (59–61), and the co-participation of peroxisome proliferator-activated receptors-γ (PPAR-γ) and κ/δ opioid receptors (62) in TMJ inflammatory pain.
Pain and inflammation due to the injection of carrageenan is short-lived, typically lasting no more than 14 days.
Capsaicin is the active ingredient in hot pepper, first isolated in 1846 (90). Trigeminal nerve afferents are predominantly peptidergic and enriched with transient receptor potential cation channel subfamily V member 1 (TRPV1) (91, 92). Capsaicin can sensitize and modulate TRPV1-positive trigeminal afferents and brainstem nociceptive neurons in the trigeminal subnucleus caudalis/upper cervical cord (Vc/UCC) (93–97). As early studies have suggested neurogenic inflammation as one of the putative mechanisms of injury leading to pTMDs (98, 99), the injection of capsaicin into the TMJ (80, 100) or masticatory muscles of animals (101) [and humans (102–106)] has been used to study neurogenic aspects of nociceptive pain that may be relevant to pTMDs. For instance, studies have shown that the injection of capsaicin into the TMJ of rats (80, 95, 108), as well as mustard oil (108) and glutamate (71, 95, 108), lead to peripheral sensitization that may contribute to the primary hyperalgesia or allodynic states characteristics of pTMD patients (109). This sensitization is attenuated by N-methyl-d-aspartate (NMDA) receptors antagonists and suggests the involvement of NMDA receptors in nociceptive trigeminal responses (80, 71, 108–111, 110). Sex differences in the orofacial nociceptive response of capsaicin-sensitive neurons also exist (111). Capsaicin cream has also been directly applied to the face of rats to induce mechanical pain sensitivity (64, 65).
The use of mustard oil to induce inflammation in the TMJ was first proposed based on the assumption that inflammation may be one of the possible contributing factors to pTMD (81), and on early studies showing that it activates small C-fibres (112) and induces inflammatory responses such as plasma extravasation (113) and neutrophil infiltration (114). The injection of mustard oil into the TMJ activates Vc/UCC neurons (115, 116) and elicits a short-lasting pain responses that are modulated by opioids and serotonin (117–121). Early studies using mustard oil injected into the TMJ have implicated mechanisms involving NMDA receptors (108, 119), other peripheral excitatory amino acid receptors (122), and neurokinins (123) in TMJ nociception, and peripheral and central γ-aminobutyric acid (GABA)-A receptors in TMJ anti-nociception (124, 125). These effects seem to vary between sexes (126–131) and throughout the estrous cycle (132).
Mustard oil has also been injected into the masseter muscle to induce short-lasting pain (68, 133), which likewise activates neurons in the trigeminal sensory nuclei (134) in a manner that involves peripheral NMDA receptors (135) and peripheral 2-amino-3-(3-hydroxy-5-methyl-isoxazol-4-yl) propanoic acid (AMPA) receptors (136). Studies using this model have also shown the involvement of TRPV1, the transient receptor potential ankyrin type 1 (TRPA1), and the transient receptor potential melastatin type 3 (TRPM3) in craniofacial muscle pain processing (65, 69).
Glutamate is an endogenous neurotransmitter produced by both neuronal [including trigeminal ganglia neurons (137)] and non-neuronal cells (138–140) which acts via the centrally and peripherally expressed NMDA, AMPA, kainate, and metabotropic glutamate receptors. As such, glutamate has complex pain modulating roles centrally and pain inducing effects peripherally (141). Glutamate is long known for its involvement in OFP transmission (116, 122, 142) and transcriptome analysis has recently reaffirmed its role in inflammatory pain-signaling (143). Notably, glutamate seems to produce similar acute pain responses in humans and rats (144).
When injected into the masseter of rodents, glutamate-induced pain leads to both nociceptor activation and sensitization and local inflammation (70, 112, 144). Contrarily, glutamate injected into the TMJ [and other joints(145)] seems to have a limited inflammatory component (146).
NGF is a neurotrophic protein that acts mainly via the tyrosine kinase receptor A (TrkA) and the p75 receptor (147). The suitability of this model for the study of TMD-related pain is largely based on experimental studies in healthy people showing that NGF injection into the masseter leads to long-lasting mechanical allodynia and hyperalgesia and to pain during strenuous jaw movements that are characteristic of pTMDs of muscular origin (148–150).
In rodents, intramuscular injection of NGF has been shown to sensitize masseter muscle afferent fibers without producing local inflammation (59, 151) in a manner that is at least partly mediated by enhanced peripheral NMDA receptor expression (71, 152) and increased glutamate expression in the sensory nerve endings in the muscle (82). Sex-related differences in nociception induced by peripheral NGF exist in both humans and animals (71, 148, 149).
Jaw trauma, including sustained mouth opening and frequency of parafunctional behaviors, are known risk factors of pTMD onset (36, 153) and are associated with chronic pTMDs (26). Hence, assays based on jaw trauma represent more construct-valid (i.e., property of an assay that seems to have a biologic basis like that of patients) models of pTMDs. However, these assays are not as widely known nor as widely used as the chemical assays and future in-depth investigations of the pain mechanisms following these assays could shed further light into the mechanisms that sustain pain in humans.
Considering microtrauma, including repetitive parafunctional oral habits, as a contributor factor to pTMDs (referred to as myofascial pain syndrome of masticatory muscles at the time), the assay employing repetitive contractions with forced lengthening of the mice's masseter was first proposed in 1995 to induce pTMD in mice (72). The model was later adapted to rats (73, 74) and consists of restraining anesthetized animals in supine position with their jaw open and using an electrical stimulator to deliver a set of supramaximal stimuli to induce repetitive tetanic eccentric contractions to the masseter unilaterally. Stimuli are delivered every 30 s with 10 s of rest between stimuli for 20 min per session for 14 consecutive days. Control animals are anesthetized, restrained, and the electrode positioned into the masseter muscle (without delivering the electrical stimuli) for the same amount of time.
This assay leads to increases in muscle thickness and reduction of maximum jaw-opening distance, suggesting a phenotype consistent with masticatory myofascial pain (147). However, direct measures of OFP, such as sensitivity to mechanical stimuli or the display of nocifensive behaviors, have not been assessed in animals subjected to this assay and the assay's ability to induce long lasting pain also remains to be demonstrated.
In this assay (75), a bite block is placed between the upper and lower front incisors of anesthetized mice to keep their mouth maximally opened for 1.5 h per day for 5 consecutive days. Starting on day 3, mice display mechanical allodynia in the masseter/TMJ region compared to controls (anesthetized animals that did not undergo sustained mouth opening) that seems to be maintained for at least 27 days after the last sustained mouth opening session. The administration of ibuprofen (nonsteroidal anti-inflammatory), amitriptyline (tricyclic antidepressant), pregabalin (antiepileptic), or morphine (opioid) either partially or completely reversed mechanical allodynia, suggesting that the pain induced by this assay involves a complex array of biological pathways, as is believed to be the case in chronic OFP patients. Notably, mice exposed to the sustained mouth opening assay also displayed a reduced intake of hard-pelleted chow accompanied by weight loss that was reverted after replacement with soft-pelleted chow. These may be considered as surrogate OFP measures that are paralleled in humans, as masticatory dysfunction is a hallmark of pTMDs. Hence, the sustained mouth opening assay may be a suitable alternative to investigate the “chronic” phase of OFP and has greater translatability.
Considering the short-lasting pain induced by other assays used in the study of pTMDs of muscular origin—from hours to few weeks at most—this assay has been developed to induce long-lasting pain in rats (76) and was later adapted to mice (77). It consists of anesthetizing and restraining animals in supine position with their mouth open and accessing, freeing, and tying their tendon of the anterior superficial part of the masseter muscle (TASM) with two chromic gut ligatures, 2-mm apart. Control animals receive the same procedure except for the TASM ligation. In rats, TASM ligation led to short-lasting local inflammation (2 weeks) that subsided after 8 weeks, but orofacial mechanical allodynia and hypersensitivity were maintained throughout 8 weeks. Furthermore, activation and upregulation of NMDA receptors in laminae I/II of Vc/UCC neurons consistent with somatotopy of the mandibular branch (V3) of the trigeminal nerve and hyperreactivity of Vc/UCC astrocytes and microglia were greater in TASM and sham-operated than in naïve animals and lasted longer in TASM than in sham-operated animals. Morphine and duloxetine (serotonin-norepinephrine reuptake inhibitor) administered at 8 weeks d displayed anti-hyperalgesic effects. Further studies employing this assay (but with timelines shorter than 8 weeks) have indicated that GABA-A receptors in trigeminal ganglia cells and Vc/UCC afferent terminals projecting to the orofacial region have OFP-inhibitory roles (154, 155) and that the use of bone marrow stromal cells (BMSC) (156–158) or of an engineered herpes virus (159) may be new alternatives for OFP control.
In an effort to create an assay that induces pTMD in manner that better emulates the multifactorial etiology of these conditions in humans, a study combining a pTMD predisposing factor—inflammation induced by the injection of carrageenan in the TMJ or sustained inhibition of the catechol-O-methyltransferase (COMT) enzyme, classically known for its role in chronic pain (160–162), including OFP (163, 164)—with a precipitating factor—jaw forced lengthening—found that the combination of COMT inhibition with jaw forced lengthening was able to extend the time rats needed to chew through obstacles—a proxy measure of OFP sensitivity—compared to exposure to each factor alone after 2 weeks (78). The assay's ability to produce longer lasting OFP remains to be demonstrated. Nevertheless, the concept underlying the development of this assay is innovative and can lead to the development of construct valid animal assays of pTMDs.
TN is a very painful condition with severe, short-lasting, spontaneous and innocuous stimulus-evoked stabbing pain attacks in the face for which the development of more efficacious treatment options is highly warranted. Recently proposed taxonomies classify primary TN into classical or idiopathic TN (165, 166). Classical TN is characterized by the demonstration (in MRI or surgery) of vascular compression with morphological changes of the trigeminal nerve root entry zone (167–169). Its etiology seems to be linked to this neurovascular compression in some but not all cases (170, 171), as not all patients who undergo surgical decompression experience improvement (172). Proposed etiologies of idiopathic TN include genetic mutations in neuronal voltage-gated ion channel gain-of-function (173, 174), neural inflammation (175), and non-specific lesions in the brainstem (176–178). Centrally mediated pain facilitation and reduced descending pain inhibition are also non-confirmed contributors to TN pain (179–181). Existing assays in support of TN research are those that induce neurogenic pain via chemical injection or nerve injury (Table 2). The latter group, in particular assays that compress the trigeminal nerve root entry, seem to better approximate the pathophysiology of classical TN attributed to neurovascular compression.
This assay consists of exposing the infraorbital nerve (ION) at its rostral extent to inject cobra venom (or saline, for control) into the ION's nerve sheath (205). As consequence, animals display long-lasting (60 days) mechanical allodynia, spontaneous pain behaviors (e.g., increased face grooming and headshaking), fewer exploratory activities that is indicative of anxiety, and impaired spatial learning and memory function (182). Injection of cobra venom also leads to demyelination changes of the ION and medulla oblongata and was thus claimed to induce changes similar to those seen in classical TN (183). Treatment with pregabalin, a gabapentinoid believed to block calcium channels thereby reducing excitatory neuronal activity, improves different pain behaviors and suggests the neuropathic nature of pain resulting from the injection of cobra venom (183).
Although this assay clearly seems to induce neuropathic pain, whether the neurobiological mechanisms underlying pain are translatable to those of TN patients and relevant for the development of new therapies for patients is debatable.
Neuropathic pain, such as that seen in TN patients, is defined by pain caused by a lesion or disease of the somatosensory nervous system (206). With a seminal paper published in 1980 (207), nerve injury assays in support of TN research constrict (ligate) or compress the ION or trigeminal nerve to induce pain. Constriction or compression of the ION consistently leads to chronic pain and mood alterations that are consistent with the clinical presentation of chronic pain patients. However, there are important limitations to methods that employ chromic suture to ligate or compress nerves, as the resulting pain may be at least partially due to chromic-induced changes in the chemical milieu around the nerve (208).
While assays of nerve transection to branches of the trigeminal nerve also exist (209, 210), they are likely more suitable to the study of mechanisms underlying pain in post-traumatic neuropathies due to, for instance, motor vehicle accident, sporting injury, or dental procedures. Transection or lesions to the TN are not putative contributors to primary TN and these assays will not be describe here.
The CCI-ION (184) is the most widely used assay to induce neuropathic pain in the orofacial region with 167 citations on PubMed and has been adapted from the assay that induces a CCI to the sciatic nerve of rats developed by Bennett and Xie in 1988 (211). In this assay, following a midline scalp incision, part of the skull and nasal bone are exposed allowing access to the edge of the orbit. Orbital contents are then deflected, and the ION—a purely sensory nerve—is dissected free at its most rostral extent just caudal to the IO foramen. Two chromic gut ligatures 2 mm apart that reduce the diameter but do not occlude circulation are then loosely tied around the ION. Animals that undergo ION-CCI display increased face-grooming and freezing-like behavior, concomitant with a decrease in exploratory behavior that peak after surgery but remain different from controls for more than 4 months. Mechanical allodynia displays delayed onset and persists for approximately 80 days. Notably, animals also display extraterritorial pain that extends to the contralateral side of injury, an indicative of the involvement of central sensitization mechanisms that is believed to play an important role in chronic pain states, including OFP (212–214).
Studies employing the ION-CCI model have contributed to our understanding of how the trigeminal nerve responds to injury and have highlighted its uniqueness. As an example, dorsal root ganglion sympathetic sprouting that is seen post sciatic nerve injury is absent in the trigeminal ganglion (215, 216). Furthermore, CCI to the sciatic or ION induce differential gene expression in the dorsal root or trigeminal ganglia, respectively (217). These findings show important differences in the mechanisms of spinal vs. trigeminal pain and underscore the need to develop animal assays that are specific to TN.
Modifications to the ION-CCI assay have been proposed mainly to simplify the complexity of the required surgical procedure (185, 186). Examples include the chronic constriction injury of the distal ION (dIoN-CCI) (187). This leads to ipsi- and contralateral mechanical allodynia that lasts at least 9 weeks and is accompanied by anxiety-like behaviors that last at least 6 weeks (188). Constrictive assays were also developed for mice but their ability to produce long-lasting hypersensitivity and/or pain-like behaviors (185, 186) remains to be demonstrated.
Originally developed in rats, performing the ION-CCI assay in mice is challenging due to the small operating space and abundant blood supply in their facial area. The trigeminal inflammatory compression (TIC) of the ION assay was developed in response to this shortcoming (193, 194). In this model (193), a 15-mm antero-posterior incision is made at the facial midline and the infraorbital muscle is dissected from the bone to allow a gentle contraction of the orbit. This allows the visualization and dissection of the ION at its most rostral extent in the orbital cavity and the placement of a 2 mm chromic gut suture (6–0) between the ION and the maxillary bone. As consequence, mice display a slight peripheral nerve demyelination accompanied by mechanical allodynia that lasts for at least 21 weeks (195). Cold but not heat-induced allodynia lasts at least 8 weeks (196–199). In addition, ION-TIC mice also display anxiety-and depression-like behaviors, a consistent feature of chronic OFP patients (162). The same research group recently developed the FRICT-ION (Foramen Rotundum Inflammatory Constriction Trigeminal Infra-Orbital Nerve) assay, in which the ION is more easily accessed using an intra-oral approach (200). The main advantage of this assay is that it does not generate any outward physical signs, allowing for blinded pre-clinical studies. This assay leads to spontaneous pain behaviors and ipsi- and contralateral mechanical allodynia that last at least 100 days. Anxiety and depression-like behaviors also develop within 3–6 weeks.
This assay was developed in response to clinical evidence that neurovascular compression of the trigeminal nerve root plays a role in the pathogenesis of TN (218–220). First, a small curved and antero-posterior skin incision is made above the rat's eye, allowing the fascia and muscles to be laterally dissected free and the lateral retraction of the contents of the orbit. As result, the ION can be visualized deep within the orbit on the infraorbital groove of the maxillary bone. Based on the determination that the distance between the inferior orbital fissure to the proximate junction of the trigeminal nerve root with the pons is of approximately 1.5 cm in adult rats, a round plastic filament with 0.1 cm in diameter is placed above the ION superior surface and inserted for approximately 1.2 com into the intracalvarium through the inferior orbital fissure (not to reach/damage the pons). The filament is inserted into the canal between the cerebral dura mater and the pars petrosa (temporalis bone) to cross the Meckel's cave and reach the trigeminal nerve root. Animals subjected to CCT, but not sham-operated animals, develop mechanical allodynia that lasts at least for 4 weeks, and exhibit face-grooming behavior consistent with OFP for 3 weeks (201, 202). Both CCT and sham-operated animals develop heat-hypersensitivity over the course of 4 weeks (201). Activation and increase in the numbers of Schwann cells, astrocytes (i.e., A1-astrocytes) and microglia/macrophages, as well as an increase in the infiltration of macrophages and lymphocytes in the trigeminal root zone are also seen 4 weeks following CCT, suggesting the contributions of neuroimmune cells to the pathogenesis of TN attributed to neurovascular compression (203, 221).
