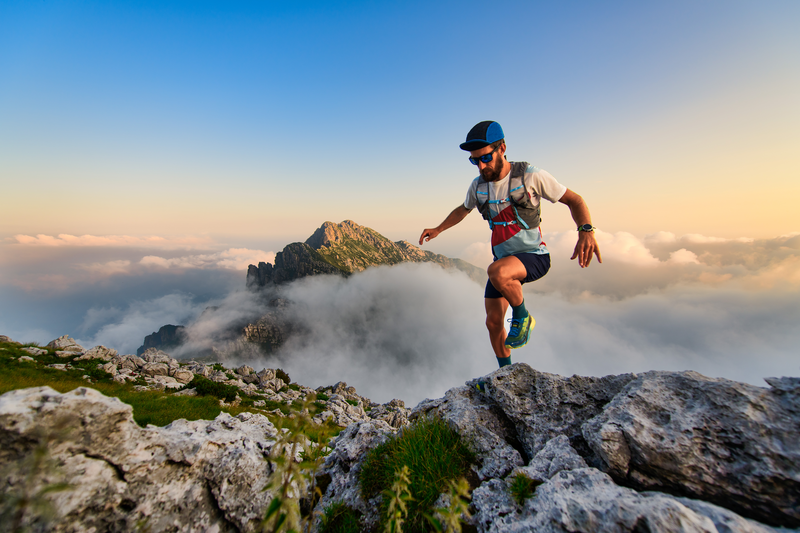
94% of researchers rate our articles as excellent or good
Learn more about the work of our research integrity team to safeguard the quality of each article we publish.
Find out more
ORIGINAL RESEARCH article
Front. Ophthalmol. , 14 June 2023
Sec. Retina
Volume 3 - 2023 | https://doi.org/10.3389/fopht.2023.1202445
Background: To assess and compare choroidal morphometric vascular parameters, using optical coherence tomographic angiography (OCTA), in highly myopic adults with and without myopic macular degeneration (MMD).
Methods: This is a clinic-based observational study of 148 eyes with axial length (AL) ≥25mm, enrolled from the high myopia clinic of the Singapore National Eye Centre. MMD was graded from fundus photographs. Swept source OCT (SS-OCT) and OCTA were performed and assessed for choroidal layer thickness (CT) and choroidal vasculature (choroidal vessel density (CVD), choroidal branch area (CBA) and mean choroidal vessel width (MCVW)) in the different choroidal layers (overall choroidal layer (CL), medium-vessel choroidal layer (MVCL), large-vessel choroidal layer (LVCL)).
Results: CTCL (r=-0.58, p<0.001), CTMVCL (r=-0.22, p=0.04), MCVWCL (r=-0.58, p<0.001), and CVDCL (r=-0.19, p=0.02) were negatively correlated with AL, while CBACL (r=0.61, p<0.001) was positively correlated. Compared to eyes with no MMD, eyes with MMD2 had lower CTCL (120.37±47.18µm vs 218.33±92.70µm, p<0.001), CTMVCL (70.57±15.28µm vs 85.32±23.71µm, p=0.04), CTLVCL (101.65±25.36µm vs 154.55±68.41µm, p=0.001) and greater CVDCL (71.10±3.97% vs 66.97±3.63%, p<0.001), CVDMVCL (66.96±2.35% vs 65.06±2.69%, p=0.002), CVDLVCL (68.36±2.56% vs 66.58±2.88%, p=0.012), MCVWMVCL (6.14±0.34µm vs 5.90±0.35µm, p=0.007), and CBACL (12.69±1.38% vs 11.34±1.18%, p<0.001). After adjusting for age, thicker CTCL (odds ratio (OR) 0.98, 95% confidence interval (CI) 0.97-0.99, p<0.001), CTMVCL (OR 0.97 (0.94-0.99), p=0.002) and CTLVCL (OR 0.97 (0.96-0.98, p<0.001) were significantly associated with lower odds of MMD2, while increased CVDCL (OR 1.37 (1.20-1.55), p<0.001), CVDMVCL (OR 1.39 (1.12-1.73), p=0.003), CVDLVCL (OR 1.31 (1.07-1.60), p=0.009), CBACL (OR 2.19 (1.55-3.08), p<0.001) and MCVWMVCL (OR 6.97 (1.59-30.51), p=0.01) was significantly associated with higher odds of MMD2.
Conclusion: Decrease in choroidal vessel width, density and thickness, and an increase in vascular branching were observed in eyes with long AL. A thinner and denser choroid with greater branching area and vessel width, which may all be signs of hypoxia, were associated with greater odds of MMD2.
Myopic macular degeneration (MMD) is a major cause of visual impairment and blindness globally. As high myopia and age are the major risk factors for MMD, an epidemic of MMD is expected in the near future, especially in East Asian countries where the prevalence of high myopia is 7% to 22% (1–3). Although our knowledge of the pathologic changes in high myopia has advanced since the past decade, a lot is still unknown about the pathogenesis of MMD. One of the unmet needs in the clinical management of high myopia lies in predicting those who are at the greatest risk of MMD progression. This may now be possible with advances in imaging technology that have enabled the in vivo study of the retina/choroid/sclera complex, some or all of which are likely to be the primary protagonists in the pathogenesis of MMD (1, 4–6).
