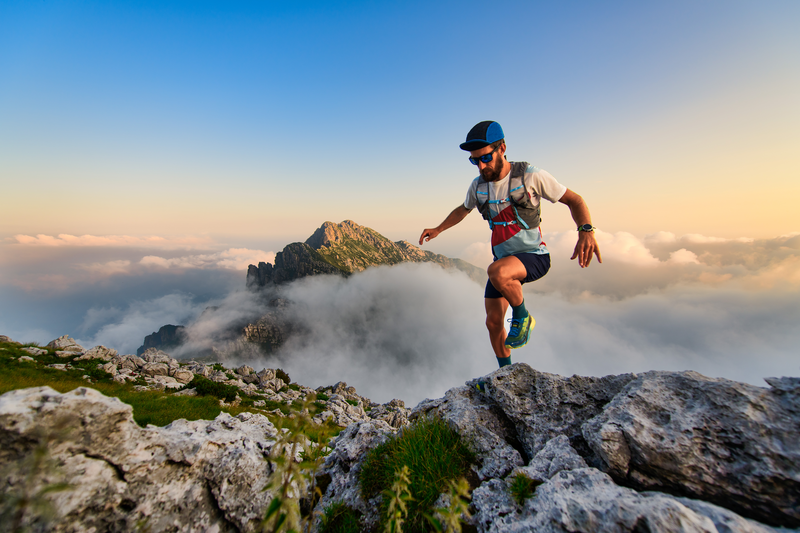
94% of researchers rate our articles as excellent or good
Learn more about the work of our research integrity team to safeguard the quality of each article we publish.
Find out more
ORIGINAL RESEARCH article
Front. Oral. Health , 14 November 2024
Sec. Oral Infections and Microbes
Volume 5 - 2024 | https://doi.org/10.3389/froh.2024.1461463
This article is part of the Research Topic Unravelling the Role of the Oral Microbiome in Cancer View all 4 articles
Objectives: To evaluate the possible changes of the oral microbiome during myelosuppressive chemotherapy (CT) and to investigate the potential relationship between the oral microbiome, the presence of oral mucositis (OM) and febrile neutropenia (FN).
Methods: A prospective, longitudinal, observational study was conducted in patients receiving myelosuppressive CT for a solid tumor or lymphoma. Oral rinsing samples were retrieved before, during and after the start of CT, but also when OM or FN was present. The samples were analyzed using 16S rRNA gene amplicon sequencing and statistical analysis was performed using alpha (Shannon) and beta (PERMANOVA) diversity analyses. Furthermore, differential abundances were analyzed using ALDEx2v1.32.0. Differences between groups were calculated using the Mann Whitney U-test, Kruskal-Wallis test and Wilcoxon Signed Rank using R.
Results: Forty-six patients, with a mean follow up of 114 days, were included for analysis and a total of 138 oral rinsing samples were available in the CLR-transformed data for PERMANOVA and 137 samples—for alpha diversity calculation. Significant changes in alpha diversity were seen when OM or FN was present. Moreover, significant changes were seen in beta diversity during the course of the CT treatment and when OM was present. Genera showing substantial changes in relative abundance were Streptococcus during the course of CT treatment and Prevotella, Fusobacterium, Selenomonas, Actinomyces and Leptotrichia when OM was present.
Conclusion: Changes in the oral microbiome were observed during the CT-regimen and when OM was present. Furthermore, changes of the oral microbiota during FN episodes were observed; however, larger studies should be performed to substantiate our results.
The role of the (oral) microbiome in health and disease is becoming evident (1, 2). Oral homeostasis is maintained due to complex interactions between the tissues of the host and resident microorganisms (3). In the oral cavity, about 700 bacterial taxa can be identified and each individual harbors about 100 different taxa known as commensal or resident oral bacteria (2–4). When the equilibrium, in which resident species in the oral cavity maintain a healthy state, becomes disturbed a dysbiotic shift occurs. This results in a disbalance and domination of a few species, that can grow to higher proportions than under healthy conditions, associated with an higher risk of disease (3). A disturbance of homeostasis can occur, for instance, due to salivary gland dysfunction, poor oral hygiene, a change in diet or smoking habits, oral or systemic diseases or medications (2, 5).
In cancer patients, a possible cause of a dysbiotic shift of the oral microbiome includes cytotoxic therapy such as chemotherapy (CT) and curative radiotherapy to the head and neck.
Myelosuppressive CT is used in the majority of cases for the treatment of solid tumors or lymphoma (6). This treatment modality has many systemic side effects, particularly affecting tissues with a high mitotic activity (6). One of the side effects of myelosuppressive CT is severe neutropenia, during which patients are unable to mount an effective inflammatory response. This poses these patients at risk of developing fever which may herald a potentially dangerous infection.
Febrile neutropenia (FN) is defined as fever during neutropenia during the course of CT which can lead to unplanned hospital admissions, and is associated with higher morbidity, delay or cancellation of the CT and even death (7). While an infection is the most probable cause of this severe side effect, causative pathogens are not identified in many cases (8). Common sites of infection are the skin, lungs and/or urine tract (9), but the oral cavity may also play a role (8). To date, little is known about the composition of the oral microbiome during episodes of FN.
Another common adverse effect of CT is oral mucositis (OM) (10). Oral mucositis is an inflammatory condition with damage to the oral mucosal lining due to direct and indirect effects of CT and/or radiotherapy (11). Clinically, CT-induced OM presents as redness and/or ulcerations of the non-keratinized oral mucosa. OM can lead to pain, reduced oral intake, a lower quality of life and delay or cancellation of CT (10, 12, 13).
The development of OM is complex and can be divided in five interrelated stages: initiation phase, primary damage response, signal amplification, ulceration and, finally, the healing phase (14). The initiation phase consists of direct physical DNA damage and triggering of biological events due to CT or radiotherapy. This results in activation of the innate immune response and apoptosis. Next, transcription factors including Nuclear Factor kappaB are activated leading to the generation of proinflammatory cytokines contributing to ulceration. These ulcerations may be colonized by mainly Gram-negative bacteria, which may in turn contribute to the production of more proinflammatory cytokines thereby aggravating inflammation. In most cases, the healing phase occurs spontaneously approximately after 2–4 weeks after the start of a CT cycle and generally simultaneously with neutrophil recovery.
Studies suggest potential associations between changes of the oral microbiota and development of OM and bloodstream infections as bacteria may translocate into the circulation via ulcerated tissue, particularly in myelosuppressed patients (6, 8, 12, 13, 15–19).
