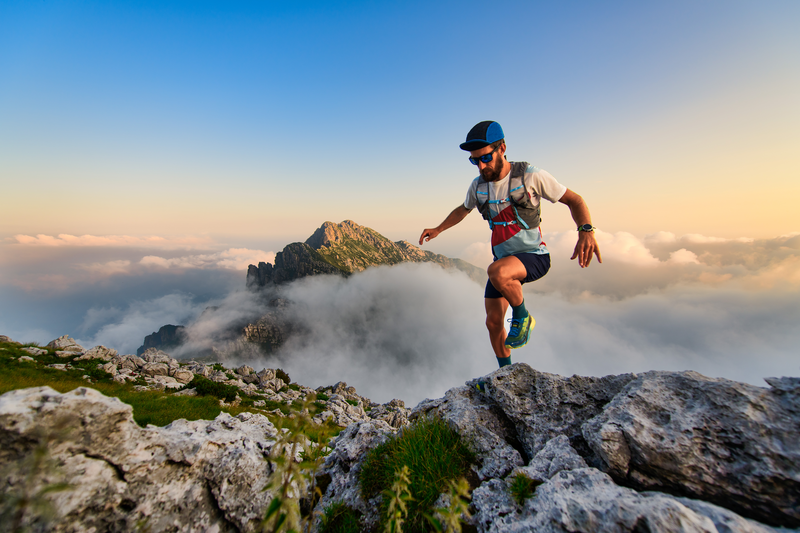
95% of researchers rate our articles as excellent or good
Learn more about the work of our research integrity team to safeguard the quality of each article we publish.
Find out more
ORIGINAL RESEARCH article
Front. Oral. Health , 14 March 2024
Sec. Oral Infections and Microbes
Volume 5 - 2024 | https://doi.org/10.3389/froh.2024.1378566
This article is part of the Research Topic Debates in Oral Infections and Microbes: 2023 View all 4 articles
Introduction: The human host defense peptide LL-37 is a component of the innate immune defense mechanisms of the oral cavity against colonization by microbes associated with periodontal disease. We have previously shown that the active form of vitamin D, 1,25(OH)2D3, can induce the expression of LL-37 in gingival epithelial cells (GEC), and prevent the invasion and growth of periopathogenic bacteria in these cells. Further, experimental vitamin D deficiency resulted in increased gingival inflammation and alveolar bone loss. Epidemiological studies have shown associations between vitamin D deficiency and periodontal disease in humans, suggesting application of vitamin D could be a useful therapeutic approach. Further, since we have shown the local activation of vitamin D by enzymes expressed in the GEC, we hypothesized that we could observe this enhancement with the stable, and inexpensive inactive form of vitamin D, which could be further increased with epigenetic regulators.
Methods: We treated 3-dimensional primary cultures of GEC topically with the inactive form of vitamin D, in the presence and absence of selected histone deacetylase (HDAC) inhibitors. LL-37 mRNA levels were quantified by quantitative RT-PCR, and inhibition of invasion of bacteria was measured by fluorescence microscopy.
Results: Vitamin D treatment led to an induction of LL-37 mRNA levels, as well as an inhibition of pro-inflammatory cytokine secretion. This effect was further enhanced by HDAC inhibitors, most strongly when the HDAC inhibitor, phenyl butyrate (PBA) was combined with Vitamin D3. This was observed both in solution and in a prototype gel formulation using sodium butyrate. Finally, this combination treatment led to an increase in the antimicrobial activity against infection by Porphyromonas gingivalis and Filifactor alocis, bacteria associated with periodontal lesions, as well as herpes simplex virus, which has also been shown to be associated with periodontal lesions.
Conclusions: Our results demonstrate that a combination of inactive vitamin D and sodium butyrate could be developed as a safe treatment for periodontal disease.
Periodontal disease is a chronic inflammatory disease of the gums and supporting tissues that if untreated, can lead to tooth loss and may also affect systemic health. It is associated with the colonization by specific keystone pathogenic bacteria, including Porphyromonas gingivalis, which leads to an inflammatory response and a dysbiosis of the commensal microbiota in the subgingival crevice [reviewed in (1)]. The initial innate immune defense against the microbial pathogens associated with periodontal disease includes a coordinated inflammatory response involving pro-inflammatory cytokines, and mediators, such as prostaglandin E2 (PGE2), as well as the regulated expression of a number of host defense peptides such as β-defensins and cathelicidins [reviewed in (2)]. The only human cathelicidin, LL-37, the product of the CAMP gene, is a multifunctional peptide, with antimicrobial activity against both Gram-positive and Gram-negative bacteria, as well as some viruses [reviewed in (3)]. In addition, it exhibits chemotactic properties and plays a role in dendritic cell maturation, identifying it as an important mediator in the innate and adaptive immune systems [reviewed in (4)]. Lack of LL-37 is associated with two human disorders, Morbus Kostman and Papillion-Lefevre Syndrome, in which there is severe periodontal disease associated with colonization by the periodontal pathogen Aggregatibacter actinomycetemcomitans (5–7). More recently, an association between aggressive periodontitis and mutations in the LL-37 gene has been identified (8). LL-37 gene expression can be induced by live bacteria (9), or by bacterial products such as LPS (10, 11), placing this peptide as part of the innate immune defense of the gingival epithelium.
LL-37 expression can also be induced by the hormonally active form of vitamin D, 1,25-dihydroxyvitamin D3 [1,25(OH)2D3] (12–15). This form is produced by two sequential hydroxylations of vitamin D. It has been generally accepted that this occurs initially by one of a number of 25-hydroxylases in the liver (leading to 25-hydroxyvitamin D3, or 25OHD3), and then by 25-hydroxyvitamin D1α-hydroxylase [1α-(OH)ase] in the kidney (16). However, recently, other cell types, including epithelial, breast, prostate and immune system cells (monocytes, macrophages and dendritic cells) were shown to produce the vitamin D activating 1α-hydroxylase (17, 18), suggesting that the active form of vitamin D can be produced locally. We have demonstrated that both steps can occur in oral epithelial cells (19). In addition to the induction of genes regulated through this pathway, vitamin D has also been shown to inhibit the induced expression of pro-inflammatory cytokines (20). This has been seen with LPS- and IL-1β-induced expression in gingival fibroblasts and periodontal ligament cells (21, 22), suggesting that vitamin D can play an important role in inhibiting the inflammation seen in periodontal disease. These data suggest that the application of inactive vitamin D to the oral epithelium can modulate the local immune system to improve gingival health.
