- 1Department of Research, Plus Identity Institute, São Paulo, Brazil
- 2Centro de Investigación en Transformación Digital, Universidad Norbert Wiener (UNW), Lima, Perú
- 3Postgraduation Program in Engineering, Universidade Paulista (UNIP), São Paulo, Brazil
- 4The Craniofacial Center, University of Illinois at Chicago, Chicago, IL, United States
- 5Postgraduation Program in Dentistry, Universidade Paulista (UNIP), São Paulo, Brazil
Historically, facial prosthetics have successfully rehabilitated individuals with acquired or congenital anatomical deficiencies of the face. This history includes extensive efforts in research and development to explore best practices in materials, methods, and artisanal techniques. Presently, extraoral maxillofacial rehabilitation is managed by a multiprofessional team that has evolved with a broadened scope of knowledge, skills, and responsibility. This includes the mandatory integration of different professional specialists to cover the bio-psycho-social needs of the patient, systemic health and pathology surveillance, and advanced restorative techniques, which may include 3D technologies. In addition, recent digital workflows allow us to optimize this multidisciplinary integration and reduce the active time of both patients and clinicians, as well as improve the cost-efficiency of the care system, promoting its access to both patients and health systems. This paper discusses factors that affect extraoral maxillofacial rehabilitation's present and future opportunities from teamwork consolidation, techniques utilizing technology, and health systems opportunities.
Introduction
Head and neck cancer management requires a reconstruction and rehabilitation multidisciplinary plan to transform the original oncological pathology and disability toward restored bio-psycho-social functioning (1, 2). Most head and neck oncology services that want to promote this multiprofessional approach do not have the necessary in-house professionals to address the patients’ broad scope of needs. Therefore, patients are often referred externally or directed to rehabilitation services remotely located (3–6).
The teamwork composition around maxillofacial patients’ needs must include oncology surveillance, systemic physiologic patient condition complications and microbiology, advanced 3D workflow technologies, biomaterials, advanced restorative techniques, osseointegration, and hyper-realistic artistic skills (7–9). All these professional competencies may be concentrated in a system with one or more professionals possessing the competence and legal responsibility necessary for the patient's care. The United States, United Kingdom, and other developed regions are examples of how an education and certification structure has been established for healthcare professionals who must face specializations, subspecializations, and board certification programs to allow patients and health systems to trust their skills and multiprofessional capabilities (10–14). However, this is a specific reality for unique countries that do not necessarily match most parts of the world's public health coverage, needs, and level of education. Furthermore, worldwide professionals cannot justify professions like anaplastology and ocularists in their own countries if their laws cannot support and protect them. Worldwide, dabbler practices are illegal and a public health risk. This is the case when insufficiently trained or supervised lab technicians are treating patients or self-taught people provide care with a self-claimed professional status. They are both dabblers and illegal practitioners facing a severe risk and possible felony. If the country's law does not recognize anaplastology or ocularistry, they have no legal foundation to provide legal patient healthcare in these regions. On the other hand, multiprofessional management empowers individual skills and, under a coordinated intervention and delimitation of responsibilities, allows patients to have a secure rehabilitation process with professionals who exercise their vocation within their defined scope of service.
The American Academy of Maxillofacial Prosthodontics was founded in 1953 when dentists’ first education and training in maxillofacial prosthodontics was of significant concern. In the United States, from 1958 to 1977, 2-year teaching programs were offered. From 1977 to 1984, 3-year programs were offered, and the ADA Commission accredited these on Dental Education (15). Maxillofacial prosthodontists obtain their title after a subspecialization of prosthodontics. This is possible after a dentistry program confers a degree that allows the professional to care for the patient's health as a doctor. The International Anaplastology Association was founded in 1980 as the American Anaplastology Association. Its consolidation as a formal profession in the Unites States arose from wartime necessity. Military hospitals provided care to veterans and identified the need for even more specialized care in both laboratory and clinical setups for the artificial replacement of more complex structures of the face requiring more artistic skills. Thanks to Walter Spohn and Stanford University in 1971, the anaplastology profession started as a formal training program. This 2-year degree course included art and basic sciences, materials and methods, ethics, and business practices. Today, very few places in the world offer formal degree training, usually a 2-year master's program with a previous bachelor’s in art, technology, or other medically related fields (6, 16).
In under-resourced regions, a vicious circle is occurring. The lack of formal education and legal framework maintains professionals without formal training. As a result, fewer professionals remain insufficient to sustain the necessary professional structure within most healthcare systems. Yet, it is a day-to-day reality worldwide among appropriately trained and certified maxillofacial prosthodontists, anaplastologists, and ocularists who are working on solving these real-life problems to serve expanding patient populations.