The advent of optical coherence tomographic angiography (OCTA) enabled imaging of the choroidal vasculature non-invasively, making it possible to assess the choroid beyond the single dimension of choroidal thickness. Our group had previously utilized swept source optical coherence tomography (SS-OCT) to identify decreased choroidal thickness (CT) as a key feature in MMD, and demonstrated that CT was more strongly associated with MMD than scleral thickness (7). This finding suggests that analysis of the choroidal layer may provide further clues to the pathogenesis of MMD. However, it was still unclear if choroidal thinning in MMD stems from atrophy of the choroidal vasculature or stroma.
OCTA remains limited in the visualization of the deeper vascular layers of the choroid, mostly because of scattering by the pigment in the RPE and by vessels in the choriocapillaris (CC), and consequent signal attenuation (8). Moreover, the CC produces strong OCTA projection artifacts in the underlying choroid. Because of the signal attenuation, choroidal vessels are typically visualized as silhouettes with complete loss of signal at greater depths. Consistent visualization of the medium and larger-sized vessels of Sattler’s and Haller’s layers may be better appreciated in eyes with areas of overlying RPE loss or depigmentation with absence of the CC, permitting greater penetration of light into the choroid.
Currently, these limitations have been partially overcome by using ultrahigh-speed SS-OCTA instruments (9). Recently, the choroid has been imaged with a novel research device with a Fourier-domain mode-locked laser system operating with a 1.7- MHz sweep rate (1.7 million A-scans/s), at a 1065-nm center wavelength. In a recent study, this device was used to obtain OCTA images with a phase-variance method and these images were qualitatively compared with those obtained with an SD-OCTA instrument operating with a 68 kHz imaging rate and an 840-nm center wavelength (10). In this comparison, the authors noted an improved visualization of the medium and larger-sized choroidal vessels in the images obtained using the SS-OCTA with increased imaging speed. Notably, the visualization of the choroid with this device was demonstrated to be even better than that obtained with ICGA, since the latter imaging modality does not resolve the meshwork of capillary vessels and has limited capability to visualize the medium-sized vessels. However, given these limitations in the visualization of the deeper choroidal vascular layers using existing commercial instruments, most OCTA studies thus far have focused on the CC, providing the greatest amount of information for this thin dense layer of capillaries, both in healthy and affected eyes.
Similarly in one of our follow-up study, we demonstrated qualitatively the presence of choriocapillaris signal voids on OCTA in eyes with MMD (11). The degree of choriocapillaris signal voids was more extensive than that indicated by the severity of MMD, suggesting that OCTA evaluation of the CC may detect pathological changes before the onset of clinically apparent atrophy. We postulated that choriocapillaris changes suggest that the pathogenesis of MMD may be related to the Bruch’s membrane, which is essentially the basement membrane of the choriocapillaris. However, we did not evaluate changes in the deeper layers of the choroid, namely the Sattler’s and Haller’s layers, and whether vascular changes in these deeper layers could be the cause of alterations in the choriocapillaris. To address this, our group developed a novel segmentation technique correlating OCTA images and the anatomical OCT structure of the choroid that allowed the detailed vascular analysis of Haller’s and Sattler’s layers in high myopes (11).
In this study, we aim to evaluate changes in the medium and large choroidal vessel layers of high myopes with MMD using the aforementioned technique.
This was a clinic-based observational clinical study in which patients with high myopia aged 21-70 years, with manifest spherical equivalent (SE) of ≤ -5.0 dioptres (D) or axial length (AL) of ≥ 25mm in either eye, were recruited from the High Myopia clinic of the Singapore National Eye Centre from January 2017 to December 2017. Both eyes of a patient may be included for analysis if the eye has a SE of ≤ -5.0 D or AL of ≥ 25mm. Patients with any coexisting or previous ocular disease in either eye, including corneal opacities, uveitis, dense cataracts, vitreous haemorrhage, diabetic retinopathy or diabetic macular edema, central serous chorioretinopathy, previous retinal laser photocoagulation or photodynamic therapy, previous and coexisting retinal detachment, retinal dystrophies, macular scarring from any cause other than myopic maculopathy, retinopathy due to any cause other than myopia, previous retinal vein or artery occlusion, and ocular ischemic syndrome were excluded from this study. This study was approved by the Centralized Institutional Review Board (CIRB) of SingHealth, Singapore (protocol number R1364/50/2016) and conducted in accordance with the Declaration of Helsinki. All participants provided signed informed consent for their participation.
All patients underwent comprehensive ophthalmic evaluation including best corrected visual acuity (BCVA), measured with the logarithm of the minimal angle of resolution (logMAR) chart (Lighthouse International, New York, New York, USA) at 4 meters, slit lamp biomicroscopy examination, and manifest refraction performed by certified study optometrists. Intraocular pressure was measured with Goldmann applanation tonometry. Spherical equivalent (SE) was calculated as the sum of the spherical power and half of the cylindrical power. The IOL Master (Carl Zeiss Meditec AG, Jena, Germany) was used for ocular biometry including AL, anterior chamber depth (ACD) and keratometry measurements.