Vitamin D deficiency is a factor associated with both the chronic and aggressive forms of periodontal disease in numerous populations (23–25). While the results of these studies vary, there is a large body of clinical data suggesting that vitamin D plays a role in the maintenance of periodontal health. Furthermore, recent animal experiments showed a reduction in alveolar bone loss by systemic supplementation of vitamin D in experimental models of periodontal disease in mice (26) and rats (27). We hypothesize that this relationship is due to the effect of vitamin D on both the innate immune activity of the gingival epithelium against periodontal pathogens to maintain microbial homeostasis.
We have previously demonstrated the regulation of the innate immune response in gingival epithelial cells (GEC) by 1,25(OH)2D3, including the induction of active LL-37 (28). Active vitamin D-mediated treatment of 3-dimensional cultures of the epithelial cells leads to an increase in antibacterial activity against A. actinomycetemcomitans on the mucosal surface of these cells, suggesting that vitamin D plays a role in the natural antibacterial defense against periodontal pathogens. We have also shown that treatment of both immortalized and primary cultures of GEC with 1,25(OH)2D3 leads to an increased defense against invasion by P. gingivalis, and an inhibition of expression of pro-inflammatory genes such as IL-6 and IL-1α (19). When mice were made deficient in serum vitamin D, they developed gingival inflammation and alveolar bone loss typical of periodontal disease (19). Together, these results support our hypothesis that vitamin D is crucial in the defense against the development of periodontal disease, at both the level of antibacterial defense and the inhibition of inflammation.
The use of the active form of vitamin D, while clearly demonstrating potential for enhancing periodontal host defense, is not therapeutically optimal (29). However, the LL-37 response to the stable and inexpensive inactive form of vitamin D is not very strong, and should we wish to develop a vitamin D-based therapy, we would like to enhance this response. Histone deacetylase (HDAC) inhibitors have been shown to enhance the transcriptional activity of vitamin D (30, 31), as well as the CAMP gene (32). One class of HDAC inhibitors, butyrate compounds, are known to activate the vitamin D receptor (VDR), by increasing the binding of PU.1 and cAMP response element binding protein (CREB1) to the CAMP promoter (33, 34), as well as inhibiting production of PGE2 (35). Thus, we hypothesize that these compounds could be useful in combination therapy with vitamin D as a method to induce LL-37 expression, as well as inhibiting the production of pro-inflammatory mediators. Here we examine the potential for direct delivery of vitamin D in its active and inactive forms, together with HDAC inhibitors, to enhance the innate defense against periodontal pathogens, and to enhance the antimicrobial response of oral epithelial cells.
Cell cultures: The human oral keratinocyte cell line OKF6/TERT-1 was grown in keratinocyte serum free medium (KSFM) supplemented with L-glutamine and penicillin-streptomycin-fungizone (Sigma-Aldrich) in the presence of 0.03 M calcium chloride and bovine pituitary extract as described previously (19). Three-dimensional cultures of normal human gingival epithelial cells were obtained from MatTek. These represent confluent, terminally differentiated, multi-layer primary cultures of ginigival epithelium (EpiGingiva), obtained in 24-well transwell plates obtained from the producer (Supplementary Figure S1). After delivery, the transwells are transferred to 6-well plates into the medium provided, and the cultures are maintained in an air-liquid interface for 1–2 days prior to their use in the experiment.
All inhibitors were obtained from Sigma. Apicidin and valproic acid were dissolved in 100% ethanol at 10 mm and 100 mm respectively. Ellagic acid and MS-275 were dissolved in DMSO at 10 mm and 2.5 mm, respectively. Phenylbutyric acid and nicotinamide were dissolved in water at 1M and 0.2M, respectively. Inhibitors were added to cultures to the following final concentration: apicidin, 10 µm; valproic acid, 100 µm; ellagic acid, 10 µm; MS-275, 2.5 µm; PBA, 1 mm; nicotinamide, 200 µm.
Vitamin D3, 25(OH)D3 and 1,25(OH)2D3 (Sigma) were dissolved in 100% ethanol at 10−5M and kept in the dark at −20°C. Stocks were freshly diluted to 10−7 M in either sterile medium or phosphate buffered saline prior to each use. Control vehicle was 0.1% ethanol in sterile medium or PBS. We observed no toxic effect of either the vitamin D metabolites at this concentration, nor of the vehicle control as measured by MTT assay (data not shown).
PGE2 levels were quantified by ELISA (InVitrogen) using conditions provided by the supplier.
Filifactor alocis ATCC 35896 was cultured in brain heart infusion as previously described in (36). Porphyromonas gingivalis ATCC 33277 was cultured in Tryptic soy broth, supplemented with 5 μg/ml hemin, 250 μg/ml L-cysteine and 1 μg/ml menadione as described previously (37). Bacteria were stained with carboxyfluorescein diacetate succinimidyl ester (CFSE) at a final concentration of 100 µm for 15 min at 37°C, followed by two washes in PBS. Bacteria were resuspended in antibiotic-free basal medium. F. alocis were added to cultured OKF6/TERT-1 cells in chamber slides at an MOI of (200:1) for 90 min, followed by washing the cultures to remove extracellular bacteria. Cultures were stained with DAPI and Texas Red to identify the cells, and were imaged by confocal microscopy. Three to five images in each of triplicate cultures were assessed for efficiency of invasion, which was calculated by dividing the number of cells positive for the presence of bacteria by the total number of cells in the image.
Total nucleic acids were extracted from tissue culture GECs with QiaShredder spin columns and the RNAeasy Plus kit (Qiagen) according to the manufacturer's guidelines. BioRad's iScript cDNA library kit was used according to the supplied directions. Relative mRNA levels were measured with a SsoAdvanced Universal SYBR Green Supermix (BioRad) on a BioRad CFX96 Touch Real-Time PCR Detection System thermal cycler in 96-well plates and quantified according to the 2−ΔΔCT method, relative to β-actin as a housekeeping gene, and control-treated cultures or tissue. Primer sequences are: CYP24A1 (F: 5′-AGTATCTGCCTCGTGTTGTATG-3′; R: 5′-GTGGCCTGGATGTCGTATTT-3′); CAMP (F:5′-CCCTGCTGGGTGATTTCTT-3′; R:5′ GTGGCCTGGATGTCGTATTT-3′); β-actin (F:5′-GGATCAGCAAGCAGGAGTATG-3′; R:5′ AGAAAGGGTACGCAACTAA-3′).