Facial prosthetics production
No other body part can reveal feelings, emotions, and character like the face of a person. Therefore, its alteration comes with a solid and intrinsic need to hide facial defects and seek restorative care. Ancient registers support this statement, like the Chinese using resins and metallic parts to hide eyes and faces. Egyptian mummies have been discovered with stone and mosaic replicas of facial parts. Romans documented “eye makers,” “doctors of the eye,” and much more. Restoring anatomy to enable function, cognition reinforcement, and esthetics is a human need (17–20).
Facial prostheses are customized medical-grade devices used to restore severe functional, cognitive, and esthetic alterations to positively impact the patient's daily living activities in a bio-psycho-social way. Three significant steps are well described in the literature to produce facial prosthetics (17, 18, 21, 22). The analog manufacturing process starts with a molding of the facial defect. With the obtained gypsum working model, a sculpture can be fabricated with a thermoplastic material that will mimic the lost anatomy, respecting functional and esthetic principles. Once finished, a mold is created as a negative version of the sculpture. Multiple layers of intrinsically characterized medical-grade silicone are packed accordingly to replicate the patient's skin color. However, in most regions of the world, the prosthetic context requires manufacturing them by analog processes such as manual molding, sculpting, and coloring, as well as using acrylic resin materials, as has been done since the origin of this specialty, among other adaptations of the procedure to the local reality (18, 23–25).
High learning curves exist to exact this technical task and to reduce the chances of a mistake or remakes of the prosthesis. To overcome this artisanal and time-consuming process, specialists have looked to digital technologies to assist or replace some steps in the process, like molding and sculpting (26–35).
3D data acquisition
Molding processes have been utilizing different 3D image acquisition methods
The first 3D data acquisition trials and digital workflows were performed using MRI data and CT scans because they were the most well-known imaging methodologies to capture the anatomy, becoming more prevalent as cone-beam computed tomography (CBCT) emerged on the market with up to 10% of the radiation dose. Its main advantage is the precision and veracity of the acquired external surface, as well as the possibility of capturing negative areas such as lumens or ears with capricious anatomies. However, nowadays, there is no indication to irradiate a patient with a CT scan for extraoral surface scanning or use expensive MRI technology, both of which lack sufficient image resolution and color information to adequately replicate the level of detail required in a surface scan in facial prosthetics. CT scans have a unique use for extraoral surface data acquisition only when osseointegrated implants are being planned. It can help transport the head's position and allow the designer to mirror the healthy anatomy with just one scan (23, 26, 27, 36–41).
In addition, DICOM images require a thorough segmentation process that can add or remove information from the surface if not handled properly. To segment the facial anatomy, the Hounsfield threshold is used on an appropriate scale. Depending on the application, there are situations where semiautomatic and automatic segmentation tools could be used, but this is at the discretion and responsibility of the treating medical staff. There is no superior tool at present that beats a trained professional with extensive software and anatomy experience performing manual segmentation. Automatic segmentation systems through artificial intelligence are an evolving present. Eventually, automatic systems with artificial intelligence will be sufficiently accessible so that they can be used routinely (42–46).
Laser scanning has been used as an alternative mobile resource to scan extraoral surface structures, with the advantage of not irradiating patients but with the limitation of a noncolored image and limitations for open-eye scanning. Industrial-grade laser scanners were outstanding regarding trueness and precision but were costly and not easily portable given their size. Therefore, they have been replaced by other optic 3D scanning technologies that allow the acquisition of color (UV-map) and were more portable. Recently, the Lidar technique of laser usage has demonstrated potential when combined with optical resources to enhance the best of two data acquisition technologies. However, more studies are necessary to understand its cost-efficiency better (35, 47–49).
The stereophotogrammetry technique is the fastest scanning system because multiple synchronized cameras acquire all of the captures needed in a fraction of a second. It became popular over the last 20 years because of its colored facial scanning. In addition, the standardized hardware presents a low learning curve and reproducibility of its trueness and precision. However, the high-cost investment and the dedicated infrastructure and space needed for a scan rig must be considered. More simplified versions are being developed to reduce space requirements and costs (34, 50–56).
In the past decade, structured light scanners came into the facial prosthesis digital workflows as an active scanning method that creates a 3D stitching process, while the scanner is focused and displaced around the subject. As an optic resource, it produces a colored 3D model. The industry around this technology claimed to be an accessible solution due to the comparison of stereophotogrammetry systems that may cost exponentially more. However, structured light scanners were still not inexpensive enough to become a widespread technology used in most hospitals and under-resourced regions. Additionally, an intermittent flash is not comfortable for an opened eye posture of the patient. Also, the stitching process may accumulate errors in more expansive areas, creating unnoticed errors and holes in the mesh. Finally, the first structured light scanners were not calibrated for facial scanning. Designed primarily for intraoral dental applications, the optical properties may not have the optimal focal distance to obtain the most delicate details of the skin (57, 58).
