- Oral Ecology Research Group, Faculty of Dentistry, Université Laval, Quebec, QC, Canada
Periodontitis, an inflammatory disease that affects tooth-supporting tissues, is the result of a polymicrobial infection involving mainly Gram negative anaerobic bacteria. The aim of the present study was to investigate the effects of a phenolic-rich extract of cocoa (Theobroma cacao L.) beans on the pathogenic properties of Porphyromonas gingivalis, which is well-known as a keystone pathogen in the development of periodontitis. The effect of the cocoa extract on P. gingivalis-induced activation of the nuclear factor kappa B (NF-κB) transcription factor in a monocyte model was also assessed. The cocoa extract, whose major phenolic compound was epicatechin, inhibited the growth, hemolytic activity, proteolytic activities, and adherence properties (basement membrane matrix, erythrocytes) of P. gingivalis in a dose-dependent manner. It also protected the barrier function of a keratinocyte model against the deleterious effects mediated by P. gingivalis, and attenuated reactive oxygen species (ROS) production by oral keratinocytes treated with P. gingivalis. Lastly, the cocoa extract showed an anti-inflammatory property by preventing P. gingivalis-induced NF-κB activation in monocytes. In conclusion, this in vitro study highlighted the potential value of an epicatechin-rich extract of cocoa beans for preventing and/or treating periodontal diseases.
Introduction
Porphyromonas gingivalis, an anaerobic Gram-negative bacterium, is known as a keystone pathogen in the development of periodontitis, which is a chronic inflammatory disease characterized by the progressive and irreversible destruction of the periodontal ligament and alveolar bone [1, 2]. The presence of P. gingivalis in subgingival biofilm is correlated with gingival bleeding, an increase in periodontal pocket depth, and bone resorption [1, 2]. This periodontal pathogen can express a large array of virulence factors, including adhesins, lipopolysaccharides (LPS), outer membrane vesicles, hemolysins, and proteinases [3–5]. These pathogenic determinants contribute to host colonization, nutrient acquisition, evasion of host immune defenses, and periodontal tissue destruction [3–5]. Arg- and Lys-gingipain cysteine proteinases, which are both extracellular and cell-bound, are the main endopeptidases produced by P. gingivalis [6, 7]. P. gingivalis gingipains have a broad spectrum of activity and degrade numerous host proteins such as type I collagen, fibronectin, and immunoglobulins [6, 7].
The oral mucosa is the first line of defense of the periodontium and acts as a barrier to protect the deeper periodontal connective tissues against bacterial invasion and toxin penetration [8, 9]. This protective function is provided by specialized intercellular tight junction proteins that seal the paracellular space between closely opposed cells and govern the permeability of the epithelial barrier [8, 9]. However, some periodontal pathogens such as P. gingivalis have evolved effective strategies to counteract the protective function of the epithelial barrier [10, 11]. Among others, the gingipains of P. gingivalis have been reported to damage cell-to-cell junctions and impair the epithelial barrier [10, 11].
P. gingivalis interactions with host cells through Toll-like receptors involving the nuclear factor kappa B (NF-κB) signaling pathway activate a complex inflammatory response that plays a crucial role in the progression and severity of periodontitis as well as associated systemic conditions [12, 13]. Macrophages and monocytes play a key role in host defenses against periodontal infections and contribute to the initiation of an adaptive immune response to periodontal pathogens such as P. gingivalis [14, 15]. These cells secrete many pro-inflammatory cytokines and matrix metalloproteinases (MMPs) [15, 16]. Although this host response contributes to gingival tissue homeostasis, excessive stimulation may result in the maintenance of a chronic inflammatory condition due to the continuous secretion of large amounts of inflammatory mediators involved in periodontal tissue destruction [12–15].
Current strategies for treating periodontitis include surgical intervention, mechanical therapy (ultrasonic debridement, scaling, and root planning), and the use of pharmacological agents [17, 18]. The objectives of these approaches are to clean periodontal pockets, polish root surfaces, and kill or eliminate bacterial biofilm. The pharmacological agents that exert an antibacterial effect or modulate the host response, enhance treatment outcomes [18, 19]. In this context, plant polyphenols have attracted the interest of several research groups due to their multi-target modes of action [20–22]. Cocoa (Theobroma cacao L.) is a plant containing a large variety of polyphenolic compounds [23, 24]. The beneficial effects of cocoa on human health have been mainly attributed to its high polyphenolic content. While studies have shown that cocoa polyphenols may be beneficial for treating cardiovascular diseases, endocrine disorders, and cancers, their benefits with regard to periodontal diseases have been poorly studied [25, 26].
Given that P. gingivalis is a major etiological agent of periodontitis, the aims of the present study were to investigate an epicatechin-rich cocoa extract for its ability to impair the pathogenic properties of P. gingivalis and attenuate P. gingivalis-induced activation of the NF-κB transcription factor in a monocyte model.
Materials and Methods
Cocoa Extract
A cocoa extract obtained by ethanol/water extraction of cocoa beans (Theobroma cacao L.) was obtained from Naturex (Avignon, France). A stock solution (20 mg/mL) was prepared in 10% dimethylsulfoxide (DMSO), sterilized by filtration (0.22 μm pore size), and kept at 4°C protected from light for up to 1 month. The phenolic characterization of the cocoa extract, as determined by chromatographic and mass spectrometry analyses, has been reported in a previous study [27]. The extract contains 30.93% (w/w) of polyphenols. Phenolic acids, flavonols, anthocyanins, flavan-3-ols, and procyanidins make up 0.15, 0.72, 0.12, 2.19, and 96.82% of the total polyphenols, respectively. Monomers, dimers, trimers, and tetramers make up 75% of the total procyanidin content.
Bacteria and Growth Conditions
P. gingivalis ATCC 33277 was cultivated in Todd-Hewitt broth (THB; Becton Dickinson and Company, Sparks, MD, USA) supplemented with 0.001% (w/v) hemin and 0.0001% (w/v) vitamin K and was incubated in an anaerobic chamber (80% N2, 10% CO2, 10% H2) at 37°C.
Minimum Inhibitory and Minimum Bactericidal Concentrations
A broth microdilution assay was used to determine the minimum inhibitory concentration (MIC) and minimum bactericidal concentration (MBC) values of the cocoa extract [28]. The MIC and MBC assays were performed in triplicate in three independent experiments.
Hemagglutinating Activity
Erythrocytes were harvested from sheep blood (Nutri-Bact, Terrebonne, QC, Canada) by centrifugation (600 x g for 5 min), washed three times in 50 mM phosphate-buffered saline (pH 7.2, PBS), and suspended in the same buffer at a concentration of 2% (v/v). The effect of the cocoa extract on the hemagglutinating activity of P. gingivalis was assessed in a round-bottom microtiter plate. Two-fold serial dilutions of a P. gingivalis suspension (optical density at 660 nm [OD660] of 1.0 in PBS) were placed in the wells of the microplate in the absence or presence of the cocoa extract (3.9, 7.81, 15.63, 31.25 μg/mL). An equal volume (100 μL) of the erythrocyte suspension was added, and the microplate was shaken at room temperature for 30 min. After a further incubation at 4°C for 4 h, the reciprocal titer of the highest dilution of bacteria causing hemagglutination was recorded. The hemagglutination assay was performed in triplicate in three independent experiments.
