- Department of Ophthalmology, Weill Cornell Medical College, New York, NY, United States
Regulatory T cells (Tregs) are critical for the maintenance of immune tolerance and the suppression of excessive inflammation. Many inflammatory autoimmune disorders, including autoimmune uveitis, involve the loss of the suppressive capacities of Tregs. Over the past decade, Tregs’ therapeutic potential in uveitis has garnered increasing attention. Specific subsets of Tregs, including TIGIT+ and PD-1+ Tregs, have emerged as potent immunosuppressors that may be particularly well-suited to cell-based therapeutics. Studies have elucidated the interaction between Treg development and the gut microbiome as well as various intracellular signaling pathways. Numerous cell-based therapies and therapeutic molecules have been proposed and investigated using the murine experimental autoimmune uveitis (EAU) model. However, certain challenges remain to be addressed. Studies involving the use of Tregs in human patients with uveitis are lacking, and there are concerns regarding Tregs’ production and purification for practical use, their plasticity towards inflammatory phenotypes, immunogenicity, and tumorigenicity. Nevertheless, recent research has brought Tregs closer to yielding viable treatment options for uveitis.
1 Introduction
Regulatory T cells (Tregs) are a subset of CD4+ T cells that play a critical role in maintaining immune tolerance. Dysfunction and/or depletion of Tregs has been implicated in the pathogenesis of autoimmune disorders including Sjogren syndrome, dry eye disease (DED), Behcet disease (BD), and Vogt-Koyanagi-Harada disease (VKH) (1, 2).
2 Mechanism of Action
Tregs make important contributions to ocular immune privilege, helping to foster a relatively immunoquiescent environment. Tregs utilize multiple mechanisms to exert their immunosuppressive effects, including granzyme-mediated cytolysis, apoptosis of effector T cells (Teff), and the secretion of various anti-inflammatory cytokines including TGF-β, IL-10, and IL-35 (3) (Figure 1). Tregs have also been shown to modulate dendritic cells (DCs) (4). The interaction between dendritic cells and Tregs is mediated, in part, by indoleamine (IDO); under inflammatory conditions, IDO activation in antigen presenting cells leads to immune tolerance and inhibition of T cell proliferation (5). Furthermore, Tregs downregulate DC markers CD80/86 and induce apoptosis of DCs via IDO (4).
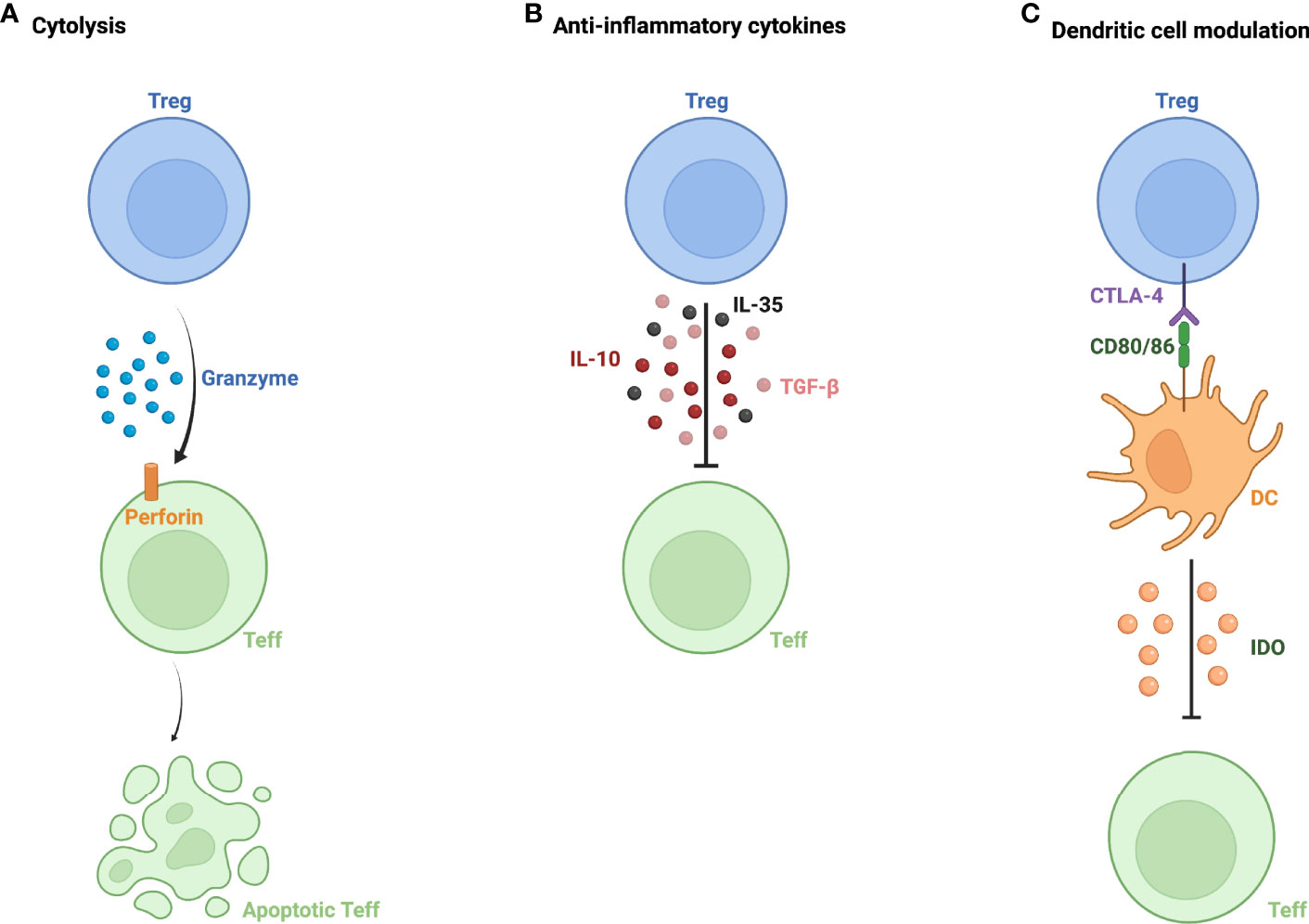
Figure 1 Mechanisms of action of regulatory T cells. (A) Granzyme and perforin-mediated apoptosis of effector T cells (Teff) by regulatory T cells (Treg). (B) Inhibition of Teff function via secretion of anti-inflammatory cytokines- IL-10, IL-35, and TGF-β. (C) Modulation of dendritic cells (DC) via interaction between CTLA-4 expressed on Treg and DC markers CD80/86, leading to Teff inactivation by DC via indoleamine 2,3-dioxygenase (IDO).