Lacking the need for special equipment, monoscopic photogrammetry is the most accessible 3D facial surface scanning technique. A unique camera and specific software can be used. Smartphones and open-source software have proven their value in this workflow. When properly used, they have no limit in the computing graphic possibilities, which can manually produce professional and high-resolution 3D images for free. The consideration needed is appropriately controlling the variables with respect to protocols for precision and trueness optimization and a high learning curve to expertly operate the open-source software for rapid data manipulation and satisfactory results (31, 59–66).
Face scans with techniques such as monoscopic photogrammetry, precisely executed, are getting closer in precision and accuracy compared to tomographic methods. Even so, in cases such as the evaluation of craniofacial implants, there is an opportunity to compose 3D scans with those of surface scans. In this way, it is possible to obtain the best advantages of multiple systems and technologies in a more digital and integrated treatment. There is no single best technology for every case. It is necessary to intelligently use all the available resources that the patient and the context allow (Figure 1) (67–73).
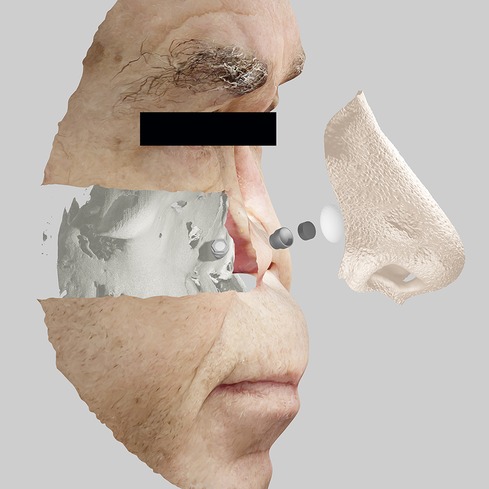
Figure 1. Integration of medical images with planning of implants, components, and prosthetic design for extraoral bucco-maxillofacial rehabilitation on implants.
3D modeling
Independent of the chosen technology for 3D facial scanning, the virtual 3D model needs to be manipulated within a CAD program. The standard tools necessary are duplicating, cutting, transforming, sculpting, and Boolean operations, which can be performed in almost any CAD software, apart from whether it is freeware like Meshmixer or high-cost commercial license software like Zbrush. Of course, previous user experience, learning curve, and user interface are individual criteria contributing to the designer's software selection. On the other hand, professional open-source software like Blender allows senior designers to take advantage of much more complex operations like modifiers, physic simulation, animations, merging, CMYK color model data in virtual reality modeling language (VRML) exportation, and others. The +Plus ID Institute programmed the first facial prosthetic design software as an add-on in Blender, which can be used for free (62, 63). Also, some algorithms are being developed to automatically detect the coloring of the facial prosthesis thanks to a deep artificial neural network approach to coloration in a facial prosthesis (74).
3D digital fabrication
Different digital manufacturing technologies have been described for facial prosthetic digital workflows, from subtractive techniques of wax, metals, and polyether ether ketone (PEEK) to additive manufacturing with fused filament fabrication (FDM), stereolithography/liquid-crystal display/digital light processing (SLA/LCD/DLP), polyjet, selective laser sintering (SLS), selective laser melting (SLM), and, more recently, silicone 3D printing (31, 63, 75–77).
FDM has been the most popular 3D printing technology since the Stratasys patent release. The thermoplastic filament is the most accessible 3D printing material that can replicate the macroanatomy of a facial structure but has a limitation on the microanatomy due to the evident layers and its staircase effect. On the other hand, all resin 3D printing technologies (SLA, LCD, DLP, Polyjet) have demonstrated their ability to reproduce the most delicate details of facial skin microanatomy characteristics (78).
Medical-grade silicone 3D printing is the most desired and expected technology consolidation. Some efforts have been made with success, although challenges still exist (79–83). However, voxel-colored Polyjet 3D printers may have a future in this realism and reliability where the +ID institute enabled the translation of color from smartphone captures into a 3D printed colored orbital prosthesis used by the patient with no complications (64).
Discussion
The future is technological and in teamwork. The ideal coming landscape for maxillofacial prosthodontists, anaplastologists, and ocularists is having worldwide opportunities for formal and accessible education. This will allow future professionals to fulfill the health system and patient needs, working together in an integrated health system with patient coverage of their advanced and accessible treatments. The next generations of 3D image acquisition systems bring an automated and self-calibrated, self-scaled 3D model that can mix more than one technology and dynamics with no high cost in a mobile and portable scenario. The next advances in 3D modeling of facial prostheses will make possible an open-source automated design created by artificial intelligence that can recognize the patient's anatomy and replace the missing part with self-created 3D meshes. The future of the 3D manufacturing process of the facial prosthesis is the final and direct 3D printed prosthesis with the high manual capacity of a gold standard exhibited by the most skilled prosthetists.
Author contributions
All authors contributed to the article and approved the submitted version.
Conflict of interest
The authors declare that the research was conducted in the absence of any commercial or financial relationships that could be construed as a potential conflict of interest.