Hemolytic Activity
Equal volumes (1 mL) of the above erythrocyte suspension, P. gingivalis suspension, and two-fold serial dilutions of the cocoa extract (final concentrations in the assay: 3.9, 7.81, 15.625, 31.25, 62.5 μg/mL) were mixed and were incubated overnight at 37°C. PBS replaced the bacteria in the negative control. Following the incubation at 37°C, the mixtures were further incubated at 4°C for 1 h. They were then centrifuged (10,000 x g for 5 min), and the absorbance of the supernatants was read at 540 nm (A540). Hemolysis caused by P. gingivalis in the absence of the cocoa extract was assigned a value of 100%. Assays were performed in triplicate in three independent experiments, and the means ± standard deviations were calculated.
Collagenase Activity
To determine the effect of the cocoa extract on the collagenase activity of P. gingivalis, a 48-h culture supernatant was incubated with the extract (final concentrations in the assay: 15.625, 31.25, 62.5, 125, 250 μg/mL) and the fluorescent substrate collagen DQ (Molecular Probes, Eugene, OR, USA) (100 μg/mL) in the wells of a clear bottom black wall microplate (Greiner Bio-One North America, Monroe, NC, USA) for 60 min at 37°C. The fluorescence corresponding to collagen degradation was then read using a Synergy 2 microplate reader (BioTek Instruments, Winooski, VT, USA), with the excitation and emission wavelengths set at 495 and 525 nm, respectively. The cocoa extract or the fluorescent substrate alone were used as controls. Leupeptin (100 μg/mL) was used as a positive inhibitor control. Collagenase activity obtained in the absence of the cocoa extract was assigned a value of 100%. Assays were performed in triplicate in three independent experiments, and the means ± standard deviations were calculated.
Gingipain Activity
To evaluate the effect of the cocoa extract on the Arg- and Lys-gingipain activities of P. gingivalis, bacteria from an overnight culture were harvested by centrifugation, suspended in PBS (OD660 of 0.4), and added into the wells of a 96-well microplate. The bacteria were incubated at 37°C with 5 mM of either N-α-benzoyl-DL-arginine-p-nitroanilide (Arg-gingipain substrate) or N-p-tosyl-glycine-proline-lysine-p-nitroanilide (Lys-gingipain substrate) and 10 mM dithiothreitol in the presence or absence of the cocoa extract (15.625, 31.25, 62.5, 125, 250 μg/mL). Hydrolysis of the chromogenic substrates was monitored by reading the absorbance at 405 nm (A405) of the cell-free supernatant of the assay mixtures after 60 min (Arg-gingipain) or 18 h (Lys-gingipain) of incubation at 37°C. Tosyl-L-lysine chloromethyl ketone hydrochloride (4 mM) was used as a positive inhibitor control. Gingipain activity in the absence of the cocoa extract was assigned a value of 100%. Assays were performed in triplicate in three independent experiments, and the means ± standard deviations were calculated.
Activity of Matrix Metalloproteinase 9
The effect of the cocoa extract (62.5, 125, 250 μg/mL) on MMP-9 activity was assessed using an MMP-9 Inhibitor Screening Fluorometric Assay Kit (Abcam Inc., Toronto, ON, Canada) according to the manufacturer's protocol. MMP-9 activity was monitored following a 20-min incubation at 37°C by recording fluorescence, with the excitation and emission wavelengths set at 328 nm and 420 nm, respectively. N-isobutyl-N-[4-methoxyphenylsulfonyl]glycyl hydroxamic acid (NNGH; 1 mM) was used as a control inhibitor of MMP-9 activity. MMP-9 activity in the absence of the cocoa extract was assigned a value of 100%. Assays were performed in triplicate in three independent experiments, and the means ± standard deviations were calculated.
Adherence to a Basement Membrane Matrix Model
The effect of the cocoa extract on the adherence of P. gingivalis to Matrigel™ (BD Biosciences, Franklin Lakes, NJ, USA), a well-known in vitro basement membrane matrix model composed of major extracellular matrix proteins, including laminin, type IV collagen, and heparin sulfate proteoglycans, was assessed using fluorescein isothiocyanate (FITC)-labeled bacteria as described in a previous study [29]. Following a 2-h incubation of the bacteria with the Matrigel™ in the presence or absence of the cocoa extract (7.81, 15.625, 31.25, 62.5, 125, 250 μg/mL), relative fluorescence units (RUF; excitation wavelength 495 nm; emission wavelength 525 nm) corresponding to the level of bacterial adherence were read with a Synergy 2 microplate reader (BioTek Instruments). Wells with no bacteria were used as a control to determine basal autofluorescence, which was subtracted from the adherence values. Bacterial adherence in the absence of the cocoa extract was assigned a value of 100%. Assays were performed in triplicate in three independent experiments, and the means ± standard deviations were calculated.
Transepithelial Electrical Resistance of Oral Keratinocytes
The human oral keratinocyte cell line B11 [30], which was kindly provided by S. Gröeger and J. Meyle (Justus-Lieig-University Giessen, Germany), was used to investigate the protective effect of the cocoa extract against P. gingivalis-mediated deleterious effects on keratinocyte barrier function. The cells were cultured in keratinocyte serum-free medium (K-SFM; Life Technologies Inc., Burlington, ON, Canada) supplemented with 50 μg/mL of bovine pituitary extract, 5 ng/mL of recombinant epidermal growth factor, and 100 μg/mL of penicillin G-streptomycin. Keratinocytes were seeded on TranswellTM clear polyester membrane inserts (6.5-mm diameter, 0.4-μm pore size; Corning Co., Cambridge, MA, USA) at a concentration of 3 × 105 cells per insert. The basolateral and apical chambers were filled with 0.6 mL and 0.1 mL of culture medium, respectively. After a 72-h incubation (37°C/5% CO2), the culture medium was replaced with fresh antibiotic-free K-SFM, and the cells were incubated for a further 16 h. Non-cytotoxic concentrations of the cocoa extract (31.25, 62.5, 125 μg/mL) identified in a previous study [27] were added to the apical chamber along with P. gingivalis at a multiplicity of infection (MOI) of 104. The barrier function of the keratinocyte model was assessed by monitoring the transepithelial electrical resistance (TEER) at time 0 and after a 48-h incubation (37°C/5% CO2) using an ohmmeter (EVOM2, World Precision Instruments, Sarasota, FL, USA). Resistance values were calculated in Ohms (Ω)/cm2 by multiplying the resistance values by the surface area of the membrane filter. A 100% value was assigned to the TEER value at time 0. Assays were performed in triplicate in three independent experiments, and the means ± standard deviations were calculated.