HSV-1 (GFP strain) was propagated in VERO cells as described (38). OKF-6/TERT-1 cells were infected at an MOI of 0.1:1 for 24 h. After infection, cells were harvested and homogenized using Qiagen QIAshredder protocol. The obtained lysate was then used to extract RNA and DNA using the Qiagen RNeasy Plus Micro kit. Once RNA was extracted using the manufacturer protocol, 50 µl of 8.0 mm NaOH was added directly to membrane. Tubes were then incubated for 10 min at 55°C. DNA was eluted by centrifugation for 3 min at 5,000 × g. qPCR was performed on a BIO RAD myCycler using SsoAdvanced Universal SYBR green supermix (Bio-RAD #1725274), using 1.5 µl cellular DNA per reaction, with HSV UL30 primers (F: 3′-AGAGGGACATCCAGGACTTTGT-5′; R: 3′-CAGGCGCTTGTTGGTGTAC5′). DNA levels were normalized to β-actin in each sample and the levels determined using the 2−ΔΔCT method compared to ethanol-treated cells.
Experiments were carried out in triplicate, and repeated at least once for reproducibility. Data were analyzed using GraphPad Prism 10 with unpaired t-tests, one and two-way analysis of variance (ANOVA) with post-hoc testing using Tukey's multiple comparisons test.
Since inactive vitamin D treatment can regulate expression of LL-37 (19), albeit at a lesser efficiency than does active 1,25(OH)2D3 (19), we wished to examine the potential to enhance this response. We treated OKF6/TERT-1 cells with vitamin D3 or in combination with HDAC inhibitors for 24 h, followed by quantification of LL-37 mRNA. Specifically, we used nicotinamide (an inhibitor of Class III HADACs); phenylbutyrate (PBA) and valproic acid (inhibitors of Class I and Class IIa HDACs); apicidin (a class 1 HDAC inhibitor), and MS-275 (an inhibitor of HDACs 1-III). We also tested ellagic acid, which is an inhibitor of coactivator associated arginine methyltransferase 1 (CARM1). The results shown in Figure 1 demonstrate that inactive vitamin D3 at 1 µm was sufficient to induce LL-37 mRNA levels from 5 to 10 fold, as did both MS-275 and PBA alone. Further, MS-275, PBA, valproic acid and ellagic acid all enhanced the expression of LL-37 when combined with vitamin D3. Nicotinamide had no effect, either alone or in combination with vitamin D3.
Figure 1. Effect of HDAC inhibitors on inactive vitamin D3-mediated induction of LL-37 gene expression in oral keratinocytes. OKF6/TERT-1 cells were incubated with either 0.1% Ethanol (EtOH) in the presence or absence of 1 µm inactive vitamin D3 for 24 h. Added to each was HDAC inhibitors at the following concentrations: PBA (1 mm); MS-275 (2 mm); Apidacin (1 µm); Ellagic acid (2 mm); Valproic acid (100 µm); and Nicotinamide (2 mm). Total mRNA was isolated and relative LL-37 mRNA levels were quantified by QRT-PCR relative to β-actin. Data are shown as mean (n = 3) ±SD. *p < 0.05; **p < 0.005; ***p < 0.0005; ****p < 0.00005 by 2-way ANOVA and Tukey's post-hoc test.
Considering all of the HDAC inhibitors tested, PBA provided the greatest enhancement of vitamin D3-mediated LL-37 expression. To examine this effect further, we carried out a dose-response study of PBA between 100 µm and 1 mm. The results in Figure 2A show a dose-dependent enhancement of vitamin D3 induction of the VDR-mediated gene CYP24A1. We see a similar response of LL-37 (Figure 2B), indicating that this combination could be used to induce LL-37 in tissues. One main difference, however, is in response to PBA alone. There is no induction of CYP24A1 to PBA, while 1 mm PBA treatment led to an induction of LL-37.
Figure 2. Synergistic effect of vitamin D3 and butyrate compounds on LL-37 and PGE2 in 3D cultures of gingival epithelial cells. OKF6/TERT-1 cells (A,B) or 3D air-liquid interface EpiGingiva cultures (C,D) were treated with vitamin D3, PBA or NaB, or a combination for 24 h. Vitamin D3 and butyrate were added to the medium in (A,B), and in 10 µl on the apical surface for (C,D). Total mRNA was isolated and relative LL-37 mRNA levels were quantified by QRT-PCR. Basolateral medium was removed, and PGE2 concentrations were quantified by ELISA (D) Data are shown as mean (n = 3) ±SD. *p < 0.05; **p < 0.005, by t-test.
To examine the effect of topical delivery, we repeated the experiments using administration of vitamin D and butyrate to the apical surface of 3D cultures (Supplementary Figure S1). The results in Figure 2C show an induction of LL-37 expression in response to topical administration of both 10 µm Vitamin D3 and 1 mm PBA. However, since PBA is not well-tolerated for topical oral applications, we repeated the experiment using sodium butyrate (NaB). The results show a similar LL-37 induction, with NaB enhancing the vitamin D3 response in topical application to 3D cultures both alone, and in synergy with vitamin D (Figure 2C). Furthermore, we also see a concomitant reduction of the pro-inflammatory mediator PGE2, as measured by ELISA of the basolateral medium (Figure 2D).
Since a topical treatment in vivo would require a much shorter challenge with the inducing agent than the in vitro studies above, we developed gel formulations containing a high concentration of vitamin D3 alone (0.01%w/v, which equals 260 µm), or in combination with NaB (0.25%w/v, 227 mm). To quantify the effect of a short-term treatment with these formulations, we treated OKF6/TERT cells with these gels for 10 min. After the treatment, the cultures were washed twice with fresh medium, and incubated for a further 3 h in medium without vitamin D3. Total mRNA was isolated, and the response of CYP24A1 and LL-37 were quantified by qRT-PCR. The results in Figure 3 show that even a very short treatment with a supra-physiological concentration of vitamin D together with NaB was sufficient to lead to a rapid response of the cells. The combination of vitamin D and NaB induced a significant increase in LL-37 expression when compared to either control or vitamin D treatment alone.