3 Classification
Tregs are identified by their surface marker, CD25, as well as their transcription factor forkhead box P3 (FOXP3) (3). However, there are multiple subsets of Tregs, with notable subsets including those that express TIGIT and PD-1. TIGIT+ Tregs promote IL-10 induced proliferation of regulatory dendritic cells, thereby suppressing T cell activation (6). Upregulation of TIGIT expression has been associated with hypomethylation of FOXP3 in Tregs, increasing its expression (7). TIGIT+ Tregs have been implicated in uveitis remission, given the correlation between TIGIT expression and remission in uveitis patients (8). Interestingly, in studies involving the murine experimental autoimmune uveitis (EAU) model, not all Tregs that remain following resolution of uveitis are suppressive (9–12). However, those post-EAU Tregs that maintain their suppressive function have been found to have high levels of TIGIT expression (13). From these data, investigators have suggested that TIGIT might serve as a sensitive marker for remission in uveitis, and that TIGIT might have some utility in identifying ‘functional’ Tregs (8). Yet another subset, PD-1+ Tregs, have similarly demonstrated marked immunosuppressive capacities in the context of autoimmune disorders (14). In uveitis, low levels of PD-1+ Tregs are implicated in developing chronic disease (14). PD-1+ Tregs utilize a melanocortin receptor (MC5r)-dependent pathway, which subsequently activates the adenosine receptor, A2Ar (14). A2Ar stimulation induces Treg proliferation and activation and has been implicated in conferring resistance to EAU relapse (15, 16). Decreased PD-1+ Tregs have been observed in patients with uveitis (14); therefore, targeting A2Ar may represent a potential therapeutic approach, with the goal of bypassing upstream PD-1 and MC5r.
Another area of recent research interest is the enhancers and promoters of FOXP3, called Treg-specific demethylated regions (TSDRs), which influence the level of FoxP3 expression (17). Studies have shown that TSDRs with low methylation levels are crucial for Treg development (18–20). Ten-Eleven-Translocation (Tet) belongs to a family of enzymes that play a role in the demethylation of TSDR (21). Using these concepts, Ito et al. have reported the development of a population of stable, antigen-specific Tregs; the group utilized vitamin C to induce Tet, and subsequent hypomethylation of TSDR within enhancers led to the generation of antigen-specific Tregs with stable FoxP3 expression (17). Given that instability of antigen-specific Tregs has been one of the main barriers to their practical implementation, the ability to maintain stable expression of FoxP3 using this model represents an important step toward clinical use.
4 Plasticity
An important consideration in the development of cellular therapies using regulatory T cell concerns their ability to differentiate into pro-inflammatory phenotypes, termed plasticity. Studies have revealed that the transformation of Tregs to T helper 17 cells (Th17) may play a crucial role in the development of various autoimmune disorders (1). This transformation can occur when Tregs are exposed to inflammatory environments, and results in the loss of FOXP3 expression and diminished suppressive ability (1, 22). Moreover, these cells may express pro-inflammatory cytokines, becoming ‘ex-Tregs’ that have been implicated in the pathogenesis of type 1 diabetes, colitis, and arthritis (23). Interestingly, Tregs expressing IL-17 may still have anti-inflammatory effects, depending on the stage of autoimmune disease (24–26). T cells co-expressing FOXP3 and retinoic acid-related orphan receptor (RORγt), a transcription factor for Th17 phenotype, have been shown to produce IL-17, yet these cells continue to suppress T cell proliferation and limit inflammation (27). The mechanism of conversion between phenotypes in Tregs co-expressing CD25 and IL-17 remains unclear at this time; noting that cellular therapies involve transferring cells to pro-inflammatory environments, it is vital to better understand the potential of Tregs to differentiate in these settings.
5 Immune Mechanisms in Uveitis
Uveitis is an inflammatory disorder of the eye caused by interactions between ocular autoantigens and T cells (1). T cells that escape negative selection in the thymus migrate to the eye, where they become activated by ocular antigens (28). IFN- γ-producing Th1 and IL-17-producing Th17 cells are the most significant effector T cells driving intra-ocular inflammation (1). In experimental autoimmune uveitis mouse models, Tregs reach their highest frequencies around the peak of disease activity, and remain elevated during resolution, corresponding to increased IL-10 and TGF-β during resolution (29). Unsurprisingly, decreased IL-6 and IL-17 levels are observed during resolution (29). There are conflicting data regarding the correlation between absolute level of Tregs and disease activity in patients with uveitis. While some studies report higher Treg levels in uveitis patients compared to healthy patients, others report lower levels (30–35). More recent studies have proposed measuring Treg/Th17 ratio to assess recovery (29). More specific suppressive Treg markers such as TIGIT and PD-1 may also be valuable in establishing a correlation between Treg level and uveitis resolution.
Current management of uveitis relies heavily on corticosteroids and immunomodulators (36). However, due to adverse systemic and ocular effects seen with prolonged use of these medications, newer therapies with higher efficacy and narrower targets are being sought. Here, we review potential therapies and targets that have been discussed in recently published studies.
6 Mouse Models of Uveitis
The role of Tregs in dampening ocular inflammation has been demonstrated in murine models of EAU. Depletion of FoxP3+ Tregs has been shown to result in more severe EAU relative to controls following exposure to IRBP; depletion of Tregs following EAU induction has been shown to inhibit disease resolution, and depletion of Tregs following resolution has been shown to result in relapse (37, 38). These data demonstrate the suppressive function of Tregs at each stage of the disease. Adoptive transfer of FOXP3+ Tregs to mice with established EAU has been shown to reduce inflammation as well as the expression of pro-inflammatory cytokines such as IL-17 and IFN- γ (38, 39). In patients with uveitis, decreased frequencies and impaired function of Tregs has been observed (22).