Reactive Oxygen Species Production by Oral Keratinocytes
A fluorometric assay was used to monitor the oxidation of 2', 7'-dichlorofluorescein-diacetate (DCF-DA; Sigma-Aldrich Canada Co., Oakville, ON, Canada) into a fluorescent compound that reflects reactive oxygen species (ROS) production. DCF-DA (40 mM) was freshly prepared in DMSO. B11 oral keratinocytes were seeded in the wells (105 cells/well) of a 96-well microplate with black walls and a clear flat bottom (Greiner Bio-One North America) and were incubated overnight at 37°C in a 5% CO2 atmosphere. The cells were washed with Hank's balanced salt solution (HBSS; HyClone Laboratories, Logan, UT, USA) and were incubated for 30 min in the presence of 100 μM DCF-DA in HBSS. Excess DCF-DA was removed, and the keratinocytes were washed with HBSS. The cells were then treated with P. gingivalis (MOI of 103 in HBSS) in the absence or presence of the cocoa extract (3.9, 7.8, 15.625, 31.25, 62.5, 125 μg/mL in culture medium). The fluorescence emission corresponding to ROS production was monitored after a 6-h incubation at 37°C using a Synergy 2 microplate reader (BioTek Instruments), with the excitation and emission wavelengths set at 485 nm and 528 nm, respectively. A 100% value was assigned to ROS production caused by P. gingivalis in the absence of the cocoa extract. Assays were performed in triplicate in three independent experiments, and the means ± standard deviations were calculated.
NF-B Activation in a Monocyte Model
The human monoblastic leukemia cell line U937-3xκB-LUC, which was kindly provided by R. Blomhoff and H. Carlsen (University of Oslo, Norway), is a subclone of the U937 cell line stably transfected with a construct consisting of three NF-κB binding sites from the Ig κ light chain promoter coupled to the luciferase gene [31]. The cells were cultivated in Roswell Park Memorial Institute 1640 medium (RPMI-1640; Life Technologies Inc.) supplemented with 10% heat-inactivated fetal bovine serum (FBS), 100 μg/mL of penicillin/streptomycin, and 75 μg/mL of hygromycin B at 37°C in a 5% CO2 atmosphere. The effect of the cocoa extract (3.9, 7.81, 15.625, 31.25, 62.5, 125 μg/mL) on P. gingivalis-induced NF-κB activation was assessed. These concentrations were found to be non-cytotoxic in a previous study [27]. The monocyte suspension (50 μL, 3 × 106 cells/mL) was seeded in the wells of a black bottom, black wall 96-well microplate (Greiner Bio-One North America). The cocoa extract (50 μL) was added and the microplate was incubated for 30 min at 37°C in a 5% CO2 atmosphere. P. gingivalis (50 μL, MOI of 102) was then added to induce the activation of the NF-κB signaling pathway, and the plate was incubated for a further 6 h at 37°C in a 5% CO2 atmosphere. NF-κB activation was then monitored using a commercial luciferase assay kit (Bright-Glo™ Luciferase Assay System; Promega, Madison, WI, USA) by adding 100 μL of the luciferase substrate to each well. Luminescence was recorded using the luminometer option of a Synergy 2 microplate reader (BioTek Instruments). BAY 11-7082 (Selleckchem, Houston, TX, USA), a known inhibitor of the NF-κB pathway, was used as a positive control. A 100% value was assigned to the NF-κB activation caused by P. gingivalis in the absence of cocoa extract. Assays were performed in triplicate in three independent experiments, and the means ± standard deviations were calculated.
Statistical Analysis
A one-way ANOVA with a post-hoc Bonferroni multiple comparison test was used to analyze the data. Results were considered statistically significant at p < 0.01 or p < 0.001.
Results
Effects on the Growth and Virulence Properties of P. Gingivalis
The antibacterial activity of the epicatechin-rich cocoa extract against the reference strain P. gingivalis ATCC 33277 was assessed using a broth microdilution assay. As shown in Figure 1, the extract dose-dependently inhibited the growth of P. gingivalis. A concentration of 1,000 μg/mL reduced bacterial growth by 84% while 2,000 μg/mL completely inhibited growth. No MBC value was obtained.
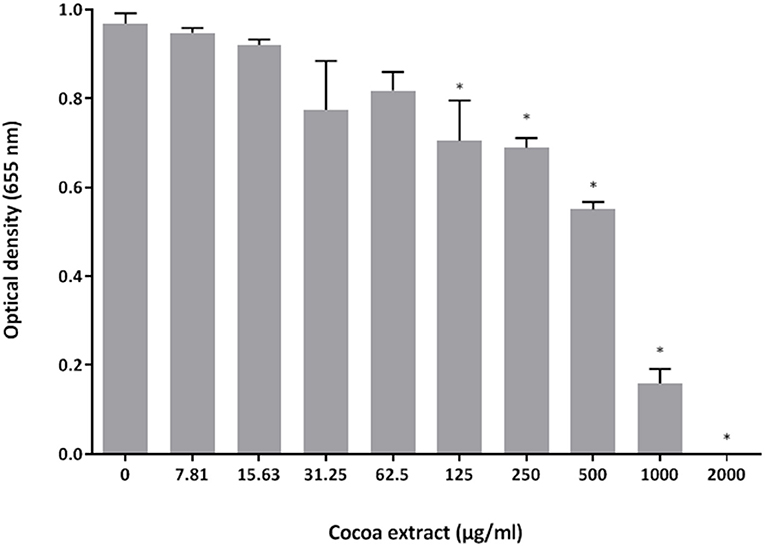
Figure 1. Effect of the cocoa extract on the growth of P. gingivalis. Bacterial growth was monitored by recording the optical density at 655 nm following a 24-h incubation at 37°C under anaerobiosis. *significant inhibition (p < 0.01) compared to the control (no cocoa extract).
The effects of the epicatechin-rich cocoa extract on selected virulence properties of P. gingivalis were then investigated. In a hemolytic assay using sheep erythrocytes, the extract dose-dependently inhibited hemolysis (Figure 2). Complete inhibition was obtained with cocoa extract concentrations as low as 31.25 μg/mL.
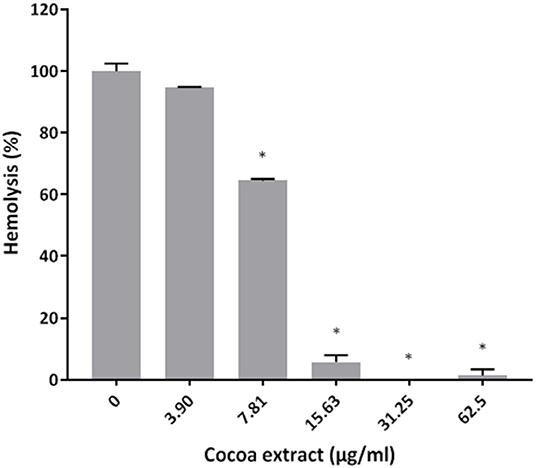
Figure 2. Effect of the cocoa extract on the hemolytic activity of P. gingivalis cells. Hemolysis of sheep red blood cells was monitored by recording the absorbance at 540 nm (A540) following an overnight incubation at 37°C and a one-h incubation at 4°C. A relative value of 100% was assigned to hemolysis obtained in the absence of the cocoa extract. *significant inhibition (p < 0.01) compared to the control (no cocoa extract).