With respect to this, it has been reported that mucosal injury is associated with a reduction in abundance of commensal bacteria occurs and a potential enrichment of pathogenic microorganisms with virulence factors like lipopolysaccharide, fimbriae and proteolytic metabolites (20). Laheij et al. (21) found a lower alpha diversity, known as the microbial diversity within a single sample (22), and dysbiosis of the oral microbiota associated with OM in patients undergoing hematopoietic cell transplantation, and recovery of the microbiota after completion of the treatment (23).
In addition to an infectious cause of FN, it has been suggested that the inflammation associated with OM (and mucositis of the rest of the gastrointestinal tract) is also a significant cause of fever induced by inflammatory products entering the circulation (24, 25).
Another route by which oral microorganisms may contribute to FN, is via pre-existing oral inflammatory diseases, such as marginal or apical periodontitis. Microorganisms, bacterial cell wall substances and inflammatory products located in ulcerated periodontal pockets or peri-apically can cause local infectious exacerbations and may enter the circulation and spread to other body parts which may eventually lead to systemic inflammation and fever (26). In myelosuppressed cancer patients, pre-existing oral pathologies have been suggested to cause life-threatening infectious complications (27, 28).
Currently, only one study reports on oral microbiome changes in patients receiving myelosuppressive CT for solid tumors (29). This longitudinal, prospective study in breast cancer patients reports no statistical difference in alpha and beta diversity indices before, during and after CT. Beta diversity describes the measure of the (dis)similarity of two communities, thus a between-sample diversity (22). The prevalence of OM and FN and their possible association with oral microbial changes has not been part of this study.
As very little is known about the relationship between the oral microbiome, FN and OM, the aim of this study was to evaluate the oral microbial changes during myelosuppressive CT and possible associations with OM and FN in patients diagnosed with a solid tumor or lymphoma.
This prospective longitudinal observational study was performed at the Department of Oral and Maxillofacial Surgery and the Department of Oncology of the Amsterdam University Medical Center, location AMC. The Institutional Review Board approved this study (NL53440.018.15). All participants signed a written informed consent. This study is part of a larger prospective longitudinal observational study (9, 30). The clinical results of the potential role of dental foci and OM in relation to development of FN is reported by Zecha et al. (9) and the additional diagnostic value of the panoramic radiograph in pre-chemotherapy dental screening is reported by Zecha et al. (30).
Patients older than 18 years, with a (partial) natural dentition and/or dental implants, no prior head and neck radiotherapy, diagnosed with a solid tumor outside of the head and neck region or lymphoma and scheduled for CT-treatment with an intermediate risk of FN (31) were eligible for inclusion.
After a written consent, patients underwent an oral examination including orthopantomogram, in which pre-existing dental and oral pathology was recorded. No other x-rays were taken. Specification of the oral examination and oral focus definitions are described elsewhere (9, 30). The patient demographics—gender, age, intoxications, medication use, American Society of Anesthesiologists (ASA) classification, World Health Organization (WHO) performance status and cancer diagnosis—were retrieved from the patient records.
During the pre-treatment dental evaluation, a baseline rinsing sample was retrieved. During the courses of the CT-regimen, multiple rinsing samples were retrieved at the same time as when the oral cavity was scored for the presence of OM, according to the CTC-AEv3.0 guidelines (32). Collection of the oral rinsing samples took place just before CT-infusion and during regular check-ups with the oncologist. The examiners (one dentist, three dental students, one resident of oral maxillofacial surgery and one oral hygienist) were trained in reliable and consistent OM scoring and (also) received an instruction card. When the CT-regimen was completed and patients visited the oncologist for their regular check-ups, a final oral rinsing sample was retrieved.
If a patient presented with fever to the emergency care, OM was also assessed and an oral rinsing sample was taken by one of the examiners. In addition, patients were examined intraorally for oral fungal and recrudescent herpes simplex virus (re)infection and acute exacerbations of dental infections.
Chemotherapy regimens and the number of planned CT cycles were recorded. Changes in treatment plan were also registered. Dose delay was defined as a delay of planned chemotherapy for more than 3 days. Dose reduction was defined as an administered dose that was 85% or less of the initially planned dose (33). A chemotherapy cancellation was defined as an initially planned dose that was not given at all.
Despite the strict inclusion criteria for FN risk (31), the actual risk of neutropenia varied between CT regimens. For analysis we therefore divided the group, before analysis, in relatively low- and relatively high risk of myelotoxicity based on the administered CT agent given [see Appendix 1 in Zecha et al. (9).]. This classification was performed by an experienced oncologist.
Prior to the start of CT, an oral examination took place consisting of the following:
• Evaluation of dental mindedness (dental visits, oral hygiene habits) and oral complaints over the last 3 months
• Intra-oral screening for dental and/or mucosal pathology
• Periodontal screening using the Dutch Periodontal Screening Index (34)
• Screening for peri-implant mucositis and peri-implantitis
• Panoramic radiograph for all patients followed by selective peri-apical radiographs if indicated
Pre-existing dental and oral pathologies that may contribute to the development of FN and infectious complications, were noted as an oral focus in accordance with the guidelines of the Dutch Association of Maxillofacial Surgery (35). These included:
• Periodontal disease (DPSI 4; periodontal probing depth of ≥6 mm; peri-implantitis was also considered as a focus)
• Profound dental caries; caries reaching far into the dentin
• Periapical pathology
• (Partially) impacted teeth
• Retained roots with surrounding pathology
Treatment of foci was only considered at the discretion of the dentist and/or when patient reported pain or any other symptoms.
OM was scored according to the CTC-AEv3.0 (32), during the CT regimen and after CT was completed. OM was graded as 1 (erythema), 2 (patchy ulcerations or pseudomembranes), 3 (confluent ulcerations or pseudomembranes, bleeding with minor trauma), 4 (tissue necrosis, significant bleeding, life threatening consequences) or 5 (death). All examiners were trained in reliable and consistent OM scoring and received an instruction card. Oral rinsing samples were taken during the period that OM was present. When a patient presented with fever, OM was also assessed and an oral rinsing sample was retrieved.
Febrile neutropenia was defined as temperature ≥38.5°C or two consecutive readings of >38.0°C for 2 h and an absolute neutrophil count <500/μl or expected to fall below this threshold (36, 37). When FN was diagnosed, laboratory and/or radiological results (including full hematological blood count, infection panel, urine sediment and chest x-ray), working diagnosis and treatment plan, including the antibiotic regimen, were noted. The set of blood cultures, collected during the fever episode and placed in an incubator for aerobic and anaerobic growth, were examined for the presence of microbial growth after 2 days. Sepsis/septic shock and/or death was also noted.