A method using crystals of a superabsorbent polymer placed next to the rat's trigeminal nerve root has also been developed but its ability to induce pain hypersensitivity and pain-like behaviors remains to be demonstrated (222).
In this assay (203), rats are mounted into a stereotaxic frame and a 21 gauge cannula is inserted into the trigeminal ganglion for the injection of 8 µl of a 4% agar solution. Control (sham-operated) animals receive all surgical procedures without agar injection. Animals with the compressed trigeminal ganglion exhibited mechanical allodynia and hypersensitivity that lasted up to 40 days and longer than those of naïve or sham-operated animals. However, there was no evidence of TN-like paroxysmal pain in animals with the compressed nerve, as there were no group differences in the number of facial grooming episodes exhibited. Further studies employing this assay to induce TN-like pain have suggested that NMDA receptors, particularly their NR-2 subunits, play an important role in the central processing of TN pain and are potential targets for the development of new therapies for TN (223).
This assay was developed based on evidence from classical studies that proposed a causal relationship between TN and focal demyelination due to compression of the trigeminal nerve root (224, 225). To induce focal demyelination, lysophosphatidic acid (LPA; 3 μl; 1 nmol) is injected into the trigeminal nerve root of anesthetized and restrained rats. Control animals receive all surgical procedures with the injection of vehicle instead of LPA. Injection of LPA produced severe demyelination of the axonal portion of the trigeminal nerve root for at least 2 weeks that was completely recovered by day 160. Despite the recovery of the myelin sheath, mechanical allodynia persisted for approximately 130 days and mechanical hypersensitivity (i.e., hyper-responsiveness to pin-prick stimulation) lasted for approximately 100 days. LPA injection produced no effect in thermal pain sensitivity. The display of nocifensive behaviors that would represent a proxy measure of TN-like paroxysmal pain has not been investigated (204).
BMS is a painful condition often described as a burning, scalding, or tingling feeling in the mouth that may occur every day or intermittently for months or longer. Dry mouth and an altered taste in the mouth typically accompany the pain. Like other chronic primary pain conditions, the etiology of BMS is believed to be multifactorial (226). Clinical research over the last two decades showing small-fiber atrophy in the tongue and increased expression of TRPV1 and purinergic receptors (P2X3) ion channels in the epithelium of the lingual mucosa of BMS patients suggest a neuropathic component in BMS (227–230). As for primary pTMDs and TN, the incomplete knowledge of the etiology of BMS makes developing a translatable preclinical model challenging and there are currently no definitive animal models of BMS. Nevertheless, assays that induce oral symptoms resembling those of BMS patients have been put forward and will be discussed here (Table 3), as they may be useful in the investigation of the pathogenesis of BMS. Methods involving nerve transection that is not a factor at play in BMS have also been developed (231). The utility of these assays for shedding light into the underlying mechanisms of BMS is debatable and they will not be described here.
This BMS mouse model was inspired by findings showing the increased expression of artemin, a member from the glial cell line-derived neurotrophic factor (GDNF) family, in the inflamed skin of wildtype mice and that this overexpression leads to hypersensitivity to noxious thermal stimuli, to increases in the expression of the pronociceptive ion channels TRPV1 and TRPA1 in neurons of the dorsal root ganglia, and to an increased heat-induced firing of C-fibers (234). These findings led to the creation of ART-OE mice that overexpress artemin in the tongue epithelium with the goal of assessing whether it would induce oral sensitivity. Trigeminal afferents of adult ART-OE mice display changes in their anatomical, physiological, and transcriptional properties that include hypertrophy of myelinated and unmyelinated fibers of the lingual nerve, and marked increases in the expression of TRPV1, TRPA1, and GDNF-family receptor alpha 3 (GFRα3; a receptor component for artemin) (232). These mice also reduced their consumption of water containing either capsaicin (a TRPV1 ligand) or mustard oil (a TRPA1 ligand) compared to wildtypes, a behavior consistent with increased oral sensitivity.
In accordance with the clinical findings of small-fiber atrophy in the tongue (227) and increased expression of TRPV1 in the epithelium of the lingual mucosa of BMS patients (228), this suggests that GFRα3/TRPV1 and artemin-responsive fibers are possible contributors to BMS and that ART-OE mice may be a suitable model for future investigations of the mechanisms underlying BMS.
This assay was created as consequence of earlier studies showing a role for artemin in pain (233, 235), including in tongue pain (232), and clinical findings of a near 3-fold increase in the expression of artemin in the tongue of BMS patients (233). To emulate this increased expression of artemin in the tongue of mice, TNBS that is known to increase artemin expression in oral mucosa tissues (236) is applied topically to the tongue of mice. In specific, a cotton saturated with a TNBS 10 mg/ml suspension (or vehicle, for controls) is placed on the dorsum of the tongue for 1 h. As result, mice develop heat hyperalgesia that lasts at least 11 days and is inhibited by treatment with a TRPV1 antagonist or with an anti-artemin antibody (233). A longer follow-up of heat sensitivity has not been done, but heat sensitivity thresholds seem to be approaching baseline levels starting on day 11 and the ability of this assay to induce long-lasting tongue pain remains to be demonstrated. Notably, TNBS induced tongue pain in the absence of inflammation or nerve damage, consistent with what is seen in BMS patients. Following the induction of artemin expression in the tongue with TNBS, the number of trigeminal ganglion neurons expressing GFRα3 and TRPV1 increased and current induced by capsaicin in trigeminal ganglion neurons enhanced. These phenotypes were reversed upon treatment with an anti-artemin antibody.
These finds reinforce a role for artemin in tongue pain and may suggest that topical application of TNBS a may be useful for the study of tongue pain hypersensitivity associated with BMS and may be particularly relevant for the understanding of pain flare ups of chronic patients.
Following orofacial pain induction by means of the selected assay, the resulting pain must be assessed to confirm the desired phenotype (i.e., ensure that the assay produced pain), and to allow the quantification of pain and comparisons pre- and post-treatment and/or between groups. Assessing pain in rodents is more challenging than in humans, given the impossibility of verbal expression. Because of that, pain is inferred based on measures of pain-associated behaviors that can be evoked (i.e., reflexive behaviors in response to mechanical and thermal stimulation), non-evoked (i.e., changes in nocifensive, survival, and elective behaviors), or mixed (e.g., conditioned place preference, conditioned place aversion, and operant behaviors). Evoked behaviors are those elicited when an exogenous stimulus is applied by an experimenter at the time of measurement. Non-evoked behaviors are those elicited without an exogenous stimulus being applied by an experimenter at the time of measurement and are often referred to as spontaneous behaviors. Measures of mixed behaviors were developed to overcome issues related to the reflexive and innate nature of commonly assessed evoked and non-evoked pain measures, respectively. These issues relate to the over-reliance of evoked and non-evoked measures on simple spinal reflexes (that can even be evoked in decerebrate animals) (237) that do not require cerebral processing of nociception (238–240). Conditioning and operant behaviors are indirect measures of pain that involve higher levels of brain processing and require animals to make specific choices based on learning processes (as do humans) (241). Here, we describe the pain-associated behaviors used to infer OFP in rodents.
Originally developed for assessing tactile sensitivity in humans (242, 243), von Frey filaments are widely used for measuring mechanical sensitivity in the skin of rodents. Von Frey filaments consist of a series of fibers (typically nylon) of equal length and gradually increasing diameter that are individually and perpendicularly attached to a hand-held base. Von Frey tests rely on the fact that the force (in grams) required to lightly bend each filament increases as the filaments' diameter increase. In the ascending/descending technique (184) developed for the orofacial region, following habituation, rats are individually placed in a small transparent cage to allow video recording and presented with a series of von Frey stimuli exerting forces of 2 gm, 9 gm, 16 gm, 4 gm, and 1 gm. The animal's response to each stimulus is scored from 0 to 4: 0, when the animal has no response to the stimulus; (1) when it detects the filament but displays no aversive behavior; (2) when it withdraws the head as an indication of a mild aversiveness to the filament; (3) when it escapes or attacks the filament as an indication of strong aversiveness; or (4) when it displays an uninterrupted series of at least three facewash strokes directed to the stimulated facial area as an indication of prolonged aversiveness. A mean score can then be calculated by averaging the response scores of each of the five stimuli.
The ascending technique (53) was sequentially proposed to overcome the potential for bias due to the subjectivity in the scoring system of the ascending/descending technique (184). Here, following a habituation period during which rats are trained to rest their heads on the experimenter's hand, testing starts with applying a small-diameter filament (typically 0.192 g for rats and 0.045 g for mice) five times perpendicularly to the skin over the masseter/TMJ region with a few seconds of interval. A response to the filament is defined by a head withdrawal in at least three of the five stimulations. If no response, the next thicker filament is used until the response threshold can be detected. The response threshold is defined as the lowest force needed to produce at least three head withdrawal responses in five stimulations. Alternatively, the response frequencies to a range of von Frey stimuli can be computed [(number of responses/number of stimuli) × 100%] and a stimulus-response frequency curve plotted. The EF50, defined as the von Frey filament force (g) that produces a 50% response frequency, can then be calculated using regression analysis and used as a measure of mechanical sensitivity (244): a smaller EF50 indicates greater sensitivity. The ascending technique, as originally described or with modifications (76, 156, 190, 246, 247) that include the use of electronic filaments (94, 247–251), is likely the most widely used to measure mechanical orofacial hypersensitivity in rodents, including in the tongue (252).
To assess OFP in an objective manner that better represents the patients' cardinal symptoms, a few methods have been developed to measure mice bite force as a proxy measure of orofacial mechanical allodynia. This is supported by clinical studies showing that bite force is significantly reduced in chronic OFP patients (253, 254). In the first of such methods developed for rodents (52, 255), rats were maintained on a water-restriction schedule and trained to deliver gradually increasing bite forces with the aid of a computerized system that triggered the delivery of water upon biting. Only successful bites (strong enough to reach the gradually increasing thresholds) resulted in water delivery. Rats can reportedly be trained to achieve the final target of 1.3 kg within 3–4 weeks. Post-training reductions in bite force (e.g., following CFA injection in the masseter) can be prevented by anti-inflammatory agents (52) and are indicative of orofacial mechanical allodynia.
A method has also been developed to assess bite force in mice with the advantage that because they exhibit aggressive voluntary biting, a training phase is not needed. In this method, mice are individually placed in a cylindrical tube with an opening at one end for accommodation of their head and a custom-made bite transducer is slowly moved towards them, naturally eliciting a bite. The bite force transducer consists of two parallel and 4.5–5 mm apart beams which deformation (due to biting) results in a proportional change in resistance that can be measured (in N or g) and represents the bite force. Reductions in bite forces can be prevented by anti-inflammatory agents and are indicative of greater pain sensitivity (47, 51, 77, 256). Variations of this method also exist but follow the same principle: reduced bite force equals increased pain sensitivity (257).
Heat hypersensitivity has been assessed mainly in studies of neuropathic orofacial pain or tongue pain. In one of the earliest studies (190), a device to loosely restrain rats while allowing access to the rat's snout was designed. The device allows animals to easily withdraw or flick their snouts. For the test, a radiant heat stimulus that raises skin temperature above 45°C within 8.5 s is placed 10 cm from the stimulation site (vibrassal pad). A cut-off time is pre-determined to prevent tissue damage. Head withdrawal or snout flicking is detected by a photocell that terminates the stimulus and stops the timer, which defines the head withdrawal latency. Latency is determined three times on each side with 2 min intervals between tests, with shorter latency being suggestive of heat hypersensitivity. Slight variations of this method also exist (201, 258–261).
Thermal hypersensitivity can also be measured by applying a small thermal probe to the animal's vibrassal pad (189, 262, 263). For this, animals habituated to being restrained by the experimenter and to being presented with the probe at room temperature are presented with the thermal probe at higher temperature. A cut-off time is pre-determined to prevent tissue damage. Repeated trials with interval in between them are typically done to determine the animal's average withdrawal latency (i.e., time until they withdraw or flick the snout). Similar methods have also been developed to assess thermal hypersensitivity in the tongue (233, 252).
Nocifensive behaviors are time-limited involuntary rodent responses to the administration of an algogen (e.g., formalin, capsaicin) to a body part, such as the TMJ, masseter muscle, or vibrassal pad (193, 264). Moving the mandible in a chewing-like motion, rubbing the orofacial region, or flinching the head are examples of commonly measured orofacial nocifensive behaviors (29, 41, 265). Typically, following a habituation period, animals are placed in a test box/cage where they can be watched/recorded while moving freely. Results are expressed as the total amount of time spent doing a specific behavior (31) or as the number of specific behaviors counted over a pre-determined period (33). The amount of time/number of behaviors displayed are positively correlated with pain, and treatment with analgesic drugs inhibits the behavior (31).
Like humans, animals also can demonstrate pain via facial expressions (266). Scoring facial features associated with pain in rodents (i.e., orbital tightening, changes in ear position, nose/cheek aspect, and whisker direction) is another measure of orofacial nocifensive behavior (267, 268). This is done using grimace scales that have been developed for both mice and rats (269, 270). For that, following acclimation, one animal at a time is placed in a partially transparent cage and filmed before and after the injection of an algogen. A set of baseline and post-injection photographs is then presented to independent scores who rate each photo: a score of “0” indicates high confidence of the scorer that no facial feature associated with pain was present; “1” indicates either high confidence of a moderate appearance of a facial feature associated with pain, or equivocation over its presence or absence; “2” indicates high confidence that a facial feature associated with pain was present. Baseline and post-injection scores are averaged, and results are typically expressed as the mean change in score, with greater changes being indicative of more pain. This process can now be automated to eliminate human error and provide an objective, reliable, and rapid way of quantifying spontaneous pain and pain relief in mice (271).
Feeding is an activity of primary importance to the animal's survival that involves actions of the TMJs, masticatory muscles, and tongue. Different aspects of feeding have been measured to infer OFP under the assumption that pain in the orofacial structures negatively affects feeding. Pain-associated feeding behaviors include food intake and meal pattern (an indirect feeding measure). Food intake is usually given by tracking the amount (in g) of food consumed over a period, corrected for spillage (54, 55, 272). Reduced food consumption is taken as a proxy measure of pain, as it suggests reduced engagement of orofacial structures. Meal pattern, which requires using a computerized strategy to track meal size, meal duration, and interval in-between meals, is a complex feeding measure which results seem to correlate to the intensity of the injury (54, 272).
Grooming is another activity of primary importance to the animal's survival and encompasses all forms of care and attention to the body surface (273). Facial grooming behaviors include facial rubbing, lower lip skin/cheek rubbing or facial scratching. Measuring facial grooming involves, following habituation of the animals to the test environment, placing them in a transparent or partially transparent cage where they can be recorded while moving freely. Results are expressed either as the total amount of time spent face-grooming (191, 201) or as the number of face-grooming episodes over a pre-determined period (187). The amount of time and number of face-grooming episodes are positively correlated with pain, and treatment with analgesic drugs inhibits the behavior (187, 274).
Developing operant tests is challenging due to the difficulty in generating behaviors that are indicative of OFP after cerebral processing. These tests offer the advantage of avoiding the stress that is likely invariably experimented by animals in experimenter-initiated tests, which can affect pain-related outcomes (265, 275). Here we describe the operant assays that have been developed specifically to assess OFP.
To assess operant thermal pain behavior and characterize orofacial heat pain sensitivity (63, 66, 192, 276), unrestrained rats or mice are placed in a cage with acrylic walls containing an opening in one wall lined with grounded metal tubing that serves as thermode. A spouted watering bottle filled with diluted sweetened condensed milk (reward) is placed just outside the opening, allowing animals to access to the reward through the spout when simultaneously contacting the thermode with their face. Following sessions in which animals are trained to obtain the reward while contacting the thermode at room temperature, temperature is increased and the data acquisition system is activated. The system records simultaneous spout and thermode contact as a “licking” event, which can be interpreted as a successful attempt at the reward; thermode contact only as a “facial contact” event, which can be interpreted as a failed attempt at the reward; and the duration of each facial contact. The amount of reward consumed is also tracked by the experimenter. The licking/facial contact events and facial duration/facial contact event ratios, the cumulative facial contact duration and reward intake can then be calculated and provide indirect measures of orofacial thermal pain sensitivity.
Using cold thermode temperatures, this method can also be used to assess cold hypersensitivity (277). Importantly, these operant measures reflect the animal's choice to obtain the reward despite the painful thermal stimulation, which involves learning cerebral processes.