Thereafter, the ability of the cocoa extract to reduce the degradation of type I collagen by proteinases in a culture supernatant of P. gingivalis was investigated. A significant dose-dependent inhibition was observed. More specifically, 250 μg/mL of the extract reduced the P. gingivalis-mediated degradation of type I collagen by 89.9%, while a concentration of 31.25 μg/mL reduced degradation by 31.4% (Figure 3). We also tested the effect of the epicatechin-rich cocoa extract on the cell-associated Arg- and Lys-gingipain activities of P. gingivalis. The extract dose-dependently decreased the activity of Arg-gingipain (Figure 4A) and, to a lesser extent, Lys-gingipain (Figure 4B). At a concentration of 250 μg/mL, the cocoa extract reduced Arg-gingipain activity by 72.3% and Lys-gingipain activity by 61.3%. Based on this interesting observation that the epicatechin-rich cocoa extract inhibits bacterial proteolytic enzymes, we hypothesized that it may also be effective against host-derived MMPs that are known to contribute to tissue destruction during periodontitis. We used MMP-9 as a model and found that the cocoa extract inhibits the catalytic activity of MMP-9 in a dose-dependent manner (Figure 5) with 62.5, 125, and 250 μg/mL of the cocoa extract inhibiting MMP-9 activity by 20.4, 40.9, and 52.3%, respectively.
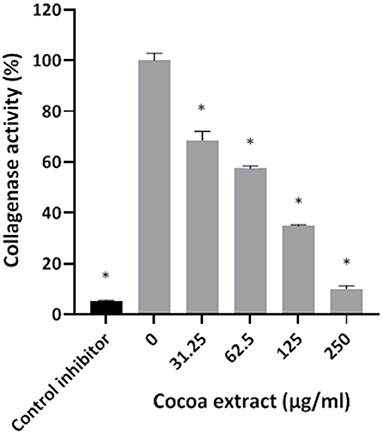
Figure 3. Effect of the cocoa extract on the collagenase activity of P. gingivalis. Leupeptin was used as a control inhibitor. Collagen degradation was monitored by recording the fluorescence at excitation and emission wavelengths of 495 and 525 nm, respectively, following a 60-min incubation at 37°C. A relative value of 100% was assigned to the degradation of type I collagen obtained in the absence of the cocoa extract. *significant inhibition (p < 0.01) compared to the control (no cocoa extract).
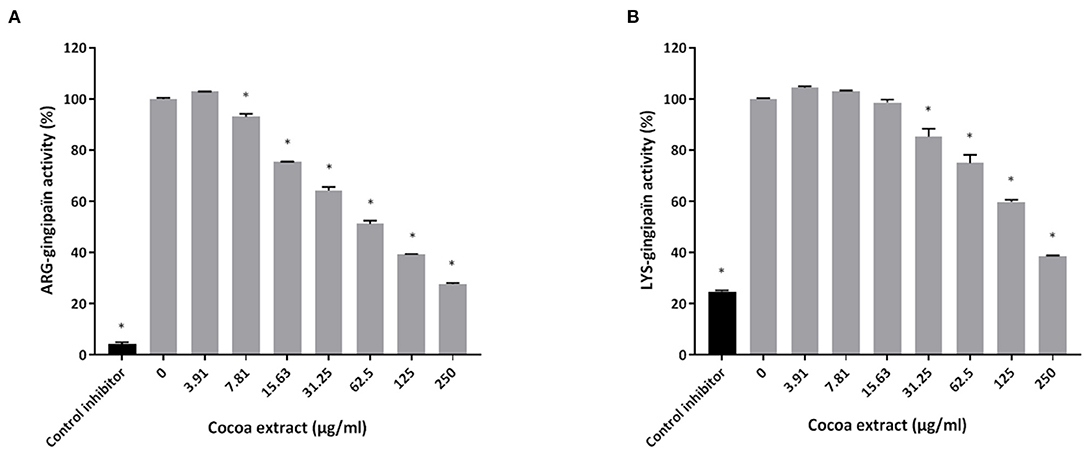
Figure 4. Effect of the cocoa extract on the Arg-gingipain activity (A) and Lys-gingipain activity (B) of P. gingivalis. Tosyl-L-lysine chloromethyl ketone hydrochloride was used as a control inhibitor. Hydrolysis of the chromogenic substrates was monitored by recording the absorbance at 405 nm (A405) after 60 min (Arg-gingipain) or 18 h (Lys-gingipain) of incubation at 37°C. A relative value of 100% was assigned to the activity obtained in the absence of the cocoa extract. *significant inhibition (p < 0.01) compared to the control (no cocoa extract).
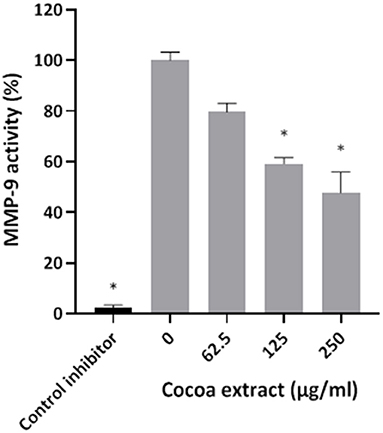
Figure 5. Effect of the cocoa extract on MMP-9 activity. N-isobutyl-N-[4-methoxyphenylsulfonyl]glycyl hydroxamic acid was used as a control inhibitor. MMP-9 activity was monitored by recording the fluorescence at excitation and emission wavelengths of 328 and 420 nm, respectively, following a 20-min incubation at 37°C. A relative value of 100% was assigned to the activity obtained in the absence of the cocoa extract. *significant inhibition (p < 0.01) compared to the control (no cocoa extract).
The ability of the cocoa extract to inhibit the adherence of P. gingivalis was then evaluated. Untreated P. gingivalis cells had an hemagglutination titer of 8 in a sheep erythrocyte assay (Table 1). The titer decreased to 2 in the presence of 3.9 μg/mL of the cocoa extract. Surprisingly, ≥ 15.625 μg/mL of the cocoa extract promoted the agglutination of erythrocytes, even in the absence of P. gingivalis. The effect of the epicatechin-rich cocoa extract on the adherence of P. gingivalis to a polystyrene surface coated with Matrigel™, a basement membrane matrix model, was also assessed. At the highest concentration tested (250 μg/mL), the cocoa extract inhibited adherence to the Matrigel™-coated polystyrene surface by 34.9% (Figure 6).