7 Therapeutic Opportunities
7.1 Gut Microbiome
The gut microbiome (GM) is known to mediate immunomodulatory effects, and the disturbance of GM has been linked to the development of autoimmune disorders, including uveitis (40–47). Nakamura et al. have documented the difference in composition of GM in patients with uveitis compared to healthy patients (48). The investigators have also demonstrated that antibiotics given orally, but not through intraperitoneal injection, affected susceptibility to EAU induction (48). Vancomycin and Metronidazole, in particular, led to an increase in Treg population in the retina and lymph nodes, associated with decreased EAU severity (48). Other studies have also established reduced incidence of EAU following antibiotic administration in mice (49–52). Kasper et al. investigated the composition of GM in human patients with Behcet Disease (BD), whose GM was characterized by decreased butyrate production (53). Butyrate and other short-chain fatty acids are essential in Treg differentiation and mediate anti-inflammatory effects Novel everolimus-loaded nanocarriers for topical treatment of murine experimental autoimmune uveoretinitis (53). Butyrate has been shown to induce thymic Tregs and suppress pro-inflammatory cytokines (53). Chen et al. studied the effect of sodium butyrate (NaB) administration in EAU (54). NaB resulted in decreased inflammation in EAU, correlated with increased splenic Tregs and a decrease in Th17 (54). NaB-treated mice had increased IL-10 expression and decreased expression of chemokines, IL-17, IFN- γ, TNF-α, and ROR γT (54). The proposed mechanism of NaB involved manipulating the plasticity between Tregs and Th17, redirecting Th17 towards the Treg phenotype by inhibiting IL-6R (54).
7.2 Signaling Pathways
Several signaling pathways that affect Treg development have been identified and targeted to study potential therapeutic candidates.
7.2.1 PI3K/AKT
PI3K/AKT pathway promotes secretion of pro-inflammatory cytokines and is essential for Th17 differentiation (55, 56). This pathway is dysregulated in autoimmune disorders, including uveitis (57). Blockage of this pathway using Apremilast, a phosphodiesterase 4 (PDE4) inhibitor, has been used to control inflammation in rheumatoid arthritis, psoriatic arthritis, dermatitis, and other autoimmune disorders (58–63). Chen et al. demonstrated that Apremilast administration results in decreased clinical and histological disease severity in EAU and is associated with increased Treg and decreased Th17 populations (57). Another compound, AS101, a tellurium-based molecule, has been shown to suppress EAU development by inhibiting the activation of AKT (64). Following administration of AS101, increased conversion of naïve T cells to Tregs and decreased Teff population has been observed (64).
7.2.2 STAT
AS101 has also been shown to suppress phosphorylation of STAT3 and STAT4, enzymes of another pathway required for Th17 and Th1 differentiation (64). Wang et al. demonstrated that progranulin (PGRN) induced expansion of antigen-specific and non-specific Tregs via STAT5 phosphorylation, reducing EAU severity (65). Correspondingly, decreased PGRN in the peripheral blood of patients with active BD and VKH has been reported (65). Vorinostat, a histone deacetylase inhibitor, has been shown to result in decreased STAT1 and STAT3 expression, associated with increased Treg frequencies (57). Aminooxy-acetic acid (AOA) has also been used to block the STAT/NF-kB pathway, leading to reduced inflammation in EAU (66). As expected, AOA led to upregulation of Tregs and IL-10 and downregulation of Th1/Th17 activity (66).
7.2.3 Notch1
Notch signaling plays an important role in inhibiting Treg differentiation and promoting Teff differentiation (67–70). Exposing Tregs in vitro with JAG1 and DLL1, downstream molecules of the Notch1 pathway, has been shown to downregulate FOXP3 expression and modulate the immunosuppressive function of Tregs (71). Furthermore, adoptive transfer of Notch-deficient Tregs in EAU mice has been shown to downregulate pro-inflammatory cytokines in the eye (71). DAPT, a Notch signaling inhibitor, restored Treg/Th17 balance, increased IL-10, and reduced inflammation in EAU (72).
8 Cell-Based Therapies
8.1 IL-35 and Bregs
IL-35-induced Bregs promote Treg expansion and suppress Th17 and Th1 response in uveitis (73). Adoptive transfer of ex-vivo produced IL-35+ Bregs led to resistance against EAU development and resolution of existing EAU in mice (73). Another study demonstrated that IL-35 containing exosomes secreted from Bregs stimulated IL-10 and IL-35 secreting Tregs, reducing EAU severity (28). Exosomes are particularly beneficial in clinical use because they may be applied topically in the form of an eyedrop and bypass the requirement of generating autologous Bregs for transplant (28).
8.2 Col-Tregs
Collagen II is constitutively expressed in the retina and the vitreous (74, 75). Collagen II-specific Tregs (Col-Tregs), activated upon exposure to collagen II, inhibit uveitis in mice (76, 77). Like type 1 Tregs, Col-Tregs display multimodal ability to suppress inflammation (76, 77). Although Col-Tregs have been studied exclusively in mice, they are similar to human Col-Tregs, lack tumorgenicity and plasticity, and minimize systemic immunosuppression, making them promising candidates for therapy (76, 77).
8.3 Mesenchymal Stem Cells (MSCs)
Human MSCs are known to exert immunomodulatory effects and generate Tregs (78). MSCs secrete TGF-β and prostaglandin E2 under inflammatory conditions, inducing differentiation of naïve T cells to Tregs (79). MSCs also upregulate their chemokines during inflammation, trapping Th17 cells and shifting them towards the Treg phenotype by taking advantage of their plasticity (80). In addition, MSCs bias macrophages towards the M2 phenotype, which secretes IL-10 and induces Treg expansion (79). Intraperitoneal injection of MSCs in EAU induced antigen-specific Treg development mediated by TGF-β (78). These Tregs remained long after a single injection, conferring protection against EAU relapse (78). However, MSCs are not exclusively anti-inflammatory; they can exert pro-inflammatory effects under certain environmental conditions (79). Therefore, further studies assessing the effect of the microenvironment on MSC phenotype are required.