The dual operant thermal and mechanical pain behavior employs a modification to the system described in the above subsection (Section 3.3.1.1) by simply adding a mechanical component (67). In specific, nickel titanium wires (0.010 or 0.007 inches in diameter) are attached horizontally to the cage opening that allows access to the spouted watering bottle filled with diluted sweetened condensed milk (reward). Thus, access to the reward requires simultaneous contact with the wires. Similar to the operant thermal pain behavior, the system records “licking” events, “facial contact” events and the duration of each facial contact. The licking/facial contact events and facial duration/facial contact event ratios, the cumulative facial contact duration and reward intake can then be calculated and provide indirect measures of orofacial mechanical pain sensitivity. This dual system allows investigators to directly assess and compare mechanical vs. thermal pain using the same outcomes.
The same group who developed the operant thermal pain test and the dual operant thermal and mechanical pain test, sequentially developed the operant mechanical pain threshold test (64). The new test consists of placing animals in a cage that contains at one end a round aperture with a 360° array of looped 0.010 in stainless steel wires with a 0.7 in opening at the center that partially blocks access to a spouted watering bottle filled with diluted sweetened condensed milk (reward). Initially, the bottle spout is aligned with the array of wires so that initial contact with the bottle does not require contact with the mechanical stimulus. Upon contact with the bottle, an electric circuit is established triggering a motor that slowly moves the bottle away from the cage, requiring the animal to tolerate greater mechanical force to continue to receive the reward. This process is repeated five times or until 10 min of testing time elapse. The average tolerance distance can then be calculated for each testing day for each animal, with greater distances being indicative of reduced mechanical pain sensitivity. The main advantage of this test to the previously developed the dual operant thermal and mechanical pain test is that it allows the quantification of each animal's withdrawal threshold.
In the operant feeding behavior test (56), rats are trained to press on a bar four times to obtain one 45 mg food pellet. At the time of testing, the times of each reward obtained during a one-hour session and the inter-feeding interval are recorded. Results are shown in a histogram of feeding behavior with inter-feeding intervals binned at 5 s intervals, normalized to the total number of pellets consumed. A right shift in the distribution indicates food was consumed with larger inter-feeding intervals and suggests increased orofacial pain.
A hallmark of OFP conditions is functional pain, such as pain during mastication (278–281). The dolognawmeter, a device that allows the quantification of mice gnawing time (52), was developed considering that other operant tests assess noxious cutaneous stimulation rather than masticatory dysfunction. The dolognawmeter is a device designed to fit into a standard mouse cage and essentially consists of a 24 mm. internal diameter tube through which are placed two dowels part by 20 mm. Once a mouse is loaded into the dolognawmeter, an endcap is placed, and the mouse finds itself confined anteriorly by the series of two dowels. The mouse instinctively gnaws through them to exit the tube. Upon severing the first dowel, pistons retract it laterally and automatically start a timer that is stopped once the second dowel is severed. The amount of time taken to gnaw through the second dowel is an index of OFP: greater gnawing-time reflects increased OFP pain and can be brought back to baseline levels upon administration of analgesic drugs. Of note, mice use their front incisors to gnaw through the dowels and their tongue to remove debris from the mouth. This is different from humans to the extent that humans employ their molars for chewing. The dolognawmeter has been used mainly to study OFP linked to oral cancer (282–284) and has thus far been under-used in the field of non-cancer OFP (285). A modified dolognawmeter to test rats (the ratgnawmeter) has also been developed (78).
Oral sensitivity, including tongue sensitivity, may be assessed based on drinking behavior. This test, modified from the paired-preference drinking aversion paradigm (286, 287), consists in attaching one bottle with water plus vehicle and another with capsaicin (1 µM) or mustard oil (100 µM) to the cage of mice housed individually for three days. Following 24 h during which animals have access to the two bottles (and to food) ad libitum, the volume consumed is recorded. At the end of each day, the bottle positions are changed to ensure findings are not due to a place preference. The average volume consumed over three days of testing and the ratio of capsaicin or mustard oil intake vs. total liquid intake (percentage) is then calculated and used as measures of oral sensitivity (i.e., lower volume and/or ratio are indicative of increased oral sensitivity) (232).
It is hard to envision the much-needed advance in our comprehension of the etiological and pathophysiological mechanisms of chronic primary pain conditions and the consequent development of new drug therapies and treatment strategies to prevent and treat them without the support of pre-clinical research. For the success of this endeavor, it is imperative that appropriate pain assays and measures are selected to adequately address a study's goals. Another important aspect that requires careful consideration is the selection of study subjects (i.e., animal species, strain, sex, age, etc.). Previous reviews have discussed factors to consider in the selection of appropriate study subjects (239). In this review, we have presented the currently available pain assays and measures in support of chronic primary OFP, in particular pTMDs, TN, and BMS. Understanding how the current knowledge on these conditions has been constructed is important to enable the development of methods that will allow us to optimize the translation of findings into better OFP care.
Chronic primary pain conditions have seemingly idiopathic etiology and complex pathophysiology and there is robust scientific evidence that both processes are multifactorial and varied at the individual level (11, 17). It is thus challenging to envisage that a single preclinical pain assay might be necessary or sufficient to engage, in animals, etiological and pathophysiological mechanisms similar to those leading to the development and maintenance of pain in humans. Single assays, in particular those relying on an inflammatory or neuropathic injury, may at best represent environmental factors that produce nociceptive afferent inputs into the central nervous system (CNS) needed to initiate the painful state. An overwhelming majority of preclinical OFP research is, however, based on single assays. While these studies have been and will continue to be important to reveal critical inflammatory, neural, and immune aspects of OFP transmission and processing, their findings likely represent only an isolated fragment of the mechanisms involved in chronic pain. Hence, one of the current challenges to be overcome by preclinical OFP research is the development of integrated assays with greater construct validity that could potentially trigger the engagement of pain mechanisms paralleled in humans. Accordingly, a study has recently shown that OFP develops and is maintained for at least 2 weeks as result of the exposure of animals to a combination of assays mimicking a predisposing (i.e., pharmacological inhibition of COMT) and a precipitating pTMD factor (i.e., jaw forced lengthening) (78). Similar examples for idiopathic TN or BMS assays are not currently available.
The measures employed to infer OFP in rodents is another component of preclinical research design that requires careful consideration to enhance the translatability of findings. It would be ideal that the phenotype (i.e., a subject's observable traits) created by virtue of the selected OFP assay emulates as best as possible the symptoms linked with the disease of interest. In addition to the cardinal symptom of spontaneous (and often oscillating) pain, pain that is modified by jaw function (e.g., chewing hard food, laughing, yawning, resting the jaw) is a hallmark of pTMDs (278–281). However, preclinical studies investigating aspects of pain in the TMJs and masticatory muscles focus mainly on nocifensive behaviors and mechanical pain sensitivity measures. While the former has the advantage of being spontaneous (i.e., non-evoked) behaviors, they are elicited in response to the injection of an algogen and are short-lasting in nature. Conceivably, nocifensive behaviors are practical measures of acute pain sensitivity but do not sensibly represent chronic OFP symptoms in humans. Mechanical pain sensitivity is mostly assessed using von Frey filaments and seem to have face validity as a measure of pain evoked by palpation. Assessing palpation pain in the TMJs and masticatory muscles is an integral part of a pTMD clinical examination for adequately rendering a diagnosis [e.g., a myalgia diagnosis requires the patient to report that the pain elicited by palpation resembles the pain they experience outside the clinical setting, a.k.a. familiar pain (12)] and assessing severity and prognosis (288). Nonetheless, evoked pain is not the chief complaint of pTMD patients and their reason for seeking care. Furthermore, it has been speculated that von Frey tests assess noxious cutaneous stimulation rather than mechanical sensitivity. In the inability to communicate pain, it seems like preclinical measures of masticatory function, such as bite force (52, 51, 77, 255–265), feeding (54, 55, 272), and the operant gnawing tests (52, 78), would be more sensible measures of OFP resembling that of pTMD patients. Assessing these measures, however, does not come without challenges and disadvantages, which include the necessity of (sometimes extensive) animal training and of specific devices and computational systems to enable testing.
There are also no construct-valid animal assays that lead to behaviors that sufficiently mimic TN (i.e., long-lasting display of spontaneous pain attacks). It is commendable that many studies employing assays that compress the trigeminal nerve in a manner that likely partly parallels the pathophysiology of classical TN (167–169, 218–220, 224, 225) assess and show OFP for long periods of time lasting at least one month (201–204). These are, however, assessments of mechanical and/or thermal hypersensitivity and there is no reported evidence that these assessments trigger pain attacks. Pain attacks in TN patients are often triggered by innocuous stimuli, such as touching the face, brushing the hair, or simply stepping out into colder temperatures. Hence, it would be expected from a face-valid animal model of TN that the mechanical or thermal stimuli such as those delivered, for example, in von Frey or in thermal pain tests, would trigger pain attacks. The assay that injects cobra venom into the ION to induce TN seems to have greater face-validity despite lacking construct-validity, as injected animals display greater face-grooming behavior that could be indicative of spontaneous pain attacks compared to controls for at least 30 days. Going forward, it is important that pre-clinical studies of TN assess behaviors that could demonstrate the paroxysmal nature of pain, which could include, for instance, freezing behaviors in addition to face-grooming. Freezing is an instinctive behavior in animals to avoid physical pain (289) and is commonly assessed in pre-clinical migraine/headache research to infer pain (290) that would likely be informative for TN research.
It is also important to reiterate a previously voiced concern regarding the lack of specificity of assays supposedly relevant for TN, as the same assays (especially those compressing the infra-orbital nerve) are also used to induce painful trigeminal neuropathy. These are two distinct conditions both in terms of clinical presentation and evidence-based treatment and it is unlikely that the same assay would validly recreate pathophysiological mechanisms simultaneously relevant for both conditions (291).
Burning pain that can feel like one has scalded their mouth (more often in the tongue but may also affect gums, lips, the inside of cheeks, palate, and/or widespread areas in the mouth) is the main complaint of patients with BMS (292). Although a neuropathic component has been suggested for BMS (227–230) and introduced into animal assays (232), this condition also is believed to be multifactorial (226). Creating a rodent assay that better approximates the pathophysiology of BMS and results in a phenotype consistent with its clinical manifestations—in terms of both chief complaint and chronicity—will likely require combined approaches. Another challenge in BMS research is the development of methods that assess burning pain in a translatable manner. Acidic foods and liquids, such as tomatoes, orange juice, carbonated beverages, and coffee, typically worsen the burning sensation. A possibility could be to invest in the development of measures that assess the intake of these commonly consumed products as opposed to water containing potent TRPV1 and TRPA1 and ligands (e.g., capsaicin and mustard oil, respectively). Notably, a recent study showing that nociceptive nerves are required for enforced hematopoietic stem cells mobilization has developed a method in which mice are fed with spicy (capsaicin-infused) chow without affecting their daily food intake or body weight compared to regular chow that could potentially be useful as an outcome measure in pre-clinical BMS research (293).
Of note, this review does not wish to make a statement that measures of mechanical and thermal pain sensitivity are not adequate or needed to demonstrate a painful phenotype. In fact, pTMD (109, 214, 294, 295), TN (182, 297, 298), and BMS (298–301) patients are more sensitive to experimental mechanical and thermal pain locally and often in other parts of the body. Hence, pairing face-valid measures of OFP sensitivity with complementary measures of pain sensitivity is not only needed for a comprehensive phenotypic characterization but is also likely informative of the disease pathophysiology.
The multifactorial etiology and complex pathophysiology of chronic primary OFP conditions is a challenge to the translatability of pre-clinical research. The development of models in which long-standing pain results from the exposure to biological, psychological, and environmental factors seems to be required if we are to better understand the mechanisms that sustain pain and to develop treatment strategies that target these mechanisms. A recent study reports that mice with genetic background susceptible to pain (i.e., transgenic mice expressing reduced COMT levels) that are not more sensitive to pain at baseline develop pain in multiple body sites following combined exposure to stress (i.e., swim stress) and to an OFP-relevant environmental factor (i.e., surgery to remove lower molars bilaterally) that lasts more than 3 months and is of greater magnitude in females (302). This multiple assay-based animal model of chronic primary pain seems to be a step in the right direction and could be used to inspire new pTMD, TN, and BMS pre-clinical models.
In the abovementioned multiple assay-based animal model of chronic primary pain, animals also displayed depressive-like behaviors for longer than 3 months. Measures of psychological well-being are additional complementary measures that should be assessed to fully characterize OFP-related rodent phenotypes, as well as to assess pain duration and response to treatment. It is well-documented that chronic OFP patients, including those living with pTMD (25, 303, 304), TN (305, 306), and BMS (307–310), display a complex array of symptoms that includes psychological comorbidities and poorer quality of life. Naturally occurring elective behaviors such as grooming, playing, socializing, and nest building are increasingly accepted as indicators of rodent well-being (311–314). Equivalent behaviors in humans include social interactions and participation in physical activities, which are negatively affected by chronic pain (315–317), including pTMDs, TN, and BMS (26, 306, 319). Hence, complementing the study design with measures of elective behaviors may be crucial to assess the entirety of an animal's orofacial pain experience.
A plethora of preclinical assays have been used and have been helpful to advance our understanding of the mechanisms of OFP. Combined with evidence originating from studies in humans, it is now time to put effort into developing construct-valid OFP assays and face-valid OFP measures that may help uncover pathophysiological aspects of chronic OFP and enable the development of drug therapies and treatment strategies that may survive the translational ladder. In this regard, this review hopes to foster the development of innovative animal models with greater translatability and potential to lead to better care for patients living with chronic primary OFP.
SS has performed the literature review and written the manuscript. FA-H has contributed to manuscript writing and editing; RS-N contributed to conceptualization and editing of the article. CM has conceptualized and edited the article. All authors contributed to the article and approved the submitted version.
The authors declare that the research was conducted in the absence of any commercial or financial relationships that could be construed as a potential conflict of interest.
All claims expressed in this article are solely those of the authors and do not necessarily represent those of their affiliated organizations, or those of the publisher, the editors and the reviewers. Any product that may be evaluated in this article, or claim that may be made by its manufacturer, is not guaranteed or endorsed by the publisher.
1. International classification of orofacial pain seI. Cephalalgia. (2020) 40(2):129–221. doi: 10.1177/0333102419893823
2. Shueb S, Nixdorf DR, John MT, Alonso BF, Durham J. What is the impact of acute and chronic orofacial pain on quality of life? J Dent. (2015) 43(10):1203–10. doi: 10.1016/j.jdent.2015.06.001
3. Benoliel R, Zini A, Zakuto A, Slutzky H, Haviv Y, Sharav Y, et al. Subjective sleep quality in temporomandibular disorder patients and association with disease characteristics and oral health-related quality of life. J Oral Facial Pain Headache. (2017) 31(4):313–22. doi: 10.11607/ofph.1824
4. Haviv Y, Zini A, Etzioni Y, Klitinich V, Dobriyan A, Sharav Y, et al. The impact of chronic orofacial pain on daily life: the vulnerable patient and disruptive pain. Oral Surg Oral Med Oral Pathol Oral Radiol. (2017) 123(1):58–66. doi: 10.1016/j.oooo.2016.08.016
5. Ridgeway JL, Beebe TJ, Chute CG, Eton DT, Hart LA, Frost MH, et al. A brief patient-reported outcomes quality of life (PROQOL) instrument to improve patient care. PLoS Med. (2013) 10(11):e1001548. doi: 10.1371/journal.pmed.1001548
6. Almoznino G, Benoliel R, Sharav Y, Haviv Y. Sleep disorders and chronic craniofacial pain: characteristics and management possibilities. Sleep Med Rev. (2017) 33:39–50. doi: 10.1016/j.smrv.2016.04.005
7. McMillan AS, Wong M, Zheng J, Lam CL. Prevalence of orofacial pain and treatment seeking in Hong Kong Chinese. J Orofac Pain. (2006) 20(3):218–25.16913431
8. Macfarlane TV, Blinkhorn AS, Davies RM, Kincey J, Worthington HV. Oro-facial pain in the community: prevalence and associated impact. Community Dent Oral Epidemiol. (2002) 30(1):52–60. doi: 10.1034/j.1600-0528.2002.300108.x
9. Ng KFJ, Tsui SL, Chan WS. Prevalence of common chronic pain in Hong Kong adults. Clin J Pain. (2002) 18(5):275–81. doi: 10.1097/00002508-200209000-00001
10. Nicholas M, Vlaeyen JW, Rief W, Barke A, Aziz Q, Benoliel R, et al. The IASP classification of chronic pain for ICD-11: chronic primary pain. Pain. (2019) 160(1):28–37. doi: 10.1097/j.pain.0000000000001390
11. Cohen SP, Vase L, Hooten WM. Chronic pain: an update on burden, best practices, and new advances. Lancet. (2021) 397(10289):2082–97. doi: 10.1016/S0140-6736(21)00393-7
12. Schiffman E, Ohrbach R, Truelove E, Look J, Anderson G, Goulet J-P, et al. Diagnostic criteria for temporomandibular disorders (DC/TMD) for clinical and research applications: recommendations of the international RDC/TMD consortium network and orofacial pain special interest group. J Oral Facial Pain Headache. (2014) 28(1):6. doi: 10.11607/jop.1151
13. Isong U, Gansky SA, Plesh O. Temporomandibular joint and muscle disorder-type pain in US adults: the national health interview survey. J Orofac Pain. (2008) 22(4):317.19090404
14. Pleis J, Ward B, Lucas J. Summary health statistics for US adults: National health interview survey, 2009. Data from the national health interview survey. Vital and health statistics. Series 10, number 249. DHHS publication No.(PHS) 2011-1577. Hyattsville, Maryland: Centers for Disease Control and Prevention (2010).
