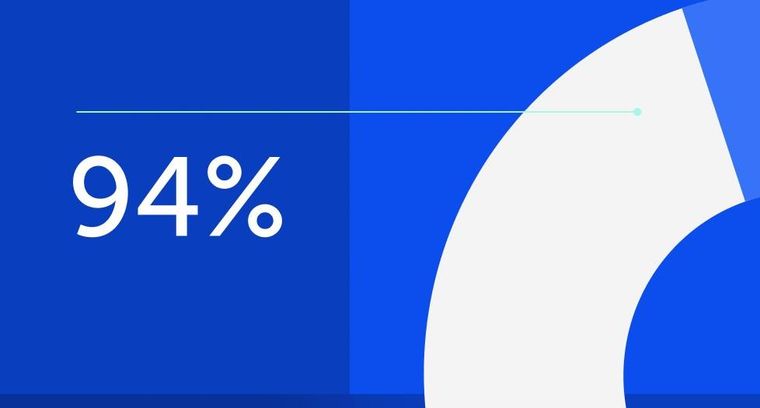
94% of researchers rate our articles as excellent or good
Learn more about the work of our research integrity team to safeguard the quality of each article we publish.
Find out more
REVIEW article
Front. Oral. Health, 14 March 2024
Sec. Oral Cancers
Volume 5 - 2024 | https://doi.org/10.3389/froh.2024.1360340
Oral squamous cell carcinoma (OSCC) is the most common type of head and neck cancer, with a high mortality rate. There is growing evidence supporting a link between oral cancer and the microbiome. The microbiome can impact various aspects of cancer, such as pathogenesis, diagnosis, treatment, and prognosis. While there is existing information on bacteria and its connection to oral cancer, the fungi residing in the oral cavity represent a significant component of the microbiome that remains in its early stages of exploration and understanding. Fungi comprise a minuscule part of the human microbiome called the mycobiome. Mycobiome is ubiquitous in the human body but a weakened immune system offers a leeway space for fungi to showcase its virulence. The role of mycobiome as a colonizer, facilitator, or driver of carcinogenesis is still ambiguous. Reactivating the mycobiome that undergoes collateral damage associated with cancer treatment can be watershed event in cancer research. The coordinated, virulent, non-virulent behavior of the fungi once they reach a critical density must be hacked, considering its diagnostic, prognostic and therapeutic implications in cancer. This review highlights the diversity of the mycobiome and its potential role in oral cancer.
Oral squamous cell carcinoma (OSCC) is a well-recognized malignant neoplasm, responsible for more than 90% of malignancies of the head and neck region (1). The main factors that contribute to the development of OSCC are alcohol and tobacco (2). Oral potentially malignant disorders (OPMD) are a group of heterogeneous lesions associated with the risk of transformation into OSCC (3). Malignant transformation across all OPMD groups is about 8% and it is generally related to genetic, geographic, and lifestyle factors (4).
Several studies using animal models have indicated a potential causal relationship between the microbiome and the development of cancer. These findings have demonstrated that the microbiome can influence cancer progression through a variety of processes, which include the production of chemical metabolites implicated in carcinogenesis, inducing DNA damage, and regulating inflammation (5). The oral cavity contains approximately 700 different species of bacteria and nurtures a diverse community of bacteria, fungi, viruses, and protozoa (6). These microorganisms have a beneficial and harmonious relationship, working together to prevent the entry and attachment of harmful pathogens in the oral cavity. Dysbiosis, an imbalance in the microbial community, disrupts the control of pathogenic microorganisms and leads to an abnormal inflammatory or immune response against commensals. Dysbiosis has been proposed as a significant factor in the development of cancer, contributing to tissue changes associated with the disease. It has also been identified as a potential “hallmark of cancer” (5, 7).
When one microbial community is knocked out of the body (bacteria), another (fungi) takes a lead, can flourish and cause illness. If the communities are undisturbed, the fungal inhabitants appear to be harmless or perhaps even beneficial. Tumors are areas where the immune system has no access, thus fungi can naturally grow and flourish expanding its territory.
In the present article, we provide a comprehensive overview of the relationship between the oral mycobiome and oral cancer. The prevailing evidence on the involvement of fungi in oral cancer and their impending ability to facilitate, initiate, or promote the disease through different mechanisms is discussed.
OPMD, such as leukoplakia and erythroplakia, have a risk of progressing to OSCC. They may present a variety of characteristics, such as a change in the color of the mucosa (i.e., white, red, or a mixture of white and red), change in size, change in morphology (i.e., smooth, corrugated, granular, verrucous, atrophic, and/or plaque) (3). Epithelial dysplasia in OPMD may be contingent upon architectural changes, exhibiting minimal or no cytological abnormalities. Moreover, while definitive features may be elusive, the amalgamation of molecular, clinical, and microscopic characteristics contributes to an increased risk of developing an OSCC (8).
OSCC stands as a prominent malignancy in the head and neck region, originating specifically from the lips, oral cavity, and oropharynx (9). The worldwide prevalence of OSCC has been escalating, notably in regions such as Southeast and South Asia, Western and Eastern Europe, the Pacific, the Caribbean, and Latin America (10, 11). Oral and oropharyngeal cancer pose a significant global health challenge, with an annual incidence of 476,000 cases and an unfortunate mortality rate of approximately half of those affected (12). In 2023, it was projected that there would be 54,540 new cases of oral and oropharyngeal cancer in the United States (13). According to GLOBOCAN 2020, India holds the third position worldwide in terms of cancer incidence, and projections indicate a substantial increase to 2.08 million cases by 2040, representing a 57% rise from 2020 (12). In India, the oral cavity and pharynx are potential sites for cancer, accounting for a burden of 198,438 cases (14).
Alcohol and tobacco are some of the risk factors for OSCC (9). Tobacco impairs oral immunity, favoring gingivitis/periodontitis and oral cancer (15). Porphyromonas gingivalis and Fusobacterium nucleatum are widely studied bacteria known for their association with the onset of gingivitis and periodontitis. Additionally, these bacteria have been identified as contributors to carcinogenesis in mice (16). Furthermore, P. gingivalis has been suggested to be involved in oral-digestive cancer (17). Alcohol, specifically ethanol, serves as both a local and systemic risk factor, increasing the permeability of the oral mucosa, causing the dissolution of epithelial lipids, inducing epithelial atrophy, and disrupting DNA synthesis and repair. Chronic alcohol use exerts genotoxic and mutagenic effects, along with a potential decrease in both innate and acquired immunity (18). Human papillomavirus (HPV) and ultraviolet (UV) exposure represent additional risk factors for oral cancer (19, 20), accounting for 2%–8% of OSCC (21).
The term “fungi” encompasses a diverse array of eukaryotic microbes that collectively constitute the human microbiota, referred to as “mycobiota”, coexisting harmoniously in virtually any anatomical location (22). Within the oral cavity, more than 75 genera, such as Candida, Cladosporium, Aureobasidium, and Aspergillus, contribute to this fungal community (23). Recent metagenomics studies have unveiled previously unidentified fungal species. Ghannoum et al. (23), in their molecular profiling study, identified a total of 85 species in the oral cavities of 20 healthy individuals, encompassing 11 non-culturable and 74 culturable fungi. Another study identified the cultivation of 101 different genera of fungi, with the number of species per individual ranging from nine to 23. Furthermore, using sequencing techniques, Malassezia sp. was identified as an additional pathogenic species commonly inhabiting the oral cavity (24). Similarly, a systematic review has documented the presence of Candida spp. and Malassezia spp. on mucous membranes, further emphasizing the diverse fungal composition within the oral environment (25).