8.4 Human Amniotic Epithelial Cells (hAECs)
hAECs share properties of Col-Tregs and MSCs that make them promising candidates for cell-based therapy in uveitis. hAECs have low immunogenicity (81–83), lack tumorgenicity (84, 85), and can be easily harvested from amniotic membrane (82). Compared to MSCs, hAECs have lower immunogenicity and tumorigenicity and are less prone to apoptosis under inflammatory environments (81, 85). Li et al. revealed that sub-retinal injection of hAECs increased Treg/Th17 ratio by shifting macrophages toward M2 expression, which was associated with a decreased pathological score in EAU (86). Additionally, hAECs were seen to reduce both induction and progression of the disease, depending on when they were administered (day 0 vs. day 6) (86).
8.5 Retinoic Acid Receptors (RAR)
RAR is necessary for Treg differentiation and maintenance in inflammatory disease (87–91). RAR contributes to the eye’s immune privilege by inducing differentiation of naïve T cells to Tregs in the aqueous humor (92). Immunization of mice with foreign antigen (to induce EAU) with RAR and low-dose IL-2 induced generation of Tr1 Tregs (93). While Tr1 Tregs do not express FOXP3, they suppress the immune response by secreting IL-10 and expressing immune checkpoints, including CTLA-4 and PD-1 (93). Interestingly, IL-2 has been shown to promote Treg proliferation without affecting Teff (94–96). Therefore, co-administration of RAR and IL-2 can expand Treg in vivo and be helpful in therapeutic settings.
8.6 Anti-CD4
A recent study by Chen et al. revealed anti-CD4 as a promising tool to generate antigen-specific Tregs in vivo (97). In vivo generation of stable antigen-specific Tregs has been challenging. This study aimed to address the issue by using anti-CD4 to deplete Teff and then introducing retinal antigen to generate antigen-specific Tregs. Anti-CD4 mediated apoptosis of Teff resulted in increased TGF-β and IL-10 expression, which subsequently led to higher levels of CD25+ FOXP3+ Tregs (97). Introduction of ocular antigens (IRBP and S-antigen, in separate trials) generated Tregs specific to these antigens and suppressed antigen-driven Th17 and Th1 response (97). An overall decrease in inflammation was observed (97). Although additional studies detailing the efficacy and toxicity are required, anti-CD4 represents a step towards the ability to produce Tregs in vivo.
9 Human Data
No human data demonstrating successful treatment of uveitis with Tregs has been published at the time of this writing (98). However, several therapies that have been used to treat uveitis in humans mediate their effects by induction of Tregs, which are reviewed in this section.
Albayrak et al. investigated the therapeutic effect of IFN-2a on patients with BD (99). The study proposed that IFN-2a leads to recovery of Treg function. 70% of the patients in the study underwent remission of uveitis with a median duration of treatment of 5 months (99). Interestingly, IFN treatment was associated with decreased Treg frequencies, but increased IL-10 and IL-35 secretion by Tregs (99). BD patients had a higher baseline level of Tregs and lower IL-10 than healthy patients. Based on these observations, the authors concluded that Treg dysfunction, rather than scarcity, was contributing to pathogenesis (99). Notably, this is consistent with murine models of ocular inflammation, in which adoptive transfer experiments have shown that far more relevant than Treg frequency is levels of FOXP3 expression, which is directly associated with Treg immunosuppressive function (100).
Given the evidence that the STAT pathway is involved in Treg development and T cell differentiation, JAK inhibitors have been proposed as having potential therapeutic benefit (101). In a case series of four patients with juvenile idiopathic arthritis with uveitis, JAK inhibitors (baricitinib [three cases] and tofacitinib [one case]), successfully suppressed uveitis (101). While tofacitinib has a black box warning for serious infections, malignancies, cardiovascular effects, thrombosis, and all-cause mortality, no such complication was seen in the patient after one year of use. Metformin has also been investigated in BD patients, the use of which has been noted to be correlated with an increase in FOXP3 and TGF-β expression and concurrent decrease in ROR γT, IL-17, and TNF-α (102). Based on reports of IL-2’s ability to expand Tregs without affecting Teff level, Liu et al. administered low-dose IL-2 to BD patients, which led to a reduction in disease severity without adverse effects (103). While all T cell subsets showed expansion under IL-2 stimulation, Tregs underwent the most dramatic increase by a factor of 4 (103).
10 Regulatory T Cells in Human Autoimmunity
Strong links between various autoimmune disorders in humans and the deficiency or dysfunction of Tregs lend support to the notion that Tregs are crucial in maintaining immune homeostasis in humans. One notable sequela of Treg dysfunction is X-linked immunodeficiency (IPEX) syndrome, an autoimmune disease that affects multiple organs (104). Mutations in FoxP3, CD25, and CTLA-4, all associated with Treg function, have been implicated in the pathogenesis of autoimmune and inflammatory disorders in humans (105, 106). Importantly, an increase in Th17/Treg ratio has been documented in autoimmune disorders, including multiple sclerosis, psoriasis, rheumatoid arthritis (RA), and inflammatory bowel disease (IBD) (107). Interestingly in both psoriatic and RA patients, Treg level was comparable to that of healthy patients; however, affected patients have Tregs with diminished suppressive capacities, which is thought to be the main contributor to these pathologies (108–110). In psoriatic patients, Tregs were less effective in suppressing IL-17 production and were shown to lose their FOXP3 expression more easily (109, 110).