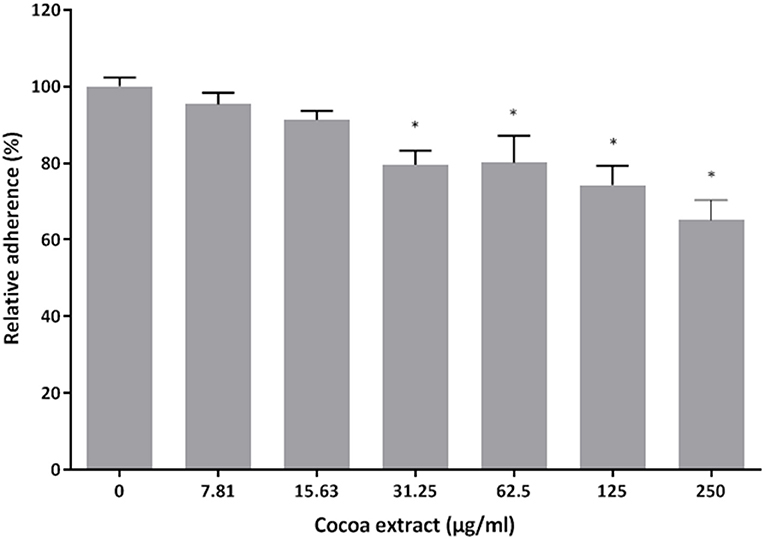
Figure 6. Effect of the cocoa extract on the adherence of P. gingivalis to the Matrigel™ basement membrane model. Adherence of FITC-labeled bacteria was monitored by recording the fluorescence at excitation and emission wavelengths of 495 and 525 nm, respectively, following a 2-h incubation. A relative value of 100% was assigned to the adherence obtained in the absence of the cocoa extract. *significant inhibition (p < 0.01) compared to the control (no cocoa extract).
Effect on P. Gingivalis-Induced Loss of Oral Keratinocyte Barrier Function
Given that P. gingivalis has been reported to have deleterious effects on keratinocyte barrier integrity [10, 11], we determined whether the epicatechin-rich cocoa extract protects oral keratinocytes from this damage. Treating the keratinocytes for 48 h with P. gingivalis at an MOI of 104 significantly decreased TEER by 68.1% compared to control untreated cells (Figure 7). However, the presence of the cocoa extract at all the concentrations tested (31.25, 62.5, 125 μg/mL) significantly reduced the P. gingivalis-induced decrease in TEER in the oral keratinocyte model used.
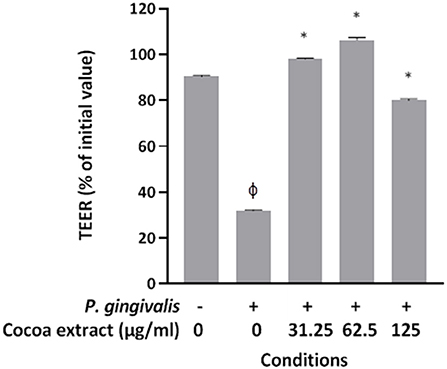
Figure 7. Effect of the cocoa extract on P. gingivalis-mediated loss of oral keratinocyte barrier function. Bacteria were used at MOI of 104, and the barrier function of the keratinocyte model was assessed by monitoring the transepithelial electrical resistance (TEER) values at time 0 and after a 48-h incubation (37°C/5% CO2) using an ohmmeter. A relative value of 100% was assigned to the TEER recorded at time 0. Results are expressed as the means ± SD of triplicate assays from three independent experiments. φ, significant decrease (p < 0.001) compared to unstimulated control cells. *, significant increase (p < 0.001) compared to P. gingivalis-stimulated cells not treated with the cocoa extract.
Effect on P. Gingivalis-Induced ROS Production by Oral Keratinocytes
Treating the oral keratinocytes with P. gingivalis (6 h) increased the production of ROS 1.64-fold compared to untreated control cells (Figure 8). However, the presence of the cocoa extract at all the concentrations tested (7.81 to 125 μg/ml) significantly reduced P. gingivalis-induced ROS production (Figure 8). ROS levels decreased by 61.4% with 7.81 μg/mL of cocoa extract.
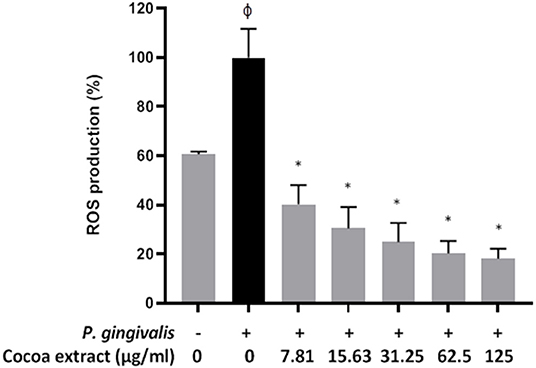
Figure 8. Effect of the cocoa extract on P. gingivalis-induced ROS production by oral keratinocytes. Bacteria were used at MOI of 103, and ROS production was monitored by recording the fluorescence at excitation and emission wavelengths of 485 and 528 nm, respectively, following a 6-h incubation (37°C/5% CO2). A relative value of 100% was assigned to ROS production in the absence of the cocoa extract. φ, significant increase compared to the control (no P. gingivalis stimulation). *significant inhibition (p < 0.001) compared to the control (no cocoa extract + P. gingivalis stimulation).
Effect on P. Gingivalis-Induced NF-κB Activation in a Monocyte Model
To assess the anti-inflammatory potential of the epicatechin-rich cocoa extract, we examined its ability to reduce P. gingivalis-induced NF-κB activation using the U937-3xκB monocytic cell line. As shown in Figure 9, the cocoa extract dose-dependently reduced P. gingivalis-induced NF-κB activation. Significant inhibitory effects (34.2% to 77.4%) were observed at concentrations of the cocoa extract ranging from 15.625 to 125 μg/mL. As expected, the commercial positive inhibitory control (BAY-11-7082) caused a complete inhibition of NF-κB activation.
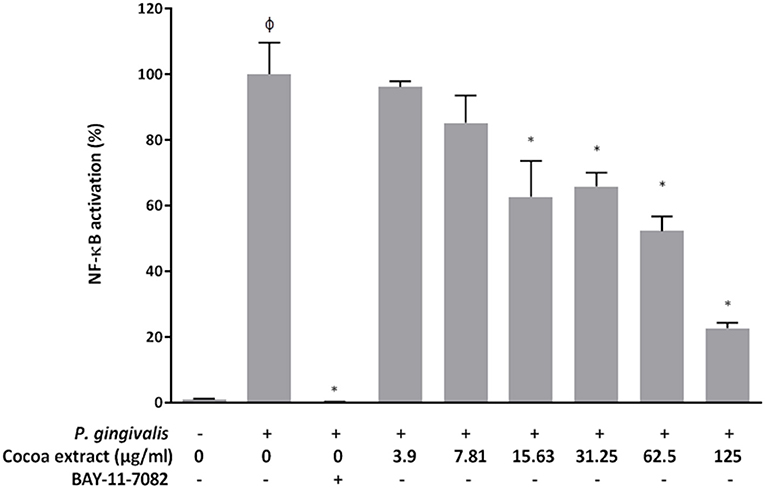
Figure 9. Effect of the cocoa extract on the activation of the NF-κB signaling pathway induced by P. gingivalis (MOI of 102) using the human monoblastic leukemia cell line U937-3xκB-LUC. BAY-11-7082 was used as a control inhibitor. NF-κB activation was monitored by recording luminescence after a 6-h incubation (37°C/5% CO2). A value of 100% was assigned to the activation in the absence of the cocoa extract. φ, significant increase (p < 0.001) compared to the control (no P. gingivalis stimulation). *significant inhibition (p < 0.001) compared to the control (no cocoa extract + P. gingivalis stimulation).