The oral mycobiota exhibits remarkable diversity, primarily composed of organisms affiliated with the phylum Ascomycota, with Candida species standing out as a particularly prominent group. Culture-independent methods have unveiled an expanded spectrum that encompasses numerous general within the Saccharomycetaceae family. Notable members of this diverse group include, but not limited to, C. albicans, Fusarium species, Pichia species, C. dubliniensis, Saccharomyces cerevisiae, C. rugose, and Hanseniaspora uvarum. Collectively, these species represent the majority of fungi populating the oral cavity (26) (Table 1).
Fungi exhibit the characteristic ability to enhance cell density and promote the growth of hyphae, forming the structural framework for multispecies biofilms. Moreover, fungi stand out among eukaryotes due to their potent impact on the host immune system, exerting diverse immunological effects (32). Interactions between fungi and the host underscore the presence of a robust host immune mechanism (22, 32–34). In the realm of microbial ecology, fungi have been proposed as “keystone species”, playing a pivotal role in influencing the overall microbiota (35, 36).
Candida is known for its cross-kingdom relationships, its near-ubiquitous nature, and ease of cultivation (35, 36). Observations indicate that C. albicans is frequently detected species among individuals with OPMD and OSCC (37, 38). In a recent study assessing 100 patients with OSCC using biochemical methods, Candida species were identified in 74% of the samples, with C. albicans being the predominant species in 84% (38). Similarly, another study reported elevated levels of C. albicans in the saliva of individuals with head and neck squamous cell carcinoma (39). A study using molecular profiling techniques investigated the co-occurrence network of C. albicans in the oral rinse of cancer patients (40). The findings revealed variations in the prevalence of certain subtypes of C. albicans, with some exhibiting higher prevalence and others lower (40). In contrast, oral rinse samples from individuals without cancer showed a higher prevalence of C. dubliniensis, Schizophyllum commune, and organisms belonging to the Agaricomycetes class (40) (Table 2).
The progression of OSCC is marked by a significant increase in the presence of Acremonium and Aspergillus, both known for their detrimental health effects (52, 53). Conversely, Morchella, recognized for its potent inhibitory activity against harmful bacteria, experiences a noteworthy reduction in reduction in individuals with premalignant and malignant conditions. The polysaccharide FMP-1 derived from Morchella esculenta is attributed with prebiotic benefits and potential anti-tumor activity owing to its antioxidant capabilities (54, 55). The decline in Morchella levels suggests the potential emergence of invasive oral niches, heightening the risk of oral disorders. These findings offer a compelling perspective on the prevention and treatment of OSCC, underscoring the crucial roles played by distinct fungal species, including Aspergillus fumigatus, C. tropicalis, and Acremonium exuviarum, all labelled as “oncogenic fungi” (56). The mitochondrial toxicity of acrebol produced by Acremonium exuviarum (57), the ability of A. fumigatus to generate reactive oxygen species linked to oral cancer (58, 59), and the collaborative biofilm formation of C. tropicalis with bacteria such as Escherichia coli and Serratia marcescens (60) exemplify the significant health consequences demonstrated by these fungi.
According to Banerjee et al. (61), Rhodotorula, Geotrichum, and Pneumocystis were exclusively found in specimens collected from cancer patients, while Fonsecaea was absent in oral tissues from healthy participants. Intriguingly, Fonsecaea was present in cancer samples and tissues adjacent to malignancy from the same patients. Another study revealed that the only fungal species in tumor samples experiencing a significant decrease in matching non-tumor tissues was Glomeromycota. Notably, high T-stage tumor samples exhibited a higher fungal population compared to low T-stage samples (62). An important observation from the study by Perera et al. (63) is the overgrowth of C. albicans in OSCC tissue.
It is well documented that candidiasis triggers type 3 responses initiated by activated IL-17-secreting cells These responses have been linked to tumor-promoting infiltrates of inflammatory cells and pose an antagonistic action to interferon-gamma (IFN-γ), a recognized anti-cancer defense mechanism (64). Conversely, candidalysin, a toxic epithelium-damaging substance produced by Candida, has been postulated to enhance tumor-promoting immunity (65–67). Despite this, the direct link between candidalysin and oral carcinogenesis remains unestablished. This recently identified Candida virulence factor has been demonstrated to activate additional oncogenic pathways within epithelial elements, involving the epidermal growth factor receptor/mitogen-activated protein kinase axis (42, 68, 69).
In a study on pancreatic cancer, the mannose-binding lectin, which binds specifically to the glycans on the fungal wall, was activated mainly by the Malassezia genus, showing potential for tumor formation (70). On the other hand, the presence of Malassezia sp. in OSCC is associated with a favorable prognosis (71).
While the oral mycobiome has demonstrated carcinogenic implications, substantial proof is currently lacking to establish whether these findings can be replicated for oral epithelial dysplasia. An alternative explanation posits that while these occurrences do exist in oral cancer growth, the associated changes may be insufficient to solely induce dysplastic alterations in oral epithelial tissue, serving more as accelerators rather that primary causes (72).
To mimic experimental oral carcinogenesis development, 4-nitrioquinolone-1-oxide (4NQO) has been used. This compound induces mutagenic changes in DNA similar to those triggered by tobacco (73). Live C. albicans has been found to enhance the migration potential, induce matrix metalloproteinase, and release associated metabolites via epithelial-mesenchymal shift, along with the upregulation of metastasis-associated genes (74). The same study explored the capacity of Candida to facilitate sequential cancer in 4NQO mice (74). These pre-neoplastic growths are supported by dysbiosis in the oral mycobiota, allowing them to progress into cancer (75). Antifungal therapy alone proves inadequate in treating the affected area and addressing this complex process; hence, the lesion should be treated as an OPMD (76).
Data from an exploratory study indicated that OSCC patients with Candida may experience lesser overall survival, serving as indirect proof for the potential tumor-promoting actions of mycobiome dysbiosis (71). In a recent metaproteomic analysis, a total of 196 fungal proteins demonstrated significant changes in abundance across different forms of OSCC. Most of the detected fungi had not been thoroughly investigated, and researchers were unaware of their toxicity or lethal associations in the oral cavity of humans (50). However, other fungi, including Verruconis gallopava (77), Dimargaris cristalligena (78, 79), and Syncephalastrum racemosum (80), were found to be more frequently present in OSCC lesions and linked to opportunistic infections.
Additionally, other well-known fungi species such as Paracoccidioides brasiliensis, Malassezia sympodialis, and Lichteimia corymbifera were detected. In an investigation comparing tissue from tumors with non-tumor controls, L. corymbifera showed an association with OSCC of the tongue (81). P. brasiliensis, a fungal yeast causing paracoccidioidomycosis leading to oral manifestations, has been associated with OSCC (82). Coexistence of oral paracoccidioidomycosis and OSCC has been reported (83), suggesting a potential role of fungi in cancer development, possibly due to continuous stimulation of epithelial cells increasing susceptibility to malignant transformation (84). Nonetheless, this association is not confirmed due to the limited number of experimental and clinical studies investigating this effect.