An oral rinsing sample was taken by using a sterile tube of 10 ml with 0.9% sterile saline solution. The patient was asked to rinse the oral cavity for 30 s and to spit the rinse in a sterile container. These containers were pelleted by centrifugation immediately after collection (7 min at 4,500 × g), and resuspended in sterile 1 ml PBS and stored at −80°C until analysis.
The tubes with oral rinsing samples were thawed and centrifuged. The pellets were resuspended in 100 µl TE buffer (Tris-EDTA) and transferred to a 96-well deepwell plate. After addition of 100 µl Lysis buffer (LGC genomics GmbH, Berlin, Germany), 250 µl 0.1 mm Zirconia beads (Biospec, Bartlesville, OK, USA), and 200 µl RotiPhenol (Carl Roth, Karlsruhe, Germany), the mixture was subjected to four bead-beating steps of 2 min each. DNA extraction and purification was performed with the LGC Magmini kit (LGC genomics GmbH), after which the bacterial DNA concentration was determined by a 16S ribosomal RNA gene quantitative polymerase chain reaction (qPCR) (38). For each sample, 1 ng of DNA was amplified, with barcoded forward and reverse primers (39), using the 16S rRNA gene-specific sequences V4F/515F: GTGCCAGCMGCCGCGGTAA and V4R/806R: GGACTACHVGGGTWTCTAAT (40). Paired-end sequencing (2 × 251 nt) was conducted on the Illumina MiSeq platform with the MiSeq Reagent Kit v3 at the Core Facility Genomics, Amsterdam UMC. The flow cell was loaded with 8 pmol, including 30% PhiX.
The reads were quality-filtered, denoised, mapped to zero-radius operational taxonomic units (zOTUs), and assigned taxonomy using the HOMD database (v14.51) (41), as described earlier (42). Control samples consisted of PBS solution (used for storage of the samples), sterile 0.9% NaCl solution (used to rinse with), blank DNA isolations, and PCR controls (controls are used to detect possible contamination of all carriers used in the sequencing process).
For the calculation of the alpha diversity, the final zOTU table was randomly subsampled at 9,500 reads/sample. One sample was lost due to too few reads after subsampling at 9,500 reads/sample. For PERMANOVA, the zOTU-table was centered-log ratio (CLR) transformed, using a pseudocount of 0.5 and a minimal sample depth of 2,600 reads.
Permutational multivariate analysis of variance (PERMANOVA; Aitchison distance as Euclidean distance on the CLR-transformed data, 99,999 permutations), and PERMANOVA with permutations restricted to the subject were performed using R v4.3.1 (43) and the R packages vegan v2.6–4 (44), microbiome v1.22.0 (45) and phyloseq v1.44.0 (46). The (unbiased) Shannon diversity index was calculated using PAST (47). Differential abundance analyses were carried out with the non-subsampled data, as for the CLR transformation above, using ALDEx2v1.32.0 (48, 49).
Differences between groups were calculated using the Mann Whitney U-test and Kruskal-Wallis test for unrelated samples (followed by Dunn's post-hoc test) and Wilcoxon Signed Rank test for related samples using R. The relations between independent variables and the longitudinally measured Shannon diversity index of the oral microbiota were analyzed using Linear Mixed Model Analysis for continuous outcome values, to correct for the dependency in outcome measurement. Independent variables were entered as fixed effects. SPSS version 26 (IBM) was used for these analyses. A p-value < 0.05 was considered statistically significant.
In this study, 93 patients were eligible for inclusion. However, for microbial analysis twenty-one patients could not be included due to several reasons: incomplete sample collection (n = 16), continuing with the study was too burdensome (n = 4) and early discontinuation of the treatment (n = 1). In 26 patients, the samples were not stored according to the protocol and therefore were excluded from analysis (Figure 1). Finally, samples of 46 patients, all with a baseline sample, were available for oral microbiota analysis with a total of 138 oral rinsing samples available in the CLR-transformed data for PERMANOVA and 137 samples—for alpha diversity calculation.
In Table 1 patient demographics and tumor/treatment characteristics are described. The majority of the included patients were female and most patients were diagnosed with a gynecological tumor, followed by a tumor in the upper GI tract. Details about the administered CT regimens is reported in Appendix 1. Alterations of the treatment plan were performed in 19 patients and dose reductions were necessary in 9 patients. The mean follow-up period was 114 days (range 28–200).
During the pre-treatment oral evaluation, a dental focus was found in 22 patients (47.8%) of which 18 patients had a periodontal focus. None of these asymptomatic dental foci were treated prior to the start of the CT regimen. In 15 patients, peak OM was grade 2 (ulcerations) and in 14 patients peak OM grade was 1 (erythema), at any time during any CT cycle. No OM was present at the evaluation when the CT regimen was completed, see Table 2. The results of the pre-treatment oral evaluation of the full cohort is published elsewhere (9).
Six patients developed fever (≥38.5°C) while neutropenic (M:F; 2:4, age 18–78). All these patients received a CT regimen with a relatively higher risk of developing neutropenia. The mean neutrophil count was 0.09 × 109/L (0.00–0.33). For one patient, the CT course was cancelled and for two it was delayed. Three patients who developed fever (50%), received CT for an osteosarcoma. Of the latter patients, a dental focus was found during the dental examination in two patients (33%), four patients reported salivary changes (67%) and all patients presenting with FN had developed oral mucositis during the CT regimen, of which five patients grade 2.
The top-10 most abundant genera at baseline were Streptococcus, Prevotella, Veillonella, Neisseria, Rothia, Actinomyces, Haemophilus, Leptotrichia, Fusobacterium and Gemella (Figure 2).
At baseline, the average (+ SD) Shannon index was 3.65 ± 0.46 (range 2.28–4.67). There was no difference in the average Shannon index by gender, CT regimen, patients with periodontal pockets ≤5 mm vs. pockets ≥6 mm, patients with or without a dental focus and different age groups (Mann Whitney U-test; Kruskal-Wallis test: p > 0.05). However, a significantly lower alpha-diversity was present in patients who never had smoked compared to those actively smoking or who quitted smoking (Kruskal-Wallis test, p = 0.04; quit vs. never p = 0.039, never vs. yes p = 0.050, quit vs. yes p = 0.704). No significant difference on the alpha-diversity of the oral microbiota was seen by the presence of an oral focus (linear mixed model, p = 0.144) or periodontal pockets ≥6 mm (linear mixed models, p = 0.720).
