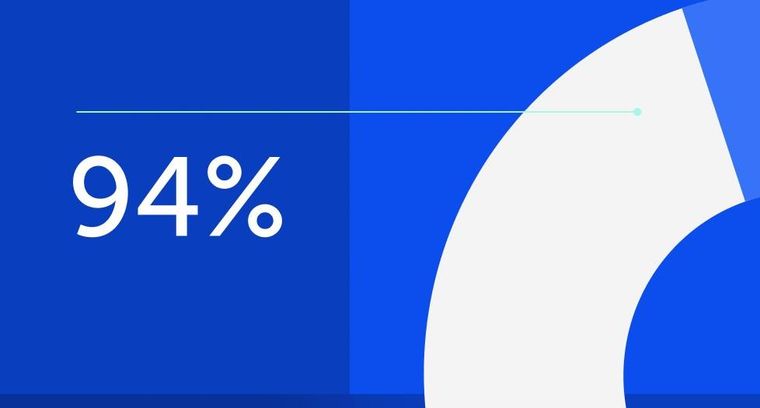
94% of researchers rate our articles as excellent or good
Learn more about the work of our research integrity team to safeguard the quality of each article we publish.
Find out more
MINI REVIEW article
Front. Pain Res., 13 December 2022
Sec. Neuropathic Pain
Volume 3 - 2022 | https://doi.org/10.3389/fpain.2022.1066069
This article is part of the Research TopicInsights in Neuropathic Pain: 2022View all 7 articles
Neuropathic pain is a frequent complication of chemotherapy-induced peripheral neurotoxicity (CIPN). Chemotherapy-induced peripheral neuropathies may serve as a model to study mechanisms of neuropathic pain, since several other common causes of peripheral neuropathy like painful diabetic neuropathy may be due to both neuropathic and non-neuropathic pain mechanisms like ischemia and inflammation. Experimental studies are ideally suited to study changes in morphology, phenotype and electrophysiologic characteristics of primary afferent neurons that are affected by chemotherapy and to correlate these changes to behaviors reflective of evoked pain, mainly hyperalgesia and allodynia. However, hyperalgesia and allodynia may only represent one aspect of human pain, i.e., the sensory-discriminative component, while patients with CIPN often describe their pain using words like annoying, tiring and dreadful, which are affective-emotional descriptors that cannot be tested in experimental animals. To understand why some patients with CIPN develop neuropathic pain and others not, and which are the components of neuropathic pain that they are experiencing, experimental and clinical pain research should be combined. Emerging evidence suggests that changes in subsets of primary afferent nerve fibers may contribute to specific aspects of neuropathic pain in both preclinical models and in patients with CIPN. In addition, the role of cutaneous neuroimmune interactions is considered. Since obtaining dorsal root ganglia and peripheral nerves in patients is problematic, analyses performed on skin biopsies from preclinical models as well as patients provide an opportunity to study changes in primary afferent nerve fibers and to associate these changes to human pain. In addition, other biomarkers of small fiber damage in CIPN, like corneal confocal microscope and quantitative sensory testing, may be considered.
Neuropathic pain is defined as “pain that arises as a direct consequence of a lesion or disease affecting the somatosensory system” (https://www.iasp-pain.org/advocacy/global-year/neuropathic-pain/) (1). It is recognized as the most common neurologic complication in patients treated with cisplatin, oxaliplatin, taxanes like paclitaxel, vinca-alkaloids, proteasome inhibitors like bortezomib and immune-modulatory drugs like thalidomide (2–4). The prevailing hypothesis is that axonal swellings, containing accumulations of mitochondria, usually occur early in the course of distal symmetric peripheral neuropathies, while (epi)dermal nerve fiber loss and degenerative Schwann cell changes occur as late consequences (5–7). Kroigard and colleagues (8–10) have described in detail clinical characteristics and the results from nerve conduction studies (NCS), quantitative sensory testing (QST) and IENFD measurements in patients with oxaliplatin or docetaxel-induced peripheral neuropathy. IENFD measurements using PGP9.5 immunohistochemistry provide a robust, objective and minimally invasive way to quantify epidermal innervation. It does, however, suffer from suboptimal sensitivity and lack of scalability/labor intensiveness, although the latter can potentially be overcome in the future using automated deep learning algorithms.
QST is a method to detect sensory deficits and evoked neuropathic pain, which usually includes mechanical, thermal, or pressure pain threshold. A reduction in different nerve fiber populations is related to a deficit of specific nerve fibre sensitivities. In particular, Abeta-fibre loss is associated with loss of vibration perception, light touch sensation and elevated mechanical detection thresholds. Heat detection and heat pain thresholds provide valuable information about C-fibre loss. Finally, Adelta-fibre loss is linked to decreased pinprick stimuli, mechanical pain and cold detection (11).
Apart from intraepidermal nerve fiber density and QST, there are other biomarkers of chemotherapy-induced peripheral neuropathy (CIPN), like neurofilament light chain for axonal damage (12–14) and corneal confocal microscopy (CCM). The latter is a non-invasive ophthalmologic imaging technique which quantifies small nerve fibre abnormalities in the sub-basal nerve plexus of the cornea (11).
Diagnostic sensitivity and several associations between the severity of intraepidermal nerve fiber loss, severity of NCS or QST abnormalities in oxaliplatin-treated patients vs. neurpathic pain were studied (8–10). Regarding CCM, different studies have shown corneal nerve microstructure and corneal sensitivity changes in patients receiving oxaliplatin (15). In addition, imaging of the corneal sub basal nerve plexus was reported as biomarker for nerve regeneration in CIPN (16). On the other hand, in oxaliplatin- and docetaxel-induced polyneuropathy changes in QST, but not in CCM, were reported (17). Neuropathic pain may arise as a consequence of loss and/or degeneration of nerve fibers, from hyperexcitability of spared and sensitized nociceptive afferents as well as from reinnervation (18).
In CIPN nor in other neuropathic pain conditions few studies have confirmed positive or negative associations between cutaneous innervation and the severity of neuropathic pain (19, 20), while many described no consistent correlations (21–24). IENF density and onset of neuropathic pain behaviors is not consistent across neuropathic pain models. One reason may be mixed pathology, for example in painful diabetic neuropathy where ischemic pain may be superimposed on purely neuropathic pain. Another reason may be the fact that selective degeneration of subsets of intraepidermal nociceptive fibers (IENF), which cannot be detected using the pan axonal marker PGP9.5, may drive hypersensitivity and has been linked to neuropathic pain. Although PGP9.5 labeling can be obtained by simple indirect immunofluorescence and bright-field immunohistochemistry on free-floating sections for IENF density quantification (25), it is important to remember that using immunolabeling of PGP 9.5 it is possible to detect intact free intraepidermal nerve fibers endings (see Figure 1), but without any distinction in various subtypes of sensory nerve fibers (27). It is known that the primary afferent sensory fibers in the epidermis and dermal layers of the skin can be structurally defined as Aβ, A∂ and C-fibers (28). In addition to this morphological classification, neurofilament 200 (NF200) is a typical immunohistochemical marker of myelinated fibers (29). C-fibers can be further subdivided using immunohistochemical/molecular markers such as calcitonin gene related peptide (CGRP), substance P, ion channels, transient receptor potential (TRP) family receptors (like the vanilloid receptor 1 TRPV1) and endocannabinoid receptors (like CB1) (30). These markers define the subclass of peptidergic nociceptors (named so, because they contain neuropeptides). In contrast, non-peptidergic nociceptors are C-fibers with free endings in the epidermis which are often identified by the binding isolectin B4 (IB4) or through expression of the purinergic receptor P2X3 (28–31). These subgroups of C-fibers may convey differential susceptibility to toxic injury, possibly associated with neuropathic pain. Peptidergic and nonpeptidergic nerve fibers have different innervation patterns in the epidermis and dermis (32), in particular there is a much denser innervation of the epidermis by P2X3-IR fibers in comparison with the peptidergic or CGRP positive ones (33, 34).