Given the importance of Th17/Treg imbalance in pathogenesis, several medications are currently undergoing clinical trials to restore their balance to treat MS, RA, SLE, and IBD (107). For instance, in RA patients, monoclonal antibodies against IL-6R and tocilizumab were shown to reduce not only the Th17 phenotype but also increase the Treg population (107, 111, 112). However, there remain controversies regarding the association between Treg level and autoimmunity. There are conflicting data on frequencies of Treg in autoimmune diseases, particularly in SLE and RA. While some studies report an increased level of Tregs in these disease states, others have discovered an unchanged or decreased level of Tregs (113, 114). The discrepant findings expose one of the main challenges to Treg therapy: the ability to identify and target Treg populations reliably. Tregs in SLE have decreased CD25 expression; therefore, using CD25 as a Treg identifier leads to reduced measurements, whereas using FOXP3 leads to increased measurements (115–117). However, a detailed analysis of Treg subpopulations demonstrates a decreased level of ‘effective’ Tregs in both SLE and RA (118). While it is known that multiple subpopulations of Tregs exist in humans, a more reliable and accurate marker to identify different types would be essential in progressing Treg therapy.
As for human therapy utilizing immunomodulatory effects of Tregs, induction of the Treg population with IL-2 has been successful in treating type 1 diabetes, alopecia areata, and hepatitis C virus-induced vasculitis (119). Because Tregs are particularly sensitive to IL-2, IL-2 has been shown to promote Treg proliferation with minimal effects on other T-cell lineages (120). Using an antibody directed against the IL-2 beta chain has been theorized to increase IL-2’s specificity, as Tregs utilize the IL-2 alpha chain for IL-2 binding unlike many other immune cells (120). In addition to IL-2, autologous transfer of in-vitro expanded naïve Tregs from human plasma has demonstrated in-vivo stability and safety in type 1 diabetes and graft versus host disease patients (121, 122). Further delineation of specific Treg markers and more human data will significantly benefit the potential of Treg therapy in human autoimmunity.
11 Discussion, Challenges, and Future Directions
Studies have proposed that ideal characteristics of Tregs for use in therapy include antigen-specificity, maximal purity/avoiding contamination, absence of plasticity toward inflammatory phenotypes, and capacity to migrate to target sites.
Challenges associated with developing antigen-specific Tregs include lack of knowledge of autoantigens that cause autoimmune disorders, and the difficulties associated with producing different sets of Tregs for every autoantigen (49). Given the difficulties, only polyclonal Tregs have been developed for human studies (123). However, polyclonal Tregs are limited in their route of administration and duration of action compared to antigen-specific Tregs. While antigen-specific Tregs have been reported to downregulate ocular inflammation when administered intravenously, the transfer of non-specific Tregs has not been shown to be effective (123). Polyclonal Tregs reduced inflammation only when they were injected in-situ (123). In addition, the protection conferred by polyclonal Tregs was transient; recipients were vulnerable to uveitis when Teff were re-introduced at later time points (123). Antigen-specific Tregs, on the other hand, may offer long-term protection. Therefore, there seem to be important advantages of transferring antigen-specific Tregs rather than polyclonal Tregs.
Even when Tregs are generated in vivo, they are unstable, limiting their clinical efficacy. Rapamycin, RAR, and IL-2 have been shown to produce stable Tregs, and may become crucial ingredients in the commercial development of Tregs (124). We also discussed that vitamin C-induced hypomethylation of TSDR led to the generation of stable Tregs in vivo; in line with this finding, some have suggested epigenetic modification of FOXP3 may be vital to producing a stable population of Tregs (125).
A reliable marker of Tregs must be identified to isolate Tregs from contaminants. Although FOXP3 is a reliable marker, it requires permeabilization, causing cell death (49). Some studies have proposed identifying Tregs with low expression of CD45RA, which corresponds to active Tregs (126, 127). As we mentioned, markers such as TIGIT or PD-1 have been found to represent ‘functional’ suppressive Tregs. As contamination is nearly inevitable even with identifying specific Treg markers, future studies should attempt to uncover the extent to which contamination can be tolerated for clinical use (49).
Finally, we need to better understand Tregs’ plasticity, tumorigenicity, and systemic immunosuppressive capabilities. Tregs have shown a tendency to convert to Th17 phenotype in inflammatory environments with an abundance of IL-6, posing a challenge for treating patients with inflammatory disorders. Some of the mentioned cell-based therapies, especially Col-Tregs (and MSCs and hAECs), which demonstrate low plasticity, tumorigenicity, and systemic immunosuppression, may overcome these challenges.
The role of Tregs in maintaining immune tolerance and as a potential therapeutic tool in uveitis has been actively investigated over the past decade. However, data from human patients are scarce, and barriers to utilizing Tregs in clinical settings remain to be addressed. Future studies addressing these issues and translating EAU studies to human subjects could potentially make cell based Treg therapies a viable treatment option in uveitis.
Author Contributions
AL and WF: conception of work, drafting and critical revision of work, final approval of the version to be published and agreement to be accountable for all aspects of work.
Funding
This project was supported in part by a department grant from the Research to Prevent Blindness Foundation.
Conflict of Interest
The authors declare that the research was conducted in the absence of any commercial or financial relationships that could be construed as a potential conflict of interest.
Publisher’s Note
All claims expressed in this article are solely those of the authors and do not necessarily represent those of their affiliated organizations, or those of the publisher, the editors and the reviewers. Any product that may be evaluated in this article, or claim that may be made by its manufacturer, is not guaranteed or endorsed by the publisher.