Discussion
Increasing evidence suggests that the phytochemical constituents of cocoa, particularly polyphenols, demonstrate beneficial health properties with the capacity to improve and prevent several human disorders [24–26]. However, the potential benefits of cocoa in terms of periodontal health have been poorly studied to date. In the present study, we hypothesized that an epicatechin-rich cocoa extract may impair the growth and virulence properties of P. gingivalis and attenuate the activation of the NF-κB transcription factor induced by this periodontal pathogen in a monocyte model.
We first showed that the cocoa extract completely inhibits the growth of P. gingivalis when added to the culture medium at a concentration of 2,000 μg/mL. Since no MBC value was obtained, it suggests that the cocoa extract has a bacteriostatic mode of action, although a bactericidal effect may be obtained at concentrations >2,000 μg/mL. Given that the cocoa extract contains a variety of polyphenols [27], it is likely that the antibacterial mechanism of action against P. gingivalis involves different targets. However, the growth inhibitory effect may be related to the ability of epicatechin, which is the main phenolic compound in the cocoa extract, to bind to the lipid bilayer of the bacterial membrane, resulting in the disruption of the cell structure, leading to cell death [32]. Previous studies have also reported that cocoa extracts exert antibacterial activity against periodontal pathogens, including Fusobacterium nucleatum, Prevotella intermedia, and P. gingivalis [27, 33].
The ability of P. gingivalis to cause lysis of erythrocytes and release hemoglobin is considered a virulence determinant since it provides an iron source to periodontal pathogens that promotes their proliferation in periodontal pockets [34]. Interestingly, the cocoa extract inhibited the hemolytic activity of P. gingivalis in a dose-dependent manner. By reducing the release of hemoglobin, the cocoa extract may not only impair the proliferation of P. gingivalis but may also reduce the levels of pro-inflammatory mediators in periodontal sites. Bodet et al. [35] previously reported that hemoglobin can synergize with P. gingivalis LPS to amplify the inflammatory response of human macrophages.
P. gingivalis produces several proteolytic enzymes active on a variety of host proteins thus contributing to local tissue destruction and immune defense perturbation [6, 7]. Inhibiting these enzymes may be an effective therapeutic strategy to prevent the initiation and progression of periodontal disease. In the present study, the cocoa extract attenuated collagen degradation by P. gingivalis. It also inhibited cell surface Arg- and Lys-gingipains, which are the main endoproteinases produced by P. gingivalis. Given this anti-proteinase property of the cocoa extract, we further investigated this aspect by assessing its effect on the activity of host MMPs, which are major contributors to periodontal tissue destruction [36, 37]. MMP-9 was used as model as it is found in high amounts in inflamed periodontitis sites and is strongly associated with the progression and severity of periodontitis [36–38]. The present study showed that the cocoa extract exerts a marked dose-dependent inhibitory effect on the catalytic activity of MMP-9, suggesting that it may help reduce periodontal tissue destruction.
The adhesion of P. gingivalis to matrix constituents of the basement membrane is crucial for the colonization of periodontal sites by this periodontal pathogen [3–5]. In the present study, we showed that the cocoa extract can impair the adherence of P. gingivalis to Matrigel™, a well-known basement membrane model containing laminin and type IV collagen as the main protein constituents. As we observed that the cocoa extract also reduces the hemagglutinating activity of the bacterium, this effect likely occurs due to the neutralization of hemagglutinins, which are key adhesins expressed by P. gingivalis [39].
The oral mucosal epithelium is a selective protective barrier that prevents the translocation of periodontal pathogens into gingival connective tissues [8, 9]. In a previous investigation, we showed that the cocoa extract used in the present study can improve the barrier function of an oral keratinocyte model by increasing TEER. As the gingipains produced by P. gingivalis can destroy cell-to-cell junctions, resulting in the disruption of epithelial barrier function [10, 11], we determined whether the cocoa extract protects against the P. gingivalis-mediated deleterious effects. We showed that the epicatechin-rich cocoa extract can protect the integrity of the oral keratinocyte barrier by preventing the decrease in TEER despite a 48-h challenge with P. gingivalis. Part of this protective effect may be related to the ability of the cocoa extract to inhibit P. gingivalis gingipains.
ROS production by mucosal and immune cells significantly increases during chronic periodontal inflammations and results in oxidative stress [40, 41]. Excessive amounts of ROS may damage DNA, proteins, and lipids, leading to cell death. We showed that P. gingivalis induces the production of intracellular ROS in the oral keratinocyte model used and that the addition of the cocoa extract during keratinocyte stimulation significantly inhibits ROS production in a dose-dependent manner.
The transcription factor NF-κB plays a central role in inflammatory disorders such as periodontitis by promoting the expression of a large array of genes encoding pro-inflammatory cytokines [42]. The inhibition of the NF-κB signaling pathway has thus been proposed as a promising therapeutic strategy [43]. In the present study, we showed that the cocoa extract can attenuate P. gingivalis-induced NF-κB activation in a monocyte model, which is in agreement with our previous study using another periodontal pathogen (F. nucleatum) [27]. This anti-inflammatory property may involve the ability of epicatechin, catechin, and procyanidins in T. cacao to modulate NF-κB binding to DNA [44–46]. Additional in vitro models, including gingival mesenchymal stem cells and periodontal ligament stem cells that has been used to demonstrate the ability of ascorbic acid to down-regulate the inflammatory cascade induced by P. gingivalis LPS [47, 48], should be tested to confirm the anti-inflammatory property of the cocoa extract.
In summary, the present study provided evidence that the epicatechin-rich cocoa extract under investigation impairs various pathogenic properties of P. gingivalis, a keystone pathogen in periodontitis. Moreover, the cocoa extract attenuated the inflammatory process associated with periodontitis by inhibiting the NF-κB signaling pathway. Interestingly, Tomofuji et al. [49] reported that a cocoa-enriched diet could reduce alveolar bone loss as well as leukocyte infiltration in a rat-periodontitis model. Further research is required to evaluate the clinical efficacy of cocoa polyphenols incorporated into mouthrinses, toothpastes, gels, or local drug delivery systems.
Data Availability Statement
The original contributions presented in the study are included in the article/supplementary material, further inquiries can be directed to the corresponding author.
Author Contributions
DG conceived and designed the experiments and wrote the manuscript. KV and AB performed the experimental assays and the statistical analyses. All authors approved the submitted version.
Funding
This study was supported by the Fonds Émile-Beaulieu.
Conflict of Interest
The authors declare that the research was conducted in the absence of any commercial or financial relationships that could be construed as a potential conflictof interest.