Figure 1. PGP 9.5 immunohistochemical staining free intraepidermal nerve fibers endings. Representative images of skin biopsy from a control (A), higher magnification in (B) and PTX-treated rats (C) after 4 weeks of treatment (bar A and C = 100 μm, bar B = 50 μm). PGP9.5 immunohistochemical staining was performed to measure the intraepidermal nerve fibers (IENF) density using a previously published protocol (26). Briefly, glabrous skin from the plantar hindpaw were fixed, cryoprotected and serially cut in 20 μm-thick sections. Sections were immunostained with rabbit polyclonal anti-protein gene product 9.5 (PGP 9.5; ProteinTech, Manchester, United Kingdom) using a free-floating protocol. The total number of PGP 9.5-positive IENF crossing the dermal-epidermal junction was counted under a light microscope at 40× magnification and then the IENF density was expressed as number IENF/length of epidermis (mm).
In contrast to experimental animal models of neuropathic pain, clinical pain research has the advantage to measure spontaneous pain instead of nociceptive reflexes (8, 9). Besides, humans have the ability to talk and thus differentiate between specific neuropathic pain components. Since the epidermis is predominantly innervated by non-peptidergic nociceptors (35) and since neuropathic pain is often qualified as both superficial as well as annoying, tring, dreadful etc., it was suggested that the predominant fibers in the most superficial layer of the skin (i.e., epidermal non-peptidergic nerve fibers) are correlated with the affective component of neuropathic pain (36). The McGill Pain questionnaire (37, 38) is a reliable and extensively validated test in many languages that was specifically designed to discern the sensory-discriminative, affective and evaluative components of neuropathic pain. Bechakra and colleagues recently published two papers, one in a rat-model of nerve-injury induced pain (34) and one in patients with bortezomib-induced peripheral neuropathy (BIPN) (39), in which they suggested that selective degeneration of non-peptidergic nerve fibers may directly or indirectly (via parasympathetic sprouting) (40), contribute to the affective and evaluative component of neuropathic pain in patients with BIPN. Impaired regeneration of peptidergic nerve fibers on the other hand may contribute to the sensory-discriminative component of neuropathic pain in BIPN patients (39). The situation may be different in more chronic neuropathies like (painful) diabetic neuropathy and chronic idiopathic axonal polyneuropathy, in which neuropathic pain intensity appears to be associated with increased (epidermal) sprouting of CGRP fibers (41, 42). It has been suggested that separate anatomical pathways exist for these respective components (26, 43), the so-called lateral and medial pain system (44). A schematic presentation of the medial and lateral and medial pain ascending pathways in chemotherapy-induced peripheral neurotoxicity is reported in Figure 2 (evidence is presented in the following citations: 34, 39, 43–50). Since non-peptidergic fibers are overrepresented in the epidermis, and since the degeneration of these fibers seems to be associated with the affective component of neuropathic pain, it is recommended to use a numerical rating scale (NRS) for pain unpleasantness in addition to an NRS for pain intensity for patients with CIPN-associated neuropathic pain.
Figure 2. The lateral and medial pain ascending pathways in chemotherapy-induced peripheral neurotoxicity: afferents of peptidergic and non-peptidergic C-fibres that form free nerve endings in the skin terminate in the superficial lamina of the spinal dorsal horn. In particular, peptidergic C-fibres synapse on lamina I and lamina IIo neurons, whereas non-peptidergic C-fibres synapse on lamina IIi (inter)neurons (39). Those second order pain neurons ascend, directly (lamina I and IIo neurons) (38) or indirectly (lamina IIi neurons, mostly via neurons in lamina V) (36), through the anterolateral system and project to brainstem and forebrain areas, amongst others the parabrachial nuclei and thalamus for peptidergic input, versus the amygdala, hypothalamus and striatal nuclei for non-peptidergic input. Functional MRI and PET studies have shown that separate brain areas process distinctive pain components: sensory-discriminative aspects of pain are processed in the contralateral primary and secondary sensory cortex, while affective components are processed in the hypothalamus, the contralateral amygdala and bilateral anterior cingulate cortex and insula (40, 41, 45). It is suggested that the pathway originating from peptidergic primary afferents (also called the lateral pain system-in green) contributes to the sensory-discriminative aspect of pain, while the pathway originating from non-peptidergic primary afferents (also called the medial pain system-in yellow) contributes to the affective component of the pain experience (34, 38, 39). In line with the clinical observation that patients with CIPN more often complain about unpleasant than about intense pain sensations, the non-peptidergic or lateral pain system may predominate in those patients (27, 32, 42). Abbreviations: K, keratinocytes; S, sympathetic; PS, parasympathetic; DRG, dorsal root ganglion; P, peptidergic sensory neuron; N-P, non peptidergic sensory neuron; I, lamina I of the dorsal horn; IIo, outer part of lamina II of the dorsal horn; IIi, inner part of lamina II of the dorsal horn; V, lamina V; ALS, anterolateral system; PB, parabrachial nuclei; Th, thalamus; H, hypothalamus; Str, striatal nuclei; S1, S2, somatosensory (association) cortex; A, amygdala; ACC, anterior gyrus cinguli; I, insula.
There is a wealth of clinical research on CIPN and of emerging pharmacological strategies for its management, which is reviewed in numerous citations (4, 50–51). However, our review focuses on the relationship between skin innervation and chemotherapy-induced neuropathic pain, which has been studied less extensively. Many studies report the loss of IENF density associated with diabetic neuropathy (52) and CIPN (53, 54), although the relationship with the development of neuropathic pain is unclear (55–58). Intraepidermal nerve terminals, arising from unmyelinated and thinly myelinated somatosensory fibers in the dermis, are exposed to inflammatory mediators which sensitize those peripheral sensory neurons (59) and are thus involved in the pathogenesis of neuropathic pain. The hyperexcitability of sensory neurons induced by the release of the neurotransmitters from their peripheral endings as well as alterations in ion-channel expression in those neurons is subsequently followed by central sensitization via spinal as well as supraspinal mechanisms, the latter involving ascending pathways (60). Together, peripheral and central sensitization mechanisms result in a neuropathic pain phenotype.
Since the pathogenic mechanisms underlying evoked neuropathic pain associated with CIPN development are still not fully understood, in vivo animal models may be useful not only to fill this knowledge gap but also to test promising pharmacological strategies aimed at preventing the development of neuropathy and/or neuropathic pain.
Several rodent models have evidenced that loss of nerve fibers innervating the skin is involved in the initiation and persistence of neuropathic pain, for example in paclitaxel- (56, 61), oxaliplatin- (55), vincristine (62), cisplatin- (63) and bortezomib-induced peripheral neuropathies (4, 64, 65). In the majority of clinical (66) and preclinical studies, skin biopsies (indicative of small nerve fibres damaged in the skin) are used to examine IENF density in the glabrous skin and evaluated by immunostaining using the pan-axonal marker PGP9.5 (67), whereas electrophysiological tests or conventional nerve histology can be performed to examine the pathology in large fiber neuropathies (68). A significant decline in IENF density appears prior to the onset of damage in more proximal nerves and sensory ganglia (69). The immunomodulatory agents minocycline and immunoglobulin, administered 24 h just before and during chemotherapy, were able to prevent mechanical hyperesthesia (exaggerated responses to noxious stimuli)/allodynia (responses to non-noxious stimuli) associated with a decrease in IENF density induced by paclitaxel and oxaliplatin (46, 47) and bortezomib (70).