The ability of C. albicans to form alliances with F. nucleatum, circumventing host defenses (85), and its interaction with Streptococcus mutans (86, 87), or S. oralis (88, 89) to exacerbate oral candidiasis or dental caries, respectively, underscores the importance of inter-kingdom associations in the development of polymicrobial conditions widely recognized today (72). Some authors propose that, as new research methods advance, the relationship between C. albicans and bacteria may emerge as a model for understanding fungal-bacterial interactions in the oral cavity (72) (Figure 1).
Figure 1. Model of fungal-bacteria relationship. (A) In contrast to when each species is grown independently, Streptococcus oralis and Candida albicans grow to a greater number when the two are combined in a biofilm. (B) The growth of glucan is one of the factors contributing to the virulence of S. mutans that is facilitated by the inclusion of C. albicans. C. albicans and S. mutans progress more densely than when cultured alone. (C) Growing C. albicans in combination with Fusobacterium nucleatum considerably reduces its capacity to produce hyphae.
Soluble factors released by biofilms of C. albicans and S. aureus have been shown to induce variations in the expression of cell cycle genes and proto-oncogenes in both malignant and normal oral epithelial cell lines. This reveals how biofilm metabolites can influence gene expression and tumor cell survival based on cell-specific characteristics (90). In a study by Bertolini et al. (91) using animal models to target Enterococcus faecalis with antibiotics, the post-chemotherapy pathological effects of C. albicans infection on oral microorganisms were examined, uncovering the role of bacterial community changes in influencing the virulence of C. albicans in oropharyngeal candidiasis. Interactions among polymicrobial communities, particularly biofilm formation involving C. albicans, Actinomyces naeslundii, and S. mutans, were found to significantly enhance the adhesion of OSCC cells to extracellular matrix (ECM) and elevate the release of pro-inflammatory cytokines (92).
Several inflammatory diseases including ulcerative colitis, inflammatory bowel disease, and pancreatitis have been linked to cancer due to the inflammatory response elicited (93). The robust immune response elicited by fungal infection raises the possibility of its involvement, increasing the risk of oral cancer (22). Pattern recognition receptors (PRR) recognize pathogen-associated molecular patterns (PAMP) composed of carbohydrates in the fungal cell wall during fungal invasion, which initiates signaling cascades across multiple pathways such as MYD88 (myeloid differentiation primary response gene 88), SYK-CARD9 (spleen tyrosine kinase-caspase recruitment domain 9), and TRIF (Toll/IL-1R domain-containing adaptor-inducing interferon) pathways. Following the activation of these pathways numerous signaling molecules are secreted, such as interleukin (IL)-1, IL-6, IL-12, IL-23, transforming growth factor (TGF), and interferon (IFN). These signaling molecules regulate the antifungal response of T helper cell 1 (Th1) and T helper cell 17 (Th17) along with phagocyte stimulation and neutrophil recruitment (94) (Figure 2).
Figure 2. Mechanism by which fungi aid in cancer development. Pathogen-associated molecular patterns (PAMP), biofilm formation, and fungal metabolites such as acetaldehyde are the three major components involved in the development of cancer.
Microbial biofilms are significant in a wide range of medical disorders (95–97). Existing research indicates that bacterial and fungal biofilms are equally significant. Few studies have highlighted that fungus and bacteria can work together to create biofilms that aggravate inflammation (98). It has been established that the disease-causing bacterial agent Fusobacterium nucleatum, which resides in the oral cavity and digestive system, plays a role in the emergence of colorectal cancer (99). Moreover, it has been shown that F. nucleatum and C. albicans co-aggregate by interacting between genetic and morphological cellular elements (100).
Mukherjee et al. (62) performed inter-kingdom analysis for fungi and bacteria in 39 samples which included oral tongue cancer and non-tumor samples. Lichtheimia, a fungal species, has demonstrated a constructive relationship with bacterial species such as Campylobacter, Porphyromonas, and Fusobacterium. Earlier studies have highlighted the role of gut bacteria, especially F. nucleatum, in the development of colorectal cancer (101). This type of cancer exhibits molecular gene silencing through CpG island methylation that has been associated with F. nucleatum (102).
Few studies have shown that the alcohol dehydrogenase enzyme found in different Candida species enables them to produce acetaldehyde (103–105) that is highly toxic, mutagenic, and carcinogenic (106). For example, Asian subjects lacking aldehyde dehydrogenase-2, an enzyme that aids in the body's elimination of the compound by oxidizing it to acetate, have higher salivary acetaldehyde levels and a 10-fold greater chance of oral cancer (107).
The mutagenic properties of the metabolic product of Candida alcohol dehydrogenase enzyme, i.e., acetaldehyde raise its possible implication in carcinogenesis (64). Candida has been demonstrated to exhibit higher metabolic rates and acetaldehyde synthesis in oral cancer compared to healthy controls (108). Moreover, Candida has pronounced nitrosation potential due to the production of nitrosamine compounds that have a significant role in oral carcinogenesis (75).
Mitochondrial aldehyde dehydrogenase-2 is beneficial in eliminating acetaldehyde. However, dysbiosis with an increase in Candida may cause an elevated level in its production, especially in frequent drinkers (109, 110) (Figure 3).
Figure 3. Acetaldehyde can be involved in the oral carcinogenesis through a variety of mechanisms. In addition to altering the framework and functionality of proteins and DNA by binding, this compound also reduces glutathione's antioxidant activity, which raises the amounts of reactive oxygen species (ROS).
Cancer occurs when normal cells in the body expand, and divide exponentially. Certain immune cells are essential for tracing and destroying these tumor cells. This immune response is influenced, at least in part, by the microbiota. Immune cells have sensors that may identify specific microorganisms, triggering various reactions. A crucial component in the body's capacity to recognize fungi is a protein called dectin-1. It is found on the surface of some immune cells and functions as a transmembrane pattern-recognition receptor through its ability to bind β-glucan carbohydrates (111). It has been discovered to be associated with tumor growth and survival rates in both mouse and human breast tumors (112) and melanomas (54, 113). The effectiveness of cancer treatments, particularly radiotherapy, and its impact on the immune system, gut microbiota, and the other collateral damage it induces on the body may have a causal link with an overgrowth of specific fungi (113). This further emphasizes the potential relevance of the combination of standard chemotherapy or radiotherapy with therapies that regulate the oral microbiota.
The discovery of a distinct fungal pattern that points to the involvement of fungal communities in tumor development highlights the need to attain traction and stretch the scope of microbiome research beyond bacterial communities. Earlier studies have illuminated the interplay between distinct microbial communities, implying that these joint communities contribute to dysbiosis or the preservation of a healthy microbiome (72). While there is a range of data on the role of Candida in oral carcinogenesis (37, 41, 114), other species such as Aspergillus, Penicillium, and Malassezzia require additional investigation (Figure 4). Overall, the study of the oral mycobiome is still in its infancy, and further research aiming at exploring the potential role of fungi in tuning and modulating the host immunity is essential to open up new possibilities for the prevention and adjunctive treatment of oral cancer.