Figure 3. Effect of gel formulations with vitamin D3 on gene expression. Gels containing vitamin D3, or a combination of vitamin D and NaB at the concentrations shown were added to OKF6/TERT1 cells for 10 min, rinsed, then the cultures were incubated in fresh medium for a further 6 h. Relative mRNA was quantified for CYP24A1 (A) and LL-37 (B) levels by QRT-PCR. Data are shown as mean (n = 3) ±SD. *p < 0.05 by t-test.
To demonstrate a protective effect of vitamin D and butyrate treatment on oral epithelial cells against microbial challenge, we pre-treated OKF6/TERT cells with Vitamin D3 in the presence or absence of 2 mm sodium butyrate, followed by infection with microbial challenge. Both P. gingivalis and F. alocis are associated with the development of periodontitis, and have been shown to invade gingival epithelial cells (39). The results in Figures 4A,B show that both vitamin D3 alone and NaB alone were sufficient to reduce the invasion of bacteria. There was a further reduction of P. gingivalis by the combination, to 2.3% of control, which was not observed with F. alocis.
Figure 4. Inhibition of microbial infection by pretreatment with vitamin D and butyrate. (A,B) Bacterial infection. OKF6/TERT-1 cells were incubated for 24 h with 100 nm Vitamin D3, 2 mm Sodium butyrate (NaB), or a combination of 2 mm sodium butyrate and 100 nm Vitamin D3. After 24 h, cells were infected with either P. gingivalis (A) or F. alocis (B) at an MOI of 500:1 or 200:1, respectively. Bacteria were detected by fluorescent microscopy using 400 µg/ml CFSE and % infected was calculated. (C) Intracellular vs. extracellular killing. OKF6/TERT-1 cells were incubated for 24 h with 100 nm 1,25(OH)2D3, 2 mm NaB, or a combination of butyrate and 1,25(OH)2D3. Medium was removed, cells were washed once, and infected with P. gingivalis at an MOI of 500:1. Intracellular bacteria were quantified after 2 h. (D) Viral infection. OKF6/TERT-1 cells were incubated for 24 h with 100 nm Vitamin D3, 2 mm Sodium butyrate (NaB), or a combination of 2 mm sodium butyrate and Vitamin D3. After 24 h, cells were infected with HSV-1 at an MOI of 0.1:1. After 24 h, total DNA was isolated and relative HSV-1 DNA levels were quantified by QPCR normalized to genomic β-actin DNA. Data are shown as mean (n = 3) ±SD. *p < 0.05; **p < 0.005, by t-test.
To determine whether the increase in killing was due to secretion of antibacterial factors, such as LL-37, or to an increase in intracellular killing, we repeated the experiment, but removed the medium after the vitamin D/NaB treatment prior to bacterial challenge. The results in Figure 4C show that removal of the vitamin D-treated medium resulted in an inhibition of the reduction in intracellular P. gingivalis, suggesting that a significant portion of the vitamin D treatment leads to the prevention of invasion by extracellular killing. However, removal of the medium after treatment with NaB led to a 60% inhibiton of invasion by P. gingivalis.
Herpesviruses are often associated with periodontal lesions, so we quantified the combined effect of vitamin D and butyrate treatment on infection of oral epithelial cells with HSV-1. The results in Figure 4D show no reduction of HSV-1 infection by Vitamin D3 alone, however NaB was sufficient to almost completely prevent HSV-1 infection. The combination of Vitamin D3 and NaB gave a similar inhibitory response to NaB alone.
Vitamin D deficiency is associated with periodontal disease in human populations, as determined by a meta-analysis of numerous worldwide studies (40). We also demonstrated an association between vitamin D deficiency and characteristics of periodontal disease in experimental mice, including increased inflammation in the gingiva, and alveolar bone loss (19). Numerous studies have shown that the active form of vitamin D, 1,25(OH)2D3, can induce the expression of LL-37, and inhibit the expression of pro-inflammatory cytokines in gingival epithelium [reviewed in (41)]. The intermediate inactive form, 25OHD3, has also been shown to carry out this activity, as the activating enzyme, 1-α hydroxylase (encoded by the CYP24A1 gene) is expressed in oral epithelial cells (28), gingival fibroblasts and periodontal ligament cells (42, 43). The vast majority of research into the activity and effects of vitamin D in human health has focused on systemic use, usually by oral supplementation, as vitamin D is very safe, even at high doses, and is easily absorbed and stored in the body (44). This has led to mixed results, most likely because oral supplementation can only increase serum 25OHD3 levels [and tissue levels of 1,25(OH)2D3] to a limited degree. Similarly, Gui et al. (45) showed that while systemic administration of 1,25(OH)2D3 (by daily intraperitoneal injection over two weeks) initially led to reduced inflammation, there was increased inflammation in the long term. Since others have shown that HDAC inhibitors can enhance the effect of 1,25(OH)2D3 (46–48), and we have demonstrated that the gingival epithelial cells are capable of converting the inactive cholecalciferol form to the active form, we hypothesized that a combination of inactive vitamin D3 and HDAC inhibitors could bolster the LL-37-specific innate immune defense against oral pathogens that can lead to gingival inflammation and other infectious conditions.
Since oral supplementation together with HDAC inhibitors is not a viable option for periodontal disease, we tested a topical administration in vitro, using 3D-cultures of gingival epithelial cells. Our results indicate that the combination of a supraphysiological concentration of inactive vitamin D3 and butyrate compounds was sufficient to enhance LL-37 gene expression in these cultures. LL-37 expression was also enhanced with the combinations of the active form of vitamin D and buyrate (data not shown). While topical administration of active vitamin D3 analogues has been used in combination with butyrate compounds to treat dermatological conditions [see, for example, (49)], this is the first demonstration that it could be used for topical oral applications to improve periodontal disease.