15. Bendtsen L, Zakrzewska JM, Heinskou TB, Hodaie M, Leal PRL, Nurmikko T, et al. Advances in diagnosis, classification, pathophysiology, and management of trigeminal neuralgia. Lancet Neurol. (2020) 19(9):784–96. doi: 10.1016/S1474-4422(20)30233-7
16. Lamey P-J. Burning mouth syndrome. Dermatol Clin. (1996) 14(2):339–54. doi: 10.1016/S0733-8635(05)70361-2
17. Sessle BJ. Chronic orofacial pain: models, mechanisms, and genetic and related environmental influences. Int J Mol Sci. (2021) 22(13). 1–27. doi: 10.3390/ijms22137112
18. Russo M, Crafa P, Guglielmetti S, Franzoni L, Fiore W, Di Mario F. Burning mouth syndrome etiology: a narrative review. J Gastrointestin Liver Dis. (2022) 31(2):223–8. doi: 10.15403/jgld-4245
19. Mogil JS, Davis KD, Derbyshire SW. The necessity of animal models in pain research. Pain. (2010) 151(1):12–7. doi: 10.1016/j.pain.2010.07.015
20. Greco R, Demartini C, De Icco R, Martinelli D, Putorti A, Tassorelli C. Migraine neuroscience: from experimental models to target therapy. Neurol Sci. (2020) 41(Suppl 2):351–61. doi: 10.1007/s10072-020-04808-5
21. Begasse de Dhaem O, Wattiez AS, de Boer I, Pavitt S, Powers SW, Pradhan A, et al. Bridging the gap between preclinical scientists, clinical researchers, and clinicians: from animal research to clinical practice. Headache. (2023) 63(1):25–39. doi: 10.1111/head.14441
22. Mognetti B, Di Carlo F, Berta GN. Animal models in oral cancer research. Oral Oncol. (2006) 42(5):448–60. doi: 10.1016/j.oraloncology.2005.07.014
23. Meloto CB, Serrano PO, Ribeiro-DaSilva MC, Rizzatti-Barbosa CM. Genomics and the new perspectives for temporomandibular disorders. Arch Oral Biol. (2011) 56(11):1181–91. doi: 10.1016/j.archoralbio.2011.03.012
24. Woolf CJ. Central sensitization: implications for the diagnosis and treatment of pain. Pain. (2011) 152(3 Suppl):S2–15. doi: 10.1016/j.pain.2010.09.030
25. Fillingim RB, Ohrbach R, Greenspan JD, Knott C, Dubner R, Bair E, et al. Potential psychosocial risk factors for chronic TMD: descriptive data and empirically identified domains from the OPPERA case-control study. J Pain. (2011) 12(11 Suppl):T46–60. doi: 10.1016/j.jpain.2011.08.007
26. Ohrbach R, Fillingim RB, Mulkey F, Gonzalez Y, Gordon S, Gremillion H, et al. Clinical findings and pain symptoms as potential risk factors for chronic TMD: descriptive data and empirically identified domains from the OPPERA case-control study. J Pain. (2011) 12(11 Suppl):T27–45. doi: 10.1016/j.jpain.2011.09.001
27. Gatchel RJ, Peng YB, Peters ML, Fuchs PN, Turk DC. The biopsychosocial approach to chronic pain: scientific advances and future directions. Psychol Bull. (2007) 133(4):581. doi: 10.1037/0033-2909.133.4.581
28. National Academies of Sciences E, Medicine. Temporomandibular disorders: Priorities for research and care. (2020).
29. Roveroni RC, Parada CA, Cecılia M, Veiga F, Tambeli CH. Development of a behavioral model of TMJ pain in rats: the TMJ formalin test. Pain. (2001) 94(2):185–91. doi: 10.1016/S0304-3959(01)00357-8
30. Luccarini P, Childeric A, Gaydier A-M, Voisin D, Dallel R. The orofacial formalin test in the mouse: a behavioral model for studying physiology and modulation of trigeminal nociception. J Pain. (2006) 7(12):908–14. doi: 10.1016/j.jpain.2006.04.010
31. Raboisson P, Dallel R. The orofacial formalin test. Neurosci Biobehav Rev. (2004) 28(2):219–26. doi: 10.1016/j.neubiorev.2003.12.003
32. Gameiro GH, Arthuri MT, Tambeli CH, de Arruda Veiga M. Effects of ethanol on deep pain evoked by formalin injected in TMJ of rat. Life Sci. (2003) 73(26):3351–61. doi: 10.1016/j.lfs.2003.06.024
33. Burgos E, Pascual D, Martín MI, Goicoechea C. Antinociceptive effect of the cannabinoid agonist, WIN 55,212-2, in the orofacial and temporomandibular formalin tests. Eur J Pain. (2010) 14(1):40–8. doi: 10.1016/j.ejpain.2009.02.003
34. Mogil JS, Lichtensteiger CA, Wilson SG. The effect of genotype on sensitivity to inflammatory nociception: characterization of resistant (A/J) and sensitive (C57BL/6J) inbred mouse strains. Pain. (1998) 76(1–2):115–25. doi: 10.1016/S0304-3959(98)00032-3
35. Hunskaar S, Hole K. The formalin test in mice: dissociation between inflammatory and non-inflammatory pain. Pain. (1987) 30(1):103–14. doi: 10.1016/0304-3959(87)90088-1
36. Sharma S, Wactawski-Wende J, LaMonte MJ, Zhao J, Slade GD, Bair E, et al. Incident injury is strongly associated with subsequent incident temporomandibular disorder: results from the OPPERA study. Pain. (2019) 160(7):1551. doi: 10.1097/j.pain.0000000000001554
37. Shinoda M, Hayashi Y, Kubo A, Iwata K. Pathophysiological mechanisms of persistent orofacial pain. J Oral Sci. (2020) 62(2):19-0373. doi: 10.2334/josnusd.19-0373
38. Eller-Smith OC, Nicol AL, Christianson JA. Potential mechanisms underlying centralized pain and emerging therapeutic interventions. Front Cell Neurosci. (2018) 12:35. doi: 10.3389/fncel.2018.00035
39. Traub RJ, Cao DY, Karpowicz J, Pandya S, Ji Y, Dorsey SG, et al. A clinically relevant animal model of TMD and IBS co-morbidity. J Pain. (2014) 15(9):956–66. doi: 10.1016/j.jpain.2014.06.008
40. Ambalavanar R, Yallampalli C, Yallampalli U, Dessem D. Injection of adjuvant but not acidic saline into craniofacial muscle evokes nociceptive behaviors and neuropeptide expression. Neuroscience. (2007) 149(3):650–9. doi: 10.1016/j.neuroscience.2007.07.058
41. Scarabelot VL, Medeiros LF, de Oliveira C, Adachi LNS, de Macedo IC, Cioato SG, et al. Melatonin alters the mechanical and thermal hyperalgesia induced by orofacial pain model in rats. Inflammation. (2016) 39(5):1649–59. doi: 10.1007/s10753-016-0399-y
42. De Rantere D, Schuster C, Reimer J, Pang D. The relationship between the rat grimace scale and mechanical hypersensitivity testing in three experimental pain models. Eur J Pain. (2016) 20(3):417–26. doi: 10.1002/ejp.742
43. Sorge RE, Mapplebeck J, Rosen S, Beggs S, Taves S, Alexander JK, et al. Different immune cells mediate mechanical pain hypersensitivity in male and female mice. Nat Neurosci. (2015) 18(8):1081–3. doi: 10.1038/nn.4053
44. Watanabe T, Ito T, Inoue G, Ohtori S, Kitajo K, Doya H, et al. The p75 receptor is associated with inflammatory thermal hypersensitivity. J Neurosci Res. (2008) 86(16):3566–74. doi: 10.1002/jnr.21808
45. Ro JY. Bite force measurement in awake rats: a behavioral model for persistent orofacial muscle pain and hyperalgesia. J Orofac Pain. (2005) 19(2):159–67.15895839
46. Wang S, Lim J, Joseph J, Wang S, Wei F, Ro JY, et al. Spontaneous and bite-evoked muscle pain are mediated by a common nociceptive pathway with differential contribution by TRPV1. J Pain. (2017) 18(11):1333–45. doi: 10.1016/j.jpain.2017.06.005
47. Donaldson LF, Seckl JR, McQueen DS. A discrete adjuvant-induced monoarthritis in the rat: effects of adjuvant dose. J Neurosci Methods. (1993) 49(1–2):5–10. doi: 10.1016/0165-0270(93)90103-X
48. Shimizu K, Guo W, Wang H, Zou S, LaGraize SC, Iwata K, et al. Differential involvement of trigeminal transition zone and laminated subnucleus caudalis in orofacial deep and cutaneous hyperalgesia: the effects of interleukin-10 and glial inhibitors. Mol Pain. (2009) 5:1744-8069-5-75. doi: 10.1186/1744-8069-5-75
49. Niu KY, Zhang Y, Ro JY. Effects of gonadal hormones on the peripheral cannabinoid receptor 1 (CB1R) system under a myositis condition in rats. Pain. (2012) 153(11):2283–91. doi: 10.1016/j.pain.2012.07.037
50. Callahan BL, Gil AS, Levesque A, Mogil JS. Modulation of mechanical and thermal nociceptive sensitivity in the laboratory mouse by behavioral state. J Pain. (2008) 9(2):174–84. doi: 10.1016/j.jpain.2007.10.011
51. Kim SH, Son CN, Lee HJ, Cho HC, Jung SW, Hur JA, et al. Infliximab partially alleviates the bite force reduction in a mouse model of temporomandibular joint pain. J Korean Med Sci. (2015) 30(5):552–8. doi: 10.3346/jkms.2015.30.5.552
52. Dolan JC, Lam DK, Achdjian SH, Schmidt BL. The dolognawmeter: a novel instrument and assay to quantify nociception in rodent models of orofacial pain. J Neurosci Methods. (2010) 187(2):207–15. doi: 10.1016/j.jneumeth.2010.01.012
53. Ren K. An improved method for assessing mechanical allodynia in the rat. Physiol Behav. (1999) 67(5):711–6. doi: 10.1016/S0031-9384(99)00136-5
54. Harper RP, Kerins CA, Talwar R, Spears R, Hutchins B, Carlson DS, et al. Meal pattern analysis in response to temporomandibular joint inflammation in the rat. J Dent Res. (2000) 79(9):1704–11. doi: 10.1177/00220345000790091101
55. Bi RY, Meng Z, Zhang P, Wang XD, Ding Y, Gan YH. Estradiol upregulates voltage-gated sodium channel 1.7 in trigeminal ganglion contributing to hyperalgesia of inflamed TMJ. PLoS One. (2017) 12(6):e0178589. doi: 10.1371/journal.pone.0178589
56. Thut PD, Hermanstyne TO, Flake NM, Gold MS. An operant conditioning model to assess changes in feeding behavior associated with temporomandibular joint inflammation in the rat. J Orofac Pain. (2007) 21(1):7–18. doi: 10.1016/S0091-3057(03)00072-8
57. Oliveira MC, Parada CA, Veiga MC, Rodrigues LR, Barros SP, Tambeli CH. Evidence for the involvement of endogenous ATP and P2X receptors in TMJ pain. Eur J Pain. (2005) 9(1):87–93. doi: 10.1016/j.ejpain.2004.04.006
58. Teixeira JM, Oliveira MC, Nociti FH Jr., Clemente-Napimoga JT, Pelegrini-da-Silva A, Parada CA, et al. Involvement of temporomandibular joint P2X3 and P2X2/3 receptors in carrageenan-induced inflammatory hyperalgesia in rats. Eur J Pharmacol. (2010) 645(1–3):79–85. doi: 10.1016/j.ejphar.2010.06.008
59. Pelegrini-da-Silva A, Oliveira MC, Parada CA, Tambeli CH. Nerve growth factor acts with the beta2-adrenoceptor to induce spontaneous nociceptive behavior during temporomandibular joint inflammatory hyperalgesia. Life Sci. (2008) 83(23–24):780–5. doi: 10.1016/j.lfs.2008.09.021
60. Rodrigues LL, Oliveira MC, Pelegrini-da-Silva A, de Arruda Veiga MC, Parada CA, Tambeli CH. Peripheral sympathetic component of the temporomandibular joint inflammatory pain in rats. J Pain. (2006) 7(12):929–36. doi: 10.1016/j.jpain.2006.05.006
61. Zanelatto FB, Dias EV, Teixeira JM, Sartori CR, Parada CA, Tambeli CH. Anti-inflammatory effects of propranolol in the temporomandibular joint of female rats and its contribution to antinociceptive action. Eur J Pain. (2018) 22(3):572–82. doi: 10.1002/ejp.1143
62. Pena-dos-Santos DR, Severino FP, Pereira SA, Rodrigues DB, Cunha FQ, Vieira SM, et al. Activation of peripheral kappa/delta opioid receptors mediates 15-deoxy-(Delta12,14)-prostaglandin J2 induced-antinociception in rat temporomandibular joint. Neuroscience. (2009) 163(4):1211–9. doi: 10.1016/j.neuroscience.2009.07.052
63. Neubert JK, Widmer CG, Malphurs W, Rossi HL, Vierck CJJ, Caudle RM. Use of a novel thermal operant behavioral assay for characterization of orofacial pain sensitivity. Pain. (2005) 116(3):386–95. doi: 10.1016/j.pain.2005.05.011
64. Rohrs EL, Kloefkorn HE, Lakes EH, Jacobs BY, Neubert JK, Caudle RM, et al. A novel operant-based behavioral assay of mechanical allodynia in the orofacial region of rats. J Neurosci Methods. (2015) 248:1–6. doi: 10.1016/j.jneumeth.2015.03.022
65. Ro JY, Lee JS, Zhang Y. Activation of TRPV1 and TRPA1 leads to muscle nociception and mechanical hyperalgesia. Pain. (2009) 144(3):270–7. doi: 10.1016/j.pain.2009.04.021
66. Neubert JK, Rossi HL, Malphurs W, Vierck CJ, Caudle RM. Differentiation between capsaicin-induced allodynia and hyperalgesia using a thermal operant assay. Behav Brain Res. (2006) 170(2):308–15. doi: 10.1016/j.bbr.2006.03.008
67. Nolan TA, Hester J, Bokrand-Donatelli Y, Caudle RM, Neubert JK. Adaptation of a novel operant orofacial testing system to characterize both mechanical and thermal pain. Behav Brain Res. (2011) 217(2):477–80. doi: 10.1016/j.bbr.2010.10.022
68. Ro JY. Contribution of peripheral NMDA receptors in craniofacial muscle nociception and edema formation. Brain Res. (2003) 979(1–2):78–84. doi: 10.1016/s0006-8993(03)02873-7
69. de Oliveira BA, Alves Rodrigues Santos SA, Menezes Pereira EW, Nogueira AB, Vieira Neto AE, de Melo Junior JMA, et al. Orofacial antinociceptive effect of nifedipine in rodents is mediated by TRPM3, TRPA1, and NMDA processes. J Oral Facial Pain Headache. (2020) 34(2):174–86. doi: 10.11607/ofph.2491
70. Cairns BE, Gambarota G, Svensson P, Arendt-Nielsen L, Berde C. Glutamate-induced sensitization of rat masseter muscle fibers. Neuroscience. (2002) 109(2):389–99. doi: 10.1016/S0306-4522(01)00489-4
71. Wong H, Kang I, Dong XD, Christidis N, Ernberg M, Svensson P, et al. NGF-induced mechanical sensitization of the masseter muscle is mediated through peripheral NMDA receptors. Neuroscience. (2014) 269:232–44. doi: 10.1016/j.neuroscience.2014.03.054
72. Hutchins MO, Skjonsby HS, Brazeau GA, Parikh UK, Jenkins RM. Weakness in mouse masticatory muscles by repetitive contractions with forced lengthening. J Dent Res. (1995) 74(2):642–8. doi: 10.1177/00220345950740020401
73. Hsieh YL, Wu BT, Yang CC. Increased substance P-like immunoreactivities in parabrachial and amygdaloid nuclei in a rat model with masticatory myofascial pain. Exp Brain Res. (2020) 238(12):2845–55. doi: 10.1007/s00221-020-05942-4
74. Hsieh YL, Yang CC, Yang NP. Ultra-Low frequency transcutaneous electrical nerve stimulation on pain modulation in a rat model with myogenous temporomandibular dysfunction. Int J Mol Sci. (2021) 22(18):1–14. doi: 10.3390/ijms22189906
75. Wang GYF, Shi XQ, Wu W, Gueorguieva M, Yang M, Zhang J. Sustained and repeated mouth opening leads to development of painful temporomandibular disorders involving macrophage/microglia activation in mice. Pain. (2018) 159(7):1277–88. doi: 10.1097/j.pain.0000000000001206
76. Guo W, Wang H, Zou S, Wei F, Dubner R, Ren K. Long lasting pain hypersensitivity following ligation of the tendon of the masseter muscle in rats: a model of myogenic orofacial pain. Mol Pain. (2010) 6:40. doi: 10.1186/1744-8069-6-40
77. Guo W, Zou S, Mohammad Z, Wang S, Yang J, Li H, et al. Voluntary biting behavior as a functional measure of orofacial pain in mice. Physiol Behav. (2019) 204:129–39. doi: 10.1016/j.physbeh.2019.02.024