Fundus photography centered at the fovea was performed with a 45-degree digital retinal camera after pupillary dilation with tropicamide 1% and phenylephrine 2.5%, using Canon CR-DGi with Canon EOS 10D SLR backing (Canon Inc, Tokyo, Japan). The optical coherence tomography (OCT) images were obtained using a swept source OCT device (Topcon DRI OCT Triton, Topcon, Tokyo, Japan). This instrument uses a light source with a central wavelength of 1050 nm and an acquisition speed of 100,000 A scans per second. 12mm raster and radial scans centered on the macula and optic nerve were obtained. Fundus photographs and OCT images were graded by 2 retinal specialists (CWW, QVH) for retinal and optic nerve lesions.
Two retinal specialists (CWW, QVH), masked to participant characteristics performed grading of the fundus photographs using the International Meta-Analysis for Pathologic Myopia classification.
3mm×3mm images from the Plex Elite (PLEX Elite, Carl Zeiss Meditec, Dublin, CA) were used for the analysis of choroidal layers. The highest-quality foveal cut of the OCT B-scans was chosen by two experienced fellowship-trained retinal specialists (CWW and QVH) for choroidal thickness grading. Only one highest-quality B-scan, closest to the foveal center was used for segmentation. The choroid was manually segmented by two masked observers, with excellent reliability (ICC = 0.987) into overall choroidal layer (CL), medium-vessel choroidal layer (MVCL), and large-vessel choroidal layer (LVCL) according to the technique described by Devarajan et al., 2019 (11). Using the PLEX Elite software, the choriocapillaris layer presented with default segmentation lines close to the Bruch’s membrane (Figure 1). CL was segmented by adjusting the top segmentation line to the Bruch’s membrane – for images where the default segmentation lines are at the wrong position – and moving the bottom segmentation line to the suprachoroid, which is indicated by the lowest visible large vessel (Figure 2). MVCL was segmented next by moving the bottom segmentation line above the highest point of the hyporeflective large vessels, leaving MVCL with a hyperreflective appearance comprising of the small and medium vessels of the Sattler’s layer (Figure 3). LVCL segmentation was performed by subtracting MVCL from CL, giving this layer the appearance of only hyporeflective large vessels of the Haller’s layer (Figure 4). The thickness of each layer was calculated by subtracting the value of the top segmentation line from the bottom segmentation line (in µm). Eyes with overall choroidal thickness less than 100 µm were excluded as it was not possible to have reliable segmentation of the choroid into the MVCL and LVCL sublayers.
Figure 1 Example of choriocapillaris layer marked by default segmentation lines (purple dashed lines).
Figure 2 Manual segmentation of overall choroidal layer (CL). Top segmentation line (top purple dashed line) sits at the innermost border of the choroidal layer, while the bottom segmentation line (lower purple dashed line) was shifted to the outermost large choroidal vessel, marking the CL as the layer between the purple segmentation lines.
Figure 3 Manual segmentation of medium-vessel choroidal layer (MVCL). Top segmentation line (top purple dashed line) sits at the innermost border of the choroidal layer, while the bottom segmentation line (lower purple dashed line) was shifted to the innermost large choroidal vessel, marking the MVCL between the purple dashed segmentation lines.
Figure 4 Manual segmentation of large-vessel choroidal layer (LVCL). Top segmentation line (top purple dashed line) sits at the innermost large choroidal vessel, while the bottom segmentation line (lower purple dashed line) was shifted to the outermost large choroidal vessel, marking the LVCL between the purple dashed segmentation lines.
Enface images obtained from segmenting the different layers were compiled and processed using Matlab as described by Devarajan et al., 2019 (11) for choroidal vasculature parameters: choroidal vessel density (CVD, %), choroidal branch area (CBA, %), and mean choroidal vessel width (MCVW, µm) see Figure 5.
Figure 5 Examples of enface images for (A) overall choroidal layer (CL), (B) medium vessel choroidal layer (MVCL), and (C) large vessel choroidal layer (LVCL). These images were subsequently processed with Matlab for choroidal vasculature parameters: choroidal vessel density (CVD), choroidal branch area (CBA), and mean choroidal vessel width (MCVW).
A total of 12 choroidal vascular parameters were obtained: overall choroidal layer thickness (CTCL), medium-vessel choroidal layer thickness (CTMVCL), large-vessel choroidal layer thickness (CTLVCL), choroidal vessel density – overall choroidal layer (CVDCL), choroidal vessel density – medium-vessel choroidal layer (CVDMVCL), choroidal vessel density – large-vessel choroidal layer (CVDLVCL), choroidal branch area – overall choroidal layer (CBACL), choroidal branch area – medium-vessel choroidal layer (CBAMVCL), choroidal branch area – large-vessel choroidal layer (CBALVCL), mean choroidal vessel width – overall choroidal layer (MCVWCL), mean choroidal vessel width – medium-vessel choroidal layer (MCVWMVCL), and mean choroidal vessel width – large-vessel choroidal layer (MCVWLVCL).