The induction of LL-37 gene expression in cultured GECs by vitamin D3 occurs at a lower level of efficiency than 25OHD3 or 1,25(OH)2D3 (19). This is most likely due to the inefficiency of the epithelial cell-mediated two-step activation of vitamin D. While the physiological concentration of active vitamin D3 is approximately 10 nm, for most experiments we increased the concentration of vitamin D3 to 10 µm, to account for low efficiency, which provided a similar level of gene induction. However, since it is known that HDAC inhibitors can enhance the effect of VDR-mediated LL-37 gene expression (31, 32, 50), we tested several inhibitors for their ability to enhance the effect of inactive vitamin D3 in the induction of LL-37 in gingival epithelial cell cultures. Our results clearly demonstrate that while inactive vitamin D3 can induce the expression of LL-37, this is regulated by several classes of HDACs, as is evidenced by the synergistic enhancement of four of the six inhibitors tested, which affect different classes of HDACs. As these inhibitors affect different classes of HDACs, this suggests that the vitamin D-mediated induction of LL-37 is complex, involving several mediators of transcription. Furthermore, the enhancement by ellagic acid suggests that the regulation also involves histone methylation via CARM1, similar to the regulation of CYP24A1 by 1,25(OH)2D3 (51). Further, addition of butyrate compounds was able to enhance this activity in both submerged cultures and with topical application to the apical surface of 3D-cultures to a great extent. Phenylbutyric acid (PBA) is an epigenetic modifier that enhances the LL-37 transcriptional activity of vitamin D3, although its activity may be through multiple mechanisms (52, 53), and thus its use as a therapeutic agent to act in synergy with vitamin D3 currently being investigated (54). Interestingly, one inhibitor, apicidin, reduced the effect of vitamin D on LL-37 expression, suggesting that its regulation of vitamin D-mediated gene expression can occur in both directions.
Periodontal disease is characterized by a dysbiosis of the gingival crevice, with P. gingivalis often considered a primary player in microbiota of the periodontal lesion. Our previous data has shown that pre-treatment of primary cultures of gingival epithelial cells with 1,25(OH)2D3 leads to a reduction in intracellular P. gingivalis, suggesting that the induced LL-37 enhances the innate antibacterial defense of the cell (19). Other pathogens are also found associated with the periodontal lesion, including F. alocis (55). Our data here show that we can recapitulate this response against P. gingivalis and F. alocis with both inactive vitamin D and butyrate. In addition, we observe a difference in their activities, in that removal of the extracellular medium after stimulation prevents this killing, suggesting that the activity is due to secretion of LL-37. However, there is still a significant amount of NaB-stimulated killing that occurs intracellularly. This suggests that butyrate may stimulate the production of other antimicrobial agents that may work intracellularly.
Members of the herpesvirus family are also found associated with periodontal lesions [reviewed in (56)]. To demonstrate that induction of LL-37 leads to a broad-spectrum antimicrobial enhancement of activity, we also treated oral epithelial cells with vitamin D3 and NaB to show a reduction in infection by both F. alocis and HSV-1 in the presence of NaB. The reduction of viral infection was similar to the pre-incubation of purified virus with 20 µg/ml LL-37 prior to infection in oral epithelial cells (57), a result similar those observed with LL-37 against HSV-1 infection of ocular epithelial cells (58). Similarly, we demonstrated that LL-37 could inactivate a related virus, Kaposi's Sarcoma-associated Herpes Virus (KSHV) through disruption of the viral envelope (59). We speculate that the induction of LL-37 is inactivating HSV-1 through the same mechanism, and would work together with the other innate immune antiviral defense mechanisms of the oral cavity (60). A similar observation has been made that LL-37 can transport the microbial DNA sensor cGAMP to enhance antiviral innate immunity (61). Others have demonstrated that treatment of cultured airway epithelial cells with 1,25(OH)2D3 can lead to an inhibition of infection by respiratory syncytial virus, and influenza virus infection, and the associated inflammatory responses (45, 62–64). However, since our observations indicate that NaB is sufficient for induction of antiviral activity, a non-LL-37 based mechanism is also possible. The butyrate-secreting probiotic bacterium Clostridium butyricum has been shown to induce antiviral activity through the induction of antiviral and interferon-related genes (65). This suggests that, like our observations with bacteria, butyrate can induce multiple antimicrobial pathways. Our current results suggest the potential use of inactive topical vitamin D3 in combination with an HDAC inhibitor, such as NaB, in as an adjunctive therapy for periodontal disease.
The raw data supporting the conclusions of this article will be made available by the authors, without undue reservation.
Ethical approval was not required for the studies on humans in accordance with the local legislation and institutional requirements because only commercially available established cell lines were used.
EF: Investigation, Writing – review & editing. PA: Writing – review & editing. DG: Investigation, Writing – review & editing. EP: Investigation, Writing – review & editing. RA: Investigation, Writing – review & editing. CD: Supervision, Writing – review & editing. GK: Investigation, Writing – review & editing. LR: Conceptualization, Methodology, Writing – review & editing. GD: Conceptualization, Supervision, Writing – original draft, Writing – review & editing.
The author(s) declare financial support was received for the research, authorship, and/or publication of this article.
This study was supported by funding from Colgate-Palmolive, Inc., and grants from the US Public Health Service: NIH R01DE028883 and R01DE031928 to GD. EP was a recipient of the University of Florida University Scholars Award.
Authors PA, RA, and CD were employed by company Colgate Palmolive Company.
The remaining authors declare that the research was conducted in the absence of any commercial or financial relationships that could be construed as a potential conflict of interest.
This study received funding from Colgate-Palmolive Company. The funder had the following involvement with the study: study design and interpretation of the data.
The author(s) declared that they were an editorial board member of Frontiers, at the time of submission. This had no impact on the peer review process and the final decision.
All claims expressed in this article are solely those of the authors and do not necessarily represent those of their affiliated organizations, or those of the publisher, the editors and the reviewers. Any product that may be evaluated in this article, or claim that may be made by its manufacturer, is not guaranteed or endorsed by the publisher.
The Supplementary Material for this article can be found online at: https://www.frontiersin.org/articles/10.3389/froh.2024.1378566/full#supplementary-material
Supplementary Figure S1
Three-dimensional model of gingival epithelium. EpiGingival cultures obtained from Mattek, Inc. are grown on porous filter inserts, maintained in basolateral serum-free medium. Vitamin D treatments are applied to the apical surface.