78. Phero A, Ferrari LF, Taylor NE. A novel rat model of temporomandibular disorder with improved face and construct validities. Life Sci. (2021) 286:120023. doi: 10.1016/j.lfs.2021.120023
79. Bereiter DA, Okamoto K, Bereiter DF. Effect of persistent monoarthritis of the temporomandibular joint region on acute mustard oil-induced excitation of trigeminal subnucleus caudalis neurons in male and female rats. Pain. (2005) 117(1–2):58–67. doi: 10.1016/j.pain.2005.05.013
80. Lam DK, Sessle BJ, Cairns BE, Hu JW. Peripheral NMDA receptor modulation of jaw muscle electromyographic activity induced by capsaicin injection into the temporomandibular joint of rats. Brain Res. (2005) 1046(1–2):68–76. doi: 10.1016/j.brainres.2005.03.040
81. Haas DA, Nakanishi O, MacMillan RE, Jordan RC, Hu JW. Development of an orofacial model of acute inflammation in the rat. Arch Oral Biol. (1992) 37(5):417–22. doi: 10.1016/0003-9969(92)90026-5
82. Wong H, Cairns BE. Nerve growth factor increases glutamate in sensory fibres innervating the masseter muscles of female rats. Chin J Dent Res. (2018) 21(2):119–25. doi: 10.3290/j.cjdr.a40438
83. Clavelou P, Pajot J, Dallel R, Raboisson P. Application of the formalin test to the study of orofacial pain in the rat. Neurosci Lett. (1989) 103(3):349–53. doi: 10.1016/0304-3940(89)90125-0
84. Stein C, Millan M, Herz A. Unilateral inflammation of the hindpaw in rats as a model of prolonged noxious stimulation: alterations in behavior and nociceptive thresholds. Pharmacol Biochem Behav. (1988) 31(2):445–51. doi: 10.1016/0091-3057(88)90372-3
85. Okamoto K, Kimura A, Donishi T, Imbe H, Goda K, Kawanishi K, et al. Persistent monoarthritis of the temporomandibular joint region enhances nocifensive behavior and lumbar spinal fos expression after noxious stimulation to the hindpaw in rats. Exp Brain Res. (2006) 170(3):358–67. doi: 10.1007/s00221-005-0218-4
86. Winter CA, Risley EA, Nuss GW. Carrageenin-induced edema in hind paw of the rat as an assay for antiiflammatory drugs. Proc Soc Exp Biol Med. (1962) 111:544–7. doi: 10.3181/00379727-111-27849
87. Yeo JF, Ong WY, Ling SF, Farooqui AA. Intracerebroventricular injection of phospholipases A2 inhibitors modulates allodynia after facial carrageenan injection in mice. Pain. (2004) 112(1–2):148–55. doi: 10.1016/j.pain.2004.08.009
88. Kopp S. Neuroendocrine, immune, and local responses related to temporomandibular disorders. J Orofac Pain. (2001) 15(1):9–28.11889652
89. Mazuqueli Pereira ESB, Basting RT, Abdalla HB, Garcez AS, Napimoga MH, Clemente-Napimoga JT. Photobiomodulation inhibits inflammation in the temporomandibular joint of rats. J Photochem Photobiol B. (2021) 222:112281. doi: 10.1016/j.jphotobiol.2021.112281
90. Szallasi A, Blumberg PM. Vanilloid (capsaicin) receptors and mechanisms. Pharmacol Rev. (1999) 51(2):159–212.10353985
91. Chung MK, Wang S, Yang J, Alshanqiti I, Wei F, Ro JY. Neural pathways of craniofacial muscle pain: implications for novel treatments. J Dent Res. (2020) 99(9):1004–12. doi: 10.1177/0022034520919384
92. Nguyen MQ, Wu Y, Bonilla LS, von Buchholtz LJ, Ryba NJP. Diversity amongst trigeminal neurons revealed by high throughput single cell sequencing. PLoS One. (2017) 12(9):e0185543. doi: 10.1371/journal.pone.0185543
93. Strassman A, Raymond S, Burstein R. Sensitization of meningeal sensory neurons and the origin of headaches. Nature. (1996) 384(6609):560–4. doi: 10.1038/384560a0
94. Lam DK, Sessle BJ, Hu JW. Glutamate and capsaicin effects on trigeminal nociception II: activation and central sensitization in brainstem neurons with deep craniofacial afferent input. Brain Res. (2009) 1253:48–59. doi: 10.1016/j.brainres.2008.11.056
95. Kyrkanides S, Tallents RH, Macher DJ, Olschowka JA, Stevens SY. Temporomandibular joint nociception: effects of capsaicin on substance P-like immunoreactivity in the rabbit brain stem. J Orofac Pain. (2002) 16(3):229–36.12221739
96. Ichikawa H, Fukunaga T, Jin HW, Fujita M, Takano-Yamamoto T, Sugimoto T. VR1-, VRL-1- and P2X3 receptor-immunoreactive innervation of the rat temporomandibular joint. Brain Res. (2004) 1008(1):131–6. doi: 10.1016/j.brainres.2004.02.029
97. Ioi H, Kido MA, Zhang JQ, Yamaza T, Nakata S, Nakasima A, et al. Capsaicin receptor expression in the rat temporomandibular joint. Cell Tissue Res. (2006) 325(1):47–54. doi: 10.1007/s00441-006-0183-7
98. Milam SB, Schmitz JP. Molecular biology of temporomandibular joint disorders: proposed mechanisms of disease. J Oral Maxillofac Surg. (1995) 53(12):1448–54. doi: 10.1016/0278-2391(95)90675-4
99. Sessle BJ. The neural basis of temporomandibular joint and masticatory muscle pain. J Orofac Pain. (1999) 13(4):238–45.10823036
100. Spears R, Hutchins B, Hinton RJ. Capsaicin application to the temporomandibular joint alters calcitonin gene-related peptide levels in the trigeminal ganglion of the rat. J Orofac Pain. (1998) 12(2):108–15.9656888
101. Rohrs EL, Neubert JK, Caudle RM, Allen KD. Behavioral characteristics of capsaicin mediated cutaneous, myogenic, and arthrogenic orofacial nociception in rats. Arch Oral Biol. (2018) 92:18–24. doi: 10.1016/j.archoralbio.2018.04.013
102. Svensson P, Arendt-Nielsen L, Bjerring P, Bak P, Hjorth T, Troest T. Human mastication modulated by experimental trigeminal and extra-trigeminal painful stimuli. J Oral Rehabil. (1996) 23(12):838–48. doi: 10.1046/j.1365-2842.1996.00441.x
103. Winocur E, Gavish A, Halachmi M, Eli I, Gazit E. Topical application of capsaicin for the treatment of localized pain in the temporomandibular joint area. J Orofac Pain. (2000) 14(1):31–6.11203735
104. Arima T, Svensson P, Arendt-Nielsen L. Capsaicin-induced muscle hyperalgesia in the exercised and non-exercised human masseter muscle. J Orofac Pain. (2000) 14(3):213–23.11203756
105. Wang K, Arendt-Nielsen L, Svensson P. Capsaicin-induced muscle pain alters the excitability of the human jaw-stretch reflex. J Dent Res. (2002) 81(9):650–4. doi: 10.1177/154405910208100915
106. Witting N, Svensson P, Gottrup H, Arendt-Nielsen L, Jensen TS. Intramuscular and intradermal injection of capsaicin: a comparison of local and referred pain. Pain. (2000) 84(2–3):407–12. doi: 10.1016/S0304-3959(99)00231-6
107. Lam DK, Sessle BJ, Hu JW. Glutamate and capsaicin effects on trigeminal nociception I: activation and peripheral sensitization of deep craniofacial nociceptive afferents. Brain Res. (2009) 1251:130–9. doi: 10.1016/j.brainres.2008.11.029
108. Yu X, Sessle JB, Haas AD, Izzo A, Vernon H, Hu WJ. Involvement of NMDA receptor mechanisms in jaw electromyographic activity and plasma extravasation induced by inflammatory irritant application to temporomandibular joint region of rats. Pain. (1996) 68(1):169–78. doi: 10.1016/S0304-3959(96)03181-8
109. Greenspan JD, Slade GD, Bair E, Dubner R, Fillingim RB, Ohrbach R, et al. Pain sensitivity risk factors for chronic TMD: descriptive data and empirically identified domains from the OPPERA case control study. J Pain. (2011) 12(11 Suppl):T61–74. doi: 10.1016/j.jpain.2011.08.006
110. Watanabe T, Tsuboi Y, Sessle BJ, Iwata K, Hu JW. P2x and NMDA receptor involvement in temporomandibular joint-evoked reflex activity in rat jaw muscles. Brain Res. (2010) 1346:83–91. doi: 10.1016/j.brainres.2010.05.055
111. Bellinger LL, Spears R, King CM, Dahm F, Hutchins B, Kerins CA, et al. Capsaicin sensitive neurons role in the inflamed TMJ acute nociceptive response of female and male rats. Physiol Behav. (2007) 90(5):782–9. doi: 10.1016/j.physbeh.2007.01.002
112. Woolf CJ, Wall PD. Relative effectiveness of C primary afferent fibers of different origins in evoking a prolonged facilitation of the flexor reflex in the rat. J Neurosci. (1986) 6(5):1433–42. doi: 10.1523/JNEUROSCI.06-05-01433.1986
113. Jancso N, Kirally E, Jancso-Gabor A. Pharmacologically induced selective degeneration of chemosensitive primary sensory neurons. Nature. (1977) 22:741–3. doi: 10.1038/270741a0
114. McMahon SB, Abel C. A model for the study of visceral pain states: chronic inflammation of the chronic decerebrate rat urinary bladder by irritant chemicals. Pain. (1987) 28(1):109–27. doi: 10.1016/0304-3959(87)91065-7
115. Hathaway CB, Hu JW, Bereiter DA. Distribution of fos-like immunoreactivity in the caudal brainstem of the rat following noxious chemical stimulation of the temporomandibular joint. J Comp Neurol. (1995) 356(3):444–56. doi: 10.1002/cne.903560311
116. Bereiter DA, Benetti AP. Excitatory amino release within spinal trigeminal nucleus after mustard oil injection into the temporomandibular joint region of the rat. Pain. (1996) 67(2–3):451–9. doi: 10.1016/0304-3959(96)03156-9
117. Yu XM, Sessle BJ, Vernon H, Hu JW. Administration of opiate antagonist naloxone induces recurrence of increased jaw muscle activities related to inflammatory irritant application to rat temporomandibular joint region. J Neurophysiol. (1994) 72(3):1430–3. doi: 10.1152/jn.1994.72.3.1430
118. Bakke M, Hu JW, Sessle BJ. Morphine application to peripheral tissues modulates nociceptive jaw reflex. Neuroreport. (1998) 9(14):3315–9. doi: 10.1097/00001756-199810050-00030
119. Bereiter DA, Bereiter DF. Morphine and NMDA receptor antagonism reduce c-fos expression in spinal trigeminal nucleus produced by acute injury to the TMJ region. Pain. (2000) 85(1–2):65–77. doi: 10.1016/S0304-3959(99)00246-8
120. Tambeli CH, Seo K, Sessle BJ, Hu JW. Central mu opioid receptor mechanisms modulate mustard oil-evoked jaw muscle activity. Brain Res. (2001) 913(1):90–4. doi: 10.1016/S0006-8993(01)02742-1
121. Seo K, Fujiwara N, Hu JW, Cairns BE, Someya G. Intrathecal administration of 5-HT(3) receptor agonist modulates jaw muscle activity evoked by injection of mustard oil into the temporomandibular joint in the rat. Brain Res. (2002) 934(2):157–61. doi: 10.1016/S0006-8993(02)02367-3
122. Cairns BE, Sessle BJ, Hu JW. Evidence that excitatory amino acid receptors within the temporomandibular joint region are involved in the reflex activation of the jaw muscles. J Neurosci. (1998) 18(19):8056–64. doi: 10.1523/JNEUROSCI.18-19-08056.1998
123. Bakke M, Hu JW, Sessle BJ. Involvement of NK-1 and NK-2 tachykinin receptor mechanisms in jaw muscle activity reflexly evoked by inflammatory irritant application to the rat temporomandibular joint. Pain. (1998) 75(2–3):219–27. doi: 10.1016/S0304-3959(97)00223-6
124. Cairns BE, Sessle BJ, Hu JW. Activation of peripheral GABAA receptors inhibits temporomandibular joint-evoked jaw muscle activity. J Neurophysiol. (1999) 81(4):1966–9. doi: 10.1152/jn.1999.81.4.1966
125. Seo K, Hu JW, Cairns BE, Someya G. Involvement of GABA(A) receptor in modulation of jaw muscle activity evoked by mustard oil application to the rat temporomandibular joint. Brain Res. (2001) 892(1):198–202. doi: 10.1016/S0006-8993(00)03283-2
126. Bereiter DA. Sex differences in brainstem neural activation after injury to the TMJ region. Cells Tissues Organs. (2001) 169(3):226–37. doi: 10.1159/000047886
127. Bereiter DA, Shen S, Benetti AP. Sex differences in amino acid release from rostral trigeminal subnucleus caudalis after acute injury to the TMJ region. Pain. (2002) 98(1–2):89–99. doi: 10.1016/S0304-3959(01)00476-6
128. Cairns BE, Sim Y, Bereiter DA, Sessle BJ, Hu JW. Influence of sex on reflex jaw muscle activity evoked from the rat temporomandibular joint. Brain Res. (2002) 957(2):338–44. doi: 10.1016/S0006-8993(02)03671-5
129. Seo K, Fujiwara N, Cairns BE, Someya G. Male rats require testosterone to develop contralateral digastric muscle activity in response to noxious stimulation of the temporomandibular joint. Neurosci Lett. (2002) 335(2):107–10. doi: 10.1016/S0304-3940(02)01173-4
130. Bereiter DA, Benetti AP. Amino acid release at the spinomedullary junction after inflammation of the TMJ region in male and female rats. Pain. (2006) 126(1–3):175–83. doi: 10.1016/j.pain.2006.06.030
131. Chang Z, Okamoto K, Bereiter DA. Differential ascending projections of temporomandibular joint-responsive brainstem neurons to periaqueductal gray and posterior thalamus of male and female rats. Neuroscience. (2012) 203:230–43. doi: 10.1016/j.neuroscience.2011.11.042
132. Okamoto K, Hirata H, Takeshita S, Bereiter DA. Response properties of TMJ units in superficial laminae at the spinomedullary junction of female rats vary over the estrous cycle. J Neurophysiol. (2003) 89(3):1467–77. doi: 10.1152/jn.00795.2002
133. Ro JY, Svensson P, Capra N. Effects of experimental muscle pain on electromyographic activity of masticatory muscles in the rat. Muscle Nerve. (2002) 25(4):576–84. doi: 10.1002/mus.10072
134. Ro JY, Harriott A, Crouse U, Capra NF. Innocuous jaw movements increase c-fos expression in trigeminal sensory nuclei produced by masseter muscle inflammation. Pain. (2003) 104(3):539–48. doi: 10.1016/S0304-3959(03)00093-9
135. Ro JY, Capra NF, Masri R. Contribution of peripheral N-methyl-D-aspartate receptors to c-fos expression in the trigeminal spinal nucleus following acute masseteric inflammation. Neuroscience. (2004) 123(1):213–9. doi: 10.1016/S0306-4522(03)00465-2
136. Chun YH, Frank D, Lee JS, Zhang Y, Auh QS, Ro JY. Peripheral AMPA receptors contribute to muscle nociception and c-fos activation. Neurosci Res. (2008) 62(2):97–104. doi: 10.1016/j.neures.2008.06.007
137. Li JL, Xiong KH, Dong YL, Fujiyama F, Kaneko T, Mizuno N. Vesicular glutamate transporters, VGluT1 and VGluT2, in the trigeminal ganglion neurons of the rat, with special reference to coexpression. J Comp Neurol. (2003) 463(2):212–20. doi: 10.1002/cne.10755
138. Klegeris A. Mcgeer PL. beta-amyloid protein enhances macrophage production of oxygen free radicals and glutamate. J Neurosci Res. (1997) 49(2):229–35. doi: 10.1002/(SICI)1097-4547(19970715)49:2%3C229::AID-JNR11%3E3.0.CO;2-W
139. Parpura V, Liu F, Jeftinija KV, Haydon PG, Jeftinija SD. Neuroligand-evoked calcium-dependent release of excitatory amino acids from Schwann cells. J Neurosci. (1995) 15(8):5831–9. doi: 10.1523/JNEUROSCI.15-08-05831.1995