Figure 4. Role of fungi, bacteria and virus in carcinogenic process. Representation of the mechanisms involved in cancer progression by microorganisms through a Venn diagram. Fungi and bacteria produce metabolites like acetaldehyde and nitrosamine, induce inflammatory response through T helper cells. Fungi and virus mimic the molecular structure similar to that of the host thereby activating the immune system. Fungi, bacteria and virus all have the common mechanism of causing inflammation, cell alteration and metastasis which ultimately contributes to the development of cancer.
The microbial communities, driven by their survival instincts within the host, employ cooperative evolutionary strategies that lead to the formation of robust biofilms. Fungi enhance their virulence through filamentation and increased secretion of aspartyl proteinases, strengthening their ability to invade the host (115). Conversely, bacteria develop antibacterial tolerance while residing under the protective fungal matrix umbrella. C. albicans, for instance, produces mucolytic enzymes that degrade the protective mucin layer of the epithelium, contributing to the formation of lesions, tumors, and other pathological conditions. This interkingdom cooperation significantly influences the host immune system (116).
The oral microbiome, comprising a diverse array of fungi, bacteria, and viruses living in biofilms, can pose a threat to immunocompromised oral cancer patients. While guidelines and protocols exist to control oral infections, they often rely more on clinical observations than robust evidence. Increased microbial load, especially in cancer patients, can be potentially fatal. Thus, maintaining good oral health and seeking regular treatment from oral health professionals is crucial. Although some components of the oral mycobiome can metabolize substances into carcinogens, their exact role in the development of oral cancer remains uncertain. For example, the involvement of mycobiota in alcohol metabolism can lead to the formation of acetaldehyde, a known carcinogen. The combination of poor oral hygiene, inadequate nutrition, and established risk factors such as alcohol and tobacco can amplify the risk of developing oral cancer.
The application of novel molecular methods is enabling the identification and understanding of the mycobiome's role in both healthy individuals and those immunosuppressed individuals. The inter-kingdom relationships between fungi and bacteria add another layer of complexity to this exploration. The detection of newly identified proteins closely associated with fungal overload allows for the assessment cancer treatment effectiveness and exploration of novel approaches. Thus, it is important to include the mycobiome—the concealed realm of potential biohackers—in research efforts. Decoding its molecular signature and evaluating its connection to oral cancer can have significant implications in prevention, diagnosis, and treatment, opening new horizons in cancer research.
JM: Writing – original draft, Writing – review & editing. KK: Writing – original draft, Writing – review & editing. JA: Writing – original draft, Writing – review & editing. EG: Writing – original draft, Writing – review & editing. AV: Writing – review & editing. TS: Writing – review & editing. DD: Writing – review & editing. CA: Writing – review & editing. SS: Writing – original draft, Writing – review & editing.
The author(s) declare that no financial support was received for the research, authorship, and/or publication of this article.
The authors declare that the research was conducted in the absence of any commercial or financial relationships that could be construed as a potential conflict of interest.
The author(s) declared that they were an editorial board member of Frontiers, at the time of submission. This had no impact on the peer review process and the final decision.
All claims expressed in this article are solely those of the authors and do not necessarily represent those of their affiliated organizations, or those of the publisher, the editors and the reviewers. Any product that may be evaluated in this article, or claim that may be made by its manufacturer, is not guaranteed or endorsed by the publisher.
1. Colevas AD, Yom SS, Pfister DG, Spencer S, Adelstein D, Adkins D, et al. NCCN guidelines insights: head and neck cancers, version 1.2018. J Natl Compr Canc Netw. (2018) 16(5):479–90. doi: 10.6004/jnccn.2018.0026
2. Dissanayaka WL, Pitiyage G, Kumarasiri PV, Liyanage RL, Dias KD, Tilakaratne WM. Clinical and histopathologic parameters in survival of oral squamous cell carcinoma. Oral Surg Oral Med Oral Pathol Oral Radiol. (2012) 113(4):518–25. doi: 10.1016/j.oooo.2011.11.001
3. Warnakulasuriya S, Kujan O, Aguirre-Urizar JM, Bagan JV, González-Moles MÁ, Kerr AR, et al. Oral potentially malignant disorders: a consensus report from an international seminar on nomenclature and classification, convened by the WHO collaborating centre for oral cancer. Oral Dis. (2021) 27(8):1862–80. doi: 10.1111/odi.13704
4. Iocca O, Sollecito TP, Alawi F, Weinstein GS, Newman JG, De Virgilio A, et al. Potentially malignant disorders of the oral cavity and oral dysplasia: a systematic review and meta-analysis of malignant transformation rate by subtype. Head Neck. (2020) 42(3):539–55. doi: 10.1002/hed.26006
5. Bhatt AP, Redinbo MR, Bultman SJ. The role of the microbiome in cancer development and therapy. CA Cancer J Clin. (2017) 67(4):326–44. doi: 10.3322/caac.21398
6. Deo PN, Deshmukh R. Oral microbiome: unveiling the fundamentals. J Oral Maxillofac Pathol. (2019) 23(1):122–8. doi: 10.4103/jomfp.JOMFP_304_18
7. Avila M, Ojcius DM, Yilmaz O. The oral microbiota: living with a permanent guest. DNA Cell Biol. (2009) 28(8):405–11. doi: 10.1089/dna.2009.0874
8. Odell E, Kujan O, Warnakulasuriya S, Sloan P. Oral epithelial dysplasia: recognition, grading and clinical significance. Oral Dis. (2021) 27(8):1947–76. doi: 10.1111/odi.13993
9. Huang J, Chan SC, Ko S, Lok V, Zhang L, Lin X, et al. Disease burden, risk factors, and trends of lip, oral cavity, pharyngeal cancers: a global analysis. Cancer Med. (2023) 12(17):18153–64. doi: 10.1002/cam4.6391