At baseline no significant difference was seen in the composition of the oral microbiota between gender, CT intensity and age groups (PERMANOVA, p > 0.05). However, there was a significant difference in microbial composition between three categories of smokers (PERMANOVA, F = 1.69, p = 0.0037), patients with vs. patients without an oral focus (PERMANOVA, F = 2.18, p = 0.0015), and patients with periodontal pockets ≤5 mm vs. ≥6 mm, (PERMANOVA, F = 2.02, p = 0.0039).
For 30 patients oral rinsing samples were available that were collected before (baseline samples), during (CT-samples) and after CT (post-CT samples). During CT, oral rinsing samples were collected between days 8 to 73. The post-CT samples were collected between 40 and more than 200 days after the end of the CT regimen. Of the 30 patients included in this analysis, six patients developed OM grade 2 at some point during the course of CT treatment. At baseline, the top-10 most abundant genera (Figure 3) only slightly differed from the entire group at baseline (Figure 2). The prevalence in descending order was Streptococcus, Prevotella, Veillonella, Rothia, Haemophilus, Actinomyces, Neisseria, Leptotrichia, Gemella and Fusobacterium.
Figure 3. Top-10 most abundant genera: changes in relative abundance over the course of the treatment (n = 30).
The average Shannon index was 3.67 ± 0.48 at baseline, 3.37 ± 0.60—during the treatment course and 3.59 ± 0.49—after the treatment was completed. Although the Shannon index was, on average, lower during CT, and recovered after CT, these changes were not significant (Wilcoxon Signed Rank test, p = 0.531).
The oral microbial composition differed significantly between baseline, during CT and after completion of the CT regimen (PERMANOVA using permutations restricted on Subject [N = 30, 90 samples, F = 0.95, p = 1e-4; post-hoc (no multiple-testing correction) for Phase 1vs 2, 1 vs. 3 and 2 vs. 3, respectively: F = 1.25, p = 0.0002; F = 0.90, p = 0.003; F = 0.71, p = 0.05].
To exclude the potential effect of OM in the changes of the oral microbiota, we analyzed the compositional changes and relative abundances without the 6 subjects who developed OM grade 2 over the course of the treatment. Again, a significant difference was seen (N = 24; F = 0.76; p = 0.0015; post-hoc for Phase 1 vs. 2, 1 vs. 3 and 2 vs. 3, respectively: F = 1.03, p = 0.0046; F = 0.77, p = 0.013; F = 0.49, p = 0.42).
During the CT regimen, several zOTUs showed a significant difference in relative abundance by using ALDEx2, of which the four most abundant ones are shown in Figure 4. Only zOTU22 (Streptococcus) remained significant (Phase 1 vs. 2) after correction for multiple testing. However, the effect sizes of the shown zOTUs were large (>0.7 or <−0.7) for at least one pair-wise comparison.
Figure 4. Four zOTUs showing significant changes in relative abundance before (phase 1), during (phase 2) or after CT (phase 3) over the course of the treatment, figures (a–d) are sorted by decreasing zOTU abundance. The p-value (Wilcoxon) and effect size (e) from ALDEx2 are indicated for each pair-wise comparison.
Of the 46 patients, 15 developed OM grade 2. The Shannon index of these 15 patients before the start of CT was 3.49 ± 0.49 and at the time point when they had developed ulcerative OM 3.19 ± 0.51 (Wilcoxon Signed Rank test, p = 0.083). When analyzing alpha-diversity data longitudinally, we found a significant linear effect of oral mucositis on the Shannon index (linear mixed model, 0.259124, p = 0.0001). Oral mucositis was associated with a lower Shannon index. Figure 5 shows the Shannon diversity of the patients who did not develop OM during the course of the treatment and patients who did develop OM grade 2.
Figure 5. Shannon diversity of patients who did and did not develop oral mucositis before (phase 1), during (phase 2) and after CT (phase 3); OM: n = 15 (grade II), non OM: n = 30.
There was a significant difference in the oral microbial composition in the 15 OM patients before the start of CT and at the moment they were diagnosed with OM [PERMANOVA 32767 restricted permutations (complete enumeration), F = 1.00, p = 0.0006].
A decrease in relative abundance of eight zOTUs assigned to the genera of Prevotella, Fusobacterium, Selenomonas, Actinomyces and Leptotrichia was seen by using ALDEx2, during OM with an effect size less than −0.5. The three largest zOTUs, with effect sizes less than −0.7 are shown in Figure 6.
Figure 6. Three zOTUs showing significant changes in relative abundance between baseline and the moment of OM grade 2. Figures (a–c) are sorted on decreasing zOTU abundance. The p-value (Wilcoxon) and effect size (e) from ALDEx2 are indicated.
Six out of the 46 patients developed neutropenic fever (13.0%). Two had an oral focus prior to the start of CT regimen. All but one developed OM grade 2 over the courses of the CT treatment. The Shannon diversity index was significantly lower at the moment of a fever (Wilcoxon Signed Rank test, p = 0.031; 3.55 ± 0.35 before the start of CT and 3.02 ± 0.64 at time of fever).
The aim of this study was to evaluate the oral microbial changes during myelosuppressive CT and possible associations with OM and FN in patients diagnosed with a solid tumor or lymphoma. We found that a lower alpha diversity of the oral microbiota and differences in beta diversity were significantly associated with OM. Additionally, a decrease was seen in the relative abundance of zOTUs assigned to the genera Prevotella, Fusobacterium, Selenomonas, Actinomyces and Leptotrichia, of which Prevotella and Actinomyces showed a greater effect size.
In general, the oral microbiota of our patient group at baseline was comparable with the general population as the abundance of the top 10 most abundant genera was similar with previously published findings (29, 50–52). A decrease in alpha diversity in relation to OM was also described by others (12, 21, 23, 53, 54). Hong et al. (12) concluded that shifts in the oral microbiota are strongly correlated with OM severity and Bruno et al. (53) found a significant difference in composition during OM.
At baseline, a significantly higher alpha diversity was seen in patients who smoked and a significant difference was seen in beta-diversity for smokers or patients with an oral focus including periodontitis. A higher alpha diversity is also reported in the review by Maki et al. (55) and the significant difference in beta diversity is also in line with the literature, as it is described that these factors can lead to dysbiosis of the oral microbiome (2, 20, 56).