140. deGroot J, Zhou S, Carlton SM. Peripheral glutamate release in the hindpaw following low and high intensity sciatic stimulation. Neuroreport. (2000) 11(3):497–502. doi: 10.1097/00001756-200002280-00014
141. Chung MK, Ro JY. Peripheral glutamate receptor and transient receptor potential channel mechanisms of craniofacial muscle pain. Mol Pain. (2020) 16:1744806920914204. doi: 10.1177/1744806920914204
142. Chandler SH. Evidence for excitatory amino acid transmission between mesencephalic nucleus of V afferents and jaw-closer motoneurons in the Guinea pig. Brain Res. (1989) 477(1–2):252–64. doi: 10.1016/0006-8993(89)91413-3
143. Chung MK, Park J, Asgar J, Ro JY. Transcriptome analysis of trigeminal ganglia following masseter muscle inflammation in rats. Mol Pain. (2016) 12:1–11. doi: 10.1177/1744806916668526
144. Cairns BE, Hu JW, Arendt-Nielsen L, Sessle BJ, Svensson P. Sex-related differences in human pain and rat afferent discharge evoked by injection of glutamate into the masseter muscle. J Neurophysiol. (2001) 86(2):782–91. doi: 10.1152/jn.2001.86.2.782
145. Lawand NB, Willis WD, Westlund KN. Excitatory amino acid receptor involvement in peripheral nociceptive transmission in rats. Eur J Pharmacol. (1997) 324(2–3):169–77. doi: 10.1016/S0014-2999(97)00072-1
146. Fiorentino PM, Cairns BE, Hu JW. Development of inflammation after application of mustard oil or glutamate to the rat temporomandibular joint. Arch Oral Biol. (1999) 44(1):27–32. doi: 10.1016/S0003-9969(98)00095-8
147. Chao MV. Neurotrophins and their receptors: a convergence point for many signalling pathways. Nat Rev Neurosci. (2003) 4(4):299–309. doi: 10.1038/nrn1078
148. Svensson P, Castrillon E, Cairns BE. Nerve growth factor-evoked masseter muscle sensitization and perturbation of jaw motor function in healthy women. J Orofac Pain. (2008) 22(4):340–8.19090407
149. Svensson P, Wang K, Arendt-Nielsen L, Cairns BE. Effects of NGF-induced muscle sensitization on proprioception and nociception. Exp Brain Res. (2008) 189(1):1–10. doi: 10.1007/s00221-008-1399-4
150. Svensson P, Cairns BE, Wang K, Arendt-Nielsen L. Injection of nerve growth factor into human masseter muscle evokes long-lasting mechanical allodynia and hyperalgesia. Pain. (2003) 104(1-2):241–7. doi: 10.1016/S0304-3959(03)00012-5
151. Mann MK, Dong XD, Svensson P, Cairns BE. Influence of intramuscular nerve growth factor injection on the response properties of rat masseter muscle afferent fibers. J Orofac Pain. (2006) 20(4):325–36.17190031
152. Wong H, Dong XD, Cairns BE. Nerve growth factor alters the sensitivity of rat masseter muscle mechanoreceptors to NMDA receptor activation. J Neurophysiol. (2014) 112(9):2275–82. doi: 10.1152/jn.00327.2014
153. Sharma S, Ohrbach R, Fillingim R, Greenspan J, Slade G. Pain sensitivity modifies risk of injury-related temporomandibular disorder. J Dent Res. (2020) 99(5):530–6. doi: 10.1177/0022034520913247
154. Kramer PR, Bellinger LL. Reduced GABAA receptor alpha6 expression in the trigeminal ganglion enhanced myofascial nociceptive response. Neuroscience. (2013) 245:1–11. doi: 10.1016/j.neuroscience.2013.04.003
155. Kramer PR, Bellinger LL. Infusion of Gabralpha6 siRNA into the trigeminal ganglia increased the myogenic orofacial nociceptive response of ovariectomized rats treated with 17beta-estradiol. Neuroscience. (2014) 278:144–53. doi: 10.1016/j.neuroscience.2014.07.066
156. Guo W, Imai S, Yang JL, Zou S, Li H, Xu H, et al. NF-KappaB Pathway is involved in bone marrow stromal cell-produced pain relief. Front Integr Neurosci. (2018) 12:49. doi: 10.3389/fnint.2018.00049
157. Guo W, Chu YX, Imai S, Yang JL, Zou S, Mohammad Z, et al. Further observations on the behavioral and neural effects of bone marrow stromal cells in rodent pain models. Mol Pain. (2016) 12:1–12. doi: 10.1177/1744806916658043
158. Guo W, Wang H, Zou S, Gu M, Watanabe M, Wei F, et al. Bone marrow stromal cells produce long-term pain relief in rat models of persistent pain. Stem Cells. (2011) 29(8):1294–303. doi: 10.1002/stem.667
159. Kramer PR, Umorin M, Bellinger LL. Attenuation of myogenic orofacial nociception and mechanical hypersensitivity by viral mediated enkephalin overproduction in male and female rats. BMC Neurol. (2015) 15:34. doi: 10.1186/s12883-015-0285-5
160. Meloto CB, Benavides R, Lichtenwalter RN, Wen X, Tugarinov N, Zorina-Lichtenwalter K, et al. Human pain genetics database: a resource dedicated to human pain genetics research. Pain. (2018) 159(4):749–63. doi: 10.1097/j.pain.0000000000001135
161. Diatchenko L, Fillingim RB, Smith SB, Maixner W. The phenotypic and genetic signatures of common musculoskeletal pain conditions. Nat Rev Rheumatol. (2013) 9(6):340–350. doi: 10.1038/nrrheum.2013.43
162. Diatchenko L, Slade GD, Nackley AG, Bhalang K, Sigurdsson A, Belfer I, et al. Genetic basis for individual variations in pain perception and the development of a chronic pain condition. Hum Mol Genet. (2005) 14(1):135–43. doi: 10.1093/hmg/ddi013
163. Meloto CB, Segall SK, Smith S, Parisien M, Shabalina SA, Rizzatti-Barbosa CM, et al. COMT Gene locus: new functional variants. Pain. (2015) 156(10):2072–83. doi: 10.1097/j.pain.0000000000000273
164. Meloto CB, Bortsov AV, Bair E, Helgeson E, Ostrom C, Smith SB, et al. Modification of COMT-dependent pain sensitivity by psychological stress and sex. Pain. (2016) 157(4):858–67. doi: 10.1097/j.pain.0000000000000449
165. Arnold M. Headache classification committee of the international headache society (IHS) the international classification of headache disorders. Cephalalgia. (2018) 38(1):1–211. doi: 10.1177/0333102417738202
166. Benoliel R, Svensson P, Evers S, Wang S-J, Barke A, Korwisi B, et al. The IASP classification of chronic pain for ICD-11: chronic secondary headache or orofacial pain. Pain. (2019) 160(1):60–8. doi: 10.1097/j.pain.0000000000001435
167. Antonini G, Di Pasquale A, Cruccu G, Truini A, Morino S, Saltelli G, et al. Magnetic resonance imaging contribution for diagnosing symptomatic neurovascular contact in classical trigeminal neuralgia: a blinded case-control study and meta-analysis. Pain. (2014) 155(8):1464–71. doi: 10.1016/j.pain.2014.04.020
168. Maarbjerg S, Wolfram F, Gozalov A, Olesen J, Bendtsen L. Significance of neurovascular contact in classical trigeminal neuralgia. Brain. (2015) 138(2):311–9. doi: 10.1093/brain/awu349
169. Rappaport ZH, Govrin-Lippmann R, Devor M. An electron-microscopic analysis of biopsy samples of the trigeminal root taken during microvascular decompressive surgery. Stereotact Funct Neurosurg. (1997) 68(1–4):182–6. doi: 10.1159/000099920
170. Miles JB, Eldridge PR, Haggett CE, Bowsher D. Sensory effects of microvascular decompression in trigeminal neuralgia. J Neurosurg. (1997) 86(2):193–6. doi: 10.3171/jns.1997.86.2.0193
171. Leandri M, Eldridge P, Miles J. Recovery of nerve conduction following microvascular decompression for trigeminal neuralgia. Neurology. (1998) 51(6):1641–6. doi: 10.1212/WNL.51.6.1641
172. Oesman C, Mooij JJ. Long-term follow-up of microvascular decompression for trigeminal neuralgia. Skull Base. (2011) 21(5):313–22. doi: 10.1055/s-0031-1284213
173. Siqueira S, Alves B, Malpartida H, Teixeira M, Siqueira J. Abnormal expression of voltage-gated sodium channels Nav1. 7, Nav1. 3 and Nav1. 8 in trigeminal neuralgia. Neuroscience. (2009) 164(2):573–7. doi: 10.1016/j.neuroscience.2009.08.037
174. Tanaka BS, Zhao P, Dib-Hajj FB, Morisset V, Tate S, Waxman SG, et al. A gain-of-function mutation in Nav1. 6 in a case of trigeminal neuralgia. Mol Med. (2016) 22(1):338–48. doi: 10.2119/molmed.2016.00131
175. Ericson H, Hamdeh SA, Freyhult E, Stiger F, Bäckryd E, Svenningsson A, et al. Cerebrospinal fluid biomarkers of inflammation in trigeminal neuralgia patients operated with microvascular decompression. Pain. (2019) 160(11):2603–11. doi: 10.1097/j.pain.0000000000001649
176. Arrese I, Lagares A, Alday R, Ramos A, Rivas JJ, Lobato RD. Typical trigeminal neuralgia associated with brainstem white matter lesions on MRI in patients without criteria of multiple sclerosis. Acta Neurochir. (2008) 150(11):1157–61. doi: 10.1007/s00701-008-0024-4
177. DeSouza DD, Hodaie M, Davis KD. Abnormal trigeminal nerve microstructure and brain white matter in idiopathic trigeminal neuralgia. Pain. (2014) 155(1):37–44. doi: 10.1016/j.pain.2013.08.029
178. Tohyama S, Hung PS-P, Cheng JC, Zhang JY, Halawani A, Mikulis DJ, et al. Trigeminal neuralgia associated with a solitary pontine lesion: clinical and neuroimaging definition of a new syndrome. Pain. (2020) 161(5):916. doi: 10.1097/j.pain.0000000000001777
179. Obermann M, Yoon M, Ese D, Maschke M, Kaube H, Diener H, et al. Impaired trigeminal nociceptive processing in patients with trigeminal neuralgia. Neurology. (2007) 69(9):835–41. doi: 10.1212/01.wnl.0000269670.30045.6b
180. Leonard G, Goffaux P, Mathieu D, Blanchard J, Kenny B, Marchand S. Evidence of descending inhibition deficits in atypical but not classical trigeminal neuralgia. Pain. (2009) 147(1–3):217–23. doi: 10.1016/j.pain.2009.09.009
181. Younis S, Maarbjerg S, Reimer M, Wolfram F, Olesen J, Baron R, et al. Quantitative sensory testing in classical trigeminal neuralgia—a blinded study in patients with and without concomitant persistent pain. Pain. (2016) 157(7):1407–14. doi: 10.1097/j.pain.0000000000000528
182. Wu Z, Xiao-yan Q, Jian-xiong A, Cai-cai L, Tian M, Cope DK, et al. Trigeminal neuralgia induced by cobra venom in the rat leads to deficits in abilities of spatial learning and memory. Pain Physician. (2015) 18(2):E207.25794221
183. Zhao Q, Xiao-yan Q, Jian-xiong A, Cai-cai L, Fang Q, Wang Y, et al. Rat model of trigeminal neuralgia using cobra venom mimics the electron microscopy, behavioral, and anticonvulsant drug responses seen in patients. Pain Physician. (2015) 18(6):E1083.26606021
184. Vos BP, Strassman AM, Maciewicz RJ. Behavioral evidence of trigeminal neuropathic pain following chronic constriction injury to the rat's infraorbital nerve. J Neurosci. (1994) 14(5):2708–23. doi: 10.1523/JNEUROSCI.14-05-02708.1994
185. Luiz AP, Schroeder SD, Chichorro JG, Calixto JB, Zampronio AR, Rae GA. Kinin B1 and B2 receptors contribute to orofacial heat hyperalgesia induced by infraorbital nerve constriction injury in mice and rats. Neuropeptides. (2010) 44(2):87–92. doi: 10.1016/j.npep.2009.10.005
186. Xu M, Aita M, Chavkin C. Partial infraorbital nerve ligation as a model of trigeminal nerve injury in the mouse: behavioral, neural, and glial reactions. J Pain. (2008) 9(11):1036–48. doi: 10.1016/j.jpain.2008.06.006
187. Ding W, You Z, Shen S, Yang J, Lim G, Doheny JT, et al. An improved rodent model of trigeminal neuropathic pain by unilateral chronic constriction injury of distal infraorbital nerve. J Pain. (2017) 18(8):899–907. doi: 10.1016/j.jpain.2017.02.427
188. Zhang M, Hu M, Montera MA, Westlund KN. Sustained relief of trigeminal neuropathic pain by a blood-brain barrier penetrable PPAR gamma agonist. Mol Pain. (2019) 15:1744806919884498. doi: 10.1177/1744806919884498
189. Shinoda M, Kawashima K, Ozaki N, Asai H, Nagamine K, Sugiura Y. P2x3 receptor mediates heat hyperalgesia in a rat model of trigeminal neuropathic pain. J Pain. (2007) 8(7):588–97. doi: 10.1016/j.jpain.2007.03.001
190. Imamura Y, Kawamoto H, Nakanishi O. Characterization of heat-hyperalgesia in an experimental trigeminal neuropathy in rats. Exp Brain Res. (1997) 116(1):97–103. doi: 10.1007/PL00005748
191. Deseure K, Hans G. Behavioral study of non-evoked orofacial pain following different types of infraorbital nerve injury in rats. Physiol Behav. (2015) 138:292–6. doi: 10.1016/j.physbeh.2014.10.009
192. Kumada A, Matsuka Y, Spigelman I, Maruhama K, Yamamoto Y, Neubert JK, et al. Intradermal injection of Botulinum toxin type A alleviates infraorbital nerve constriction-induced thermal hyperalgesia in an operant assay. J Oral Rehabil. (2012) 39(1):63–72. doi: 10.1111/j.1365-2842.2011.02236.x
193. Ma F, Zhang L, Lyons D, Westlund KN. Orofacial neuropathic pain mouse model induced by trigeminal inflammatory compression (TIC) of the infraorbital nerve. Mol Brain. (2012) 5:44. doi: 10.1186/1756-6606-5-44
194. Ma F, Zhang L, Oz HS, Mashni M, Westlund KN. Dysregulated TNFα promotes cytokine proteome profile increases and bilateral orofacial hypersensitivity. Neuroscience. (2015) 300:493–507. doi: 10.1016/j.neuroscience.2015.05.046
195. Lyons DN, Kniffin TC, Zhang LP, Danaher RJ, Miller CS, Bocanegra JL, et al. Trigeminal inflammatory compression (TIC) injury induces chronic facial pain and susceptibility to anxiety-related behaviors. Neuroscience. (2015) 295:126–38. doi: 10.1016/j.neuroscience.2015.03.051
196. Yalcin I, Bohren Y, Waltisperger E, Sage-Ciocca D, Yin JC, Freund-Mercier M-J, et al. A time-dependent history of mood disorders in a murine model of neuropathic pain. Biol Psychiatry. (2011) 70(10):946–53. doi: 10.1016/j.biopsych.2011.07.017
197. Yalcin I, Barthas F, Barrot M. Emotional consequences of neuropathic pain: insight from preclinical studies. Neurosci Biobehav Rev. (2014) 47:154–64. doi: 10.1016/j.neubiorev.2014.08.002
198. Kniffin TC, Danaher RJ, Westlund KN, Ma F, Miller CS, Carlson CR. Persistent neuropathic pain influences persistence behavior in rats. J Oral Facial Pain Headache. (2015) 29(2):183. doi: 10.11607/ofph.1300
199. Lyons DN, Zhang L, Pandya JD, Danaher RJ, Ma F, Miller CS, et al. Combination drug therapy of pioglitazone and D-cycloserine attenuates chronic orofacial neuropathic pain and anxiety by improving mitochondrial function following trigeminal nerve injury. Clin J Pain. (2018) 34(2):168. doi: 10.1097/AJP.0000000000000515
200. Montera MA, Westlund KN. Minimally invasive oral surgery induction of the FRICT-ION chronic neuropathic pain model. Bio Protoc. (2020) 10(8):e3591. doi: 10.21769/BioProtoc.3591
201. Luo D-S, Zhang T, Chang-Xu Z, Zuo Z-F, Li H, Sheng-Xi W, et al. An animal model for trigeminal neuralgia by compression of the trigeminal nerve root. Pain Physician. (2012) 15(2):187.22430657
202. Luo D, Lin R, Luo L, Li Q, Chen T, Qiu R, et al. Glial plasticity in the trigeminal root entry zone of a rat trigeminal neuralgia animal model. Neurochem Res. (2019) 44(8):1893–902. doi: 10.1007/s11064-019-02824-2