10. Warnakulasuriya S. Global epidemiology of oral and oropharyngeal cancer. Oral Oncol. (2009) 45(4–5):309–16. doi: 10.1016/j.oraloncology.2008.06.002
11. Miranda-Filho A, Bray F. Global patterns and trends in cancers of the lip, tongue and mouth. Oral Oncol. (2020) 102:104551. doi: 10.1016/j.oraloncology.2019.104551
12. Sung H, Ferlay J, Siegel RL, Laversanne M, Soerjomataram I, Jemal A, et al. Global cancer statistics 2020: GLOBOCAN estimates of incidence and mortality worldwide for 36 cancers in 185 countries. CA Cancer J Clin. (2021) 71(3):209–49. doi: 10.3322/caac.21660
13. Siegel RL, Miller KD, Wagle NS, Jemal A. Cancer statistics, 2023. CA Cancer J Clin. (2023) 73(1):17–48. doi: 10.3322/caac.21763
14. Sathishkumar K, Chaturvedi M, Das P, Stephen S, Mathur P. Cancer incidence estimates for 2022 & projection for 2025: result from national cancer registry programme, India. Indian J Med Res. (2022) 156(4&5):598–607. doi: 10.4103/ijmr.ijmr_1821_22
15. Lee J, Taneja V, Vassallo R. Cigarette smoking and inflammation: cellular and molecular mechanisms. J Dent Res. (2012) 91(2):142–9. doi: 10.1177/0022034511421200
16. Binder Gallimidi A, Fischman S, Revach B, Bulvik R, Maliutina A, Rubinstein AM, et al. Periodontal pathogens porphyromonas gingivalis and fusobacterium nucleatum promote tumor progression in an oral-specific chemical carcinogenesis model. Oncotarget. (2015) 6(26):22613–23. doi: 10.18632/oncotarget.4209
17. Irfan M, Delgado RZR, Frias-Lopez J. The oral microbiome and cancer. Front Immunol. (2020) 11:591088. doi: 10.3389/fimmu.2020.591088
18. Reidy J, McHugh E, Stassen LF. A review of the relationship between alcohol and oral cancer. Surgeon. (2011) 9(5):278–83. doi: 10.1016/j.surge.2011.01.010
19. Chaitanya NC, Allam NS, Gandhi Babu DB, Waghray S, Badam RK, Lavanya R. Systematic meta-analysis on association of human papilloma virus and oral cancer. J Cancer Res Ther. (2016) 12(2):969–74. doi: 10.4103/0973-1482.179098
20. Adams S, Lin J, Brown D, Shriver CD, Zhu K. Ultraviolet radiation exposure and the incidence of oral, pharyngeal and cervical cancer and melanoma: an analysis of the SEER data. Anticancer Res. (2016) 36(1):233–7.26722048
21. Hübbers CU, Akgül B. HPV and cancer of the oral cavity. Virulence. (2015) 6(3):244–8. doi: 10.1080/21505594.2014.999570
22. Underhill DM, Iliev ID. The mycobiota: interactions between commensal fungi and the host immune system. Nat Rev Immunol. (2014) 14(6):405–16. doi: 10.1038/nri3684
23. Ghannoum MA, Jurevic RJ, Mukherjee PK, Cui F, Sikaroodi M, Naqvi A, et al. Characterization of the oral fungal microbiome (mycobiome) in healthy individuals. PLoS Pathog. (2010) 6(1):e1000713. doi: 10.1371/journal.ppat.1000713
24. Dupuy AK, David MS, Li L, Heider TN, Peterson JD, Montano EA, et al. Redefining the human oral mycobiome with improved practices in amplicon-based taxonomy: discovery of malassezia as a prominent commensal. PLoS One. (2014) 9(3):e90899. doi: 10.1371/journal.pone.0090899
25. Huffnagle GB, Noverr MC. The emerging world of the fungal microbiome. Trends Microbiol. (2013) 21(7):334–41. doi: 10.1016/j.tim.2013.04.002
26. Mukherjee PK, Chandra J, Retuerto M, Sikaroodi M, Brown RE, Jurevic R, et al. Oral mycobiome analysis of HIV-infected patients: identification of pichia as an antagonist of opportunistic fungi. PLoS Pathog. (2014) 10(3):e1003996. doi: 10.1371/journal.ppat.1003996
27. Urzúa B, Hermosilla G, Gamonal J, Morales-Bozo I, Canals M, Barahona S, et al. Yeast diversity in the oral microbiota of subjects with periodontitis: Candida albicans and Candida dubliniensis colonize the periodontal pockets. Med Mycol. (2008) 46(8):783–93. doi: 10.1080/13693780802060899
28. Monteiro-da-Silva F, Araujo R, Sampaio-Maia B. Interindividual variability and intraindividual stability of oral fungal microbiota over time. Med Mycol. (2014) 52(5):498–505. doi: 10.1093/mmy/myu027
29. Peters BA, Wu J, Hayes RB, Ahn J. The oral fungal mycobiome: characteristics and relation to periodontitis in a pilot study. BMC Microbiol. (2017) 17:157. doi: 10.1186/s12866-017-1064-9
30. Baraniya D, Chen T, Nahar A, Alakwaa F, Hill J, Tellez M, et al. Supragingival mycobiome and inter-kingdom interactions in dental caries. J Oral Microbiol. (2020) 12(1):1729305. doi: 10.1080/20002297.2020.1729305
31. Khadija B, Imran M, Faryal R. Keystone salivary mycobiome in postpartum period in health and disease conditions. J Mycol Med. (2021) 31(1):101101. doi: 10.1016/j.mycmed.2020.101101
32. Cui L, Morris A, Ghedin E. The human mycobiome in health and disease. Genome Med. (2013) 5(7):63. doi: 10.1186/gm467
33. Rizzetto L, De Filippo C, Cavalieri D. Richness and diversity of mammalian fungal communities shape innate and adaptive immunity in health and disease. Eur J Immunol. (2014) 44(11):3166–81. doi: 10.1002/eji.201344403
34. Oever JT, Netea MG. The bacteriome-mycobiome interaction and antifungal host defense. Eur J Immunol. (2014) 44(11):3182–91. doi: 10.1002/eji.201344405
35. Janus MM, Willems HM, Krom BP. Candida albicans in multispecies oral communities; a keystone commensal? Adv Exp Med Biol. (2016) 931:13–20. doi: 10.1007/5584_2016_5
36. Diaz PI, Strausbaugh LD, Dongari-Bagtzoglou A. Fungal-bacterial interactions and their relevance to oral health: linking the clinic and the bench. Front Cell Infect Microbiol. (2014) 4:101. doi: 10.3389/fcimb.2014.00101
37. Arya CP, Jaiswal R, Tandon A, Jain A. Isolation and identification of oral Candida species in potentially malignant disorder and oral squamous cell carcinoma. Natl J Maxillofac Surg. (2021) 12(3):387–91. doi: 10.4103/njms.NJMS_80_19
38. Mäkinen A, Nawaz A, Mäkitie A, Meurman JH. Role of non-albicans candida and Candida albicans in oral squamous cell cancer patients. J Oral Maxillofac Surg. (2018) 76(12):2564–71. doi: 10.1016/j.joms.2018.06.012
39. Vesty A, Gear K, Biswas K, Radcliff FJ, Taylor MW, Douglas RG. Microbial and inflammatory-based salivary biomarkers of head and neck squamous cell carcinoma. Clin Exp Dent Res. (2018) 4(6):255–62. doi: 10.1002/cre2.139
40. Shay E, Sangwan N, Padmanabhan R, Lundy S, Burkey B, Eng C. Bacteriome and mycobiome and bacteriome-mycobiome interactions in head and neck squamous cell carcinoma. Oncotarget. (2020) 11(25):2375–86. doi: 10.18632/oncotarget.27629