Patients suffering from CIPN-associated neuropathic pain experience both spontaneous as well as stimulus-dependent pain that manifests as hyperalgesia and allodynia (71). Pain-like behaviours in animal models of CIPN are mainly studied through tests based on stimulus-evoked responses to define the onset, severity and duration of mechanical allodynia and/or thermal hyperalgesia (72). As mentioned earlier, the association between pain-like behaviours and IENF density is not clear, i.e., some studies suggest that neuropathic pain behavior is associated with increased IENF density, while other studies suggest an inverse correlation. This may be related to re-innervation in epidermis and dermis of rats after nerve injury, selective degeneration of subsets of nociceptors (62, 73) and to the fact that specific subsets of nociceptors serve specific aspects of neuropathic pain (34).
Following a sciatic nerve lesion in rats, peptidergic nerve fibers demonstrated early and increased nerve sprouting from uninjured afferents resulting in a faster restoration of nociception and increased IENF density (74) as compared to non-peptidergic primary afferents, which took more time to recover than the peptidergic ones in a chronic constriction injury model (75). In addition, changes in the interactions between autonomic fiber types (i.e., sympathetic vs. parasympathetic) and sensory fibers occur in rat skin after peripheral nerve injury, suggesting involvement of autonomic nerve fibers in neuropathic pain mechanisms (76, 77). Regarding CIPN, it has been shown that paclitaxel induces the degeneration of CGRP and Substance P positive peptidergic nerve fiber terminals in the skin, leading to the onset of pain-like behaviors (73). However, other authors reported that vincristine induces a reduction of nonpeptidergic IENF density while it did not affect pepdidergic nerve fibers, resulting in the development of mechanical allodynia as a consequence of selective damage of vincristine to nonpeptidergic population (62). Thus, epidermal nerve fiber denervation and regeneration are probably not the only factors involved in the pathogenesis of neuropathic pain. Data from the literature suggest a possible key role of mitochondrial dysfunction and cutaneous neuroimmune interactions.
In order to maintain epidermis integrity, IENFs are continuously subjected to a process of remodelling (78). This process implicates a high energy demand and may explain the high vulnerability of IENFs to the mitotoxic effects of antineoplastic agents (7). In the last few years, several preclinical studies have shown the effectiveness of pharmacological strategies aimed to improve mitochondrial abnormalities in reducing and or preventing CIPN and CIPN-associated neuropathic pain (79). This evidence led to the hypothesis that the mitochondrial dysfunction induced by antineoplastic agents is implicated in the onset and maintenance of CIPN (7). The prevailing idea is that chemotherapy can directly or indirectly cause damage to mitochondria that lead to a reduction in cellular bioenergetic capacity and an increased release of nitric oxide and superoxide. Nitro-oxidative stress and mitochondrial dysfunction can induce IENF degeneration and an increase in spontaneous discharges of damaged IENFs leading to neuropathic pain (79). For instance, Ma et al. described a cisplatin-induced peripheral neurotoxicity mouse model, in which they demonstrated that loss of IENF is correlated with bioenergetic deficits in peripheral nerves (80). In addition, Shim et al. reported cisplatin-induced mechanical hypersensitivity due to peripheral oxidative stress, sensitizing mechanical nociceptors (81). In line with this hypothesis, pharmacological agents such as pifithrin-µ (82, 83) that prevent p53 mitochondrial accumulation, as well as HDAC6 inhibitors (84) like metformin (85) and other compounds acting through reduction of nitro-oxidative stress (64), have shown to prevent and/or reduce IENF loss.
Apart from associations of neuropathic pain intensity with nerve fiber degeneration or regeneration and mitochondrial dysfunction, a relationship with epidermal macrophages, Schwann cells and keratinocytes have been described in humans with neuropathic pain, although not specifically in patients with CIPN. Cutaneous cells (i.e., keratinocytes, the predominant epidermal cell type) and immune cells (i.e., macrophages, neutrophils, lymphocyte cells, mast cells and Langerhans cells) contribute to peripheral sensitization via their interactions with nociceptors (86).
Keratinocytes express several receptors and ion channels like, for example, TRPV1–4 (87). Similarly, Langerhans cells (88) express, amongst others, T-type calcium channels, interleukin receptors, cannabinoid receptors, calcitonin receptor-like receptor (89–91). As an example of neuro-immune-cutaneous interactions, Cav3.2 T type calcium -channels are abundantly expressed within skin nerve endings thus regulating neuronal excitability and stimulus-evoked pain (92, 93).
Proposed mechanisms by which keratinocytes modulate neighboring neuronal and immune cells include the production and release of several neurotransmitters, chemokines (94), neuropeptides and/or cytokines, both via direct physical relationship between keratinocytes and cutaneous afferent fibers as well as via synaptic-like contacts (95) and/or contact with Schwann cells (96). In complex regional pain syndrome and post-herpetic neuralgia, increased activity and expression of voltage-gated sodium channels (Nav) on keratinocytes resulted in increased epidermal ATP release and excessive activation of P2X receptors on primary sensory axons (97). Moreover, it was reported that paclitaxel-induced keratinocyte damage and ectopic expression of matrix-metalloproteinase 13 (MMP-13) in the skin of a zebrafish model of CIPN is a fundamental event that precedes axonal degeneration (98). The inducers of this event could be mitochondrial damage and reactive oxygen species formation (99), as mentioned earlier. Not surprisingly, selective inhibition of MMP-13 improved skin defects and rescued paclitaxel-induced epithelial damage and neurotoxicity TRPV1 is expressed in a subpopulation of unmyelinated C and thinly myelinated A∂ nociceptors that mediate responsiveness to capsaicin and heat (100). Although little is known about the role of TRPV1 in keratinocytes, a recent study of Pang and colleagues reported that selective keratinocyte stimulation in a conditional TRPV1-knockout mice model, in which TRPV1 was exclusively expressed in keratinocytes, is sufficient to evoke acute nociceptive-related responses (84). Recently, a study described the distribution of cannabinoid receptors in the skin and peripheral nervous system. More specifically, CB1 receptors are expressed on nociceptive nerve endings and dorsal root ganglion neurons, whereas CB2 receptors are expressed on immune cells and keratinocytes (101). Endocannabinoids, such as 2-arachidonoylglycerol (2-AG) and N-arachidonoylethanolamine (AEA), are neurotransmitters derived from membrane phospholipid precursors. Their homeostasis is maintained by transporters and by the activity of fatty acid amide hydrolase (FAAH) and monoacylglycerol lipase (MAGL), enzymes that convert 2-AG to arachidonic acid and glycerol and that convert AEA to arachidonic acid and ethanolamine respectively (102). It has been described that chemotherapy induces increased expression of FAAH and MAGL in the footpads of experimental animals, which subsequently causes a decrease in 2-AG and AEA levels. As a consequence of decreased CB2 receptor activation, there is an increased sensitivity and response to nociceptor-stimulation leading to pain-like behaviors in animal models of CIPN 103). Studies from Ibrahim's laboratory tested the hypothesis that CB2 receptor activation stimulates the release of the endogenous opioid β-endorphin from keratinocytes, acting on primary afferent neurons via opioid receptors, thus reducing nociception (103).