References
1. Gery I, Caspi RR. Tolerance Induction in Relation to the Eye. Front Immunol (2018) 9:2304. doi: 10.3389/FIMMU.2018.02304
2. Foulsham W, Marmalidou A, Amouzegar A, Coco G, Chen Y, Dana R. Review: The Function of Regulatory T Cells at the Ocular Surface. Ocul Surf (2017) 15:652–9. doi: 10.1016/j.jtos.2017.05.013
3. Josefowicz SZ, Lu L-F, Rudensky AY. Regulatory T Cells: Mechanisms of Differentiation and Function. Annu Rev Immunol (2012) 30:531–64. doi: 10.1146/annurev.immunol.25.022106.141623
4. Okeke EB, Uzonna JE. The Pivotal Role of Regulatory T Cells in the Regulation of Innate Immune Cells. Front Immunol (2019) 10:680. doi: 10.3389/FIMMU.2019.00680
5. Zhang L, Huang Y, Cui X, Tan X, Zhu Y, Zhou W, et al. Increased Expression of Indoleamine 2,3-Dioxygenase (IDO) in Vogt-Koyanagi-Harada (VKH) Disease May Lead to a Shift of T Cell Responses Toward a Treg Population. Inflammation (2020) 43:1780–8. doi: 10.1007/S10753-020-01252-7
6. Lee DJ. The Relationship Between TIGIT + Regulatory T Cells and Autoimmune Disease. Int Immunopharmacol (2020) 83:106378. doi: 10.1016/j.intimp.2020.106378
7. Zhang Y, Maksimovic J, Naselli G, Qian J, Chopin M, Blewitt ME, et al. Genome-Wide DNA Methylation Analysis Identifies Hypomethylated Genes Regulated by FOXP3 in Human Regulatory T Cells. Blood (2013) 122:2823–36. doi: 10.1182/BLOOD-2013-02-481788
8. Gilbert RM, Zhang X, Sampson RD, Ehrenstein MR, Nguyen DX, Chaudhry M, et al. Clinical Remission of Sight-Threatening Non-Infectious Uveitis Is Characterized by an Upregulation of Peripheral T-Regulatory Cell Polarized Towards T-Bet and TIGIT. Front Immunol (2018) 9:907. doi: 10.3389/FIMMU.2018.00907
9. Lambrecht BN, Salomon B, Klatzmann D, Pauwels RA, Mizuno S, Matsumoto K, et al. Dendritic Cells are Required for the Development of Chronic Eosinophilic Airway Inflammation in Response to Inhaled Antigen in Sensitized Mice. J Immunol (1998) 160:4090–7. doi: 10.4049/jimmunol.175.7.4745
10. Gavin MA, Torgerson TR, Houston E, DeRoos P, Ho WY, Stray-Pedersen A, et al. Single-Cell Analysis of Normal and FOXP3-Mutant Human T Cells: FOXP3 Expression Without Regulatory T Cell Development. Proc Natl Acad Sci USA (2006) 103:6659–64. doi: 10.1073/PNAS.0509484103
11. Tran DQ, Ramsey H, Shevach EM. Induction of FOXP3 Expression in Naive Human CD4+FOXP3 T Cells by T-Cell Receptor Stimulation is Transforming Growth Factor-Beta Dependent But Does Not Confer a Regulatory Phenotype. Blood (2007) 110:2983–90. doi: 10.1182/BLOOD-2007-06-094656
12. Wang J, Ioan-Facsinay A, van der Voort EIH, Huizinga TWJ, Toes REM. Transient Expression of FOXP3 in Human Activated Nonregulatory CD4+ T Cells. Eur J Immunol (2007) 37:129–38. doi: 10.1002/EJI.200636435
13. Muhammad F, Wang D, McDonald T, Walsh M, Drenen K, Montieth A, et al. TIGIT + A2Ar-Dependent Anti-Uveitic Treg Cells are a Novel Subset of Tregs Associated With Resolution of Autoimmune Uveitis. J Autoimmun (2020) 111:102441. doi: 10.1016/j.jaut.2020.102441
14. Muhammad F, Wang D, Montieth A, Lee S, Preble J, Foster CS, et al. PD-1+ Melanocortin Receptor Dependent-Treg Cells Prevent Autoimmune Disease. Sci Rep (2019) 9:1–11. doi: 10.1038/s41598-019-53297-w
15. Lee DJ, Taylor AW. Following EAU Recovery There is an Associated MC5r-Dependent APC Induction of Regulatory Immunity in the Spleen. Invest Ophthalmol Vis Sci (2011) 52:8862–7. doi: 10.1167/IOVS.11-8153
16. Lee DJ, Taylor AW. Both MC5r and A2Ar Are Required for Protective Regulatory Immunity in the Spleen of Post-Experimental Autoimmune Uveitis in Mice. J Immunol (2013) 191:4103–11. doi: 10.4049/JIMMUNOL.1300182
17. Ito M, Iizuka-Koga M, Ando M, Yoshimura A. Development and Functional Modulation of Regulatory T Cells by Transcription Factors and Epigenetics. Cornea (2018) 37(Suppl 1):S42–9. doi: 10.1097/ICO.0000000000001720
18. Kitagawa Y, Ohkura N, Kidani Y, Vandenbon A, Hirota K, Kawakami R, et al. Guidance of Regulatory T Cell Development by Satb1-Dependent Super-Enhancer Establishment. Nat Immunol (2017) 18:173–83. doi: 10.1038/NI.3646
19. Ohkura N, Hamaguchi M, Morikawa H, Sugimura K, Tanaka A, Ito Y, et al. T Cell Receptor Stimulation-Induced Epigenetic Changes and Foxp3 Expression Are Independent and Complementary Events Required for Treg Cell Development. Immunity (2012) 37:785–99. doi: 10.1016/J.IMMUNI.2012.09.010
20. Lal G, Zhang N, van der Touw W, Ding Y, Ju W, Bottinger EP, et al. Epigenetic Regulation of Foxp3 Expression in Regulatory T Cells by DNA Methylation. J Immunol (2009) 182:259–73. doi: 10.4049/JIMMUNOL.182.1.259
21. Toker A, Engelbert D, Garg G, Polansky JK, Floess S, Miyao T, et al. Active Demethylation of the Foxp3 Locus Leads to the Generation of Stable Regulatory T Cells Within the Thymus. J Immunol (2013) 190:3180–8. doi: 10.4049/JIMMUNOL.1203473