Publisher's note
All claims expressed in this article are solely those of the authors and do not necessarily represent those of their affiliated organizations, or those of the publisher, the editors and the reviewers. Any product that may be evaluated in this article, or claim that may be made by its manufacturer, is not guaranteed or endorsed by the publisher.
References
1. Moreno F, Salazar-Gamarra R, Öhman D, Nannmark U, Pecorari V, Dib LL. Quality of life assessment of patients utilizing orbital implant-supported prostheses. Clin Implant Dent Relat Res. (2018) 20(4):438–43. doi: 10.1111/cid.12602
2. Taylor JC, Terrell JE, Ronis DL, Fowler KE, Bishop C, Lambert MT, et al. Disability in patients with head and neck cancer. Arch Otolaryngol Head Neck Surg. (2004) 130(6):764–9. doi: 10.1001/archotol.130.6.764. Available from: http://archotol.jamanetwork.com/.15210560
3. Nemli SK, Aydin C, Yilmaz H, Bal BT, Arici YK. Quality of life of patients with implant-retained maxillofacial prostheses: a prospective and retrospective study. J Prosthet Dent. (2013) 109(1):44–52. doi: 10.1016/S0022-3913(13)60010-4
4. Ariani N, Reintsema H, Ward K, Sukotjo C, Wee AG. Maxillofacial prosthodontics practice profile: a survey of non-United States prosthodontists. J Otolaryngol Head Neck Surg. (2017) 46(1):35. doi: 10.1186/s40463-017-0211-5
5. Rosen EB, Palin CL, Huryn JM, Wong RJ. The role of maxillofacial prosthetics for the surgically treated patient at national cancer institute–designated comprehensive cancer centers. Laryngoscope. (2019) 129(2):409–14. doi: 10.1002/lary.27330
6. Tetteh S, Bibb RJ, Martin SJ. Maxillofacial prostheses challenges in resource constrained regions. Disabil Rehabil. (2019) 41(3):348–56. doi: 10.1080/09638288.2017.1390697
7. de Felice F, Tombolini V, de Vincentiis M, Magliulo G, Greco A, Valentini V, et al. Multidisciplinary team in head and neck cancer: a management model. Med Oncol. (2018) 36(1):2. doi: 10.1007/s12032-018-1227-z
8. Indoe J, Lane S, Davies K, Rogers SN. Pilot of the patient concerns inventory—ward discharge in patients following major reconstructive surgery for head and neck cancer. Br J Oral Maxillofac Surg. (2021) 59(4):425–32. doi: 10.1016/j.bjoms.2020.08.080
9. Rogers SN, Allmark C, Bekiroglu F, Edwards RT, Fabbroni G, Flavel R, et al. Improving quality of life through the routine use of the patient concerns inventory for head and neck cancer patients: main results of a cluster preference randomised controlled trial. Eur Arch Otorhinolaryngol. (2021) 278(9):3435–49. doi: 10.1007/s00405-020-06533-3
10. American Academy of Maxillofacial Prosthetics. What is a maxillofacial prosthodontist? (2022). Available from: https://www.maxillofacialprosthetics.org/referring_physicians/what_is_mp/
11. Board for Certification in Clinical Anaplastology. Candidate handbook for certification in clinical anaplastology. (2013). p. 1–11. Available from: http://www.bcca-cca.com/images/PDFs/bcca_candidate_handbook.pdf.
12. The American Board of Prosthodontics. What is board certification? (2022). Available from: https://www.abpros.org/potential-candidates
13. National Examining Board of Ocularists. Becoming a board certified ocularist. (2022). Available from: https://neboboard.org/new-certification
14. Board for Certification in Clinical Anaplastology. Pathways to certification in clinical anaplastology. (2022). Available from: https://www.bcca-cca.com/pathways-to-certification
15. Chalian VA. American Academy of Maxillofacial Prosthetics. A brief history of the American Academy of Maxillofacial Prosthetics. (2022). Available from: https://www.maxillofacialprosthetics.org/about/history/.
16. International Anaplastology Association. History of anaplastology. (2022). Available from: https://www.anaplastology.org/history
17. Lepley JB. Current practices in maxillofacial prosthetics. Surg Clin North Am. (1974) 54(4):787–92. doi: 10.1016/S0039-6109(16)40381-6
19. Salazar-Gamarra R. Tecnologías 3D que nos devuelven la identidad. Perú: TED (2018). Available from: https://www.ted.com/talks/rodrigo_salazar_tecnologias_3d_que_nos_devuelven_la_identidad?language=es.
20. Salazar-Gamarra R, Oliveira JAP, Dib LL. A estética em reabilitação bucomaxilofacial. Rev APCD Estética. (2015) 03(1):42–52. doi: 10.13140/RG.2.1.4872.8729. Available from: https://www.researchgate.net/publication/297735002_Aesthetics_in_Maxillofacial_Prosthetics.