Recent evidence suggests that infiltrating immune cells can facilitate direct neuro-immune interactions (104). Interestingly, Shepherd et al. demonstrated that activation of the angiotensin II receptor on skin macrophages and consequent TRPA1 activation on sensory nerves is correlated with mechanical hypersensitivity and pain sensation (105). In addition, in the presence of IENF degeneration, activation of the skin's resident immune cells, i.e., Langerhans cells, was reported. Recently, murine models of paclitaxel and vincristine-induced painful neuropathies have shown a significant degeneration of IENF concomitant with the activation of PGP 9.5-positive Langerhans cells 106), suggesting a possible neuroimmune interaction mediated through an increased synthesis of proinflammatory cytokines by activated Langerhans cells (107), which are capable of sensitizing IENF terminals by evoking ectopic discharges and sensitizing C fibers (108). Finally, recent evidence has demonstrated that oxaliplatin induces changes in cutaneous mast cells that are correlated with the development of mechanical allodynia in mice (109). In particular, Sakamoto and colleagues reported a significant increase in total number and number of degranulated mast cells after oxaliplatin treatment, leading to the release of serine protease and activation of proteinase-activated receptor 2 (PAR2) in the skin, which caused mechanical allodynia. Similarly, release of tryptase from mast cells and activation of PAR2 in the skin lead to paclitaxel-induced mechanical allodynia through sensitization of TRPV1, TRPV4, TRPA1 receptors on primary afferent sensory neurons (110).
In summary, degeneration and regeneration of unmyelinated C-fibers, mitochondrial dysfunction, keratinocytes, Langerhans cells and other cutaneous immune cells as well as TRP expressed by keratinocyte and sensory nerves, closely participate in sensory transduction and eventually contribute to the development of neuropathic pain in CIPN. In addition, selective epidermal nerve fiber degeneration and regeneration may affect two distinctive pain components, i.e., sensory-discriminatory vs. affective, via the lateral and medial pain systems respectively. Future studies should focus on the complex neuroimmuno interactions in the skin to define a strategy for the development of topical analgesics and neuroprotective drugs.
CM, LM and JLMJ: conceptualized and wrote the review, defined the search strategies and reviewed the literature. CM and JLMJ: reviewed and edited the final manuscript. All authors contributed to the article and approved the submitted version.
This work was funded by Fondazione Cariplo, grant number 2019-1482 and 2020-ATE-0556 from University of Milano-Bicocca to C. Meregalli; and Erasmus MC Grant 2011 to J.L.M. Jongen
The authors are particularly thankful to Dr. A. Chiorazzi and Dr. A. Malacrida for his help in the preparation of Figures.
The authors declare that the research was conducted in the absence of any commercial or financial relationships that could be construed as a potential conflict of interest.
All claims expressed in this article are solely those of the authors and do not necessarily represent those of their affiliated organizations, or those of the publisher, the editors and the reviewers. Any product that may be evaluated in this article, or claim that may be made by its manufacturer, is not guaranteed or endorsed by the publisher.
1. Jensen TS, Baron R, Haanpää M, Kalso E, Loeser JD, Rice ASC, et al. A new definition of neuropathic pain. Pain. (2011) 152(10):2204–5. doi: 10.1016/j.pain.2011.06.017
2. Richardson PG, Delforge M, Beksac M, Wen P, Jongen JL, Sezer O, et al. Management of treatment-emergent peripheral neuropathy in multiple myeloma. Leukemia. (2012) 26(4):595–608. doi: 10.1038/leu.2011.346
3. Rampen AJ, Jongen JL, van Heuvel I, Scheltens-de Boer M, Sonneveld P, van den Bent MJ. Bortezomib-induced polyneuropathy. Neth J Med. (2013);71(3):128–33.23712808
4. Jongen JL, Broijl A, Sonneveld P. Chemotherapy-induced peripheral neuropathies in hematological malignancies. J Neurooncol. (2015) 121(2):229–37. doi: 10.1007/s11060-014-1632-x
5. Lauria G, Morbin M, Lombardi R, Borgna M, Mazzoleni G, Sghirlanzoni A, et al. Axonal swellings predict the degeneration of epidermal nerve fibers in painful neuropathies. Neurology. (2003) 61(5):631–6. doi: 10.1212/01.WNL.0000070781.92512.A4
6. Ebenezer GJ, McArthur JC, Thomas D, Murinson B, Hauer P, Polydefkis M, et al. Denervation of skin in neuropathies: the sequence of axonal and Schwann cell changes in skin biopsies. Brain. (2007) 130(Pt 10):2703–14. doi: 10.1093/brain/awm199
7. Bennett GJ, Doyle T, Salvemini D. Mitotoxicity in distal symmetrical sensory peripheral neuropathies. Nat Rev Neurol. (2014) 10(6):326–36. doi: 10.1038/nrneurol.2014.77
8. Krøigård T, Svendsen TK, Wirenfeldt M, Schrøder HD, Qvortrup C, Pfeiffer P, et al. Early changes in tests of peripheral nerve function during oxaliplatin treatment and their correlation with chemotherapy-induced polyneuropathy symptoms and signs. Eur J Neurol. (2020) 27(1):68–76. doi: 10.1111/ene.14035
9. Krøigård T, Svendsen TK, Wirenfeldt M, Schrøder HD, Qvortrup C, Pfeiffer P, et al. Oxaliplatin neuropathy: predictive values of skin biopsy, QST and nerve conduction. J Neuromuscul Dis. (2021) 8(4):679–88. doi: 10.3233/JND-210630
10. Krøigård T, Schrøder HD, Qvortrup C, Eckhoff L, Pfeiffer P, Gaist D, et al. Characterization and diagnostic evaluation of chronic polyneuropathies induced by oxaliplatin and docetaxel comparing skin biopsy to quantitative sensory testing and nerve conduction studies. Eur J Neurol. (2014) 21(4):623–9. doi: 10.1111/ene.12353