All data were expressed as mean ± standard deviation or proportions as appropriate. Means were compared with the two-sample Kolmogorov-Smirnov test. Binary logistic regression analysis was performed to identify independent factors associated with poorer visual acuity, adjusted for age. Each individual’s left and right eye was accounted for as fixed effects. Each choroidal morphometric vascular parameter was corrected for magnification error calculated using Littman’s method and the Bennett formula, F = 3.48 × 0.01306 × (AL – 1.82), where F represents the magnification factor and AL represents axial length. A p-value of <0.05 was considered to be statistically significant. All statistical analyses were performed using Stata/MP 16.1 (College Station, TX 77845, USA).
In the original cohort, there were very few eyes that were META-PM category 3 or 4 (five and two, respectively). In addition, we were also unable to segment the choroid into sublayers for such eyes with very thin choroids. We therefore excluded the META-PM category 3 and 4 eyes. A total of 148 eyes were included and were split into two groups for analysis, with 74 eyes in the MMD2 group and 74 eyes in the no MMD group.
Patients with MMD2 were significantly older (56.4 ± 12.0 vs. 51.2 ± 12.2 years, p = 0.009) and had longer AL (29.0 ± 1.6 vs. 27.4 ± 1.4 mm, p < 0.001) than those without see Table 1.
Age and 12 choroidal vascular parameters were analysed for any significant correlation with AL, BCVA and CT. Longer AL was associated with thinner CTCL (r = -0.58, p < 0.001), thinner CTMVCL (r = -0.22, p = 0.04), lower CVDCL (r = -0.19, p = 0.02), reduced MCVWCL (r = -0.58, p < 0.001), and greater CBACL (r = 0.61, p < 0.001). Poorer BCVA was associated with thinner CTCL (r = -0.21, p = 0.01). Thinner CTCL was also associated with older age (r = -0.38, p < 0.001), and greater CBACL (r = -0.60, p < 0.001) with an equal increase in CBAMVCL and CBALVCL (r = -0.22, p = 0.04 for both). Thinner CTCL was also associated with thinner CTMVCL (r = 0.79, p < 0.001) and CTLVCL (r = 0.98, p < 0.001), decreased CVDCL (r = 0.38, p < 0.001) with significant decrease in CVDLVCL (r = 0.41, p < 0.001), and reduced MCVWCL (r = 0.61, p < 0.001) also with significant decrease in MCVWLVCL (r = 0.27, p = 0.01) but the changes for CVDMVCL and MCVWMVCL were not considered as significant (p ≥ 0.05) (Table 2).
Comparison of the choroidal vascular parameters between eyes with MMD2 and with no MMD revealed that eyes with MMD2 have thinner CTCL (120.37±47.18µm vs 218.33±92.70µm, p<0.001), along with thinner CTMVCL (70.57±15.28µm vs 85.32±23.71µm, p=0.04) and CTLVCL (101.65±25.36µm vs 154.55±68.41µm, p=0.001). But had greater CVDCL (71.10±3.97% vs 66.97±3.63%, p<0.001), CVDMVCL (66.96±2.35% vs 65.06±2.69%, p=0.002), CVDLVCL (68.36±2.56% vs 66.58±2.88%, p=0.012), MCVWMVCL (6.14±0.34µm vs 5.90±0.35µm, p=0.007), and greater CBACL (12.69±1.38% vs 11.34±1.18%, p<0.001) in eyes with MMD2 (Table 3).
Binary logistic regression, prior to adjusting for age (unadjusted odds ratio), revealed thicker CTCL (odds ratio (OR) 0.98, 95% confidence interval (CI) 0.97-0.98, p < 0.001), thicker CTMVCL (OR 0.96, 95% CI 0.94-0.99, p = 0.001) and thicker CTLVCL (OR 0.97, 95% CI 0.96-0.98, p < 0.001) were associated with lower odds of MMD2. Presence of MMD2 was associated with greater odds of increased CVDCL (OR 1.35, 95% CI 1.20-1.53, p < 0.001), increased CVDMVCL (OR 1.34, 95% CI 1.10-1.63, p = 0.004), increased CVDLVCL (OR 1.26, 95% CI 1.05-1.51, p = 0.01), increased CBACL (OR 2.25, 95% CI 1.60-3.17, p < 0.001) and increased MCVWMVCL (OR 6.79, 95% CI 1.53-30.22, p = 0.01). After adjusting for age (adjusted odds ratio), thicker CTCL (OR 0.98, 95% CI 0.97-0.99, p < 0.001), thicker CTMVCL (OR 0.97, 95% CI 0.94-0.99, p = 0.002) and thicker CTLVCL (OR 0.97, 95% CI 0.96-0.98, p < 0.001) remained associated with lower odds of MMD2. Presence of MMD2 remained significantly associated with greater odds of increased CVDCL (OR 1.37, 95% CI 1.20-1.55, p < 0.001), increased CVDMVCL (OR 1.39, 95% CI 1.12-1.73, p = 0.003), increased CVDLVCL (OR 1.31, 95% CI 1.07-1.60, p = 0.009), increased CBACL (OR 2.19, 95% CI 1.55-3.08, p < 0.001) and increased MCVWMVCL (OR 6.97, 95% CI 1.59-30.51, p = 0.01) (Table 4).