21. Mitra A, Choudhary S, Garg H, Jagadeesh HG. Maxillofacial prosthetic materials-an inclination towards silicones. J Clin Diagn Res. (2014) 8(12):ZE08–13. doi: 10.7860/JCDR/2014/9229.5244
22. Cobein MV, Coto NP, Crivello Junior O, Lemos JBD, Vieira LM, Pimentel ML, et al. Retention systems for extraoral maxillofacial prosthetic implants: a critical review. Br J Oral Maxillofac Surg. (2017) 55(8):763–9. doi: 10.1016/j.bjoms.2017.04.012
23. Beumer J, Marunick M, Esposito S. Maxillofacial rehabilitation prosthodontic and surgical management of cancer-related, acquired, and congenital defects of the head and neck. 3rd ed. Vol. 1. Bywaters L, editor. Hanover Park, IL: Quintessence (2011). p. 1–440.
24. Alvarez A. Creación e implementación de la red asistencial nacional de rehabilitación de la cara y prótesis bucomaxilofacial. (2014). Available from: http://tesis.repo.sld.cu/862/1/AlvarezRivero.pdf.
25. Zhang F, Hattori M, Sumita Y. Facial prosthesis fabricated from dental material for an exposed reconstruction plate: a clinical report. Int J Maxillofac Prosthet. (2022) 5(1):10–2. doi: 10.26629/ijmp.2022.04
26. Jiao T, Zhang F, Huang X, Wang C. Design and fabrication of auricular prostheses by CAD/CAM system. Int J Prosthodont. (2004) 17(4):460–3. PMID: 15382783
27. Chen LH, Tsutsumi S, Iizuka T. A CAD/CAM technique for fabricating facial prostheses: a preliminary report. Int J Prosthodont. (1997) 10(5):467–72. PMID: 9495166
28. Ciocca L, Fantini M, Crescenzio FD, Persiani F, Scotti R. New protocol for construction of eyeglasses-supported provisional nasal prosthesis using CAD/CAM techniques. J Rehabil Res Dev. (2010) 47(7):595. doi: 10.1682/JRRD.2009.11.0189
29. Kawabata SC. Avaliação da percepção estética de prótese óculo-palpebral confeccionada pelo método convencional e pelo sistema CAD/CAM. Biblioteca Digital de Teses e Dissertações da USP (BDTD). (2016) 80:1–80. doi: 10.11606/T.23.2016.tde-03112016-200733. Availale from: https://www.teses.usp.br/teses/disponiveis/23/23152/tde-03112016-200733/pt-br.php27477520
30. Ciocca L, de Crescenzio F, Fantini M, Scotti R. Rehabilitation of the nose using CAD/CAM and rapid prototyping technology after ablative surgery of squamous cell carcinoma: a pilot clinical report. Int J Oral Maxillofac Implants. (2010) 25(4):808–12. PMID: 20657878
31. Salazar-Gamarra R, Seelaus R, da Silva JVL, da Silva AM, Dib LL. Monoscopic photogrammetry to obtain 3D models by a mobile device: a method for making facial prostheses. J Otolaryngol Head Neck Surg. (2016) 45(1):33. doi: 10.1186/s40463-016-0145-3
32. Jauregui Ulloa J, Salazar-Gamarra R, Mesquita AMM, Aguirre F, Dib LL. A digital method to fabricate the ocular portion of an orbital prosthesis with a smartphone camera, color calibration and digital printing. J Prosthodont. (2021) 30(1):91–4. doi: 10.1111/jopr.13299
33. Salazar-Gamarra R, Moraes C, da Rosa E, Seelaus R, da Silva J, Dib LL. Multiresolution and displacement: modifier attribution to enhance realistic 3D photogrammetry for models of the face. Biomed J Sci Tech Res. (2020) 29(5):22812–7. doi: 10.26717/BJSTR.2020.29.004864. Available from: https://biomedres.us/pdfs/BJSTR.MS.ID.004864.pdf.