41. Ayuningtyas NF, Mahdani FY, Pasaribu TAS, Chalim M, Ayna VKP, Santosh ABR, et al. Role of Candida albicans in oral carcinogenesis. Pathophysiology. (2022) 29(4):650–62. doi: 10.3390/pathophysiology29040051
42. Theofilou VI, Alfaifi A, Montelongo-Jauregui D, Pettas E, Georgaki M, Nikitakis NG, et al. The oral mycobiome: oral epithelial dysplasia and oral squamous cell carcinoma. J Oral Pathol Med. (2022) 51(5):413–20. doi: 10.1111/jop.13295
43. İlhan B, Vural C, Gürhan C, Vural C, Veral A, Wilder-Smith P, et al. Real-time PCR detection of Candida species in biopsy samples from non-smokers with oral dysplasia and oral squamous cell cancer: a retrospective archive study. Cancers (Basel). (2023) 15(21):5251. doi: 10.3390/cancers15215251
44. Murugan K, Padmavathy K, Aswath N, Mallika L. Taciturn role of oral microbiome in oral squamous cell carcinoma: the hidden truth—a systematic review. J Indian Acad Oral Med Radiol. (2023) 35(4):599–604. doi: 10.4103/jiaomr.jiaomr_306_22
45. Fan Y, Wu L, Zhai B. The mycobiome: interactions with host and implications in diseases. Curr Opin Microbiol. (2023) 75:102361. doi: 10.1016/j.mib.2023.102361
46. Tasso CO, Ferrisse TM, de Oliveira AB, Ribas BR, Jorge JH. Candida species as potential risk factors for oral squamous cell carcinoma: systematic review and meta-analysis. Cancer Epidemiol. (2023) 86:102451. doi: 10.1016/j.canep.2023.102451
47. Talapko J, Meštrović T, Dmitrović B, Juzbašić M, Matijević T, Bekić S, et al. A putative role of Candida albicans in promoting cancer development: a current state of evidence and proposed mechanisms. Microorganisms. (2023) 11(6):1476. doi: 10.3390/microorganisms11061476
48. Wang X, Wu S, Wu W, Zhang W, Li L, Liu Q, et al. Candida albicans promotes oral cancer via IL-17A/IL-17RA-macrophage axis. mBio. (2023) 14(3):e0044723. doi: 10.1128/mbio.00447-23
49. Wang X, Zhang W, Wu W, Wu S, Young A, Yan Z. Is Candida albicans a contributor to cancer? A critical review based on the current evidence. Microbiol Res. (2023) 272:127370. doi: 10.1016/j.micres.2023.127370
50. He S, Chakraborty R, Ranganathan S. Metaproteomic analysis of an oral squamous cell carcinoma dataset suggests diagnostic potential of the mycobiome. Int J Mol Sci. (2023) 24(2):1050. doi: 10.3390/ijms24021050
51. Wang X, Wu S, Li L, Yan Z. Candida albicans overgrowth disrupts the gut microbiota in mice bearing oral cancer. Mycology. (2023) 1:1–3. doi: 10.1080/21501203.2023.2256761
52. Anis A, Sameeullah F, Bhatti JM. A rare case of brain abscesses caused by acremonium species. Cureus. (2021) 13(4):e14396. doi: 10.7759/cureus.14396
53. van de Veerdonk FL, Gresnigt MS, Romani L, Netea MG, Latgé JP. Aspergillus fumigatus morphology and dynamic host interactions. Nat Rev Microbiol. (2017) 15(11):661–74. doi: 10.1038/nrmicro.2017.90
54. Liu C, Sun Y, Mao Q, Guo X, Li P, Liu Y, et al. Characteristics and antitumor activity of morchella esculenta polysaccharide extracted by pulsed electric field. Int J Mol Sci. (2016) 17(6):986. doi: 10.3390/ijms17060986
55. Li W, Cai ZN, Mehmood S, Wang Y, Pan WJ, Zhang WN, et al. Polysaccharide FMP-1 from morchella esculenta attenuates cellular oxidative damage in human alveolar epithelial A549 cells through PI3K/AKT/Nrf2/HO-1 pathway. Int J Biol Macromol. (2018) 120(Pt A):865–75. doi: 10.1016/j.ijbiomac.2018.08.148
56. Heng W, Wang W, Dai T, Jiang P, Lu Y, Li R, et al. Oral bacteriome and mycobiome across stages of oral carcinogenesis. Microbiol Spectr. (2022) 10(6):e0273722. doi: 10.1128/spectrum.02737-22
57. Andersson MA, Mikkola R, Raulio M, Kredics L, Maijala P, Salkinoja-Salonen MS. Acrebol, a novel toxic peptaibol produced by an acremonium exuviarum indoor isolate. J Appl Microbiol. (2009) 106(3):909–23. doi: 10.1111/j.1365-2672.2008.04062.x
58. Du J, Chi Y, Song Z, Di Q, Mai Z, Shi J, et al. Crocin reduces Aspergillus fumigatus-induced airway inflammation and NF-κB signal activation. J Cell Biochem. (2018) 119(2):1746–54. doi: 10.1002/jcb.26335
59. Bahar G, Feinmesser R, Shpitzer T, Popovtzer A, Nagler RM. Salivary analysis in oral cancer patients: DNA and protein oxidation, reactive nitrogen species, and antioxidant profile. Cancer. (2007) 109(1):54–9. doi: 10.1002/cncr.22386
60. Hoarau G, Mukherjee PK, Gower-Rousseau C, Hager C, Chandra J, Retuerto MA, et al. Bacteriome and mycobiome interactions underscore microbial dysbiosis in familial Crohn’s disease. mBio. (2016) 7(5):e01250–16. doi: 10.1128/mBio.01250-16
61. Banerjee S, Tian T, Wei Z, Peck KN, Shih N, Chalian AA, et al. Microbial signatures associated with oropharyngeal and oral squamous cell carcinomas. Sci Rep. (2017) 7(1):4036. doi: 10.1038/s41598-017-03466-6
62. Mukherjee PK, Wang H, Retuerto M, Zhang H, Burkey B, Ghannoum MA, et al. Bacteriome and mycobiome associations in oral tongue cancer. Oncotarget. (2017) 8(57):97273–89. doi: 10.18632/oncotarget.21921
63. Perera M, Al-Hebshi NN, Perera I, Ipe D, Ulett GC, Speicher DJ, et al. A dysbiotic mycobiome dominated by Candida albicans is identified within oral squamous-cell carcinomas. J Oral Microbiol. (2017) 9(1):1385369. doi: 10.1080/20002297.2017.1385369
64. Ramirez-Garcia A, Rementeria A, Aguirre-Urizar JM, Moragues MD, Antoran A, Pellon A, et al. Candida albicans and cancer: can this yeast induce cancer development or progression? Crit Rev Microbiol. (2016) 42(2):181–93. doi: 10.3109/1040841X.2014.913004
65. Verma AH, Richardson JP, Zhou C, Coleman BM, Moyes DL, Ho J, et al. Oral epithelial cells orchestrate innate type 17 responses to Candida albicans through the virulence factor candidalysin. Sci Immunol. (2017) 2(17):eaam8834. doi: 10.1126/sciimmunol.aam8834
66. Engku Nasrullah Satiman EAF, Ahmad H, Ramzi AB, Abdul Wahab R, Kaderi MA, Wan Harun WHA, et al. The role of Candida albicans candidalysin ECE1 gene in oral carcinogenesis. J Oral Pathol Med. (2020) 49(9):835–41. doi: 10.1111/jop.13014
67. Di Cosola M, Cazzolla AP, Charitos IA, Ballini A, Inchingolo F, Santacroce L. Candida albicans and oral carcinogenesis. A brief review. J Fungi (Basel). (2021) 7(6):476. doi: 10.3390/jof7060476