11. Burgess J, Ferdousi M, Gosal D, Boon C, Matsumoto K, Marshall A, et al. Chemotherapy-Induced peripheral neuropathy: epidemiology, pathomechanisms and treatment. Oncol Ther. (2021) 9(2):385–450. doi: 10.1007/s40487-021-00168-y
12. Meregalli C, Fumagalli G, Alberti P, Canta A, Carozzi VA, Chiorazzi A, et al. Neurofilament light chain as disease biomarker in a rodent model of chemotherapy induced peripheral neuropathy. Exp Neurol. (2018) 307:129–32. doi: 10.1016/j.expneurol.2018.06.005
13. Meregalli C, Fumagalli G, Alberti P, Canta A, Chiorazzi A, Monza L, et al. Neurofilament light chain: a specific serum biomarker of axonal damage severity in rat models of chemotherapy-induced peripheral neurotoxicity. Arch Toxicol. (2020) 94(7):2517–22. doi: 10.1007/s00204-020-02755-w
14. Velasco R, Argyriou AA, Marco C, Mariotto S, Stradella A, Hernández J, et al. Serum neurofilament levels correlate with electrodiagnostic evidence of axonal loss in paclitaxel-induced peripheral neurotoxicity. J Neurol. (2022). doi: 10.1007/s00415-022-11377-4. [Epub ahead of print]36094631
15. Tyler EF, McGhee CNJ, Lawrence B, Braatvedt GD, Mankowski JL, Oakley JD, et al. Corneal nerve changes observed by in vivo confocal microscopy in patients receiving oxaliplatin for colorectal cancer: the COCO study. J Clin Med. (2022) 11(16):4770. doi: 10.3390/jcm11164770
16. Ferdousi M, Azmi S, Petropoulos IN, Fadavi H, Ponirakis G, Marshall A, et al. Corneal confocal microscopy detects small fibre neuropathy in patients with upper gastrointestinal cancer and nerve regeneration in chemotherapy induced peripheral neuropathy. PLoS One. (2015) 10(10):e0139394. doi: 10.1371/journal.pone.0139394
17. Bennedsgaard K, Ventzel L, Andersen NT, Themistocleous AC, Bennett DL, Jensen TS, et al. Oxaliplatin- and docetaxel-induced polyneuropathy: clinical and neurophysiological characteristics. J Peripher Nerv Syst. (2020) 25(4):377–87. doi: 10.1111/jns.12413
18. Truini A, Biasiotta A, Di Stefano G, Leone C, La Cesa S, Galosi E, et al. Does the epidermal nerve fibre density measured by skin biopsy in patients with peripheral neuropathies correlate with neuropathic pain? Pain. (2014) 155(4):828–32. doi: 10.1016/j.pain.2014.01.022
19. Galosi E, La Cesa S, Di Stefano G, Karlsson P, Fasolino A, Leone C, et al. A pain in the skin. Regenerating nerve sprouts are distinctly associated with ongoing burning pain in patients with diabetes. Eur J Pain. (2018) 22(10):1727–34. doi: 10.1002/ejp.1259
20. Karlsson P, Hincker AM, Jensen TS, Freeman R, Haroutounian S. Structural, functional, and symptom relations in painful distal symmetric polyneuropathies: a systematic review. Pain. (2019) 160(2):286–97. doi: 10.1097/j.pain.0000000000001381
21. Lindenlaub T, Sommer C. Epidermal innervation density after partial sciatic nerve lesion and pain-related behavior in the rat. Acta Neuropathol. (2002) 104(2):137–43. doi: 10.1007/s00401-002-0527-7
22. Vlckova-Moravcova E, Bednarik J, Belobradkova J, Sommer C. Small-fibre involvement in diabetic patients with neuropathic foot pain. Diabet Med. (2008) 25(6):692–9. doi: 10.1111/j.1464-5491.2008.02446.x
23. Kalliomäki M, Kieseritzky JV, Schmidt R, Hägglöf B, Karlsten R, Sjögren N, et al. Structural and functional differences between neuropathy with and without pain? Exp Neurol. (2011) 231(2):199–206. doi: 10.1016/j.expneurol.2011.05.019
24. Schley M, Bayram A, Rukwied R, Dusch M, Konrad C, Benrath J, et al. Skin innervation at different depths correlates with small fibre function but not with pain in neuropathic pain patients. Eur J Pain. (2012) 16(10):1414–25. doi: 10.1002/j.1532-2149.2012.00157.x
25. Nolano M, Biasiotta A, Lombardi R, Provitera V, Stancanelli A, Caporaso G, et al. Epidermal innervation morphometry by immunofluorescence and bright-field microscopy. J Peripher Nerv Syst. (2015) 20(4):387–91. doi: 10.1111/jns.12146
26. Craig AD. Pain mechanisms: labeled lines versus convergence in central processing. Annu Rev Neurosci. (2003) 26:1–30. doi: 10.1146/annurev.neuro.26.041002.131022
27. Lauria G, Faber CG, Cornblath DR. Skin biopsy and small fibre neuropathies: facts and thoughts 30 years later. J Neurol Neurosurg Psychiatry. (2022) 93:915–8. doi: 10.1136/jnnp-2021-327742
28. Crawford LK, Caterina MJ. Functional anatomy of the sensory nervous system: updates from the neuroscience bench. Toxicol Pathol. (2020) 48(1):174–89. doi: 10.1177/0192623319869011
29. Ruscheweyh R, Forsthuber L, Schoffnegger D, Sandkühler J. Modification of classical neurochemical markers in identified primary afferent neurons with abeta-, adelta-, and C-fibers after chronic constriction injury in mice. J Comp Neurol. (2007) 502(2):325–36. doi: 10.1002/cne.21311
30. Huet F, Misery L. Sensitive skin is a neuropathic disorder. Exp Dermatol. (2019) 28(12):1470–3. doi: 10.1111/exd.13991
31. Bradbury EJ, Burnstock G, McMahon SB. The expression of P2X3 purinoreceptors in sensory neurons: effects of axotomy and glial-derived neurotrophic factor. Mol Cell Neurosci. (1998) 12(4-5):256–68. doi: 10.1006/mcne.1998.0719
32. Duraku LS, Hossaini M, Schüttenhelm BN, Holstege JC, Baas M, Ruigrok TJ, et al. Re-innervation patterns by peptidergic substance-P, non-peptidergic P2X3, and myelinated NF-200 nerve fibers in epidermis and dermis of rats with neuropathic pain. Exp Neurol. (2013) 241:13–24. doi: 10.1016/j.expneurol.2012.11.029
33. Taylor AM, Peleshok JC, Ribeiro-da-Silva A. Distribution of P2X(3)-immunoreactive fibers in hairy and glabrous skin of the rat. J Comp Neurol. (2009) 514(6):555–66. doi: 10.1002/cne.22048
34. Bechakra M, Schüttenhelm BN, Pederzani T, van Doorn PA, de Zeeuw CI, Jongen JLM. The reduction of intraepidermal P2X3 nerve fiber density correlates with behavioral hyperalgesia in a rat model of nerve injury-induced pain. J Comp Neurol. (2017) 525(17):3757–68. doi: 10.1002/cne.24302