34. Artopoulos A, Buytaert JAN, Dirckx JJJ, Coward TJ. Comparison of the accuracy of digital stereophotogrammetry and projection moiré profilometry for three-dimensional imaging of the face. Int J Oral Maxillofac Surg. (2014) 43(5):654–62. doi: 10.1016/j.ijom.2013.10.005
35. Seelaus R, Arias E, Morris D, Cohen M. State of the art care in computer-assisted facial prosthetic rehabilitation. J Craniofac Surg. (2021) 32(3):1255–63. doi: 10.1097/scs.0000000000007530
36. Coward TJ, Scott BJJ, Watson RM, Richards R. A comparison of prosthetic ear models created from data captured by computerized tomography, magnetic resonance imaging, and laser scanning. Int J Prosthodont. (2007) 20(3):275–85. PMID: 17580460
37. Federspil PA. Implant-retained craniofacial prostheses for facial defects. GMS Curr Top Otorhinolaryngol Head Neck Surg. (2009) 8:Doc03. doi: 10.3205/cto000055
38. Jiao T, Zhu C, Dong X, Gu X. Rehabilitation of maxillectomy defects with obturator prostheses fabricated using computer-aided design and rapid prototyping: a pilot study. Int J Prosthodont. (2014) 27(5):480–6. doi: 10.11607/ijp.3733
39. Jamayet NB, Abdullah YJ, Rajion ZA, Husein A, Alam MK. New approach to 3D printing of facial prostheses using combination of open source software and conventional techniques: a case report. Bull Tokyo Dent Coll. (2017) 58(2):117–24. doi: 10.2209/tdcpublication.2016-0021
40. Ciocca L, Scotti R. Oculo-facial rehabilitation after facial cancer removal: updated CAD/CAM procedures. A pilot study. Prosthet Orthot Int. (2014) 38(6):505–9. doi: 10.1177/0309364613512368
41. Jablonski RY, Osnes CA, Khambay BS, Nattress BR, Keeling AJ. Accuracy of capturing oncology facial defects with multimodal image fusion versus laser scanning. J Prosthet Dent. (2019) 122(3):333–8. doi: 10.1016/j.prosdent.2018.10.017
42. Meinzer HP, Tolxdorff T, Lehmann TM. Advances in biomedical image analysis. Methods Inf Med. (2004) 43(4):308–14. doi: 10.1055/s-0038-1633873
43. Chung H, Ko H, Jeon SJ, Yoon KH, Lee J. Automatic lung segmentation with juxta-pleural nodule identification using active contour model and Bayesian approach. IEEE J Transl Eng Health Med. (2018) 6:1–13. doi: 10.1109/jtehm.2018.2837901
44. González Izard S, Sánchez Torres R, Alonso Plaza Ó, Juanes Méndez JA, García-Peñalvo FJ. Nextmed: automatic imaging segmentation, 3D reconstruction, and 3D model visualization platform using augmented and virtual reality. Sensors. (2020) 20(10):2962. doi: 10.3390/s20102962
45. El H, Palomo JM. Measuring the airway in 3 dimensions: a reliability and accuracy study. Am J Orthod Dentofacial Orthop. (2010) 137(4):S50–2. doi: 10.1016/j.ajodo.2010.01.014
46. Heiberg E, Sjögren J, Ugander M, Carlsson M, Engblom H, Arheden H. Design and validation of segment—freely available software for cardiovascular image analysis. BMC Med Imaging. (2010) 10:1. doi: 10.1186/1471-2342-10-1
47. Cheah Cm, Chua Ck, Tan Kh, Teo Ck. Integration of laser surface digitizing with CAD/CAM. Production. (2003) 16(4):435–41. PMID: 12956501
48. Cheah Cm, Chua Ck, Tan Kh. Integration of laser surface digitizing with CAD/CAM techniques for developing facial prostheses. Part 2: development of molding techniques for casting prosthetic parts. Int J Prosthodont. (2003) 16(5):543–8. PMID: 14651243
49. Che E, Jung J, Olsen MJ. Object recognition, segmentation, and classification of mobile laser scanning point clouds: a state of the art review. Sensors. (2019) 19:1–42. MDPI AG. doi: 10.3390/s19040810
50. Heike CL, Upson K, Stuhaug E, Weinberg SM. 3D digital stereophotogrammetry: a practical guide to facial image acquisition. Head Face Med. (2010) 6(1):18. doi: 10.1186/1746-160X-6-18
51. de Menezes M, Rosati R, Ferrario VF, Sforza C. Accuracy and reproducibility of a 3-dimensional stereophotogrammetric imaging system. J Oral Maxillofac Surg. (2010) 68(9):2129–35. doi: 10.1016/j.joms.2009.09.036
52. Dindaroǧlu F, Kutlu P, Duran GS, Görgülü S, Aslan E. Accuracy and reliability of 3D stereophotogrammetry: a comparison to direct anthropometry and 2D photogrammetry. Angle Orthodontist. (2016) 86(3):487–94. doi: 10.2319/041415-244.1
53. Winder RJ, Darvann TA, McKnight W, Magee JDM, Ramsay-Baggs P. Technical validation of the Di3D stereophotogrammetry surface imaging system. Br J Oral Maxillofac Surg. (2008) 46(1):33–7. doi: 10.1016/j.bjoms.2007.09.005