22. Sun JB, Czerkinsky C, Holmgren J. Mucosally Induced Immunological Tolerance, Regulatory T Cells and the Adjuvant Effect by Cholera Toxin B Subunit. Scand J Immunol (2010) 71:1–11. doi: 10.1111/J.1365-3083.2009.02321.X
23. Geri G, Terrier B, Rosenzwajg M, Wechsler B, Touzot M, Seilhean D, et al. Critical Role of IL-21 in Modulating TH17 and Regulatory T Cells in Behçet Disease. J Allergy Clin Immunol (2011) 128:655–64. doi: 10.1016/J.JACI.2011.05.029
24. O’Connor W, Zenewicz LA, Flavell RA. The Dual Nature of T(H)17 Cells: Shifting the Focus to Function. Nat Immunol (2010) 11:471–6. doi: 10.1038/NI.1882
25. O’Connor W, Kamanaka M, Booth CJ, Town T, Nakae S, Iwakura Y, et al. A Protective Function for Interleukin 17A in T Cell-Mediated Intestinal Inflammation. Nat Immunol (2009) 10:603–9. doi: 10.1038/NI.1736
26. Ogawa A, Andoh A, Araki Y, Bamba T, Fujiyama Y. Neutralization of Interleukin-17 Aggravates Dextran Sulfate Sodium-Induced Colitis in Mice. Clin Immunol (2004) 110:55–62. doi: 10.1016/J.CLIM.2003.09.013
27. Beriou G, Costantino CM, Ashley CW, Yang L, Kuchroo VK, Baecher-Allan C, et al. IL-17-Producing Human Peripheral Regulatory T Cells Retain Suppressive Function. Blood (2009) 113:4240–9. doi: 10.1182/BLOOD-2008-10-183251
28. Kang M, Choi JK, Jittayasothorn Y, Egwuagu CE. Interleukin 35-Producing Exosomes Suppress Neuroinflammation and Autoimmune Uveitis. Front Immunol (2020) 11:1051. doi: 10.3389/FIMMU.2020.01051
29. Zhang L, Wan F, Song J, Tang K, Zheng F, Guo J, et al. Imbalance Between Th17 Cells and Regulatory T Cells During Monophasic Experimental Autoimmune Uveitis. Inflammation (2016) 39:113–22. doi: 10.1007/s10753-015-0229-7
30. Demir N, Ilhan F, Demir T, Godekmerdan A. sCTLA-4, CD4+CD25+Foxp3+ Regulatory T Cells in Behçet’s Disease Patients. Clin Exp Rheumatol (2012) 30:S116–7.
31. Hamzaoui K, Hamzaoui A, Houman H. CD4+CD25+ Regulatory T Cells in Patients With Behçet’s Disease. Clin Exp Rheumatol (2006) 24:S71–8.
32. Deniz R, Tulunay-Virlan A, Ture Ozdemir F, Unal AU, Ozen G, Alibaz-Oner F, et al. Th17-Inducing Conditions Lead to In Vitro Activation of Both Th17 and Th1 Responses in Behcet’s Disease. Immunol Invest (2017) 46:518–25. doi: 10.1080/08820139.2017.1306865
33. Shimizu J, Takai K, Fujiwara N, Arimitsu N, Ueda Y, Wakisaka S, et al. Excessive CD4+ T Cells Co-Expressing Interleukin-17 and Interferon-γ in Patients With Behçet’s Disease. Clin Exp Immunol (2012) 168:68–74. doi: 10.1111/J.1365-2249.2011.04543.X
34. Direskeneli H, Fujita H, Akdis CA. Regulation of TH17 and Regulatory T Cells in Patients With Behçet Disease. J Allergy Clin Immunol (2011) 128:665–6. doi: 10.1016/J.JACI.2011.07.008
35. Hamzaoui K. Th17 Cells in Behçet’s Disease: A New Immunoregulatory Axis. Clin Exp Rheumatol (2011) 29:S71–6.
36. Lee RWJ, Dick AD. Current Concepts and Future Directions in the Pathogenesis and Treatment of non-Infectious Intraocular Inflammation. Eye (Lond) (2012) 26:17–28. doi: 10.1038/EYE.2011.255
37. Grajewski RS, Silver PB, Agarwal RK, Su SB, Chan CC, Liou GI, et al. Endogenous IRBP can be Dispensable for Generation of Natural CD4+CD25+ Regulatory T Cells That Protect From IRBP-Induced Retinal Autoimmunity. J Exp Med (2006) 203:851–6. doi: 10.1084/JEM.20050429
38. Silver PB, Agarwal RK, Su S-B, Suffia I, Grajewski RS, Luger D, et al. Hydrodynamic Vaccination With DNA Encoding an Immunologically Privileged Retinal Antigen Protects From Autoimmunity Through Induction of Regulatory T Cells. J Immunol (2007) 179:5146–58. doi: 10.4049/JIMMUNOL.179.8.5146
39. Imai A, Sugita S, Kawazoe Y, Horie S, Yamada Y, Keino H, et al. Immunosuppressive Properties of Regulatory T Cells Generated by Incubation of Peripheral Blood Mononuclear Cells With Supernatants of Human RPE Cells. Invest Ophthalmol Vis Sci (2012) 53:7299–309. doi: 10.1167/IOVS.12-10182
40. Berer K, Mues M, Koutrolos M, AlRasbi Z, Boziki M, Johner C, et al. Commensal Microbiota and Myelin Autoantigen Cooperate to Trigger Autoimmune Demyelination. Nat (2011) 479:538–41. doi: 10.1038/nature10554
41. Brown CT, Davis-Richardson AG, Giongo A, Gano KA, Crabb DB, Mukherjee N, et al. Gut Microbiome Metagenomics Analysis Suggests a Functional Model for the Development of Autoimmunity for Type 1 Diabetes. PloS One (2011) 6(10):e25792. doi: 10.1371/journal.pone.0025792
42. Cantarel BL, Waubant E, Chehoud C, Kuczynski J, Desantis TZ, Warrington J, et al. Gut Microbiota in Multiple Sclerosis: Possible Influence of Immunomodulators. J Investig Med (2015) 63:729–34. doi: 10.1097/JIM.0000000000000192