35. Guedon JG, Longo G, Majuta LA, Thomspon ML, Fealk MN, Mantyh PW. Dissociation between the relief of skeletal pain behaviors and skin hypersensitivity in a model of bone cancer pain. Pain. (2016) 157(6):1239–47. doi: 10.1097/j.pain.0000000000000514
36. Willcockson H, Valtschanoff J. AMPA And NMDA glutamate receptors are found in both peptidergic and non-peptidergic primary afferent neurons in the rat. Cell Tissue Res. (2008) 334(1):17–23. doi: 10.1007/s00441-008-0662-0
37. Melzack R, Torgerson WS. On the language of pain. Anesthesiology. (1971) 34(1):50–9. doi: 10.1097/00000542-197101000-00017
38. Melzack R. The McGill pain questionnaire: from description to measurement. Anesthesiology. (2005) 103(1):199–202. doi: 10.1097/00000542-200507000-00028
39. Bechakra M, Nieuwenhoff MD, van Rosmalen J, Groeneveld GJ, Scheltens-de Boer M, Sonneveld P, et al. Clinical, electrophysiological, and cutaneous innervation changes in patients with bortezomib-induced peripheral neuropathy reveal insight into mechanisms of neuropathic pain. Mol Pain. (2018) 14:1744806918797042. doi: 10.1177/1744806918797042
40. Grelik C, Bennett GJ, Ribeiro-da-Silva A. Autonomic fibre sprouting and changes in nociceptive sensory innervation in the rat lower lip skin following chronic constriction injury. Eur J Neurosci. (2005) 21(9):2475–87. doi: 10.1111/j.1460-9568.2005.04089.x
41. Karlsson P, Provitera V, Caporaso G, Stancanelli A, Saltalamacchia AM, Borreca I, et al. Increased peptidergic fibers as a potential cutaneous marker of pain in diabetic small fiber neuropathy. Pain. (2021) 162(3):778–86. doi: 10.1097/j.pain.0000000000002054
42. Braz JM, Nassar MA, Wood JN, Basbaum AI. Parallel “pain” pathways arise from subpopulations of primary afferent nociceptor. Neuron. (2005) 47(6):787–93. doi: 10.1016/j.neuron.2005.08.015
43. Huang T, Lin SH, Malewicz NM, Zhang Y, Goulding M, LaMotte RH, et al. Identifying the pathways required for coping behaviours associated with sustained pain. Nature. (2019) 565(7737):86–90. doi: 10.1038/s41586-018-0793-8
44. Merighi A. The histology, physiology, neurochemistry and circuitry of the substantia gelatinosa rolandi (lamina II) in mammalian spinal cord. Prog Neurobiol. (2018) 169:91–134. doi: 10.1016/j.pneurobio.2018.06.012
45. Bechakra M, Nieuwenhoff MD, Rosmalen JV, Groeneveld GJ, Huygen F JPM, Zeeuw CI, et al. Pain-related changes in cutaneous innervation of patients suffering from bortezomib-induced, diabetic or chronic idiopathic axonal polyneuropathy. Brain Res. (2020) 1730:146621. doi: 10.1016/j.brainres.2019.146621
46. Kulkarni B, Bentley DE, Elliott R, Youell P, Watson A, Derbyshire SW, et al. Attention to pain localization and unpleasantness discriminates the functions of the medial and lateral pain systems. Eur J Neurosci. (2005) 21(11):3133–42. doi: 10.1111/j.1460-9568.2005.04098.x
47. Bushnell MC, Ceko M, Low LA. Cognitive and emotional control of pain and its disruption in chronic pain. Nat Rev Neurosci. (2013) 14(7):502–11. doi: 10.1038/nrn3516
48. Michelle Welman FHS, Smit AE, Jongen JLM, Tibboel D, van der Geest JN, Holstege JC. Pain experience is somatotopically organized and overlaps with pain anticipation in the human cerebellum. Cerebellum. (2018) 17(4):447–60. eng. doi: 10.1007/s12311-018-0930-9. Cited in: Pubmed; PMID 29480507. doi: 10.1007/s12311-018-0930-9
49. Hsieh JC, Belfrage M, Stone-Elander S, Hansson P, Ingvar M. Central representation of chronic ongoing neuropathic pain studied by positron emission tomography. Pain. (1995) 63(2):225–36. doi: 10.1016/0304-3959(95)00048-W
50. Gu J, Hu M, Gu Z, Yu J, Ji Y, Li L, et al. Bibliometric analysis reveals a 20-year research trend for chemotherapy-induced peripheral neuropathy. Front Neurol. (2022) 12:793663. doi: 10.3389/fneur.2021.793663
51. Argyriou AA, Bruna J, Park SB, Cavaletti G. Emerging pharmacological strategies for the management of chemotherapy-induced peripheral neurotoxicity (CIPN), based on novel CIPN mechanisms. Expert Rev Neurother. (2020) 20(10):1005–16. doi: 10.1080/14737175.2020.1796639
52. Beiswenger KK, Calcutt NA, Mizisin AP. Dissociation of thermal hypoalgesia and epidermal denervation in streptozotocin-diabetic mice. Neurosci Lett. (2008) 442(3):267–72. doi: 10.1016/j.neulet.2008.06.079
53. Meregalli C, Monza L, Chiorazzi A, Scali C, Guarnieri C, Fumagalli G, et al. Human intravenous immunoglobulin alleviates neuropathic symptoms in a rat model of paclitaxel-induced peripheral neurotoxicity. Int J Mol Sci. (2021) 22(3):1058. doi: 10.3390/ijms22031058
54. Meregalli C, Maricich Y, Cavaletti G, Canta A, Carozzi VA, Chiorazzi A, et al. Reversal of bortezomib-induced neurotoxicity by suvecaltamide, a selective T-type ca-channel modulator, in preclinical models. Cancers. (2021) 13(19):5013. doi: 10.3390/cancers13195013
55. Boyette-Davis J, Dougherty PM. Protection against oxaliplatin-induced mechanical hyperalgesia and intraepidermal nerve fiber loss by minocycline. Exp Neurol. (2011) 229(2):353–7. doi: 10.1016/j.expneurol.2011.02.019
56. Boyette-Davis J, Xin W, Zhang H, Dougherty PM. Intraepidermal nerve fiber loss corresponds to the development of taxol-induced hyperalgesia and can be prevented by treatment with minocycline. Pain. (2011) 152(2):308–13. doi: 10.1016/j.pain.2010.10.030
57. Marmiroli P, Riva B, Pozzi E, Ballarini E, Lim D, Chiorazzi A, et al. Susceptibility of different mouse strains to oxaliplatin peripheral neurotoxicity: phenotypic and genotypic insights. PLoS One. (2017) 12(10):e0186250. doi: 10.1371/journal.pone.0186250
58. Bruna J, Alberti P, Calls-Cobos A, Caillaud M, Damaj MI, Navarro X. Methods for in vivo studies in rodents of chemotherapy induced peripheral neuropathy. Exp Neurol. (2020) 03(325):113154. doi: 10.1016/j.expneurol.2019.113154, Declaration of Competing Interest The authors have no conflict of interest to declare.