54. Plooij JM, Swennen GRJ, Rangel FA, Maal TJJ, Schutyser FAC, Bronkhorst EM, et al. Evaluation of reproducibility and reliability of 3D soft tissue analysis using 3D stereophotogrammetry. Int J Oral Maxillofac Surg. (2009) 38(3):267–73. doi: 10.1016/j.ijom.2008.12.009
55. Ceinos R, Tardivo D, Bertrand MF, Lupi-Pegurier L. Inter- and intra-operator reliability of facial and dental measurements using 3D-stereophotogrammetry. J Esthet Restor Dent. (2016) 28(3):178–89. doi: 10.1111/jerd.12194
56. Verhoeven TJ, Coppen C, Barkhuysen R, Bronkhorst EM, Merkx MAW, Bergé SJ, et al. Three dimensional evaluation of facial asymmetry after mandibular reconstruction: validation of a new method using stereophotogrammetry. Int J Oral Maxillofac Surg. (2013) 42(1):19–25. doi: 10.1016/j.ijom.2012.05.036
57. Tsuchida Y, Shiozawa M, Handa K, Takahashi H, Nikawa H. Comparison of the accuracy of different handheld-type scanners in three-dimensional facial image recognition. J Prosthodont Res. (2022):JPR_D_22_00001. doi: 10.2186/jpr.JPR_D_22_00001. Available from: https://www.jstage.jst.go.jp/article/jpr/advpub/0/advpub_JPR_D_22_00001/_article/-char/en
58. Unkovskiy A, Spintzyk S, Beuer F, Huettig F, Röhler A, Kraemer-Fernandez P. Accuracy of capturing nasal, orbital, and auricular defects with extra- and intraoral optical scanners and smartphone: an in vitro study. J Dent. (2022) 117:103916. doi: 10.1016/j.jdent.2021.103916
59. Salazar Gamarra R, Moraes CADC, da Rosa ELS, Seelaus R, da Silva JVL, Dib L. Multiresolution and displacement: modifier attribution to enhance realistic 3D photogrammetry for models of the face. Biomed J Sci Technol Res. (2020) 29(5):22812–7. doi: 10.26717/BJSTR.2020.29.004864
60. Moraes C, Sobral D, Duarte D, Cavalcanti G, Salazar-Gamarra R, Dornelles R. Protocolo complementar para melhor resolução do nariz em fotogrametria 3D. In: OrtogOnLineMag. São Paulo, Brasil (2020). Available from: http://ortogonline.com/doc/pt_br/OrtogOnLineMag/1/Nariz.html#id1
61. Dornelles RdF, Alonso N. New virtual tool for accurate evaluation of facial volume. Acta Cir Bras. (2017) 32(12):1075–86. doi: 10.1590/s0102-865020170120000009
62. Falih MR, Abed FM, Salazar-Gamarra R, Dib LL. Efficiency of +ID on Blender photogrammetric tool in facial prosthetics rehabilitation—an evaluation study. Karbala Int J Mod Sci. (2021) 7:421–36. doi: 10.33640/2405-609X.3167
63. Salazar-Gamarra R, Cárdenas-Bocanegra A, Masch U, da Costa Moraes CA, Seelaus R, Lopes Da Silva JV, et al. Color translation from monoscopic photogrammetry +ID methodology into a Polyjet final 3D printed facial prosthesis. F1000Res. (2022) 11:582. doi: 10.12688/f1000research.111196.1
64. Gallardo YNR, Salazar-Gamarra R, Bohner L, de Oliveira JI, Dib LL, Sesma N. Evaluation of the 3D error of 2 face-scanning systems: An in vitro analysis. J Prosthet Dent. (2021) S0022-3913(21):00368-1. doi: 10.1016/j.prosdent.2021.06.049
65. Elbashti MG, Sumita Y, Aswehlee A, Seelaus R. Smartphone application as a low-cost alternative for digitizing facial defects: is it accurate enough for clinical application? Int J Prosthodont. (2019) 32(6):541–3. doi: 10.11607/ijp.6347
66. Aswehlee AM, Elbashti ME, Hattori M, Sumita YI, Taniguchi H. Feasibility and accuracy of noncontact three-dimensional digitizers for geometric facial defects: an in vitro comparison. Int J Prosthodont. (2018) 31(6):601–6. doi: 10.11607/ijp.5855
67. Ayoub AF, Xiao Y, Khambay B, Siebert JP, Hadley D. Towards building a photo-realistic virtual human face for craniomaxillofacial diagnosis and treatment planning. Int J Oral Maxillofac Surg. (2007) 36(5):423–8. doi: 10.1016/j.ijom.2007.02.003
68. Khambay B, Nebel JC, Bowman J, Walker F, Hadley DM, Ayoub A. 3D Stereophotogrammetric image superimposition onto 3D CT scan images: the future of orthognathic surgery. A pilot study. Int J Adult Orthodon Orthognath Surg. (2002) 17(4):331–41. PMID: 12593005
69. Hajeer MY, Ayoub AF, Millett DT, Bock M, Siebert JP. Three-dimensional imaging in orthognathic surgery: the clinical application of a new method. Int J Adult Orthodon Orthognath Surg. (2002) 17(4):318–30. PMID: 12593004