Consistent with previous studies (12–14), our study found a strong correlation between AL and CT, where the longer the AL, the thinner the CTCL (r = -0.58, p < 0.001). Besides CTCL, CVDCL (r = -0.19, p = 0.02) and MCVWCL (r = -0.58, p < 0.001) were also noted to decrease with longer AL, while CBACL increases with longer AL (r = 0.61, p < 0.001). None of the parameters for the middle- and large-vessel choroidal layers, except for CTMVCL (r = -0.22, p = 0.04) were significantly associated with changes in AL. Other investigators had noted similar findings for the negative correlation for CTCL, CVDCL and MCVWCL with AL, and positive correlation for CBACL with AL (15–18). Taken together, these findings suggest that the increase in AL could have exerted a stretching force on the choroidal vessels, thereby decreasing choroidal vessel width. With the decrease in vessel width, there would be a decrease in blood flow which would affect the choroidal thermoregulation (19). Thus, this could have led to the increase in CBA, which could be an increase in choroidal vascular branching or elongation or both, in an attempt by the eye to regulate the retinal/choroidal blood supply, similar to the mechanism where choroidal neovascularisation (CNV) occurs. Interestingly, blood flow in choroidal vessels has been reported to be lower in highly myopic versus non-highly myopic eyes, with a possible negative correlation between axial length and choroidal blood flow (20). This supports the idea that the thinning of MCVW could have led to a noticeable decrease in blood flow and vessel branching increase could be a compensatory mechanism to overcome the limitation in blood flow. Further investigation is necessary to verify if the molecular mechanisms of choroidal vessel changes in elongated, pathological eyes have any similarities to CNV.
Thinner CTCL was also associated with worse BCVA (r = -0.21, p = 0.01), older age (r = -0.38, p < 0.001), and thinner CTMVCL and CTLVCL (r = 0.79 and r = 0.98, p < 0.001 for both), mirroring what several other groups had observed (12–14, 21, 22). CVDCL was also noted to be positively correlated with CTCL (r = 0.38, p < 0.001), with the changes in CVDLVCL being the main driver (r = 0.41, p < 0.001). Wang et al. (2020) similarly noted that CVDLVCL was larger in patients with central serous chorioretinopathy (CSC) as compared to controls (62.3 ± 6.6% vs. 54.1 ± 8.0%, p = 0.02) (23). Although these eyes were affected by a different disease, it seems that regardless of the type of disease, CVDLVCL appears to play a more impactful role than CVDMVCL for changes in the overall choroidal layer.
Our understanding of the changes in CVD could be further broken down through understanding the changes for CBA and MVCW, with MCVWCL (r = 0.61, p < 0.001) and MCVWLVCL (r = 0.27, p = 0.01) noted to have a significant positive correlation with changes in CT as well. Together with the results for CVD, the large-vessel choroidal layer appears to be a more active player in driving changes for the overall choroidal layer than the medium-vessel choroidal layer as the correlation for CVDMVCL was not significant (p = 0.86) and MCVWMVCL was marginally significant (p = 0.06). For CBACL (r = -0.60, p < 0.001), it was negatively correlated with CTCL, with equal contributions of both MVCL and LVCL (r = -0.22, p = 0.04 for both) affecting the increase in the choroidal branch area. From the changes in CBA and CVD, it seemed that changes for MCVW were probably larger than that of the choroidal branching (which could either be an increase in vessel branching or increased length of vessels) to result in overall changes in CVD to be similarly negatively correlated with CT changes.
Several choroidal vasculature parameters that we studied (CTCL, CTMVCL, CTLVCL, CVDCL, CVDMVCL, CVDLVCL, CBACL, and MCVWMVCL) were significantly altered in the presence of MMD2 (Table 3). Compared to eyes with no MMD, MMD2 eyes showed thinner CTCL (120.37µm vs 218.33µm, p < 0.001), which was consistent for both MVCL (70.57µm vs 85.32µm, p = 0.04) and LVCL (101.65µm vs 154.55µm, p=0.001). This finding is similar to other studies, in which Wong et al. noted that CT was highly correlated to MMD (7), and Zhou et al. similarly noted that CT is thinner in more myopic eyes (24). Meanwhile, Zhao et al. also found that MVCL was only significantly associated with a higher prevalence of some, and not all, macular diseases (14).