Publisher's Note
All claims expressed in this article are solely those of the authors and do not necessarily represent those of their affiliated organizations, or those of the publisher, the editors and the reviewers. Any product that may be evaluated in this article, or claim that may be made by its manufacturer, is not guaranteed or endorsed by the publisher.
Acknowledgments
We wish to thank S. Gröeger and J. Meyle (Justus-Liebig-University Giessen, Germany) for providing the B11 keratinocyte cell line. We also thank R. Blomhoff and H. Carlsen (University of Oslo, Norway) for their generous gift of the U937-3xκB-LUC cell line.
References
1. Curtis MA, Diaz PI, Van Dyke TE. The role of the microbiota in periodontal disease. Periodontol 2000. (2020) 83:14–25. doi: 10.1111/prd.12296
2. Abusleme L, Hoare A, Hong B-Y, Diaz PI. Microbial signatures of health, gingivitis, and periodontitis. Periodontol 2000. (2021) 86:57–78. doi: 10.1111/prd.12362
3. Dahlen G, Basic A, Bylund J. Importance of virulence factors for the persistence of oral bacteria in the inflamed gingival crevice and in the pathogenesis of periodontal disease. J Clin Med. (2019) 8:1339. doi: 10.3390/jcm8091339
4. How KY, Song KP, Chan KG. Porphyromonas gingivalis : an overview of periodontopathogenic pathogen below the gum line. Front Microbiol. (2016) 7:53. doi: 10.3389/fmicb.2016.00053
5. Silva IL, Cascales E. Molecular strategies underlying Porphyromonas gingivalis virulence. J Mol Biol. (2021) 433:166836. doi: 10.1016/j.jmb.2021.166836
6. Fitzpatrick RE, Wijeyewickrema LC, Pike RN. The gingipains: scissors and glue of the periodontal pathogen, Porphyromonas gingivalis. Future Microbiol. (2009) 4:471–87. doi: 10.2217/fmb.09.18
7. Grenier D, La VD. Proteases of Porphyromonas gingivalis as virulence factors in periodontal diseases and potential targets for plant-derived compounds: a review article. Curr Drug Targets. (2011) 12:322–31. doi: 10.2174/138945011794815310
8. Groeger SE, Meyle J. Epithelial barrier and oral bacterial infection. Periodontol 2000. (2015) 69:46–67. doi: 10.1111/prd.12094
9. Senel S. An overview of physical, microbiological and immune barriers of oral mucosa. Int J Mol Sci. (2021) 22:7821. doi: 10.3390/ijms22157821
10. Groeger S, Doman E, Chakraborty T, Meyle J. Effects of Porphyromonas gingivalis infection on human gingival epithelial barrier function in vitro. Eur J Oral Sci. (2010) 118:582–89. doi: 10.1111/j.1600-0722.2010.00782.x
11. Sakanaka A, Takeuchi H, Kuboniwa M, Amano A. Dual lifestyle of Porphyromonas gingivalis in biofilm and gingival cells. Microb Pathog. (2016) 94:42–7. doi: 10.1016/j.micpath.2015.10.003
12. Jia L, Han N, Du J, Guo L, Luo Z, Liu Y. Pathogenesis of important virulence factors of Porphyromonas gingivalis via Toll-Like receptors. Front Cell Infect Microbiol. (2019) 9:262. doi: 10.3389/fcimb.2019.00262
13. Hajishengallis G. Periodontitis: from microbial immune subversion to systemic inflammation. Nat Rev Immunol. (2015) 15:30–44. doi: 10.1038/nri3785
14. Hasturk H, Kantarci A, Van Dyke TE. Oral inflammatory diseases and systemic inflammation: role of macrophage. Front Immunol. (2012) 3:118. doi: 10.3389/fimmu.2012.00118
15. Wang W, Zheng C, Yang J, Li B. Intersection between macrophages and periodontal pathogens in periodontitis. J Leukoc Biol. (2021) 110:577–83. doi: 10.1002/JLB.4MR0421-756R
16. Cecil JD, O'Brien-Simpson NM, Lenzo JC, Holden JA, Singleton W, Perez-Gonzalez A, et al. Outer membrane vesicles prime and activate macrophage inflammasomes and cytokine secretion in vitro and in vivo. Front Immunol. (2017) 8:1017. doi: 10.3389/fimmu.2017.01017
17. Graziani F, Karapetsa D, Alonso B, Herrera D. Nonsurgical and surgical treatment of periodontitis: how many options for one disease? Periodontol 2000. (2017) 75:152–88. doi: 10.1111/prd.12201
18. Kwon T, Lamster IB, Levin L. Current concepts in the management of periodontitis. Int Dent J. (2021) 71:462–76. doi: 10.1111/idj.12630
19. Balta MG, Papathanasiou E, Blix IJ, Van Dyke TE. Host modulation and treatment of periodontal disease. J Dent Res. (2021) 100:798–809. doi: 10.1177/0022034521995157
20. Bunte K, Hensel A, Beikler T. Polyphenols in the prevention and treatment of periodontal disease: A systematic review of in vivo, ex vivo and in vitro studies. Fitoterapia. (2019) 132:30–9. doi: 10.1016/j.fitote.2018.11.012
21. Fernandez-Rojas B, Gutierrez-Venegas G. Flavonoids exert multiple periodontic benefits including anti-inflammatory, periodontal ligament-supporting, and alveolar bone-preserving effects. Life Sci. (2018) 209:435–54. doi: 10.1016/j.lfs.2018.08.029
22. Feghali K, Feldman M, La VD, Santos J, Grenier D. Cranberry proanthocyanidins : Natural weapons against periodontal diseases. J Agric Food Chem. (2011) 60:5728–35. doi: 10.1021/jf203304v
23. Oracz J, Zyzelewicz D. Nebesny E. The content of polyphenolic compounds in cocoa beans (Theobroma cacao L), depending on variety, growing region, and processing operations: a review. Crit Rev Food Sci Nutr. (2015) 55:1176–92. doi: 10.1080/10408398.2012.686934
24. Andújar I, Recio MC, Giner RM, Ríos JL. Cocoa polyphenols and their potential benefits for human health. Oxid Med Cell Longev. (2012) 2012:906252. doi: 10.1155/2012/906252
25. Lamuela-Raventos RM, Romero-Pérez AI, Andrés-La Cueva C, Tornero A. Health effects of cocoa flavonoids. Food Sci Tech Int. (2005) 11:159–76. doi: 10.1177/1082013205054498
26. De Araujo QR, Gattward JN, Almoosawi S, Silva Md, Dantas PA, De Araujo Júnior QR. Cocoa and human health: From head to foot- a review. Crit Rev Food Sci Nutr. (2016) 56:1–12. doi: 10.1080/10408398.2012.657921
27. Ben Lagha A, Maquera-Huacho P. Grenier D. A cocoa (Theobroma cacao L) extract impairs the growth, virulence properties, and inflammatory potential of Fusobacterium nucleatum and improves oral epithelial barrier function. PLoS ONE. (2021) 16:e0252029. doi: 10.1371/journal.pone.0252029
28. Ben Lagha A, LeBel G. Grenier D. Tart cherry (Prunus cerasus L) fractions inhibit biofilm formation and adherence properties of oral pathogens and enhance oral epithelial barrier function. Phytother Res. (2020) 34:886–95. doi: 10.1002/ptr.6574
29. Ben Lagha A, Grenier D. Black tea theaflavins attenuate Porphyromonas gingivalis virulence properties, modulate gingival keratinocyte tight junction integrity and exert anti-inflammatory activity. J Periodont Res. (2017) 52:458–70. doi: 10.1111/jre.12411
30. Gröeger S, Michel J, Meyle J. Establishment and characterization of immortalized human gingival keratinocyte cell lines. J Periodont Res. (2008) 43:604–14. doi: 10.1111/j.1600-0765.2007.01019.x
31. Carlsen H, Moskaug JØ, Fromm SH, Blomhoff R. In vivo imaging of NF-kappa B activity. J Immunol. (2002) 168:1441–6. doi: 10.4049/jimmunol.168.3.1441
32. Koech KR, Wachira FN, Ngure RN, Wanyoko JK, Bii CC, Karori SM, et al. Antimicrobial, synergistic and antioxidant activities of tea polyphenols. In: Mendez-Vilas A, editor. Microbial Pathogens and Strategies for Combating Them: Science, Technology and Education. Badajoz: Formatex Research Center (2013). pp. 971–81.