1. Hajishengallis G. Periodontitis: from microbial immune subversion to systemic inflammation. Nat Rev Immunol. (2015) 15(1):30–44. doi: 10.1038/nri3785
2. Diamond G, Beckloff N, Ryan LK. Host defense peptides in the oral cavity and the lung: similarities and differences. J Dent Res. (2008) 87(10):915–27. doi: 10.1177/154405910808701011
3. Zanetti M. Cathelicidins, multifunctional peptides of the innate immunity. J Leukoc Biol. (2004) 75(1):39–48. doi: 10.1189/jlb.0403147
4. Bowdish DM, Davidson DJ, Hancock RE. Immunomodulatory properties of defensins and cathelicidins. Curr Top Microbiol Immunol. (2006) 306:27–66.16909917
5. Carlsson G, Wahlin YB, Johansson A, Olsson A, Eriksson T, Claesson R, et al. Periodontal disease in patients from the original Kostmann family with severe congenital neutropenia. J Periodontol. (2006) 77(4):744–51. doi: 10.1902/jop.2006.050191
6. de Haar SF, Hiemstra PS, van Steenbergen MT, Everts V, Beertsen W. Role of polymorphonuclear leukocyte-derived serine proteinases in defense against Actinobacillus actinomycetemcomitans. Infect Immun. (2006) 74(9):5284–91. doi: 10.1128/IAI.02016-05
7. Putsep K, Carlsson G, Boman HG, Andersson M. Deficiency of antibacterial peptides in patients with Morbus Kostmann: an observation study. Lancet. (2002) 360(9340):1144–9. doi: 10.1016/S0140-6736(02)11201-3
8. Turkoglu O, Berdeli A, Emingil G, Atilla G. A novel p.S34N mutation of CAMP gene in patients with periodontal disease. Arch Oral Biol. (2011) 56(6):573–9. doi: 10.1016/j.archoralbio.2010.11.016
9. Midorikawa K, Ouhara K, Komatsuzawa H, Kawai T, Yamada S, Fujiwara T, et al. Staphylococcus aureus susceptibility to innate antimicrobial peptides, beta-defensins and CAP18, expressed by human keratinocytes. Infect Immun. (2003) 71(7):3730–9. doi: 10.1128/IAI.71.7.3730-3739.2003
10. Nell MJ, Tjabringa GS, Vonk MJ, Hiemstra PS, Grote JJ. Bacterial products increase expression of the human cathelicidin hCAP-18/LL-37 in cultured human sinus epithelial cells. FEMS Immunol Med Microbiol. (2004) 42(2):225–31. doi: 10.1016/j.femsim.2004.05.013
11. Larrick JW, Hirata M, Balint RF, Lee J, Zhong J, Wright SC. Human CAP18: a novel antimicrobial lipopolysaccharide-binding protein. Infect Immun. (1995) 63:1291–97. doi: 10.1128/iai.63.4.1291-1297.1995
12. Gombart AF, Borregaard N, Koeffler HP. Human cathelicidin antimicrobial peptide (CAMP) gene is a direct target of the vitamin D receptor and is strongly up-regulated in myeloid cells by 1,25-dihydroxyvitamin D3. FASEB J. (2005) 19(9):1067–77. doi: 10.1096/fj.04-3284com
13. Wang TT, Nestel FP, Bourdeau V, Nagai Y, Wang Q, Liao J, et al. Cutting edge: 1,25-dihydroxyvitamin D3 is a direct inducer of antimicrobial peptide gene expression. J Immunol. (2004) 173(5):2909–12. doi: 10.4049/jimmunol.173.5.2909
14. Liu PT, Stenger S, Li H, Wenzel L, Tan BH, Krutzik SR, et al. Toll-like receptor triggering of a vitamin D-mediated human antimicrobial response. Science. (2006) 311(5768):1770–3. doi: 10.1126/science.1123933
15. Yim S, Dhawan P, Ragunath C, Christakos S, Diamond G. Induction of cathelicidin in normal and CF bronchial epithelial cells by 1,25-dihydroxyvitamin D3. J Cystic Fibrosis. (2007) 6:403–10. doi: 10.1016/j.jcf.2007.03.003
16. Bikle DD, Adams JS, Christakos S. Vitamin D: production, metabolism, mechanism of action and clinical requirements. In: Rosen CJ, editor. Primer on the Metabolic Bone Diseases and Disorders of Mineral Metabolism. Hoboken: Wiley Blackwell (2013). p. 235–48.
17. Bikle DD. Vitamin D: newly discovered actions require reconsideration of physiologic requirements. Trends Endocrinol Metab. (2010) 21(6):375–84. doi: 10.1016/j.tem.2010.01.003
18. Lin R, White JH. The pleiotropic actions of vitamin D. Bioessays. (2004) 26(1):21–8. doi: 10.1002/bies.10368
19. Menzel LP, Ruddick W, Chowdhury MH, Brice DC, Clance R, Porcelli E, et al. Activation of vitamin D in the gingival epithelium and its role in gingival inflammation and alveolar bone loss. J Periodontal Res. (2019) 54(4):444–52. doi: 10.1111/jre.12646
20. Sun J. Vitamin D and mucosal immune function. Curr Opin Gastroenterol. (2010) 26(6):591–5. doi: 10.1097/MOG.0b013e32833d4b9f
21. Andrukhov O, Andrukhova O, Hulan U, Tang Y, Bantleon HP, Rausch-Fan X. Both 25-hydroxyvitamin-D3 and 1,25-dihydroxyvitamin-D3 reduces inflammatory response in human periodontal ligament cells. PLoS One. (2014) 9(2):e90301. doi: 10.1371/journal.pone.0090301
22. Nakashyan V, Tipton DA, Karydis A, Livada R, Stein SH. Effect of 1,25(OH)2 D3 and 20(OH)D3 on interleukin-1beta-stimulated interleukin-6 and -8 production by human gingival fibroblasts. J Periodontal Res. (2017) 52(5):832–41. doi: 10.1111/jre.12452
23. Antonoglou GN, Knuuttila M, Niemela O, Raunio T, Karttunen R, Vainio O, et al. Low serum level of 1,25(OH)2 D is associated with chronic periodontitis. J Periodontal Res. (2015) 50(2):274–80. doi: 10.1111/jre.12207
24. Laky M, Bertl K, Haririan H, Andrukhov O, Seemann R, Volf I, et al. Serum levels of 25-hydroxyvitamin D are associated with periodontal disease. Clin Oral Investig. (2017) 21(5):1553–8. doi: 10.1007/s00784-016-1965-2
25. Lee HJ, Je DI, Won SJ, Paik DI, Bae KH. Association between vitamin D deficiency and periodontal status in current smokers. Community Dent Oral Epidemiol. (2015) 43(5):471–8. doi: 10.1111/cdoe.12173