Interestingly, it was noted by Yang and Koh that impediment of ocular blood was possibly associated with axial length increase, especially so in high myopes (20). In the present study, we find three choroidal vascular parameters that may reflect worsened hypoxia (Table 3). For example, compared to eyes with no MMD, choroidal vessel density was consistently higher in MMD2 eyes for CVDCL (71.10 vs. 66.97%, p < 0.001) as well as for CVDMVCL and CVDLVCL (p = 0.002 and p = 0.012). Moreover, MCVWMVCL was greater in MMD2 eyes versus eyes with no MMD (6.14 vs. 5.90 µm, p = 0.007). Additionally, the choroidal branching area (CBACL) was significantly greater in eyes with MMD2 (12.69 vs. 11.34%, p < 0.001). As discussed further below, hypoxia, specifically in the choriocapillaris layer could readily result in a compensatory response in medium- as well as the large-vessel layers in attempts improve perfusion with increased vessel density, increased vessel width and increased branching area.
Thicker CTCL, with significant contributions from both MVCL and LVCL, were significantly associated with lower odds of MMD2, after adjusting for age (OR 0.98, 95% CI 0.97-0.99, p < 0.001; OR 0.97, 95% CI 0.94-0.99, p = 0.002; OR 0.97, 95% CI 0.96-0.98, p < 0.001, respectively). As mentioned above, a compensatory response to hypoxia in the choriocapillaris likely explains our finding that presence of MMD2 increases the odds of higher CVD (of CL, MVCL and LVCL), CBACL and MCVWMVCL. Another possible explanation for the increase in CVD could be the increased risk of myopic complications in MMD eyes. MMD eyes are known to have a higher risk of developing posterior staphylomas and myopic CNV, with both features being associated with pronounced choroidal thinning (19, 25, 26). Limitations in discerning the individual choroidal layers within staphyloma eyes leave open the possibility of an increased CVD within the MVCL and LVCL as some sort of defense mechanism which the eye could have taken to prevent staphyloma formation/progression. As the choriocapillaris is not readily resolvable in standard OCTA imaging, it could very well be the changes in the MVCL and LVCL mask changes occurring in the much thinner choriocapillaris, which would contribute relatively less to the overall change in the CL. Also, as it is currently unknown which choroidal layer myopic neovascularization originates from (19), we cannot eliminate the possibility of mCNV originating from the MVCL or LVCL versus the choroicapillaris. With overall thinning of the choroid in MMD eyes, the increase in CVDMVCL and CVDLVCL could be a defensive mechanism where the choroid tries to regulate choroidal blood flow to prevent hypoxia. Similarly, an increase in vessel width (MCVWMVCL) could be a similar defense mechanism. Moreover, past studies have suggested that a response to hypoxia could be increased vessel branching (27). Increased branching would result in increased CVD. Hypoxia may very well explain why the presence of MMD increases the odds of greater CBA, CVD and MCVW.
Our study is not without its limitations, some of which are discussed above. In the original cohort, there were very few eyes that were META-PM category 3 or 4 (five and two, respectively). In addition, we were also unable to segment the choroid into the MVCL and LVCL sublayers for such eyes which would have extremely thin choroids. We therefore excluded the META-PM category 3 and 4 eyes. Hence, this study was not designed to assess the effect of MMD severity, and we could only assess the effect of its presence. Furthermore, our study has a cross-sectional design and this precludes the inference of a temporal or sequential relationship. In this regard, we cannot tell which choroidal layer the main changes in axial elongation occur in. Moreover, we did not account for chronic conditions such as diabetes, kidney disease and hypertension. These conditions are associated with changes in the choroidal vasculature (20), which could lead to a different interpretation of results if they were taken into account. Also, questions remain on whether choroidal vessels appeared thinner mainly due to the mechanical stretching forces or if they were congenitally thinner in eyes with a higher risk of myopia (28). Histological studies had revealed that the choroid thins with age and increased refractive error, but the cause of choroidal thinning remains poorly understood. Comparing choroidal thickness in similar AL could provide a better idea of how different the choroidal vessels are. Staphyloma, mCNV and other MMD-related features were not investigated in this study as well, which could have provided us with a better idea of why CVDMVCL increases with an increase in odds of MMD.
In conclusion, longer eyes were observed to have decreased choroidal vessel width, reduced choroidal vessel density, thinner choroidal layer, and increased vascular branching. The latter could be a compensatory mechanism to maintain choroidal blood supply in elongated eyes. A thinner choroid, with almost equal MVCL and LVCL thinning, and denser MVCL, which may be signs of hypoxia, were also associated with greater odds of MMD.
The raw data supporting the conclusions of this article will be made available by the authors, without undue reservation.
The studies involving human participants were reviewed and approved by Centralized Institutional Review Board (CIRB) of SingHealth, Singapore. The patients/participants provided their written informed consent to participate in this study.
YD and KC drafted the manuscript. SL performed all statistical analysis and created all tables. QW helped acquire the data. RC, CW and QH conceived the project, acquired the data and directed the writing and analysis. All authors proofread the manuscript. All authors contributed to the article and approved the submitted version.
The authors would like to thank Dr. Bridget Ng for her assistance with image segmentation. This work is supported by: National Medical Research Council (MOH-000151-00 (QVH), MOH-001103-00 (QVH) and MOH-000531-00 (QVH)), the SERI-Lee Foundation (LF0621-1 (QVH)) and the Lee Foundation (TLF 1021-3 (QVH) and TLF 0322-8 (QVH)) in Singapore. The sponsor or funding organisation had no role in the design or conduct of this research.