203. Ahn DK, Lim EJ, Kim BC, Yang GY, Lee MK, Ju JS, et al. Compression of the trigeminal ganglion produces prolonged nociceptive behavior in rats. Eur J Pain. (2009) 13(6):568–75. doi: 10.1016/j.ejpain.2008.07.008
204. Kim MJ, Shin HJ, Won KA, Yang KY, Ju JS, Park YY, et al. Progesterone produces antinociceptive and neuroprotective effects in rats with microinjected lysophosphatidic acid in the trigeminal nerve root. Mol Pain. (2012) 8:16. doi: 10.1186/1744-8069-8-16
205. An J-X, He Y, Qian X-Y, Wu J-P, Xie Y-K, Guo Q-L, et al. A new animal model of trigeminal neuralgia produced by administration of cobra venom to the infraorbital nerve in the rat. Anesth Analg. (2011) 113(3):652–6. doi: 10.1213/ANE.0b013e3182245add
206. International Association for the Study of Pain. IASP Taxonomy. Pain terms. Neuropathic pain. IASP Taxonomy. (2017). Available at: www.iasp-pain.org/Taxonomy#Neuropathicpain
207. Burchiel KJ. Abnormal impulse generation in focally demyelinated trigeminal roots. J Neurosurg. (1980) 53(5):674–83. doi: 10.3171/jns.1980.53.5.0674
208. Maves TJ, Pechman PS, Gebhart GF, Meller ST. Possible chemical contribution from chromic gut sutures produces disorders of pain sensation like those seen in man. Pain. (1993) 54(1):57–69. doi: 10.1016/0304-3959(93)90100-4
209. Pozza DH, Castro-Lopes JM, Neto FL, Avelino A. Spared nerve injury model to study orofacial pain. Indian J Med Res. (2016) 143(3):297. doi: 10.4103/0971-5916.182619
210. Miyamoto M, Tsuboi Y, Takamiya K, Huganir RL, Kondo M, Shinoda M, et al. Involvement of GluR2 and GluR3 subunit C-termini in the trigeminal spinal subnucleus caudalis and C1–C2 neurons in trigeminal neuropathic pain. Neurosci Lett. (2011) 491(1):8–12. doi: 10.1016/j.neulet.2010.12.060
211. Bennett GJ, Xie Y-K. A peripheral mononeuropathy in rat that produces disorders of pain sensation like those seen in man. Pain. (1988) 33(1):87–107. doi: 10.1016/0304-3959(88)90209-6
212. La Touche R, Paris-Alemany A, Hidalgo-Pérez A, López-de-Uralde-Villanueva I, Angulo-Diaz-Parreño S, Muñoz-García D. Evidence for central sensitization in patients with temporomandibular disorders: a systematic review and meta-analysis of observational studies. Pain Pract. (2018) 18(3):388–409. doi: 10.1111/papr.12604
213. Garrigós-Pedrón M, La Touche R, Navarro-Desentre P, Gracia-Naya M, Segura-Ortí E. Widespread mechanical pain hypersensitivity in patients with chronic migraine and temporomandibular disorders: relationship and correlation between psychological and sensorimotor variables. Acta Odontol Scand. (2019) 77(3):224–31. doi: 10.1080/00016357.2018.1538533
214. Slade GD, Smith SB, Zaykin DV, Tchivileva IE, Gibson DG, Yuryev A, et al. Facial pain with localized and widespread manifestations: separate pathways of vulnerability. Pain. (2013) 154(11):2335–43. doi: 10.1016/j.pain.2013.07.009
215. Bongenhielm U, Boissonade FM, Westermark A, Robinson PP, Fried K. Sympathetic nerve sprouting fails to occur in the trigeminal ganglion after peripheral nerve injury in the rat. Pain. (1999) 82(3):283–8. doi: 10.1016/S0304-3959(99)00064-0
216. Benoliel R, Eliav E, Tal M. No sympathetic nerve sprouting in rat trigeminal ganglion following painful and non-painful infraorbital nerve neuropathy. Neurosci Lett. (2001) 297(3):151–4. doi: 10.1016/S0304-3940(00)01681-5
217. Korczeniewska OA, Katzmann Rider G, Gajra S, Narra V, Ramavajla V, Chang YJ, et al. Differential gene expression changes in the dorsal root versus trigeminal ganglia following peripheral nerve injury in rats. Eur J Pain. (2020) 24(5):967–82. doi: 10.1002/ejp.1546
218. Love S, Coakham HB. Trigeminal neuralgia: pathology and pathogenesis. Brain. (2001) 124(12):2347–60. doi: 10.1093/brain/124.12.2347
219. Marinković S, Todorović V, Gibo H, Budeč M, Drndarević N, Pešić D, et al. The trigeminal vasculature pathology in patients with neuralgia. Headache. (2007) 47(9):1334–9. doi: 10.1111/j.1526-4610.2007.00933.x
220. Ramesh VG, Premkumar G. An anatomical study of the neurovascular relationships at the trigeminal root entry zone. J Clin Neurosci. (2009) 16(7):934–6. doi: 10.1016/j.jocn.2008.09.011
221. Lin J, Zhou L, Luo Z, Adam MI, Zhao L, Wang F, et al. Flow cytometry analysis of immune and glial cells in a trigeminal neuralgia rat model. Sci Rep. (2021) 11(1):23569. doi: 10.1038/s41598-021-02911-x
222. Yeomans DC, Klukinov M. A rodent model of trigeminal neuralgia. Pain Res. (2012) 851:121–31. doi: 10.1007/978-1-61779-561-9_8
223. Jeon HJ, Han SR, Lim KH, Won KA, Bae YC, Ahn DK. Intracisternal administration of NR2 subunit antagonists attenuates the nociceptive behavior and p-p38 MAPK expression produced by compression of the trigeminal nerve root. Mol Pain. (2011) 7:46. doi: 10.1186/1744-8069-7-46
224. Gardner WJ. Concerning the mechanism of trigeminal neuralgia and hemifacial spasm. J Neurosurg. (1962) 19:947–58. doi: 10.3171/jns.1962.19.11.0947
225. Jannetta PJ. Arterial compression of the trigeminal nerve at the pons in patients with trigeminal neuralgia. J Neurosurg. (1967) 26(1):159–62. doi: 10.3171/jns.1967.26.1part2.0159
226. Minguez Sanz MP, Salort Llorca C, Silvestre Donat FJ. Etiology of burning mouth syndrome: a review and update. (2011).
227. Lauria G, Majorana A, Borgna M, Lombardi R, Penza P, Padovani A, et al. Trigeminal small-fiber sensory neuropathy causes burning mouth syndrome. Pain. (2005) 115(3):332–7. doi: 10.1016/j.pain.2005.03.028
228. Yilmaz Z, Renton T, Yiangou Y, Zakrzewska J, Chessell I, Bountra C, et al. Burning mouth syndrome as a trigeminal small fibre neuropathy: increased heat and capsaicin receptor TRPV1 in nerve fibres correlates with pain score. J Clin Neurosci. (2007) 14(9):864–71. doi: 10.1016/j.jocn.2006.09.002
229. Beneng K, Yilmaz Z, Yiangou Y, McParland H, Anand P, Renton T. Sensory purinergic receptor P2X3 is elevated in burning mouth syndrome. Int J Oral Maxillofac Surg. (2010) 39(8):815–9. doi: 10.1016/j.ijom.2010.03.013
230. Borsani E, Majorana A, Cocchi MA, Conti G, Bonadeo S, Padovani A, et al. Epithelial expression of vanilloid and cannabinoid receptors: A potential role in burning mouth syndrome pathogenesis. Histol Histopathol. (2014) 29(4):523–33. doi: 10.14670/HH-29.10.523
231. Boucher Y, Simons CT, Carstens MI, Carstens E. Effects of gustatory nerve transection and/or ovariectomy on oral capsaicin avoidance in rats. Pain. (2014) 155(4):814–20. doi: 10.1016/j.pain.2014.01.020
232. Elitt CM, Malin SA, Koerber HR, Davis BM, Albers KM. Overexpression of artemin in the tongue increases expression of TRPV1 and TRPA1 in trigeminal afferents and causes oral sensitivity to capsaicin and mustard oil. Brain Res. (2008) 1230:80–90. doi: 10.1016/j.brainres.2008.06.119
233. Shinoda M, Takeda M, Honda K, Maruno M, Katagiri A, Satoh-Kuriwada S, et al. Involvement of peripheral artemin signaling in tongue pain: possible mechanism in burning mouth syndrome. Pain. (2015) 156(12):2528–37. doi: 10.1097/j.pain.0000000000000322
234. Elitt CM, McIlwrath SL, Lawson JJ, Malin SA, Molliver DC, Cornuet PK, et al. Artemin overexpression in skin enhances expression of TRPV1 and TRPA1 in cutaneous sensory neurons and leads to behavioral sensitivity to heat and cold. J Neurosci. (2006) 26(33):8578–87. doi: 10.1523/JNEUROSCI.2185-06.2006
235. Malin SA, Molliver DC, Koerber HR, Cornuet P, Frye R, Albers KM, et al. Glial cell line-derived neurotrophic factor family members sensitize nociceptors in vitro and produce thermal hyperalgesia in vivo. J Neurosci. (2006) 26(33):8588–99. doi: 10.1523/JNEUROSCI.1726-06.2006
236. Malin S, Molliver D, Christianson JA, Schwartz ES, Cornuet P, Albers KM, et al. TRPV1 And TRPA1 function and modulation are target tissue dependent. J Neurosci. (2011) 31(29):10516–28. doi: 10.1523/JNEUROSCI.2992-10.2011
237. Woolf CJ. Long term alterations in the excitability of the flexion reflex produced by peripheral tissue injury in the chronic decerebrate rat. Pain. (1984) 18(4):325–43. doi: 10.1016/0304-3959(84)90045-9
238. Mogil JS. Animal models of pain: progress and challenges. Nat Rev Neurosci. (2009) 10(4):283–94. doi: 10.1038/nrn2606
239. Rice AS, Cimino-Brown D, Eisenach JC, Kontinen VK, Lacroix-Fralish ML, Machin I, et al. Animal models and the prediction of efficacy in clinical trials of analgesic drugs: a critical appraisal and call for uniform reporting standards. Pain. (2008) 139(2):243–7. doi: 10.1016/j.pain.2008.08.017
240. Watson C. Campbell JN, Basbaum AI, Dray A, Dubner R, Dworkin RH, Sang CN, eds. Emerging strategies for the treatment of neuropathic pain. Seattle: IASP Press, 2006. ISBN 0-93109261-2; 2514 pages; US $80; IASP member price US $64. Pain Res Manag. (2007) 12(1):50–2. doi: 10.1155/2007/561827
241. Vierck CJ, Hansson PT, Yezierski RP. Clinical and pre-clinical pain assessment: are we measuring the same thing? Pain. (2008) 135(1):7–10. doi: 10.1016/j.pain.2007.12.008
242. Bell-Krotoski J, Tomancik E. The repeatability of testing with semmes-weinstein monofilaments. J Hand Surg Am. (1987) 12(1):155–61. doi: 10.1016/S0363-5023(87)80189-2
243. Semmes J, Weinstein S, Ghent L, Teuber H-L. Somatosensory changes after penetrating brain wounds in man. Yale J Biol Med. (1960) 33(3) 249–50.
244. Guo W, Wei F, Zou S, Robbins MT, Sugiyo S, Ikeda T, et al. Group I metabotropic glutamate receptor NMDA receptor coupling and signaling cascade mediate spinal dorsal horn NMDA receptor 2B tyrosine phosphorylation associated with inflammatory hyperalgesia. J Neurosci. (2004) 24(41):9161–73. doi: 10.1523/JNEUROSCI.3422-04.2004
245. Cornelison LE, Chelliboina N, Woodman SE, Durham PL. Dietary supplementation with grape seed extract prevents development of trigeminal sensitization and inhibits pain signaling in a preclinical chronic temporomandibular disorder model. J Oral Pathol Med. (2020) 49(6):514–21. doi: 10.1111/jop.13066
246. Rotpenpian N, Tapechum S, Vattarakorn A, Chindasri W, Care C, Pakaprot N, et al. Evolution of mirror-image pain in temporomandibular joint osteoarthritis mouse model. J Appl Oral Sci. (2021) 29:e20200575. doi: 10.1590/1678-7757-2020-0575
247. Wong H, Cairns BE. Cannabidiol, cannabinol and their combinations act as peripheral analgesics in a rat model of myofascial pain. Arch Oral Biol. (2019) 104:33–9. doi: 10.1016/j.archoralbio.2019.05.028
248. Simonic-Kocijan S, Zhao X, Liu W, Wu Y, Uhac I, Wang K. TRPV1 channel-mediated bilateral allodynia induced by unilateral masseter muscle inflammation in rats. Mol Pain. (2013) 9:68. doi: 10.1186/1744-8069-9-68
249. Garattini EG, Santos BM, Ferrari DP, Capel CP, Francescato HDC, Coimbra TM, et al. Propargylglycine decreases neuro-immune interaction inducing pain response in temporomandibular joint inflammation model. Nitric Oxide. (2019) 93:90–101. doi: 10.1016/j.niox.2019.10.001
250. Bradman MJ, Ferrini F, Salio C, Merighi A. Practical mechanical threshold estimation in rodents using von frey hairs/semmes–weinstein monofilaments: towards a rational method. J Neurosci Methods. (2015) 255:92–103. doi: 10.1016/j.jneumeth.2015.08.010
251. Cao Y, Xie Q-F, Li K, Light AR, Fu K-Y. Experimental occlusal interference induces long-term masticatory muscle hyperalgesia in rats. Pain. (2009) 144(3):287–93. doi: 10.1016/j.pain.2009.04.029
252. Liu MG, Matsuura S, Shinoda M, Honda K, Suzuki I, Shibuta K, et al. Metabotropic glutamate receptor 5 contributes to inflammatory tongue pain via extracellular signal-regulated kinase signaling in the trigeminal spinal subnucleus caudalis and upper cervical spinal cord. J Neuroinflammation. (2012) 9:258. doi: 10.1186/1742-2094-9-258
253. Kogawa EM, Calderon PS, Lauris JR, Araujo CR, Conti PC. Evaluation of maximal bite force in temporomandibular disorders patients. J Oral Rehabil. (2006) 33(8):559–65. doi: 10.1111/j.1365-2842.2006.01619.x
254. Kroon GW, Naeije M. Electromyographic evidence of local muscle fatigue in a subgroup of patients with myogenous craniomandibular disorders. Arch Oral Biol. (1992) 37(3):215–8. doi: 10.1016/0003-9969(92)90091-L
255. Nies M, Ro JY. Bite force measurement in awake rats. Brain Res Brain Res Protoc. (2004) 12(3):180–5. doi: 10.1016/j.brainresprot.2003.11.003
256. Chen Y, Williams SH, McNulty AL, Hong JH, Lee SH, Rothfusz NE, et al. Temporomandibular joint pain: a critical role for Trpv4 in the trigeminal ganglion. Pain. (2013) 154(8):1295–304. doi: 10.1016/j.pain.2013.04.004
257. Ter-Avetisyan G, Dumoulin A, Herrel A, Schmidt H, Strump J, Afzal S, et al. Loss of axon bifurcation in mesencephalic trigeminal neurons impairs the maximal biting force in Npr2-deficient mice. Front Cell Neurosci. (2018) 12:153. doi: 10.3389/fncel.2018.00153
258. Luo X, He T, Wang Y, Wang JL, Yan XB, Zhou HC, et al. Ceftriaxone relieves trigeminal neuropathic pain through suppression of spatiotemporal synaptic plasticity via restoration of glutamate transporter 1 in the medullary dorsal horn. Front Cell Neurosci. (2020) 14:199. doi: 10.3389/fncel.2020.00199
259. Suzuki I, Tsuboi Y, Shinoda M, Shibuta K, Honda K, Katagiri A, et al. Involvement of ERK phosphorylation of trigeminal spinal subnucleus caudalis neurons in thermal hypersensitivity in rats with infraorbital nerve injury. PLoS One. (2013) 8(2):e57278. doi: 10.1371/journal.pone.0057278
260. Liang Y-C, Huang C-C, Hsu K-S. The synthetic cannabinoids attenuate allodynia and hyperalgesia in a rat model of trigeminal neuropathic pain. Neuropharmacology. (2007) 53(1):169–77. doi: 10.1016/j.neuropharm.2007.04.019
261. Zhang ZJ, Dong YL, Lu Y, Cao S, Zhao ZQ, Gao YJ. Chemokine CCL2 and its receptor CCR2 in the medullary dorsal horn are involved in trigeminal neuropathic pain. J Neuroinflammation. (2012) 9:136. doi: 10.1186/1742-2094-9-136
262. Urano H, Ara T, Fujinami Y, Hiraoka BY. Aberrant TRPV1 expression in heat hyperalgesia associated with trigeminal neuropathic pain. Int J Med Sci. (2012) 9(8):690–7. doi: 10.7150/ijms.4706
263. Bagüés A, Martín-Fontelles MI, Esteban-Hernández J, Sánchez-Robles EM. Characterization of the nociceptive effect of carrageenan: masseter versus gastrocnemius. Muscle Nerve. (2017) 56(4):804–13. doi: 10.1002/mus.25538