26. Li H, Zhong X, Li W, Wang Q. Effects of 1,25-dihydroxyvitamin D3 on experimental periodontitis and AhR/NF-κB/NLRP3 inflammasome pathway in a mouse model. J Appl Oral Sci. (2019) 27:e20180713. doi: 10.1590/1678-7757-2018-0713
27. Bi CS, Wang J, Qu HL, Li X, Tian BM, Ge S, et al. Calcitriol suppresses lipopolysaccharide-induced alveolar bone damage in rats by regulating T helper cell subset polarization. J Periodontal Res. (2019) 54(6):612–23. doi: 10.1111/jre.12661
28. McMahon L, Schwartz K, Yilmaz O, Brown E, Ryan LK, Diamond G. Vitamin D-mediated induction of innate immunity in gingival epithelial cells. Infect Immun. (2011) 79(6):2250–6. doi: 10.1128/IAI.00099-11
29. Plum LA, DeLuca HF. Vitamin D, disease and therapeutic opportunities. Nat Rev Drug Discov. (2010) 9(12):941–55. doi: 10.1038/nrd3318
30. Chandel N, Malhotra A, Singhal PC. Vitamin D receptor and epigenetics in HIV infection and drug abuse. Front Microbiol. (2015) 6:788. doi: 10.3389/fmicb.2015.00788
31. Wei R, Dhawan P, Baiocchi RA, Kim KY, Christakos S. PU.1 and epigenetic signals modulate 1,25-dihydroxyvitamin D(3) and C/EBPalpha regulation of the human cathelicidin antimicrobial peptide gene in lung epithelial cells. J Cell Physiol. (2019) 234(7):10345–59. doi: 10.1002/jcp.27702
32. Miraglia E, Nylen F, Johansson K, Arner E, Cebula M, Farmand S, et al. Entinostat up-regulates the CAMP gene encoding LL-37 via activation of STAT3 and HIF-1alpha transcription factors. Sci Rep. (2016) 6:33274. doi: 10.1038/srep33274
33. Chakraborty K, Maity PC, Sil AK, Takeda Y, Das S. cAMP stringently regulates human cathelicidin antimicrobial peptide expression in the mucosal epithelial cells by activating cAMP-response element-binding protein, AP-1, and inducible cAMP early repressor. J Biol Chem. (2009) 284(33):21810–27. doi: 10.1074/jbc.M109.001180
34. Termen S, Tollin M, Rodriguez E, Sveinsdottir SH, Johannesson B, Cederlund A, et al. PU.1 and bacterial metabolites regulate the human gene CAMP encoding antimicrobial peptide LL-37 in colon epithelial cells. Mol Immunol. (2008) 45(15):3947–55. doi: 10.1016/j.molimm.2008.06.020
35. Zhang L, Deng M, Lu A, Chen Y, Chen Y, Wu C, et al. Sodium butyrate attenuates angiotensin II-induced cardiac hypertrophy by inhibiting COX2/PGE2 pathway via a HDAC5/HDAC6-dependent mechanism. J Cell Mol Med. (2019) 23(12):8139–50. doi: 10.1111/jcmm.14684
36. Iskander MMZ, Lamont GJ, Tan J, Pisano M, Uriarte SM, Scott DA. Tobacco smoke exacerbates Filifactor alocis pathogenicity. J Clin Periodontol. (2023) 50(1):121–30. doi: 10.1111/jcpe.13729
37. Moradali MF, Ghods S, Bahre H, Lamont RJ, Scott DA, Seifert R. Atypical cyclic di-AMP signaling is essential for Porphyromonas gingivalis growth and regulation of cell envelope homeostasis and virulence. NPJ Biofilms Microbiomes. (2022) 8(1):53. doi: 10.1038/s41522-022-00316-w
38. Ryan LK, Dai J, Yin Z, Megjugorac N, Uhlhorn V, Yim S, et al. Modulation of human beta-defensin-1 (hBD-1) in plasmacytoid dendritic cells (PDC), monocytes, and epithelial cells by influenza virus, herpes simplex virus, and sendai virus and its possible role in innate immunity. J Leukoc Biol. (2011) 90:343–56. doi: 10.1189/jlb.0209079
39. Aruni AW, Roy F, Fletcher HM. Filifactor alocis has virulence attributes that can enhance its persistence under oxidative stress conditions and mediate invasion of epithelial cells by Porphyromonas gingivalis. Infect Immun. (2011) 79(10):3872–86. doi: 10.1128/IAI.05631-11
40. Machado V, Lobo S, Proenca L, Mendes JJ, Botelho J. Vitamin D and periodontitis: a systematic review and meta-analysis. Nutrients. (2020) 12(8):e44–e53. doi: 10.3390/nu12082177
41. Lu EM. The role of vitamin D in periodontal health and disease. J Periodontal Res. (2023) 58(2):213–24. doi: 10.1111/jre.13083
42. Liu K, Meng H, Hou J. Characterization of the autocrine/paracrine function of vitamin D in human gingival fibroblasts and periodontal ligament cells. PLoS One. (2012) 7(6):e39878. doi: 10.1371/journal.pone.0039878
43. Zhang C, Liu K, Hou J. Extending the vitamin D pathway to vitamin D3 and CYP27A1 in periodontal ligament cells. J Periodontol. (2020) 92(7):44–53. doi: 10.1002/JPER.20-0225
44. Kearns MD, Alvarez JA, Seidel N, Tangpricha V. Impact of vitamin D on infectious disease. Am J Med Sci. (2015) 349(3):245–62. doi: 10.1097/MAJ.0000000000000360
45. Gui B, Chen Q, Hu C, Zhu C, He G. Effects of calcitriol (1, 25-dihydroxy-vitamin D3) on the inflammatory response induced by H9N2 influenza virus infection in human lung A549 epithelial cells and in mice. Virol J. (2017) 14(1):10. doi: 10.1186/s12985-017-0683-y
46. Hossain S, Liu Z, Wood RJ. Association between histone deacetylase activity and vitamin D-dependent gene expressions in relation to sulforaphane in human colorectal cancer cells. J Sci Food Agric. (2021) 101(5):1833–43. doi: 10.1002/jsfa.10797
47. Li C, Chen Y, Zhu H, Zhang X, Han L, Zhao Z, et al. Inhibition of histone deacetylation by MS-275 alleviates colitis by activating the vitamin D receptor. J Crohns Colitis. (2020) 14(8):1103–18. doi: 10.1093/ecco-jcc/jjaa016
48. Miki H, Okito A, Akiyama M, Ono T, Tachikawa N, Nakahama KI. Genetic and epigenetic regulation of osteopontin by cyclic adenosine 3′ 5′-monophosphate in osteoblasts. Gene. (2020) 763:145059. doi: 10.1016/j.gene.2020.145059