During CT-regimen a decrease in alpha diversity was seen, but after completion of the treatment course a tendency to recover was also visible. However, these results were not significant. The decrease in alpha diversity is also reported by others (12, 21, 57–60). In contrast, Mougeot et al. (50) concluded in a review that CT treatment increases oral microbiome diversity and radiotherapy had the opposite effect. However, this review included different types of analysis for microbial identification, which potentially could lead to biased results. The tendency to recover after completion of the treatment is in line with Laheij et al. (23) who found a significant recovery of the oral microbiota after 3 months and stabilization of the oral microbiome after 1 year. Possibly, with a longer follow-up time, a significant recovery of the oral microbiota could also be demonstrated in our study.
Secondly, the beta diversity significantly differed over the course of the treatment. This effect remained after excluding patients who developed OM during the course of the treatment. However, no significant recovery in beta-diversity was seen after the course of the treatment was completed. Laheij et al. (23) did find a recovery of beta diversity to baseline levels after HCT, but they had a longer follow up time after the completion of cancer therapy. In contrast with our findings, Klymiuk et al. (29) found no changes over the course of the treatment in alpha and beta diversity, but clinical outcomes, like the development of OM, were not mentioned in the results. This could be a potential bias, as we and others, found a significant effect of OM in diversity changes (12, 57).
Significant changes were seen in relative abundance of zOTUs assigned to the genera of Streptococcus, Veillonella and Prevotella. Streptococcus and Veillonella are associated with oral health by preventing pathogenic bacteria to attach on the oral surfaces (61). Prevotella is an anaerobic commensal oral resident, but has been associated also with (extra)-oral disease (62). There is some evidence that suggesting that Prevotella may also have beneficial effects on human health, like for instance improved glucose control (63). Decrease of its relative abundance, as seen in the patients experiencing OM, may potentially reduce these benefits. Actinomyces is one of the genera that is predominantly present in the oral cavity and plays a crucial role in biofilm formation on teeth (64). However, the overall function of Actinomyces within the oral microbiome is not yet fully known. In a recent systematic review, Frey et al. (54) reported a decrease in relative abundance of Prevotella and Actinomyces during OM. So, our results conform to their findings.
Our study found a significantly lower alpha diversity in patients during a FN episode. To our knowledge, we are the first to study the oral microbiota in patients during a FN episode. The samples retrieved were all taken before or immediately after the start of antibiotic treatment for FN. However, these results are preliminary as only 6 patients could be included in this analysis. Furthermore, the potential role of the antibiotic treatment is not established. There is a lack of evidence on the role of the oral microbiome and the development of FN, as no research is published directly investigating microorganisms in blood and in the oral microbiome (20, 50). McMahon et al. (65) investigated the potential role of oral and gastrointestinal microbiome in bloodstream infections, however, they only analyzed the patients who had a positive blood culture. It is known that blood cultures of patients developing FN, are positive in only 0.2%–15% of cultures (66), so potential correlation could be missed. Moreover, several microorganisms present in the oral cavity cannot be cultured, or need different culture circumstances than are customary for regular blood cultures, such as longer incubation time or specific nutrients. For that reason, we obtained residual material from the blood collection during FN to analyze and identify potential oral microorganisms. However, the quality of the material was not sufficient, so no in-depth identification of microorganisms in blood could be performed. Furthermore, the blood cultures drawn during the FN episodes were all negative and no further analysis on cultured microorganisms could be performed. Sardzikova et al. (67) reported a significant relation of decreased alpha diversity in the gut microbiome in relation with FN. However, the studied patient group included paediatric patients undergoing hematopoietic stem cell transplantation, but these findings might give an indication of a potential relation of microbiome changes and the development of FN.
This study houses several limitations. The main limitation is the relatively small study population, due to the fact that the samples of 26 patient were not stored according to protocol, meaning that less robust conclusions can be drawn from our results. Furthermore, the patient group was heterogenic because of different tumor locations and different treatment regimens. However, this patient group encounters the majority of the cancer patients treated with myelosuppressive CT and no earlier study has been performed in this patient group. Only two studies (12, 29) investigated the oral microbiome changes in patients with solid tumors, of which one (29) only included breast cancer patients and the other study selected on the type of CT, not mentioning tumor diagnosis.
Another limitation of this study is the absence of a (healthy) control group. Therefore, we could not establish if the tumor itself had an influence on the oral microbiota. But the baseline composition of the oral microbiome showed no large deviation to what is known in healthy individuals. Even with these limitations, this study brought results about the oral microbiome of a specific patient group, where data is scarce. Further larger longitudinal studies are needed to draw more robust conclusions about the possible relation between febrile neutropenia, where the duration and depth of the neutropenic episodes also needs to be further analyzed; oral mucositis and the oral microbiota.
In conclusion, in patients treated with myelosuppressive chemotherapy for solid tumors, a lower alpha diversity and changed beta diversity of the oral microbiota was significantly associated with oral mucositis. Furthermore, a change of the oral microbiota occurs during episodes of febrile neutropenia. However, these results should be interpreted with caution because of low number of participants. Further study is needed to draw more robust conclusions and to identify bacteria (that are often present in the oral cavity) in blood during febrile neutropenia.
The raw data supporting the conclusions of this article will be made available by the authors, without undue reservation.
The studies involving humans were approved by Medical Ethical Board, Amsterdam University Medical Center. The studies were conducted in accordance with the local legislation and institutional requirements. The participants provided their written informed consent to participate in this study.
JZ: Conceptualization, Data curation, Formal Analysis, Investigation, Methodology, Project administration, Visualization, Writing – original draft, Writing – review & editing. JR-D: Funding acquisition, Resources, Supervision, Writing – review & editing. BB: Formal Analysis, Methodology, Software, Supervision, Writing – review & editing. MB: Methodology, Software, Writing – review & editing. EZ: Formal Analysis, Methodology, Supervision, Writing – review & editing. JdL: Resources, Supervision, Writing – review & editing. LS: Resources, Supervision, Writing – review & editing. AL: Formal Analysis, Investigation, Methodology, Software, Supervision, Writing – review & editing.
The author(s) declare financial support was received for the research, authorship, and/or publication of this article. The author(s) declare support from the Eklund Foundation (project number: 2016–005).
The authors declare that the research was conducted in the absence of any commercial or financial relationships that could be construed as a potential conflict of interest.
All claims expressed in this article are solely those of the authors and do not necessarily represent those of their affiliated organizations, or those of the publisher, the editors and the reviewers. Any product that may be evaluated in this article, or claim that may be made by its manufacturer, is not guaranteed or endorsed by the publisher.