59. Pinho-Ribeiro FA, Verri WA, Chiu IM. Nociceptor sensory neuron-immune interactions in pain and inflammation. Trends Immunol. (2017) 38(1):5–19. doi: 10.1016/j.it.2016.10.001
60. Colloca L, Ludman T, Bouhassira D, Baron R, Dickenson AH, Yarnitsky D, et al. Neuropathic pain. Nat Rev Dis Primers. (2017) 3:17002. doi: 10.1038/nrdp.2017.2
61. Xiao WH, Bennett GJ. Effects of mitochondrial poisons on the neuropathic pain produced by the chemotherapeutic agents, paclitaxel and oxaliplatin. Pain. (2012) 153(3):704–9. doi: 10.1016/j.pain.2011.12.011
62. Bessaguet F, Danigo A, Bouchenaki H, Duchesne M, Magy L, Richard L, et al. Neuroprotective effect of angiotensin II type 2 receptor stimulation in vincristine-induced mechanical allodynia. Pain. (2018) 159(12):2538–46. doi: 10.1097/j.pain.0000000000001361
63. Uhelski ML, Khasabova IA, Simone DA. Inhibition of anandamide hydrolysis attenuates nociceptor sensitization in a murine model of chemotherapy-induced peripheral neuropathy. J Neurophysiol. (2015) 113(5):1501–10. doi: 10.1152/jn.00692.2014
64. Canta A, Chiorazzi A, Pozzi E, Fumagalli G, Monza L, Meregalli C, et al. Calmangafodipir reduces sensory alterations and prevents intraepidermal nerve fibers loss in a mouse model of oxaliplatin induced peripheral neurotoxicity. Antioxidants. (2020) 9(7):594. doi: 10.3390/antiox9070594
65. Fumagalli G, Monza L, Cavaletti G, Rigolio R, Meregalli C. Neuroinflammatory process involved in different preclinical models of chemotherapy-induced peripheral neuropathy. Front Immunol. (2020) 11:626687. doi: 10.3389/fimmu.2020.626687
66. Nolano M, Tozza S, Caporaso G, Provitera V. Contribution of skin biopsy in peripheral neuropathies. Brain Sci. (2020) 10(12):989. doi: 10.3390/brainsci10120989 Cited in: Pubmed; PMID: 33333929.33333929
67. Mangus LM, Rao DB, Ebenezer GJ. Intraepidermal nerve fiber analysis in human patients and animal models of peripheral neuropathy: a comparative review. Toxicol Pathol. (2020) 48(1):59–70. doi: 10.1177/0192623319855969
68. Herrmann DN, Griffin JW, Hauer P, Cornblath DR, McArthur JC. Epidermal nerve fiber density and sural nerve morphometry in peripheral neuropathies. Neurology. (1999) 53(8):1634–40. doi: 10.1212/WNL.53.8.1634
69. Wozniak KM, Vornov JJ, Wu Y, Liu Y, Carozzi VA, Rodriguez-Menendez V, et al. Peripheral neuropathy induced by microtubule-targeted chemotherapies: insights into acute injury and long-term recovery. Cancer Res. (2018) 78(3):817–29. doi: 10.1158/0008-5472.CAN-17-1467
70. Meregalli C, Marjanovic I, Scali C, Monza L, Spinoni N, Galliani C, et al. High-dose intravenous immunoglobulins reduce nerve macrophage infiltration and the severity of bortezomib-induced peripheral neurotoxicity in rats. J Neuroinflammation. (2018) 15(1):232. doi: 10.1186/s12974-018-1270-x
71. Wu Y, Li J, Zhou J, Feng Y. Dynamic long-term microstructural and ultrastructural alterations in sensory nerves of rats of paclitaxel-induced neuropathic pain. Chin Med J. (2014) 127(16):2945–52.25131233
72. Deuis JR, Dvorakova LS, Vetter I. Methods used to evaluate pain behaviors in rodents. Front Mol Neurosci. (2017) 10:284. doi: 10.3389/fnmol.2017.00284
73. Ko MH, Hu ME, Hsieh YL, Lan CT, Tseng TJ. Peptidergic intraepidermal nerve fibers in the skin contribute to the neuropathic pain in paclitaxel-induced peripheral neuropathy. Neuropeptides. (2014) 48(3):109–17. doi: 10.1016/j.npep.2014.02.001
74. Kambiz S, Duraku LS, Baas M, Nijhuis TH, Cosgun SG, Hovius SE, et al. Long-term follow-up of peptidergic and nonpeptidergic reinnervation of the epidermis following sciatic nerve reconstruction in rats. J Neurosurg. (2015) 123(1):254–69. doi: 10.3171/2014.12.JNS141075
75. Peleshok JC, Ribeiro-da-Silva A. Delayed reinnervation by nonpeptidergic nociceptive afferents of the glabrous skin of the rat hindpaw in a neuropathic pain model. J Comp Neurol. (2011) 519(1):49–63. doi: 10.1002/cne.22500
76. Ramien M, Ruocco I, Cuello AC, St-Louis M, Ribeiro-Da-Silva A. Parasympathetic nerve fibers invade the upper dermis following sensory denervation of the rat lower lip skin. J Comp Neurol. (2004) 469(1):83–95. doi: 10.1002/cne.10998
77. Ruocco I, Cuello AC, Ribeiro-Da-Silva A. Peripheral nerve injury leads to the establishment of a novel pattern of sympathetic fibre innervation in the rat skin. J Comp Neurol. (2000) 422(2):287–96. doi: 10.1002/(SICI)1096-9861(20000626)422:2%3C287::AID-CNE9%3E3.0.CO;2-E
78. Bennett GJ, Liu GK, Xiao WH, Jin HW, Siau C. Terminal arbor degeneration–a novel lesion produced by the antineoplastic agent paclitaxel. Eur J Neurosci. (2011) 33(9):1667–76. doi: 10.1111/j.1460-9568.2011.07652.x
79. Doyle TM, Salvemini D. Mini-Review: mitochondrial dysfunction and chemotherapy-induced neuropathic pain. Neurosci Lett. (2021) 760:136087. doi: 10.1016/j.neulet.2021.136087
80. Ma J, Trinh RT, Mahant ID, Peng B, Matthias P, Heijnen CJ, et al. Cell-specific role of histone deacetylase 6 in chemotherapy-induced mechanical allodynia and loss of intraepidermal nerve fibers. Pain. (2019) 160(12):2877–90. doi: 10.1097/j.pain.0000000000001667
81. Shim HS, Bae C, Wang J, Lee KH, Hankerd KM, Kim HK, et al. Peripheral and central oxidative stress in chemotherapy-induced neuropathic pain. Mol Pain. (2019) 15:1744806919840098. doi: 10.1177/1744806919840098
82. Krukowski K, Nijboer CH, Huo X, Kavelaars A, Heijnen CJ. Prevention of chemotherapy-induced peripheral neuropathy by the small-molecule inhibitor pifithrin-μ. Pain. (2015) 156(11):2184–92. doi: 10.1097/j.pain.0000000000000290
83. Maj MA, Ma J, Krukowski KN, Kavelaars A, Heijnen CJ. Inhibition of mitochondrial p53 accumulation by PFT-μ prevents cisplatin-induced peripheral neuropathy. Front Mol Neurosci. (2017) 10:108. doi: 10.3389/fnmol.2017.00108
84. Krukowski K, Ma J, Golonzhka O, Laumet GO, Gutti T, van Duzer JH, et al. HDAC6 Inhibition effectively reverses chemotherapy-induced peripheral neuropathy. Pain. (2017) 158(6):1126–37. doi: 10.1097/j.pain.0000000000000893
85. Mao-Ying QL, Kavelaars A, Krukowski K, Huo XJ, Zhou W, Price TJ, et al. The anti-diabetic drug metformin protects against chemotherapy-induced peripheral neuropathy in a mouse model. PLoS One. (2014) 9(6):e100701. doi: 10.1371/journal.pone.0100701
86. Lowy DB, Makker PGS, Moalem-Taylor G. Cutaneous neuroimmune interactions in peripheral neuropathic pain states. Front Immunol. (2021) 12:660203. doi: 10.3389/fimmu.2021.660203
87. Luo J, Feng J, Yu G, Yang P, Mack MR, Du J, et al. Transient receptor potential vanilloid 4-expressing macrophages and keratinocytes contribute differentially to allergic and nonallergic chronic itch. J Allergy Clin Immunol. (2018) 141(2):608–619.e7. doi: 10.1016/j.jaci.2017.05.051
88. Kocot-Kępska M, Zajączkowska R, Mika J, Wordliczek J, Dobrogowski J, Przeklasa-Muszyńska A. Peripheral mechanisms of neuropathic pain-the role of neuronal and non-neuronal interactions and their implications for topical treatment of neuropathic pain. Pharmaceuticals. (2021) 14(2):77. doi: 10.3390/ph14020077