49. Okubo Y, Natsume S, Usui K, Muro M, Tsuboi R. Combination therapy using maxacalcitol and corticosteroid lotions preliminary to monotherapy with maxacalcitol lotion for scalp psoriasis. J Dermatolog Treat. (2014) 25(1):34–7. doi: 10.3109/09546634.2012.687087
50. Liu Q, Liu J, Roschmann KIL, van Egmond D, Golebski K, Fokkens WJ, et al. Histone deacetylase inhibitors up-regulate LL-37 expression independent of toll-like receptor mediated signalling in airway epithelial cells. J Inflamm (Lond). (2013) 10(1):15. doi: 10.1186/1476-9255-10-15
51. Mady LJ, Zhong Y, Dhawan P, Christakos S. Role of coactivator associated arginine methyltransferase 1 (CARM1) in the regulation of the biological function of 1,25-dihydroxyvitamin D(3). Cells. (2023) 12(10):1407. doi: 10.3390/cells12101407
52. Kulkarni NN, Yi Z, Huehnken C, Agerberth B, Gudmundsson GH. Phenylbutyrate induces cathelicidin expression via the vitamin D receptor: linkage to inflammatory and growth factor cytokines pathways. Mol Immunol. (2015) 63(2):530–9. doi: 10.1016/j.molimm.2014.10.007
53. Steinmann J, Halldorsson S, Agerberth B, Gudmundsson GH. Phenylbutyrate induces antimicrobial peptide expression. Antimicrob Agents Chemother. (2009) 53(12):5127–33. doi: 10.1128/AAC.00818-09
54. Mily A, Rekha RS, Kamal SM, Arifuzzaman AS, Rahim Z, Khan L, et al. Significant effects of oral phenylbutyrate and vitamin D3 adjunctive therapy in pulmonary Tuberculosis: a randomized controlled trial. PLoS One. (2015) 10(9):e0138340. doi: 10.1371/journal.pone.0138340
55. Aruni AW, Mishra A, Dou Y, Chioma O, Hamilton BN, Fletcher HM. Filifactor alocis–a new emerging periodontal pathogen. Microbes Infect. (2015) 17(7):517–30. doi: 10.1016/j.micinf.2015.03.011
56. Slots J. Herpesviruses in periodontal diseases. Periodontol 2000. (2005) 38:33–62. doi: 10.1111/j.1600-0757.2005.00109.x
57. Diamond G, Molchanova N, Herlan C, Fortkort JA, Lin JS, Figgins E, et al. Potent antiviral activity against HSV-1 and SARS-CoV-2 by antimicrobial peptoids. Pharmaceuticals (Basel). (2021) 14(4):304. doi: 10.3390/ph14040304
58. Gordon YJ, Huang LC, Romanowski EG, Yates KA, Proske RJ, McDermott AM. Human cathelicidin (LL-37), a multifunctional peptide, is expressed by ocular surface epithelia and has potent antibacterial and antiviral activity. Curr Eye Res. (2005) 30(5):385–94. doi: 10.1080/02713680590934111
59. Brice DC, Toth Z, Diamond G. LL-37 disrupts the Kaposi’s sarcoma-associated herpesvirus envelope and inhibits infection in oral epithelial cells. Antiviral Res. (2018) 158:25–33. doi: 10.1016/j.antiviral.2018.07.025
60. Brice DC, Figgins E, Yu F, Diamond G. Type I interferon and interferon-stimulated gene expression in oral epithelial cells. Mol Oral Microbiol. (2019) 34:245–53. doi: 10.1111/omi.12270
61. Wei X, Zhang L, Yang Y, Hou Y, Xu Y, Wang Z, et al. LL-37 transports immunoreactive cGAMP to activate STING signaling and enhance interferon-mediated host antiviral immunity. Cell Rep. (2022) 39(9):110880. doi: 10.1016/j.celrep.2022.110880
62. Khare D, Godbole NM, Pawar SD, Mohan V, Pandey G, Gupta S, et al. Calcitriol [1, 25(OH)2 D3] pre- and post-treatment suppresses inflammatory response to influenza A (H1N1) infection in human lung A549 epithelial cells. Eur J Nutr. (2013) 52(4):1405–15. doi: 10.1007/s00394-012-0449-7
63. Telcian AG, Zdrenghea MT, Edwards MR, Laza-Stanca V, Mallia P, Johnston SL, et al. Vitamin D increases the antiviral activity of bronchial epithelial cells in vitro. Antiviral Res. (2017) 137:93–101. doi: 10.1016/j.antiviral.2016.11.004
64. Vaccaro JA, Qasem A, Naser SA. Cathelicidin mediates an anti-inflammatory role of active vitamin D (calcitriol) during M. paratuberculosis infection. Front Cell Infect Microbiol. (2022) 12:875772. doi: 10.3389/fcimb.2022.875772
Keywords: anti-inflammatory, antiviral, antimicrobial peptide, vitamin D, periodontal
Citation: Figgins EL, Arora P, Gao D, Porcelli E, Ahmed R, Daep CA, Keele G, Ryan LK and Diamond G (2024) Enhancement of innate immunity in gingival epithelial cells by vitamin D and HDAC inhibitors. Front. Oral. Health 5:1378566. doi: 10.3389/froh.2024.1378566
Received: 29 January 2024; Accepted: 5 March 2024;
Published: 14 March 2024.
Edited by:
Richard Cannon, University of Otago, New ZealandReviewed by:
Ashu Sharma, University at Buffalo, United States© 2024 Figgins, Arora, Gao, Porcelli, Ahmed, Daep, Keele, Ryan and Diamond. This is an open-access article distributed under the terms of the Creative Commons Attribution License (CC BY). The use, distribution or reproduction in other forums is permitted, provided the original author(s) and the copyright owner(s) are credited and that the original publication in this journal is cited, in accordance with accepted academic practice. No use, distribution or reproduction is permitted which does not comply with these terms.
*Correspondence: Gill Diamond Z2lsbC5kaWFtb25kQGxvdWlzdmlsbGUuZWR1
Disclaimer: All claims expressed in this article are solely those of the authors and do not necessarily represent those of their affiliated organizations, or those of the publisher, the editors and the reviewers. Any product that may be evaluated in this article or claim that may be made by its manufacturer is not guaranteed or endorsed by the publisher.
Research integrity at Frontiers
Learn more about the work of our research integrity team to safeguard the quality of each article we publish.