1. Baker JL, Mark Welch JL, Kauffman KM, McLean JS, He X. The oral microbiome: diversity, biogeography and human health. Nat Rev Microbiol. (2024) 22:89–104. doi: 10.1038/s41579-023-00963-6
2. Kilian M, Chapple IL, Hannig M, Marsh PD, Meuric V, Pedersen AM, et al. The oral microbiome—an update for oral healthcare professionals. Br Dent J. (2016) 221(10):657–66. doi: 10.1038/sj.bdj.2016.865
3. Radaic A, Kapila YL. The oralome and its dysbiosis: new insights into oral microbiome-host interactions. Comput Struct Biotechnol J. (2021) 19:1335–60. doi: 10.1016/j.csbj.2021.02.010
4. eHOMD. Available online at: https://ehomd.org/ (accessed June 12, 2024).
5. Santacroce L, Passarelli PC, Azzolino D, Bottalico L, Charitos IA, Cazzolla AP, et al. Oral microbiota in human health and disease: a perspective. Exp Biol Med. (2023) 248(15):1288–301. doi: 10.1177/15353702231187645
6. Villafuerte KRV, Martinez CJH, Dantas FT, Carrara HHA, Dos Reis FJC, Palioto DB. The impact of chemotherapeutic treatment on the oral microbiota of patients with cancer: a systematic review. Oral Surg Oral Med Oral Pathol Oral Radiol. (2018) 125(6):552–66. doi: 10.1016/j.oooo.2018.02.008
7. Kuderer NM, Dale DC, Crawford J, Cosler LE, Lyman GH. Mortality, morbidity, and cost associated with febrile neutropenia in adult cancer patients. Cancer. (2006) 106(10):2258–66. doi: 10.1002/cncr.21847
8. Zecha J, Raber-Durlacher JE, Laheij A, Westermann AM, Epstein JB, de Lange J, et al. The impact of the oral cavity in febrile neutropenia and infectious complications in patients treated with myelosuppressive chemotherapy. Support Care Cancer. (2019) 27(10):3667–79. doi: 10.1007/s00520-019-04925-8
9. Zecha JAEM, Raber-Durlacher JE, Laheij AMGA, Westermann AM, de Lange J, Smeele LE. The potential contribution of dental foci and oral mucositis to febrile neutropenia in patients treated with myelosuppressive chemotherapy for solid tumors and lymphoma. Front Oral Health. (2022) 3:940044. doi: 10.3389/froh.2022.940044
10. Köstler WJ, Hejna M, Wenzel C, Zielinski CC. Oral mucositis complicating chemotherapy and/or radiotherapy: options for prevention and treatment. CA Cancer J Clin. (2001) 51(5):290–315. doi: 10.3322/canjclin.51.5.290
11. Sonis ST. The pathobiology of mucositis. Nat Rev Cancer. (2004) 4(4):277–84. doi: 10.1038/nrc1318
12. Hong BY, Sobue T, Choquette L, Dupuy AK, Thompson A, Burleson JA, et al. Chemotherapy-induced oral mucositis is associated with detrimental bacterial dysbiosis. Microbiome. (2019) 7(1):66. doi: 10.1186/s40168-019-0679-5
13. Napeñas JJ, Brennan MT, Bahrani-Mougeot FK, Fox PC, Lockhart PB. Relationship between mucositis and changes in oral microflora during cancer chemotherapy. Oral Surg Oral Med Oral Pathol Oral Radiol Endod. (2007) 103(1):48–59. doi: 10.1016/j.tripleo.2005.12.016
14. Lalla RV, Brennan MT, Gordon SM, Sonis ST, Rosenthal DI, Keefe DM. Oral mucositis due to high-dose chemotherapy and/or head and neck radiation therapy. J Natl Cancer Inst Monogr. (2019) 2019(53):lgz011. doi: 10.1093/jncimonographs/lgz011
15. Stringer AM, Logan RM. The role of oral flora in the development of chemotherapy-induced oral mucositis. J Oral Pathol Med. (2015) 44(2):81–7. doi: 10.1111/jop.12152
16. de Mendonca RM, de Araujo M, Levy CE, Morari J, Silva RA, Yunes JA, et al. Prospective evaluation of HSV, Candida spp., and oral bacteria on the severity of oral mucositis in pediatric acute lymphoblastic leukemia. Support Care Cancer. (2012) 20(5):1101–7. doi: 10.1007/s00520-011-1190-0
17. Napenas JJ, Brennan MT, Coleman S, Kent ML, Noll J, Frenette G, et al. Molecular methodology to assess the impact of cancer chemotherapy on the oral bacterial flora: a pilot study. Oral Surg Oral Med Oral Pathol Oral Radiol Endod. (2010) 109(4):554–60. doi: 10.1016/j.tripleo.2009.11.015
18. Lucas VS, Beighton D, Roberts GJ, Challacombe SJ. Changes in the oral streptococcal flora of children undergoing allogeneic bone marrow transplantation. J Infect. (1997) 35(2):135–41. doi: 10.1016/S0163-4453(97)91545-0
19. van Winkelhoff AJ, Slots J. Actinobacillus actinomycetemcomitans and Porphyromonas gingivalis in nonoral infections. Periodontol 2000. (1999) 20:122–35. doi: 10.1111/j.1600-0757.1999.tb00160.x
20. Bruno JS, Al-Qadami GH, Laheij A, Bossi P, Fregnani ER, Wardill HR. From pathogenesis to intervention: the importance of the microbiome in oral mucositis. Int J Mol Sci. (2023) 24(9):8274. doi: 10.3390/ijms24098274
21. Laheij A, Raber-Durlacher JE, Koppelmans RGA, Huysmans M, Potting C, van Leeuwen SJM, et al. Microbial changes in relation to oral mucositis in autologous hematopoietic stem cell transplantation recipients. Sci Rep. (2019) 9(1):16929. doi: 10.1038/s41598-019-53073-w
22. Claesson MJ, Clooney AG, O'Toole PW. A clinician’s guide to microbiome analysis. Nat Rev Gastroenterol Hepatol. (2017) 14(10):585–95. doi: 10.1038/nrgastro.2017.97
23. Laheij A, Rozema FR, Brennan MT, von Bültzingslöwen I, van Leeuwen SJM, Potting C, et al. Long-term analysis of resilience of the oral microbiome in allogeneic stem cell transplant recipients. Microorganisms. (2022) 10(4):734. doi: 10.3390/microorganisms10040734