68. Moyes DL, Wilson D, Richardson JP, Mogavero S, Tang SX, Wernecke J, et al. Candidalysin is a fungal peptide toxin critical for mucosal infection. Nature. (2016) 532(7597):64–8. doi: 10.1038/nature17625
69. Ho J, Yang X, Nikou SA, Kichik N, Donkin A, Ponde NO, et al. Candidalysin activates innate epithelial immune responses via epidermal growth factor receptor. Nat Commun. (2019) 10(1):2297. doi: 10.1038/s41467-019-09915-2
70. Aykut B, Pushalkar S, Chen R, Li Q, Abengozar R, Kim JI, et al. The fungal mycobiome promotes pancreatic oncogenesis via activation of MBL. Nature. (2019) 574(7777):264–7. doi: 10.1038/s41586-019-1608-2
71. Mohamed N, Litlekalsøy J, Ahmed IA, Martinsen EMH, Furriol J, Javier-Lopez R, et al. Analysis of salivary mycobiome in a cohort of oral squamous cell carcinoma patients from Sudan identifies higher salivary carriage of malassezia as an independent and favorable predictor of overall survival. Front Cell Infect Microbiol. (2021) 11:673465. doi: 10.3389/fcimb.2021.673465
72. Baker JL, Bor B, Agnello M, Shi W, He X. Ecology of the oral microbiome: beyond bacteria. Trends Microbiol. (2017) 25(5):362–74. doi: 10.1016/j.tim.2016.12.012
73. Kanojia D, Vaidya MM. 4-nitroquinoline-1-oxide Induced experimental oral carcinogenesis. Oral Oncol. (2006) 42(7):655–67. doi: 10.1016/j.oraloncology.2005.10.013
74. Vadovics M, Ho J, Igaz N, Alföldi R, Rakk D, Veres É, et al. Candida albicans enhances the progression of oral squamous cell carcinoma in vitro and in vivo. mBio. (2021) 13(1):e0314421. doi: 10.1128/mBio.03144-21
75. Sanjaya PR, Gokul S, Gururaj Patil B, Raju R. Candida in oral pre-cancer and oral cancer. Med Hypotheses. (2011) 77(6):1125–8. doi: 10.1016/j.mehy.2011.09.018
76. Woo SB. Oral epithelial dysplasia and premalignancy. Head Neck Pathol. (2019) 13(3):423–39. doi: 10.1007/s12105-019-01020-6
77. Jennings Z, Kable K, Halliday CL, Nankivell BJ, Kok J, Wong G, et al. Verruconis gallopava cardiac and endovascular infection with dissemination after renal transplantation: case report and lessons learned. Med Mycol Case Rep. (2016) 15:5–8. doi: 10.1016/j.mmcr.2016.12.006
78. Jeffries P, Young TWK. Ultrastructure of the haustorial apparatus of dimargaris cristalligena. Ann Bot. (1981) 47(1):107–19. doi: 10.1093/oxfordjournals.aob.a085985
79. Kemna ME, Neri RC, Ali R, Salkin IF. Cokeromyces recurvatus, a mucoraceous zygomycete rarely isolated in clinical laboratories. J Clin Microbiol. (1994) 32(3):843–5. doi: 10.1128/jcm.32.3.843-845.1994
80. Irshad M, Nasir N, Hashmi UH, Farooqi J, Mahmood SF. Invasive pulmonary infection by syncephalastrum species: two case reports and review of literature. IDCases. (2020) 21:e00913. doi: 10.1016/j.idcr.2020.e00913
81. Kalaskar RR, Kalaskar AR, Ganvir S. Oral mucormycosis in an 18-month-old child: a rare case report with a literature review. J Korean Assoc Oral Maxillofac Surg. (2016) 42(2):105–10. doi: 10.5125/jkaoms.2016.42.2.105
82. de Arruda JAA, Schuch LF, Abreu LG, Silva LVO, Mosconi C, Monteiro JLGC, et al. A multicentre study of oral paracoccidioidomycosis: analysis of 320 cases and literature review. Oral Dis. (2018) 24(8):1492–502. doi: 10.1111/odi.12925
83. Azevedo RS, Gouvêa AF, Lopes MA, Corrêa MB, Jorge J. Synchronous oral paracoccidioidomycosis and oral squamous cell carcinomas with submandibular enlargement. Med Mycol. (2011) 49(1):84–9. doi: 10.3109/13693786.2010.496118
84. Rodrigues GdaS, Severo CB, Oliveira FdeM, Moreira JdaS, Prolla JC, Severo LC. Association between paracoccidioidomycosis and cancer. J Bras Pneumol. (2010) 36(3):356–62. (English, Portuguese). doi: 10.1590/s1806-37132010000300014
85. Bor B, Cen L, Agnello M, Shi W, He X. Morphological and physiological changes induced by contact-dependent interaction between Candida albicans and Fusobacterium nucleatum. Sci Rep. (2016) 6:27956. doi: 10.1038/srep27956
86. Falsetta ML, Klein MI, Colonne PM, Scott-Anne K, Gregoire S, Pai CH, et al. Symbiotic relationship between Streptococcus mutans and Candida albicans synergizes virulence of plaque biofilms in vivo. Infect Immun. (2014) 82(5):1968–81. doi: 10.1128/IAI.00087-14
87. Sztajer H, Szafranski SP, Tomasch J, Reck M, Nimtz M, Rohde M, et al. Cross-feeding and interkingdom communication in dual-species biofilms of Streptococcus mutans and Candida albicans. ISME J. (2014) 8(11):2256–71. doi: 10.1038/ismej.2014.73
88. Cavalcanti IM, Del Bel Cury AA, Jenkinson HF, Nobbs AH. Interactions between Streptococcus oralis, Actinomyces oris, and Candida albicans in the development of multispecies oral microbial biofilms on salivary pellicle. Mol Oral Microbiol. (2017) 32(1):60–73. doi: 10.1111/omi.12154
89. Xu H, Sobue T, Bertolini M, Thompson A, Dongari-Bagtzoglou A. Streptococcus oralis and Candida albicans synergistically activate μ-calpain to degrade E-cadherin from oral epithelial junctions. J Infect Dis. (2016) 214(6):925–34. doi: 10.1093/infdis/jiw201
90. Amaya Arbeláez MI, de Paula E Silva ACA, Navegante G, Valente V, Barbugli PA, Vergani CE. Proto-oncogenes and cell cycle gene expression in normal and neoplastic oral epithelial cells stimulated with soluble factors from single and dual biofilms of Candida albicans and Staphylococcus aureus. Front Cell Infect Microbiol. (2021) 11:627043. doi: 10.3389/fcimb.2021.627043
91. Bertolini M, Vazquez Munoz R, Archambault L, Shah S, Souza JGS, Costa RC, et al. Mucosal bacteria modulate Candida albicans virulence in oropharyngeal candidiasis. mBio. (2021) 12(4):e0193721. doi: 10.1128/mBio.01937-21
92. Arzmi MH, Dashper S, McCullough M. Polymicrobial interactions of Candida albicans and its role in oral carcinogenesis. J Oral Pathol Med. (2019) 48(7):546–51. doi: 10.1111/jop.12905
93. Greten FR, Grivennikov SI. Inflammation and cancer: triggers, mechanisms, and consequences. Immunity. (2019) 51(1):27–41. doi: 10.1016/j.immuni.2019.06.025
94. Qin X, Gu Y, Liu T, Wang C, Zhong W, Wang B, et al. Gut mycobiome: a promising target for colorectal cancer. Biochim Biophys Acta Rev Cancer. (2021) 1875(1):188489. doi: 10.1016/j.bbcan.2020.188489
95. Masters EA, Trombetta RP, de Mesy Bentley KL, Boyce BF, Gill AL, Gill SR, et al. Evolving concepts in bone infection: redefining “biofilm”, “acute vs. chronic osteomyelitis”, “the immune proteome” and “local antibiotic therapy”. Bone Res. (2019) 7:20. doi: 10.1038/s41413-019-0061-z