70. Naudi K, Benramadan R, Brocklebank LM, Xiangyang J, Balvinder K, Ashraf A. The virtual human face: superimposing the simultaneously captured 3D photorealistic skin surface of the face on the untextured skin image of the CBCT scan. Int J Oral Maxillofac Surg. (2013) 42(3):393–400. doi: 10.1016/J.IJOM.2012.10.032
71. Lin CY, Hsung TC, Khambay B. Reducing cone beam CT scan height as a method of radiation reduction for photorealistic three-dimensional orthognathic planning. J Craniomaxillofac Surg. (2015) 43(6):907–12. doi: 10.1016/j.jcms.2015.04.002
72. Mathys A, Brecko J, Semal P. Comparing 3D digitizing technologies: what are the differences? In Proceedings of the 2013. Digital Heritage International Congress (DigitalHeritage), Vol. 1. Marseille, France: IEEE (2013). p. 201–4. doi: 10.1109/digitalheritage.2013.6743733
73. Tzou CH, Artner NM, Pona I, Hold A, Placheta E, Kropatsch WG, et al. Comparison of three-dimensional surface-imaging systems. J Plast Reconstr Aesthet Surg. (2014) 67(4):489–97. doi: 10.1016/j.bjps.2014.01.003
74. Mine Y, Suzuki S, Eguchi T, Murayama T. Applying deep artificial neural network approach to maxillofacial prostheses coloration. J Prosthodont Res. (2020) 64(3):296–300. doi: 10.1016/j.jpor.2019.08.006
75. Xu Y, Unkovskiy A, Klaue F, Rupp F, Geis-Gerstorfer J, Spintzyk S. Compatibility of a silicone impression/adhesive system to FDM-printed tray materials—a laboratory peel-off study. Materials. (2018) 11(10):1–12. doi: 10.3390/ma11101905
76. Kamio T, Hayashi K, Onda T, Takaki T, Shibahara T, Yakushiji T, et al. Utilizing a low-cost desktop 3D printer to develop a “one-stop 3D printing lab” for oral and maxillofacial surgery and dentistry fields. 3D Print Med. (2018) 4(1):1–2. doi: 10.1186/s41205-018-0028-5
77. He Y, Xue GH, Fu JZ. Fabrication of low cost soft tissue prostheses with the desktop 3D printer. Sci Rep. (2014) 4:1–7. doi: 10.1038/srep06973
78. Eyzaguirre D, Salazar-Gamarra R, Binasco Lengua S, Lauria Dib L. Evaluation of additive manufacturing processes in the production of oculo-palpebral prosthesis. F1000Res. (2022) 11:505. doi: 10.12688/f1000research.111231.1
79. Jindal SK, Sherriff M, Waters MG, Smay JE, Coward TJ. Development of a 3D printable maxillofacial silicone: part II. Optimization of moderator and thixotropic agent. J Prosthet Dent. (2018) 119(2):299–304. doi: 10.1016/j.prosdent.2017.04.028
80. Zhou LY, Gao Q, Fu JZ, Chen QY, Zhu JP, Sun Y, et al. Multimaterial 3D printing of highly stretchable silicone elastomers. ACS Appl Mater Interfaces. (2019) 11(26):23573–83. doi: 10.1021/acsami.9b04873
81. Jindal SK, Sherriff M, Waters MG, Coward TJ. Development of a 3D printable maxillofacial silicone: part I. Optimization of polydimethylsiloxane chains and cross-linker concentration. J Prosthet Dent. (2016) 116(4):617–22. doi: 10.1016/j.prosdent.2016.02.020
82. Unkovskiy A, Wahl E, Huettig F, Keutel C, Spintzyk S. Multimaterial 3D printing of a definitive silicone auricular prosthesis: an improved technique. J Prosthet Dent. (2020) 125(6):946–50. doi: 10.1016/j.prosdent.2020.02.021
Keywords: maxillofacial prosthodontics, facial prosthetics, 3D technologies, oral cancer, head and neck cancer, anaplastology
Citation: Salazar-Gamarra R, Binasco S, Seelaus R and Dib LL (2022) Present and future of extraoral maxillofacial prosthodontics: Cancer rehabilitation. Front. Oral. Health 3:1003430. doi: 10.3389/froh.2022.1003430
Received: 26 July 2022; Accepted: 12 September 2022;
Published: 19 October 2022.
Edited by:
Wilfredo Alejandro González-Arriagada, University of the Andes, ChileReviewed by:
Sohaib Shujaat, KU Leuven, BelgiumMariko Hattori, Tokyo Medical and Dental University, Japan
© 2022 Salazar-Gamarra, Binasco, Seelaus and Dib. This is an open-access article distributed under the terms of the Creative Commons Attribution License (CC BY). The use, distribution or reproduction in other forums is permitted, provided the original author(s) and the copyright owner(s) are credited and that the original publication in this journal is cited, in accordance with accepted academic practice. No use, distribution or reproduction is permitted which does not comply with these terms.
*Correspondence: Rodrigo Salazar-Gamarra rodrigo.salazar@uwiener.edu.pe
Specialty Section: This article was submitted to Oral Cancers, a section of the journal Frontiers in Oral Health