24. van der Velden WJ, Herbers AH, Netea MG, Blijlevens NM. Mucosal barrier injury, fever and infection in neutropenic patients with cancer: introducing the paradigm febrile mucositis. Br J Haematol. (2014) 167(4):441–52. doi: 10.1111/bjh.13113
25. Family L, Li Y, Chen LH, Page JH, Klippel ZK, Chao C. A study of novel febrile neutropenia risk factors related to bone marrow or immune suppression, barrier function, and bacterial flora. J Natl Compr Canc Netw. (2018) 16(10):1201–8. doi: 10.6004/jnccn.2018.7051
26. Sogawa Y, Fukui M, Nakamura S, Sogabe K, Sumitani R, Yoshioka M, et al. Involvement of oral bacteria and oral immunity as risk factors for chemotherapy-induced fever with neutropenia in patients with hematological cancer. Int J Hematol. (2020) 112(6):851–9. doi: 10.1007/s12185-020-02975-x
27. Kishimoto M, Akashi M, Tsuji K, Kusumoto J, Furudoi S, Shibuya Y, et al. Intensity and duration of neutropenia relates to the development of oral mucositis but not odontogenic infection during chemotherapy for hematological malignancy. PLoS One. (2017) 12(7):e0182021. doi: 10.1371/journal.pone.0182021
28. Spijkervet FKL, Schuurhuis JM, Stokman MA, Witjes MJH, Vissink A. Should oral foci of infection be removed before the onset of radiotherapy or chemotherapy? Oral Dis. (2021) 27(1):7–13. doi: 10.1111/odi.13329
29. Klymiuk I, Bilgilier C, Mahnert A, Prokesch A, Heininger C, Brandl I, et al. Chemotherapy-associated oral microbiome changes in breast cancer patients. Front Oncol. (2022) 12:949071. doi: 10.3389/fonc.2022.949071
30. Zecha J, Laheij A, Raber-Durlacher JE, Westermann AM, de Lange J, Smeele LE. Pre-chemotherapy dental screening: is there additional diagnostic value for a panoramic radiograph? Dent J. (2023) 11(5):122. doi: 10.3390/dj11050122
31. Lyman GH, Abella E, Pettengell R. Risk factors for febrile neutropenia among patients with cancer receiving chemotherapy: a systematic review. Crit Rev Oncol Hematol. (2014) 90(3):190–9. doi: 10.1016/j.critrevonc.2013.12.006
32. National Institutes of Health NCI. Common Terminology Criteria for Adverse Events v3.0 (CTCAE). (2006).
33. Chan A, Lee CP, Chiang J, Ng R. Breakthrough febrile neutropenia and associated complications among elderly cancer patients receiving myelosuppressive chemotherapy for solid tumors and lymphomas. Support Care Cancer. (2013) 21(8):2137–43. doi: 10.1007/s00520-013-1768-9
34. Van der Velden U. The Dutch periodontal screening index validation and its application in The Netherlands. J Clin Periodontol. (2009) 36(12):1018–24. doi: 10.1111/j.1600-051X.2009.01495.x
35. Nederlandse Vereniging voor Mondziekten en Kaak- en Aangezichtschirurgie (NVMKA). Nederlandse Vereniging voor Mondziekten K-eA. Focus onderzoek. (2016).
36. Klastersky J, de Naurois J, Rolston K, Rapoport B, Maschmeyer G, Aapro M, et al. Management of febrile neutropaenia: eSMO clinical practice guidelines. Ann Oncol. (2016) 27(suppl 5):v111–v8. doi: 10.1093/annonc/mdw325
37. Punnapuzha S, Edemobi PK, Elmoheen A. Febrile Neutropenia. StatPearls. Treasure Island (FL): StatPearls Publishing LLC. (2021).
38. Ciric L, Pratten J, Wilson M, Spratt D. Development of a novel multi-triplex qPCR method for the assessment of bacterial community structure in oral populations. Environ Microbiol Rep. (2010) 2(6):770–4. doi: 10.1111/j.1758-2229.2010.00183.x
39. Kozich JJ, Westcott SL, Baxter NT, Highlander SK, Schloss PD. Development of a dual-index sequencing strategy and curation pipeline for analyzing amplicon sequence data on the MiSeq Illumina sequencing platform. Appl Environ Microbiol. (2013) 79(17):5112–20. doi: 10.1128/AEM.01043-13
40. Caporaso JG, Lauber CL, Walters WA, Berg-Lyons D, Lozupone CA, Turnbaugh PJ, et al. Global patterns of 16S rRNA diversity at a depth of millions of sequences per sample. Proc Natl Acad Sci. (2011) 108(Suppl 1):4516–22. doi: 10.1073/pnas.1000080107
41. Chen T, Yu WH, Izard J, Baranova OV, Lakshmanan A, Dewhirst FE. The human oral microbiome database: a web accessible resource for investigating oral microbe taxonomic and genomic information. Database (Oxford). (2010) 2010:baq013. doi: 10.1093/database/baq013
42. Kahharova D, Brandt BW, Buijs MJ, Peters M, Jackson R, Eckert G, et al. Maturation of the oral microbiome in caries-free toddlers: a longitudinal study. J Dent Res. (2020) 99(2):159–67. doi: 10.1177/0022034519889015
43. R Core Team. A Language and Environment for Statistical Computing Vienna, Austria. (2023). Available online at: https://www.R-project.org/ (accessed June 12, 2024).
44. Oksanen JSG, Blanchet F, Kindt R, Legendre P, Minchin P, O'Hara R, et al. Vegan: Community Ecology Package. R Package Version 2.6-4. (2022).
45. Lahti L, Shetty S. Tools for Microbiome Analysis in R. (2017). Available online at: https://microbiome.github.io/tutorials/ (accessed June 12, 2024).
46. McMurdie PJ, Holmes S. Phyloseq: an R package for reproducible interactive analysis and graphics of microbiome census data. PLoS One. (2013) 8(4):e61217. doi: 10.1371/journal.pone.0061217
47. Hammer Ø, Harper DAT, Ryan PD. PAST: paleontological statistics software package for education and data analysis. Palaeontologia Electronica. (2001) 4:9.