89. Hou Q, Barr T, Gee L, Vickers J, Wymer J, Borsani E, et al. Keratinocyte expression of calcitonin gene-related peptide β: implications for neuropathic and inflammatory pain mechanisms. Pain. (2011) 152(9):2036–51. doi: 10.1016/j.pain.2011.04.033
90. Southall MD, Li T, Gharibova LS, Pei Y, Nicol GD, Travers JB. Activation of epidermal vanilloid receptor-1 induces release of proinflammatory mediators in human keratinocytes. J Pharmacol Exp Ther. (2003) 304(1):217–22. doi: 10.1124/jpet.102.040675
91. Denda M, Fujiwara S, Hibino T. Expression of voltage-gated calcium channel subunit alpha1C in epidermal keratinocytes and effects of agonist and antagonists of the channel on skin barrier homeostasis. Exp Dermatol. (2006) 15(6):455–60. doi: 10.1111/j.0906-6705.2006.00430.x
92. Sekiguchi F, Kawabata A. T-type calcium channels: functional regulation and implication in pain signaling. J Pharmacol Sci. (2013) 122(4):244–50. doi: 10.1254/jphs.13R05CP
93. Rose KE, Lunardi N, Boscolo A, Dong X, Erisir A, Jevtovic-Todorovic V, et al. Immunohistological demonstration of CaV3.2 T-type voltage-gated calcium channel expression in soma of dorsal root ganglion neurons and peripheral axons of rat and mouse. Neuroscience. (2013) 250:263–74. doi: 10.1016/j.neuroscience.2013.07.005
94. Słoniecka M, Le Roux S, Boman P, Byström B, Zhou Q, Danielson P. Expression profiles of neuropeptides, neurotransmitters, and their receptors in human keratocytes in vitro and in situ. PLoS One. (2015) 10(7):e0134157. doi: 10.1371/journal.pone.0134157
95. Talagas M, Lebonvallet N, Leschiera R, Sinquin G, Elies P, Haftek M, et al. Keratinocytes communicate with sensory neurons via synaptic-like contacts. Ann Neurol. (2020) 88(6):1205–19. doi: 10.1002/ana.25912
96. Abdo H, Calvo-Enrique L, Lopez JM, Song J, Zhang MD, Usoskin D, et al. Specialized cutaneous Schwann cells initiate pain sensation. Science. (2019) 365(6454):695–9. doi: 10.1126/science.aax6452
97. Zhao P, Barr TP, Hou Q, Dib-Hajj SD, Black JA, Albrecht PJ, et al. Voltage-gated sodium channel expression in rat and human epidermal keratinocytes: evidence for a role in pain. Pain. (2008) 139(1):90–105. doi: 10.1016/j.pain.2008.03.016
98. Lisse TS, Middleton LJ, Pellegrini AD, Martin PB, Spaulding EL, Lopes O, et al. Paclitaxel-induced epithelial damage and ectopic MMP-13 expression promotes neurotoxicity in zebrafish. Proc Natl Acad Sci U S A. (2016) 113(15):E2189–98. doi: 10.1073/pnas.1525096113
99. Cirrincione AM, Pellegrini AD, Dominy JR, Benjamin ME, Utkina-Sosunova I, Lotti F, et al. Paclitaxel-induced peripheral neuropathy is caused by epidermal ROS and mitochondrial damage through conserved MMP-13 activation. Sci Rep. (2020) 10(1):3970. doi: 10.1038/s41598-020-60990-8
100. Caterina MJ, Leffler A, Malmberg AB, Martin WJ, Trafton J, Petersen-Zeitz KR, et al. Impaired nociception and pain sensation in mice lacking the capsaicin receptor. Science. (2000) 288(5464):306–13. doi: 10.1126/science.288.5464.306
101. Lötsch J, Weyer-Menkhoff I, Tegeder I. Current evidence of cannabinoid-based analgesia obtained in preclinical and human experimental settings. Eur J Pain. (2018) 22(3):471–84. doi: 10.1002/ejp.1148
102. Masocha W. Targeting the endocannabinoid system for prevention or treatment of chemotherapy-induced neuropathic pain: studies in animal models. Pain Res Manag. (2018) 2018:5234943. doi: 10.1155/2018/5234943
103. Ibrahim MM, Porreca F, Lai J, Albrecht PJ, Rice FL, Khodorova A, et al. CB2 Cannabinoid receptor activation produces antinociception by stimulating peripheral release of endogenous opioids. Proc Natl Acad Sci U S A. (2005) 102(8):3093–8. doi: 10.1073/pnas.0409888102
104. Stucky CL, Mikesell AR. Cutaneous pain in disorders affecting peripheral nerves. Neurosci Lett. (2021) 765:136233. doi: 10.1016/j.neulet.2021.136233
105. Shepherd AJ, Copits BA, Mickle AD, Karlsson P, Kadunganattil S, Haroutounian S, et al. Angiotensin II triggers peripheral macrophage-to-sensory neuron redox crosstalk to elicit pain. J Neurosci. (2018) 38(32):7032–57. doi: 10.1523/JNEUROSCI.3542-17.2018
106. Siau C, Xiao W, Bennett GJ. Paclitaxel- and vincristine-evoked painful peripheral neuropathies: loss of epidermal innervation and activation of langerhans cells. Exp Neurol. (2006) 201(2):507–14. doi: 10.1016/j.expneurol.2006.05.007
107. By U A, Tani E, Andersson U, Henter JI. Tumor necrosis factor, interleukin 11, and leukemia inhibitory factor produced by langerhans cells in langerhans cell histiocytosis. J Pediatr Hematol Oncol. (2004) 26(11):706–11. doi: 10.1097/00043426-200411000-00004
108. Watkins LR, Maier SF. Beyond neurons: evidence that immune and glial cells contribute to pathological pain states. Physiol Rev. (2002) 82(4):981–1011. doi: 10.1152/physrev.00011.2002
109. Sakamoto A, Andoh T, Kuraishi Y. Involvement of mast cells and proteinase-activated receptor 2 in oxaliplatin-induced mechanical allodynia in mice. Pharmacol Res. (2016) 105:84–92. doi: 10.1016/j.phrs.2016.01.008
110. Chen Y, Yang C, Wang ZJ. Proteinase-activated receptor 2 sensitizes transient receptor potential vanilloid 1, transient receptor potential vanilloid 4, and transient receptor potential ankyrin 1 in paclitaxel-induced neuropathic pain. Neuroscience. (2011) 193:440–51. doi: 10.1016/j.neuroscience.2011.06.085
Keywords: cutaneous sensory endings, IENF density, chemotherapy-induced peripheral neuropathy, neuropathic pain, skin biopsy
Citation: Meregalli C, Monza L and Jongen JLM (2022) A mechanistic understanding of the relationship between skin innervation and chemotherapy-induced neuropathic pain. Front. Pain Res. 3:1066069. doi: 10.3389/fpain.2022.1066069
Received: 10 October 2022; Accepted: 24 November 2022;
Published: 13 December 2022.
Edited by:
Rayaz A. Malik, Weill Cornell Medicine, QatarReviewed by:
Ioannis Nikolaos Petropoulos, Weill Cornell Medicine, Qatar© 2022 Meregalli, Monza and Jongen. This is an open-access article distributed under the terms of the Creative Commons Attribution License (CC BY). The use, distribution or reproduction in other forums is permitted, provided the original author(s) and the copyright owner(s) are credited and that the original publication in this journal is cited, in accordance with accepted academic practice. No use, distribution or reproduction is permitted which does not comply with these terms.
*Correspondence: Cristina Meregalli Y3Jpc3RpbmEubWVyZWdhbGxpQHVuaW1pYi5pdA==
†These authors have contributed equally to this work
Specialty Section: This article was submitted to Neuropathic Pain, a section of the journal Frontiers in Pain Research
Disclaimer: All claims expressed in this article are solely those of the authors and do not necessarily represent those of their affiliated organizations, or those of the publisher, the editors and the reviewers. Any product that may be evaluated in this article or claim that may be made by its manufacturer is not guaranteed or endorsed by the publisher.
Research integrity at Frontiers
Learn more about the work of our research integrity team to safeguard the quality of each article we publish.