The authors declare that the research was conducted in the absence of any commercial or financial relationships that could be construed as a potential conflict of interest.
All claims expressed in this article are solely those of the authors and do not necessarily represent those of their affiliated organizations, or those of the publisher, the editors and the reviewers. Any product that may be evaluated in this article, or claim that may be made by its manufacturer, is not guaranteed or endorsed by the publisher.
1. Saw SM, Matsumura S, Hoang QV. Prevention and management of myopia and myopic pathology. Invest Ophthalmol Vis Sci (2019) 60(2):488. doi: 10.1167/iovs.18-25221
2. Ohno-Matsui K, Wu PC, Yamashiro K, Vutipongsatorn K, Fang Y, Cheung CMG, et al. IMI pathologic myopia. Invest Ophthalmol Vis Sci (2021) 62(5):5. doi: 10.1167/iovs.62.5.5
3. Holden BA, Fricke TR, Wilson DA, Jong M, Naidoo KS, Sankaridurg P, et al. Global prevalence of myopia and high myopia and temporal trends from 2000 through 2050. Ophthalmology (2016) 123(5):1036–42. doi: 10.1016/j.ophtha.2016.01.006
4. Saw SM, Chua WH, Hong CY, Wu HM, Chan WY, Chia KS, et al. Nearwork in early-onset myopia. Invest Ophthalmol Vis Sci (2002) 43(2):332–9.
5. French AN, Morgan IG, Mitchell P, Rose KA. Patterns of myopigenic activities with age, gender and ethnicity in Sydney schoolchildren. Ophthalmic Physiol Opt (2013) 33(3):318–28. doi: 10.1111/opo.12045
6. Mutti DO, Mitchell GL, Moeschberger ML, Jones LA, Zadnik K. Parental myopia, near work, school achievement, and children’s refractive error. Invest Ophthalmol Vis Sci (2002) 43(12):3633–40.
7. Wong CW, Phua V, Lee SY, Wong TY, Cheung CMG. Is choroidal or scleral thickness related to myopic macular degeneration? Invest Ophthalmol Vis Sci (2017) 58(2):907. doi: 10.1167/iovs.16-20742
8. Diaz JD, Wang JC, Oellers P, Lains I, Sobrin L, Husain D, et al. Imaging the deep choroidal vasculature using spectral domain and swept source optical coherence tomography angiography. J VitreoRetinal Diseases (2018) 2(3):146–54. doi: 10.1177/2474126418771805
9. Choi W, Mohler KJ, Potsaid B, Lu CD, Liu JJ, Jayaraman V, et al. Choriocapillaris and choroidal microvasculature imaging with ultrahigh speed OCT angiography. Wedrich A Ed PloS One (2013) 8(12):e81499. doi: 10.1371/journal.pone.0081499
10. Poddar R, Migacz JV, Schwartz DM, Werner JS, Gorczynska I. Challenges and advantages in wide-field optical coherence tomography angiography imaging of the human retinal and choroidal vasculature at 1.7-MHz a-scan rate. J BioMed Opt (2017) 22(10):106018. doi: 10.1117/1.JBO.22.10.106018
11. Devarajan K, Sim R, Chua J, Wong CW, Matsumura S, Htoon HM, et al. Optical coherence tomography angiography for the assessment of choroidal vasculature in high myopia. Br J Ophthalmol (2020) 104(7):917–23. doi: 10.1136/bjophthalmol-2019-314769
12. Flores-Moreno I, Lugo F, Duker JS, Ruiz-Moreno JM. The relationship between axial length and choroidal thickness in eyes with high myopia. Am J Ophthalmol (2013) 155(2):314–319.e1. doi: 10.1016/j.ajo.2012.07.015
13. Nishida Y, Fujiwara T, Imamura Y, Lima LH, Kurosaka D, Spaide RF. Choroidal thickness and visual acuity in highly myopic eyes. Retina (2012) 32(7):1229–36. doi: 10.1097/IAE.0b013e318242b990
14. Zhao J, Wang YX, Zhang Q, Wei WB, Xu L, Jonas JB. Macular choroidal small-vessel layer, sattler’s layer and haller’s layer thicknesses: the Beijing eye study. Sci Rep (2018) 8(1):4411. doi: 10.1038/s41598-018-22745-4
15. Alshareef RA, Khuthaila MK, Januwada M, Goud A, Ferrara D, Chhablani J. Choroidal vascular analysis in myopic eyes: evidence of foveal medium vessel layer thinning. Int J Retin Vitr (2017) 3(1):28. doi: 10.1186/s40942-017-0081-z
16. Sato M, Minami S, Nagai N, Suzuki M, Kurihara T, Shinojima A, et al. Association between axial length and choroidal thickness in early age-related macular degeneration. PloS One (2020) 15(10):e0240357. doi: 10.1371/journal.pone.0240357
17. Shiihara H, Sakamoto T, Terasaki H, Kakiuchi N, Shinohara Y, Tomita M, et al. Running pattern of choroidal vessel in en face OCT images determined by machine learning–based quantitative method. Graefes Arch Clin Exp Ophthalmol (2019) 257(9):1879–87. doi: 10.1007/s00417-019-04399-8