33. Hirao C. Nishimura E, Kamei M, Ohshima T, Maeda N. Antibacterial effects of cocoa on periodontal pathogenic bacteria. J Oral Biosci. (2010) 52:283–91. doi: 10.1016/S1349-0079(10)80033-7
34. Olczak T, Simpson W, Liu X, Genco CA. Iron and heme utilization in Porphyromonas gingivalis. FEMS Microbiol Rev. (2005) 29:119–44. doi: 10.1016/j.femsre.2004.09.001
35. Bodet C, Chandad F, Grenier D. Hemoglobin and LPS act in synergy to amplify the inflammatory response. J Dent Res. (2007) 86:878–82. doi: 10.1177/154405910708600914
36. Checchi V, Maravic T, Bellini P, Generali L, Consolo U, Breschi L, et al. The role of matrix metalloproteinases in periodontal disease. Int J Environ Res Public Hlth. (2020) 17:4923. doi: 10.3390/ijerph17144923
37. Cavalla F, Hernandez-Rios P, Sorsa T, Biguetti C, Hernandez M. Matrix metalloproteinases as regulators of periodontal inflammation. Int J Mol Sci. (2017) 18:440. doi: 10.3390/ijms18020440
38. Kinney JS, Morelli T, Oh M, Braun TM, Ramseier CA, Sugai JV, et al. Crevicular fluid biomarkers and periodontal disease progression. J Clin Periodontol. (2014) 41:113–20. doi: 10.1111/jcpe.12194
39. Lamont RJ, Jenkinson HF. Subgingival colonization by Porphyromonas gingivalis. Oral Microbiol Immunol. (2000) 15:341–9. doi: 10.1034/j.1399-302x.2000.150601.x
40. Wang Y, Andrukhov O, Rausch-Fan X. Oxidative stress and antioxidant system in periodontitis. Front Physiol. (2017) 8:910. doi: 10.3389/fphys.2017.00910
41. Chapple IL, Matthews JB. The role of reactive oxygen and antioxidant species in periodontal tissue destruction. Periodontol 2000. (2007) 43:160–232. doi: 10.1111/j.1600-0757.2006.00178.x
42. Liu T, Zhang L, Joo D, Sun SC. NF-κB signaling in inflammation. Signal Transduct Target Ther. (2017) 2:17023. doi: 10.1038/sigtrans.2017.23
43. Yu H, Lin L, Zhang Z, Zhang H, Hu H. Targeting NF-κB pathway for the therapy of diseases: mechanism and clinical study. Signal Transduct Target Ther. (2020) 5:209. doi: 10.1038/s41392-020-00312-6
44. Selmi C, Mao TK, Keen CL, Schmitz HH, Eric Gershwin M. The anti-inflammatory properties of cocoa flavanols. J Cardiovasc Pharmacol. (2006) 47:S163–76. doi: 10.1097/00005344-200606001-00010
45. Mackenzie GG, Delfino JM, Keen CL, Fraga CG, Oteiza PI. Dimeric procyanidins are inhibitors of NF-kappaB-DNA binding. Biochem Pharmacol. (2009) 78:1252–62. doi: 10.1016/j.bcp.2009.06.111
46. Mao TK, van de Water J, Keen CL, Schmitz HH, Gershwin ME. Modulation of TNF-alpha secretion in peripheral blood mononuclear cells by cocoa flavanols and procyanidins. Dev Immunol. (2002) 9:135–41. doi: 10.1080/1044667031000137601
47. Pizzicannella J, Fonticoli L, Guarnieri S, Marconi GD, Rajan TS, Trubiani O, et al. Antioxidant ascorbic acid modulates NLRP3 inflammasome in LPS-G treated oral stem cells through NFκB/caspase-1/IL-1β pathway. Antioxidants. (2021) 10:797. doi: 10.3390/antiox10050797
48. Marconi GD, Fonticoli L, Guarnieri S, Cavalcanti MFXB, Franchi S, Gatta V, et al. Ascorbic acid: a new player of epigenic regulation in LPS-gingivalis treated human periodontal ligament stem cells. Oxid Med Cell Longev. (2021) 2021:6679708. doi: 10.1155/2021/6679708
Keywords: cocoa, epicatechin, oral keratinocytes, virulence factors, Porphyromonas gingivalis, periodontal disease
Citation: Vaillancourt K, Ben Lagha A and Grenier D (2022) A Phenolic-rich Extract of Cocoa (Theobroma cacao L.) Beans Impairs the Pathogenic Properties of Porphyromonas gingivalis and Attenuates the Activation of Nuclear Factor Kappa B in a Monocyte Model. Front. Oral. Health 3:867793. doi: 10.3389/froh.2022.867793
Received: 01 February 2022; Accepted: 25 February 2022;
Published: 22 March 2022.
Edited by:
Fabian Cieplik, University Medical Center Regensburg, GermanyReviewed by:
Guya Diletta Marconi, University of Studies G. d'Annunzio Chieti and Pescara, ItalyHiroyuki Tada, Tohoku University, Japan
Copyright © 2022 Vaillancourt, Ben Lagha and Grenier. This is an open-access article distributed under the terms of the Creative Commons Attribution License (CC BY). The use, distribution or reproduction in other forums is permitted, provided the original author(s) and the copyright owner(s) are credited and that the original publication in this journal is cited, in accordance with accepted academic practice. No use, distribution or reproduction is permitted which does not comply with these terms.
*Correspondence: Daniel Grenier, RGFuaWVsLkdyZW5pZXJAZ3JlYi51bGF2YWwuY2E=