48. Fernandes LL, Torres SR, Garnica M, de Souza Goncalves L, Junior AS, de Vasconcellos AC, et al. Oral status of patients submitted to autologous hematopoietic stem cell transplantation. Support Care Cancer. (2014) 22(1):15–21. doi: 10.1007/s00520-013-1940-2
49. Gloor GB, Macklaim JM, Fernandes AD. Displaying variation in large datasets: plotting a visual summary of effect sizes. J Comput Graph Stat. (2016) 25:971–9. doi: 10.1080/10618600.2015.1131161
50. Mougeot JC, Stevens CB, Morton DS, Brennan MT, Mougeot FB. Research frontiers in oral toxicities of cancer therapies: osteoradionecrosis of the jaws. J Natl Cancer Inst Monogr. (2019) 2019(53):lgz002. doi: 10.1093/jncimonographs/lgz006
51. Zaura E, Nicu EA, Krom BP, Keijser BJ. Acquiring and maintaining a normal oral microbiome: current perspective. Front Cell Infect Microbiol. (2014) 4:85. doi: 10.3389/fcimb.2014.00085
52. Segata N, Haake SK, Mannon P, Lemon KP, Waldron L, Gevers D, et al. Composition of the adult digestive tract bacterial microbiome based on seven mouth surfaces, tonsils, throat and stool samples. Genome Biol. (2012) 13(6):R42. doi: 10.1186/gb-2012-13-6-r42
53. Bruno JS, Heidrich V, Knebel FH, de Molla VC, Parahyba CJ, Miranda-Silva W, et al. Commensal oral microbiota impacts ulcerative oral mucositis clinical course in allogeneic stem cell transplant recipients. Sci Rep. (2022) 12(1):17527. doi: 10.1038/s41598-022-21775-3
54. Frey-Furtado L, Magalhães I, Sampaio-Maia B, Azevedo MJ. Oral microbiome characterization in oral mucositis patients-a systematic review. J Oral Pathol Med. (2023) 52(10):911–8. doi: 10.1111/jop.13492
55. Maki KA, Ganesan SM, Meeks B, Farmer N, Kazmi N, Barb JJ, et al. The role of the oral microbiome in smoking-related cardiovascular risk: a review of the literature exploring mechanisms and pathways. J Transl Med. (2022) 20(1):584. doi: 10.1186/s12967-022-03785-x
56. Graves DT, Corrêa JD, Silva TA. The oral microbiota is modified by systemic diseases. J Dent Res. (2019) 98(2):148–56. doi: 10.1177/0022034518805739
57. Omori M, Kato-Kogoe N, Sakaguchi S, Komori E, Inoue K, Yamamoto K, et al. Characterization of oral microbiota following chemotherapy in patients with hematopoietic malignancies. Integr Cancer Ther. (2023) 22:15347354231159309. doi: 10.1177/15347354231159309
58. Wang Y, Zeng X, Yang X, Que J, Du Q, Zhang Q, et al. Oral health, caries risk profiles, and oral microbiome of pediatric patients with leukemia submitted to chemotherapy. Biomed Res Int. (2021) 2021:6637503. doi: 10.1155/2021/6637503
59. Lee A, Hong J, Shin DY, Koh Y, Yoon SS, Kim PJ, et al. Association of HSV-1 and reduced oral bacteriota diversity with chemotherapy-induced oral mucositis in patients undergoing autologous hematopoietic stem cell transplantation. J Clin Med. (2020) 9(4):1090. doi: 10.3390/jcm9041090
60. Shouval R, Eshel A, Dubovski B, Kuperman AA, Danylesko I, Fein JA, et al. Patterns of salivary microbiota injury and oral mucositis in recipients of allogeneic hematopoietic stem cell transplantation. Blood Adv. (2020) 4(13):2912–7. doi: 10.1182/bloodadvances.2020001827
61. Mosaddad SA, Tahmasebi E, Yazdanian A, Rezvani MB, Seifalian A, Yazdanian M, et al. Oral microbial biofilms: an update. Eur J Clin Microbiol Infect Dis. (2019) 38(11):2005–19. doi: 10.1007/s10096-019-03641-9
62. Könönen E, Fteita D, Gursoy UK, Gursoy M. Prevotella species as oral residents and infectious agents with potential impact on systemic conditions. J Oral Microbiol. (2022) 14(1):2079814. doi: 10.1080/20002297.2022.2079814
63. Abdelsalam NA, Hegazy SM, Aziz RK. The curious case of Prevotella copri. Gut Microbes. (2023) 15(2):2249152. doi: 10.1080/19490976.2023.2249152
64. Könönen E, Wade WG. Actinomyces and related organisms in human infections. Clin Microbiol Rev. (2015) 28(2):419–42. doi: 10.1128/CMR.00100-14
65. McMahon S, Sahasrabhojane P, Kim J, Franklin S, Chang CC, Jenq RR, et al. Contribution of the oral and gastrointestinal microbiomes to bloodstream infections in leukemia patients. Microbiol Spectr. (2023) 11(3):e0041523. doi: 10.1128/spectrum.00415-23
66. Wang S. Timing of blood cultures in the setting of febrile neutropenia: an Australian institutional experience. Turk J Haematol. (2021) 38(1):57–63. doi: 10.4274/tjh.galenos.2020.2020.0302
67. Sardzikova S, Andrijkova K, Svec P, Beke G, Klucar L, Minarik G, et al. Gut diversity and the resistome as biomarkers of febrile neutropenia outcome in paediatric oncology patients undergoing hematopoietic stem cell transplantation. Sci Rep. (2024) 14(1):5504. doi: 10.1038/s41598-024-56242-8
Keywords: oral microbiota, febrile neutropenia, dental infection, oral mucositis, myelosuppressive chemotherapy, solid tumor
Citation: Zecha JAEM, Raber-Durlacher JE, Brandt BW, Buijs MJ, Zaura E, de Lange J, Smeele LE and Laheij AMGA (2024) Oral microbial changes, oral mucositis and febrile neutropenia during myelosuppressive chemotherapy in patients diagnosed with a solid tumor or lymphoma. Front. Oral. Health 5:1461463. doi: 10.3389/froh.2024.1461463
Received: 8 July 2024; Accepted: 24 October 2024;
Published: 14 November 2024.
Edited by:
Gena D. Tribble, University of Texas Health Science Center at Houston, United StatesReviewed by:
Janina P. Lewis, Virginia Commonwealth University, United StatesCopyright: © 2024 Zecha, Raber-Durlacher, Brandt, Buijs, Zaura, de Lange, Smeele and Laheij. This is an open-access article distributed under the terms of the Creative Commons Attribution License (CC BY). The use, distribution or reproduction in other forums is permitted, provided the original author(s) and the copyright owner(s) are credited and that the original publication in this journal is cited, in accordance with accepted academic practice. No use, distribution or reproduction is permitted which does not comply with these terms.
*Correspondence: Judith A. E. M. Zecha, ai56ZWNoYUBhbXN0ZXJkYW11bWMubmw=
Disclaimer: All claims expressed in this article are solely those of the authors and do not necessarily represent those of their affiliated organizations, or those of the publisher, the editors and the reviewers. Any product that may be evaluated in this article or claim that may be made by its manufacturer is not guaranteed or endorsed by the publisher.
Research integrity at Frontiers
Learn more about the work of our research integrity team to safeguard the quality of each article we publish.