18. Gao J, Rao CH, Li F, Liu L, Liu KJ. Ultra-widefield swept-source optical coherence tomography angiography in the assessment of choroidal changes in young adults with myopia. Transl Vis Sci Technol (2022) 11(12):14. doi: 10.1167/tvst.11.12.14
19. Brinks J, van Dijk EHC, Klaassen I, Schlingemann RO, Kielbasa SM, Emri E, et al. Exploring the choroidal vascular labyrinth and its molecular and structural roles in health and disease. Prog Retinal Eye Res (2022) 87:100994. doi: 10.1016/j.preteyeres.2021.100994
20. Yang YS, Koh JW. Choroidal blood flow change in eyes with high myopia. Korean J Ophthalmol (2015) 29(5):309. doi: 10.3341/kjo.2015.29.5.309
21. Nivison-Smith L, Khandelwal N, Tong J, Mahajan S, Kalloniatis M, Agrawal R. Normal aging changes in the choroidal angioarchitecture of the macula. Sci Rep (2020) 10(1):10810. doi: 10.1038/s41598-020-67829-2
22. Cheong KX, Lim LW, Li KZ, Tan CS. A novel and faster method of manual grading to measure choroidal thickness using optical coherence tomography. Eye (Lond) (2018) 32(2):433–8. doi: 10.1038/eye.2017.210
23. Wang E, Zhao X, Yang J, Chen Y. Visualization of deep choroidal vasculatures and measurement of choroidal vascular density: a swept-source optical coherence tomography angiography approach. BMC Ophthalmol (2020) 20(1):321. doi: 10.1186/s12886-020-01591-x
24. Zhou Y, Song M, Zhou M, Liu Y, Wang F, Sun X. Choroidal and retinal thickness of highly myopic eyes with early stage of myopic chorioretinopathy: tessellation. J Ophthalmol (2018) 2018:1–9. doi: 10.1155/2018/2181602
25. Lu X, Zhang G, Cen L, Du Y, Liu L, Jin C, et al. Choroidal vascular density quantification in high myopia with or without choroidal neovascularization using optical coherence tomography angiography. J Ophthalmol (2023) 2023:1504834. doi: 10.1155/2023/1504834
26. Barteselli G, Lee SN, El-Emam S, Hou H, Ma F, Chhablani J, et al. Macular choroidal volume variations in highly myopic eyes with myopic traction maculopathy and choroidal neovascularization. Retina (2014) 34(5):880–9. doi: 10.1097/IAE.0000000000000015
27. Mirzapour-Shafiyi F, Kametani Y, Hikita T, Hasegawa Y, Nakayama M. Numerical evaluation reveals the effect of branching morphology on vessel transport properties during angiogenesis. PloS Comput Biol (2021) 17(6):e1008398. doi: 10.1371/journal.pcbi.1008398
Keywords: myopic macular degeneration, optical coherence tomographic angiography, choroidal thickness, choroidal vessel density, choroidal branch area, choroidal vessel width, medium vessel, large vessel
Citation: Dan YS, Cheong KX, Lim SY, Wong QY, Chong RS, Wong CW and Hoang QV (2023) Quantitative assessment of the choroidal vasculature in myopic macular degeneration with optical coherence tomographic angiography. Front. Ophthalmol. 3:1202445. doi: 10.3389/fopht.2023.1202445
Received: 08 April 2023; Accepted: 25 May 2023;
Published: 14 June 2023.
Edited by:
Yuxin Fang, Capital Medical University, ChinaReviewed by:
Shiqi Xie, Tokyo Medical and Dental University, JapanCopyright © 2023 Dan, Cheong, Lim, Wong, Chong, Wong and Hoang. This is an open-access article distributed under the terms of the Creative Commons Attribution License (CC BY). The use, distribution or reproduction in other forums is permitted, provided the original author(s) and the copyright owner(s) are credited and that the original publication in this journal is cited, in accordance with accepted academic practice. No use, distribution or reproduction is permitted which does not comply with these terms.
*Correspondence: Quan V. Hoang, ZG9ubnkuaG9hbmdAc2luZ2hlYWx0aC5jb20uc2c=; Chee Wai Wong, d29uZy5jaGVlLndhaUBzaW5naGVhbHRoLmNvbS5zZw==
†These authors have contributed equally to this work and share first authorship
‡These authors have contributed equally to this work and share senior authorship
Disclaimer: All claims expressed in this article are solely those of the authors and do not necessarily represent those of their affiliated organizations, or those of the publisher, the editors and the reviewers. Any product that may be evaluated in this article or claim that may be made by its manufacturer is not guaranteed or endorsed by the publisher.
Research integrity at Frontiers
Learn more about the work of our research integrity team to safeguard the quality of each article we publish.