264. Constandil L, Goich M, Hernández A, Bourgeais L, Cazorla M, Hamon M, et al. Cyclotraxin-B, a new TrkB antagonist, and glial blockade by propentofylline, equally prevent and reverse cold allodynia induced by BDNF or partial infraorbital nerve constriction in mice. J Pain. (2012) 13(6):579–89. doi: 10.1016/j.jpain.2012.03.008
265. Sadler KE, Mogil JS, Stucky CL. Innovations and advances in modelling and measuring pain in animals. Nat Rev Neurosci. (2022) 23(2):70–85. doi: 10.1038/s41583-021-00536-7
266. Dolensek N, Gehrlach DA, Klein AS, Gogolla N. Facial expressions of emotion states and their neuronal correlates in mice. Science. (2020) 368(6486):89–94. doi: 10.1126/science.aaz9468
267. Schneider LE, Henley KY, Turner OA, Pat B, Niedzielko TL, Floyd CL. Application of the rat grimace scale as a marker of supraspinal pain sensation after cervical spinal cord injury. J Neurotrauma. (2017) 34(21):2982–93. doi: 10.1089/neu.2016.4665
268. Rea BJ, Wattiez A-S, Waite JS, Castonguay WC, Schmidt CM, Fairbanks AM, et al. Peripherally administered CGRP induces spontaneous pain in mice: implications for migraine. Pain. (2018) 159(11):2306. doi: 10.1097/j.pain.0000000000001337
269. Langford DJ, Bailey AL, Chanda ML, Clarke SE, Drummond TE, Echols S, et al. Coding of facial expressions of pain in the laboratory mouse. Nat Methods. (2010) 7(6):447–9. doi: 10.1038/nmeth.1455
270. Sotocina SG, Sorge RE, Zaloum A, Tuttle AH, Martin LJ, Wieskopf JS, et al. The rat grimace scale: a partially automated method for quantifying pain in the laboratory rat via facial expressions. Mol Pain. (2011) 7:1744–8069. doi: 10.1186/1744-8069-7-55
271. Tuttle AH, Molinaro MJ, Jethwa JF, Sotocinal SG, Prieto JC, Styner MA, et al. A deep neural network to assess spontaneous pain from mouse facial expressions. Mol Pain. (2018) 14:1744806918763658. doi: 10.1177/1744806918763658
272. Kerins CA, Carlson DS, McIntosh JE, Bellinger LL. Meal pattern changes associated with temporomandibular joint inflammation/pain in rats; analgesic effects. Pharmacol Biochem Behav. (2003) 75(1):181–9. doi: 10.1016/S0091-3057(03)00072-8
274. Romero-Reyes M, Akerman S, Nguyen E, Vijjeswarapu A, Hom B, Dong HW, et al. Spontaneous behavioral responses in the orofacial region: a model of trigeminal pain in mouse. Headache. (2013) 53(1):137–51. doi: 10.1111/j.1526-4610.2012.02226.x
275. Chesler EJ, Wilson SG, Lariviere WR, Rodriguez-Zas SL, Mogil JS. Identification and ranking of genetic and laboratory environment factors influencing a behavioral trait, thermal nociception, via computational analysis of a large data archive. Neurosci Biobehav Rev. (2002) 26(8):907–23. doi: 10.1016/S0149-7634(02)00103-3
276. Neubert JK, King C, Malphurs W, Wong F, Weaver JP, Jenkins AC, et al. Characterization of mouse orofacial pain and the effects of lesioning TRPV1-expressing neurons on operant behavior. Mol Pain. (2008) 4:43. doi: 10.1186/1744-8069-4-43
277. Rossi HL, Vierck CJ Jr., Caudle RM, Neubert JK. Characterization of cold sensitivity and thermal preference using an operant orofacial assay. Mol Pain. (2006) 2:37. doi: 10.1186/1744-8069-2-37
278. Al Sayegh S, Borgwardt A, Svensson KG, Kumar A, Grigoriadis A, Christidis N. Effects of chronic and experimental acute masseter pain on precision biting behavior in humans. Front Physiol. (2019) 10:1369. doi: 10.3389/fphys.2019.01369
279. Dworkin SF, LeResche L. Research diagnostic criteria for temporomandibular disorders: review, criteria, examinations and specifications, critique. J Craniomandib Disord. (1992) 6(4):301–55.1298767
280. Dao TT, Lund JP, Lavigne GJ. Pain responses to experimental chewing in myofascial pain patients. J Dent Res. (1994) 73(6):1163–7. doi: 10.1177/00220345940730060601
281. Gavish A, Winocur E, Menashe S, Halachmi M, Eli I, Gazit E. Experimental chewing in myofascial pain patients. J Orofac Pain. (2002) 16(1):22–8.11889656
282. Naik K, Janal MN, Chen J, Bandary D, Brar B, Zhang S, et al. The histopathology of oral cancer pain in a mouse model and a human cohort. J Dent Res. (2021) 100(2):194–200. doi: 10.1177/0022034520961020
283. Scheff NN, Wall IM, Nicholson S, Williams H, Chen E, Tu NH, et al. Oral cancer induced TRPV1 sensitization is mediated by PAR(2) signaling in primary afferent neurons innervating the cancer microenvironment. Sci Rep. (2022) 12(1):4121. doi: 10.1038/s41598-022-08005-6
284. Horan NL, McIlvried LA, Atherton MA, Yuan MM, Dolan JC, Scheff NN. The impact of tumor immunogenicity on cancer pain phenotype using syngeneic oral cancer mouse models. Front Pain Res (Lausanne). (2022) 3:991725. doi: 10.3389/fpain.2022.991725
285. Bai Q, Liu S, Shu H, Tang Y, George S, Dong T, et al. TNFalpha in the trigeminal nociceptive system is critical for temporomandibular joint pain. Mol Neurobiol. (2019) 56(1):278–91. doi: 10.1007/s12035-018-1076-y
286. Furuse T, Blizard DA, Moriwaki K, Miura Y, Yagasaki K, Shiroishi T, et al. Genetic diversity underlying capsaicin intake in the mishima battery of mouse strains. Brain Res Bull. (2002) 57(1):49–55. doi: 10.1016/S0361-9230(01)00636-0
287. Simons CT, Dessirier JM, Jinks SL, Carstens E. An animal model to assess aversion to intra-oral capsaicin: increased threshold in mice lacking substance p. Chem Senses. (2001) 26(5):491–7. doi: 10.1093/chemse/26.5.491
288. Meloto CB, Slade GD, Lichtenwalter RN, Bair E, Rathnayaka N, Diatchenko L, et al. Clinical predictors of persistent temporomandibular disorder in people with first-onset temporomandibular disorder: a prospective case-control study. J Am Dent Assoc. (2019) 150(7):572–81.e10. doi: 10.1016/j.adaj.2019.03.023
289. Tovote P, Esposito MS, Botta P, Chaudun F, Fadok JP, Markovic M, et al. Midbrain circuits for defensive behaviour. Nature. (2016) 534(7606):206–12. doi: 10.1038/nature17996
290. Lin YT, Yu Z, Tsai SC, Hsu PH, Chen JC. Neuropeptide FF receptor 2 inhibits capsaicin-induced CGRP upregulation in mouse trigeminal ganglion. J Headache Pain. (2020) 21(1):87. doi: 10.1186/s10194-020-01152-z
291. Fried K, Hansson PT. Animal models of trigeminal neuralgia: a commentary. Mol Pain. (2020) 16:1744806920980538. doi: 10.1177/1744806920980538
292. Alvarenga-Brant R, Costa FO, Mattos-Pereira G, Esteves-Lima RP, Belem FV, Lai H, et al. Treatments for burning mouth syndrome: a network meta-analysis. J Dent Res. (2022) 102(2):220345221130025. doi: 10.1177/00220345221130025
293. Gao X, Zhang D, Xu C, Li H, Caron KM, Frenette PS. Nociceptive nerves regulate haematopoietic stem cell mobilization. Nature. (2021) 589(7843):591–6. doi: 10.1038/s41586-020-03057-y
294. Greenspan JD, Slade GD, Bair E, Dubner R, Fillingim RB, Ohrbach R, et al. Pain sensitivity and autonomic factors associated with development of TMD: the OPPERA prospective cohort study. J Pain. (2013) 14(12 Suppl):T63–74. e1–6. doi: 10.1016/j.jpain.2013.06.007
295. Fernandez-de-las-Penas C, Galan-del-Rio F, Fernandez-Carnero J, Pesquera J, Arendt-Nielsen L, Svensson P. Bilateral widespread mechanical pain sensitivity in women with myofascial temporomandibular disorder: evidence of impairment in central nociceptive processing. J Pain. (2009) 10(11):1170–8. doi: 10.1016/j.jpain.2009.04.017
296. Li Y, Yang G, Zhai X, Kang Y, Xie QF. Somatosensory and trigeminal pathway abnormalities in Chinese patients with trigeminal neuralgia. Odontology. (2022) 111(1):217–227. doi: 10.1007/s10266-022-00737-1
297. Sinay VJ, Bonamico LH, Dubrovsky A. Subclinical sensory abnormalities in trigeminal neuralgia. Cephalalgia. (2003) 23(7):541–4. doi: 10.1046/j.1468-2982.2003.00581.x
298. Granot M, Nagler RM. Association between regional idiopathic neuropathy and salivary involvement as the possible mechanism for oral sensory complaints. J Pain. (2005) 6(9):581–7. doi: 10.1016/j.jpain.2005.03.010
299. Svensson P, Bjerring P, Arendt-Nielsen L, Kaaber S. Sensory and pain thresholds to orofacial argon laser stimulation in patients with chronic burning mouth syndrome. Clin J Pain. (1993) 9(3):207–15. doi: 10.1097/00002508-199309000-00009
300. Puhakka A, Forssell H, Soinila S, Virtanen A, Roytta M, Laine M, et al. Peripheral nervous system involvement in primary burning mouth syndrome–results of a pilot study. Oral Dis. (2016) 22(4):338–44. doi: 10.1111/odi.12454
301. Jaaskelainen SK, Forssell H, Tenovuo O. Abnormalities of the blink reflex in burning mouth syndrome. Pain. (1997) 73(3):455–60. doi: 10.1016/S0304-3959(97)00140-1
302. Zhang X, Wang Y, Chen J, Kim SH, Baldi R, Nackley AG, editors. Novel model of functional pain syndromes that integrates COMT genetic background, stress, and Minor surgery. Abstract presented at the international association for the study of pain (IASP) 2020 world congress on pain (2021). Virtual Event.
303. De La Torre Canales G, Camara-Souza MB, Munoz Lora VRM, Guarda-Nardini L, Conti PCR, Rodrigues Garcia RM, et al. Prevalence of psychosocial impairment in temporomandibular disorder patients: a systematic review. J Oral Rehabil. (2018) 45(11):881–9. doi: 10.1111/joor.12685
304. Resende C, Rocha L, Paiva RP, Cavalcanti CDS, Almeida EO, Roncalli AG, et al. Relationship between anxiety, quality of life, and sociodemographic characteristics and temporomandibular disorder. Oral Surg Oral Med Oral Pathol Oral Radiol. (2020) 129(2):125–32. doi: 10.1016/j.oooo.2019.10.007
305. Wu TH, Hu LY, Lu T, Chen PM, Chen HJ, Shen CC, et al. Risk of psychiatric disorders following trigeminal neuralgia: a nationwide population-based retrospective cohort study. J Headache Pain. (2015) 16:64. doi: 10.1186/s10194-015-0548-y
306. Macianskyte D, Januzis G, Kubilius R, Adomaitiene V, Sciupokas A. Associations between chronic pain and depressive symptoms in patients with trigeminal neuralgia. Medicina (Kaunas). (2011) 47(7):386–92. doi: 10.3390/medicina47070055
307. Galli F, Lodi G, Sardella A, Vegni E. Role of psychological factors in burning mouth syndrome: a systematic review and meta-analysis. Cephalalgia. (2017) 37(3):265–77. doi: 10.1177/0333102416646769
308. Bergdahl J, Anneroth G, Perris H. Personality characteristics of patients with resistant burning mouth syndrome. Acta Odontol Scand. (1995) 53(1):7–11. doi: 10.3109/00016359509005936
309. Eli I, Baht R, Littner MM, Kleinhauz M. Detection of psychopathologic trends in glossodynia patients. Psychosom Med. (1994) 56(5):389–94. doi: 10.1097/00006842-199409000-00002
310. Taiminen T, Kuusalo L, Lehtinen L, Forssell H, Hagelberg N, Tenovuo O, et al. Psychiatric (axis I) and personality (axis II) disorders in patients with burning mouth syndrome or atypical facial pain. Scand J Pain. (2011) 2(4):155–60. doi: 10.1016/j.sjpain.2011.06.004
311. Arras M, Rettich A, Cinelli P, Kasermann HP, Burki K. Assessment of post-laparotomy pain in laboratory mice by telemetric recording of heart rate and heart rate variability. BMC Vet Res. (2007) 3(1):1–10. doi: 10.1186/1746-6148-3-16
312. Boissy A, Manteuffel G, Jensen MB, Moe RO, Spruijt B, Keeling LJ, et al. Assessment of positive emotions in animals to improve their welfare. Physiol Behav. (2007) 92(3):375–97. doi: 10.1016/j.physbeh.2007.02.003
313. Jirkof P. Burrowing and nest building behavior as indicators of well-being in mice. J Neurosci Methods. (2014) 234:139–46. doi: 10.1016/j.jneumeth.2014.02.001
314. Jirkof P, Cesarovic N, Rettich A, Nicholls F, Seifert B, Arras M. Burrowing behavior as an indicator of post-laparotomy pain in mice. Front Behav Neurosci. (2010) 4:165. doi: 10.3389/fnbeh.2010.00165
315. Breivik H, Collett B, Ventafridda V, Cohen R, Gallacher D. Survey of chronic pain in Europe: prevalence, impact on daily life, and treatment. Eur J Pain. (2006) 10(4):287–333. doi: 10.1016/j.ejpain.2005.06.009
316. Dueñas M, Ojeda B, Salazar A, Mico JA, Failde I. A review of chronic pain impact on patients, their social environment and the health care system. J Pain Res. (2016) 9:457. doi: 10.2147/JPR.S105892
317. Fortier MA, Chou J, Maurer EL, Kain ZN. Acute to chronic postoperative pain in children: preliminary findings. J Pediatr Surg. (2011) 46(9):1700–5. doi: 10.1016/j.jpedsurg.2011.03.074
318. Moisak GI, Amelina EV, Zubok NA, Rzaev JA. Psychological status before and after surgery in patients with trigeminal neuralgia. Clin Neurol Neurosurg. (2021) 203:106578. doi: 10.1016/j.clineuro.2021.106578
Keywords: orofacial pain, primary pain, preclinical studies & animal models, temporomandibular disorder, trigeminal neuralgia, burning mouth syndrome (BMS)
Citation: Sadighparvar S, Al-Hamed FS, Sharif-Naeini R and Meloto CB (2023) Preclinical orofacial pain assays and measures and chronic primary orofacial pain research: where we are and where we need to go. Front. Pain Res. 4:1150749. doi: 10.3389/fpain.2023.1150749
Received: 24 January 2023; Accepted: 11 April 2023;
Published: 24 May 2023.
Edited by:
Lene Baad-Hansen, Aarhus University, DenmarkReviewed by:
Nicole N Scheff, University of Pittsburgh, United States© 2023 Sadighparvar, Al Hamed, Sharif-Naeini and Meloto. This is an open-access article distributed under the terms of the Creative Commons Attribution License (CC BY). The use, distribution or reproduction in other forums is permitted, provided the original author(s) and the copyright owner(s) are credited and that the original publication in this journal is cited, in accordance with accepted academic practice. No use, distribution or reproduction is permitted which does not comply with these terms.
*Correspondence: Carolina Beraldo Meloto Y2Fyb2wubWVsb3RvQG1jZ2lsbC5jYQ==
Disclaimer: All claims expressed in this article are solely those of the authors and do not necessarily represent those of their affiliated organizations, or those of the publisher, the editors and the reviewers. Any product that may be evaluated in this article or claim that may be made by its manufacturer is not guaranteed or endorsed by the publisher.
Research integrity at Frontiers
Learn more about the work of our research integrity team to safeguard the quality of each article we publish.