96. Bjarnsholt T. The role of bacterial biofilms in chronic infections. APMIS Suppl. (2013) 136:1–51. doi: 10.1111/apm.12099
97. Khatoon Z, McTiernan CD, Suuronen EJ, Mah TF, Alarcon EI. Bacterial biofilm formation on implantable devices and approaches to its treatment and prevention. Heliyon. (2018) 4(12):e01067. doi: 10.1016/j.heliyon.2018.e01067
98. Hager CL, Ghannoum MA. The mycobiome: role in health and disease, and as a potential probiotic target in gastrointestinal disease. Dig Liver Dis. (2017) 49(11):1171–6. doi: 10.1016/j.dld.2017.08.025
99. Sun CH, Li BB, Wang B, Zhao J, Zhang XY, Li TT, et al. The role of Fusobacterium nucleatum in colorectal cancer: from carcinogenesis to clinical management. Chronic Dis Transl Med. (2019) 5(3):178–87. doi: 10.1016/j.cdtm.2019.09.001
100. Wu J, Li Q, Fu X. Fusobacterium nucleatum contributes to the carcinogenesis of colorectal cancer by inducing inflammation and suppressing host immunity. Transl Oncol. (2019) 12(6):846–51. doi: 10.1016/j.tranon.2019.03.003
101. Sami A, Elimairi I, Stanton C, Ross RP, Ryan CA. The role of the microbiome in oral squamous cell carcinoma with insight into the microbiome-treatment axis. Int J Mol Sci. (2020) 21(21):8061. doi: 10.3390/ijms21218061
102. Brennan K, Koenig JL, Gentles AJ, Sunwoo JB, Gevaert O. Identification of an atypical etiological head and neck squamous carcinoma subtype featuring the CpG island methylator phenotype. EBioMedicine. (2017) 17:223–36. doi: 10.1016/j.ebiom.2017.02.025
103. Nieminen MT, Uittamo J, Salaspuro M, Rautemaa R. Acetaldehyde production from ethanol and glucose by non-Candida albicans yeasts in vitro. Oral Oncol. (2009) 45(12):e245–8. doi: 10.1016/j.oraloncology.2009.08.002
104. Uittamo J, Siikala E, Kaihovaara P, Salaspuro M, Rautemaa R. Chronic candidosis and oral cancer in APECED-patients: production of carcinogenic acetaldehyde from glucose and ethanol by Candida albicans. Int J Cancer. (2009) 124(3):754–6. doi: 10.1002/ijc.23976
105. Tillonen J, Homann N, Rautio M, Jousimies-Somer H, Salaspuro M. Role of yeasts in the salivary acetaldehyde production from ethanol among risk groups for ethanol-associated oral cavity cancer. Alcohol Clin Exp Res. (1999) 23(8):1409–15. doi: 10.1111/j.1530-0277.1999.tb04364.x
106. Miyazaki Y, Okuyama A, Hoshino M, Nishimura M, Kentaro K. Influence of acetaldehyde on oral epithelial cells. J Oral Cancer Res. (2021) 4(1):44–8. doi: 10.36959/915/577
107. Väkeväinen S, Tillonen J, Agarwal DP, Srivastava N, Salaspuro M. High salivary acetaldehyde after a moderate dose of alcohol in ALDH2-deficient subjects: strong evidence for the local carcinogenic action of acetaldehyde. Alcohol Clin Exp Res. (2000) 24(6):873–7. doi: 10.1111/j.1530-0277.2000.tb02068.x
108. Alnuaimi AD, Ramdzan AN, Wiesenfeld D, O'Brien-Simpson NM, Kolev SD, Reynolds EC, et al. Candida virulence and ethanol-derived acetaldehyde production in oral cancer and non-cancer subjects. Oral Dis. (2016) 22(8):805–14. doi: 10.1111/odi.12565
109. Salaspuro MP. Acetaldehyde, microbes, and cancer of the digestive tract. Crit Rev Clin Lab Sci. (2003) 40(2):183–208. doi: 10.1080/713609333
110. Homann N, Tillonen J, Meurman JH, Rintamäki H, Lindqvist C, Rautio M, et al. Increased salivary acetaldehyde levels in heavy drinkers and smokers: a microbiological approach to oral cavity cancer. Carcinogenesis. (2000) 21(4):663–8. doi: 10.1093/carcin/21.4.663
111. Mata-Martínez P, Bergón-Gutiérrez M, Del Fresno C. Dectin-1 signaling update: new perspectives for trained immunity. Front Immunol. (2022) 13:812148. doi: 10.3389/fimmu.2022.812148
112. Shiao SL, Ruffell B, DeNardo DG, Faddegon BA, Park CC, Coussens LM. TH2-polarized CD4(+) T cells and macrophages limit efficacy of radiotherapy. Cancer Immunol Res. (2015) 3(5):518–25. doi: 10.1158/2326-6066.CIR-14-0232
113. Shiao SL, Kershaw KM, Limon JJ, You S, Yoon J, Ko EY, et al. Commensal bacteria and fungi differentially regulate tumor responses to radiation therapy. Cancer Cell. (2021) 39(9):1202–1213.e6. doi: 10.1016/j.ccell.2021.07.002
114. Yang Z, Zhang S, Ji N, Li J, Chen Q. The evil companion of OSCC: candida albicans. Oral Dis. (2023):1–14. doi: 10.1111/odi.14700
115. Arita GS, Faria DR, Capoci IR, Kioshima ES, Bonfim-Mendonça PS, Svidzinski TI. Cell wall associated proteins involved in filamentation with impact on the virulence of Candida albicans. Microbiol Res. (2022) 258:126996. doi: 10.1016/j.micres.2022.126996
Keywords: fungi, microbiota, mycobiome, oral cancer, oral mucosa, oral oncology
Citation: Monteiro JS, Kaushik K, de Arruda JAA, Georgakopoulou E, Vieira AT, Silva TA, Devadiga D, Anyanechi CE and Shetty S (2024) Fungal footprints in oral cancer: unveiling the oral mycobiome. Front. Oral. Health 5:1360340. doi: 10.3389/froh.2024.1360340
Received: 22 December 2023; Accepted: 14 February 2024;
Published: 14 March 2024.
Edited by:
Ronell Bologna-Molina, Universidad de la República, UruguayReviewed by:
Divyashri Baraniya, Temple University, United States© 2024 Monteiro, Kaushik, de Arruda, Georgakopoulou, Vieira, Silva, Devadiga, Anyanechi and Shetty. This is an open-access article distributed under the terms of the Creative Commons Attribution License (CC BY). The use, distribution or reproduction in other forums is permitted, provided the original author(s) and the copyright owner(s) are credited and that the original publication in this journal is cited, in accordance with accepted academic practice. No use, distribution or reproduction is permitted which does not comply with these terms.
*Correspondence: Sameep Shetty c2FtZWVwLnNoZXR0eUBtYW5pcGFsLmVkdQ==
Disclaimer: All claims expressed in this article are solely those of the authors and do not necessarily represent those of their affiliated organizations, or those of the publisher, the editors and the reviewers. Any product that may be evaluated in this article or claim that may be made by its manufacturer is not guaranteed or endorsed by the publisher.
Research integrity at Frontiers
Learn more about the work of our research integrity team to safeguard the quality of each article we publish.