43. Wang Y, Yin Y, Chen X, Zhao Y, Wu Y, Li Y, et al. Induction of Intestinal Th17 Cells by Flagellins From Segmented Filamentous Bacteria. Front Immunol (2019) 10:2750. doi: 10.3389/FIMMU.2019.02750
44. Lee YK, Menezes JS, Umesaki Y, Mazmanian SK. Proinflammatory T-Cell Responses to Gut Microbiota Promote Experimental Autoimmune Encephalomyelitis. Proc Natl Acad Sci USA (2011) 108:4615–22. doi: 10.1073/PNAS.1000082107
45. Ochoa-Repáraz J, Mielcarz DW, Ditrio LE, Burroughs AR, Foureau DM, Haque-Begum S, et al. Role of Gut Commensal Microflora in the Development of Experimental Autoimmune Encephalomyelitis. J Immunol (2009) 183:6041–50. doi: 10.4049/JIMMUNOL.0900747
46. Scher JU, Sczesnak A, Longman RS, Segata N, Ubeda C, Bielski C, et al. Expansion of Intestinal Prevotella Copri Correlates With Enhanced Susceptibility to Arthritis. Elife (2013) 2:e01202. doi: 10.7554/eLife.01202
47. Vijay-Kumar M, Aitken JD, Carvalho FA, Cullender TC, Mwangi S, Srinivasan S, et al. Metabolic Syndrome and Altered Gut Microbiota in Mice Lacking Toll-Like Receptor 5. Science (2010) 328:228–31. doi: 10.1126/SCIENCE.1179721
48. Nakamura YK, Metea C, Karstens L, Asquith M, Gruner H, Moscibrocki C, et al. Gut Microbial Alterations Associated With Protection From Autoimmune Uveitis. Invest Ophthalmol Vis Sci (2016) 57:3747. doi: 10.1167/IOVS.16-19733
49. Horai R, Silver PB, Chen J, Agarwal RK, Chong WP, Jittayasothorn Y, et al. Breakdown of Immune Privilege and Spontaneous Autoimmunity in Mice Expressing a Transgenic T Cell Receptor Specific for a Retinal Autoantigen. J Autoimmun (2013) 44:21–33. doi: 10.1016/j.jaut.2013.06.003
50. Horai R, Zárate-Bladés CR, Dillenburg-Pilla P, Chen J, Kielczewski JL, Silver PB, et al. Microbiota-Dependent Activation of an Autoreactive T Cell Receptor Provokes Autoimmunity in an Immunologically Privileged Site. Immunity (2015) 43:343–53. doi: 10.1016/J.IMMUNI.2015.07.014
51. Tan C, Wandu WS, Lee RS, Hinshaw SH, Klinman DM, Wawrousek E, et al. Shedding New Light on the Process of “Licensing” for Pathogenicity by Th Lymphocytes. J Immunol (2017) 198:681–90. doi: 10.4049/JIMMUNOL.1502108
52. Chen J, Vistica BP, Takase H, Il Ham D, Fariss RN, Wawrousek EF, et al. A Unique Pattern of Up- and Down-Regulation of Chemokine Receptor CXCR3 on Inflammation-Inducing Th1 Cells. Eur J Immunol (2004) 34:2885–94. doi: 10.1002/EJI.200425318
53. Kasper M, Gabriel D, Möller M, Bauer D, Wildschütz L, Courthion H, et al. Novel Everolimus-Loaded Nanocarriers for Topical Treatment of Murine Experimental Autoimmune Uveoretinitis (EAU). Exp Eye Res (2018) 168:49–56. doi: 10.1016/J.EXER.2018.01.003
54. Chen X, Su W, Wan T, Yu J, Zhu W, Tang F, et al. Sodium Butyrate Regulates Th17/Treg Cell Balance to Ameliorate Uveitis via the Nrf2/HO-1 Pathway. Biochem Pharmacol (2017) 142:111–9. doi: 10.1016/J.BCP.2017.06.136
55. Khan H, Sureda A, Belwal T, Çetinkaya S, Süntar İ, Tejada S, et al. Polyphenols in the Treatment of Autoimmune Diseases. Autoimmun Rev (2019) 18:647. doi: 10.1016/J.AUTREV.2019.05.001
56. Villegas SN, Gombos R, García-López L, Gutiérrez-Pérez I, García-Castillo J, Vallejo DM, et al. PI3K/Akt Cooperates With Oncogenic Notch by Inducing Nitric Oxide-Dependent Inflammation. Cell Rep (2018) 22:2541–9. doi: 10.1016/J.CELREP.2018.02.049
57. Chen Y, Li Z, Li H, Su W, Xie Y, Pan Y, et al. Apremilast Regulates the Teff/Treg Balance to Ameliorate Uveitis via PI3K/AKT/FoxO1 Signaling Pathway. Front Immunol (2020) 11:581673. doi: 10.3389/FIMMU.2020.581673
58. Schafer PH, Parton A, Gandhi AK, Capone L, Adams M, Wu L, et al. Apremilast, A cAMP Phosphodiesterase-4 Inhibitor, Demonstrates Anti-Inflammatory Activity In Vitro and in a Model of Psoriasis. Br J Pharmacol (2010) 159:842–55. doi: 10.1111/J.1476-5381.2009.00559.X
59. Gooderham M, Papp K. Selective Phosphodiesterase Inhibitors for Psoriasis: Focus on Apremilast. BioDrugs (2015) 29:327–39. doi: 10.1007/S40259-015-0144-3
60. Schafer PH, Adams M, Horan G, Truzzi F, Marconi A, Pincelli C. Apremilast Normalizes Gene Expression of Inflammatory Mediators in Human Keratinocytes and Reduces Antigen-Induced Atopic Dermatitis in Mice. Drugs R D (2019) 19:329–38. doi: 10.1007/S40268-019-00284-1
61. McCann FE, Palfreeman AC, Andrews M, Perocheau DP, Inglis JJ, Schafer P, et al. Apremilast, A Novel PDE4 Inhibitor, Inhibits Spontaneous Production of Tumour Necrosis Factor-Alpha From Human Rheumatoid Synovial Cells and Ameliorates Experimental Arthritis. Arthritis Res Ther (2010) 12(3):R107. doi: 10.1186/ar3041
62. Chen W, Wang J, Xu Z, Huang F, Qian W, Ma J, et al. Apremilast Ameliorates Experimental Arthritis via Suppression of Th1 and Th17 Cells and Enhancement of CD4+Foxp3+ Regulatory T Cells Differentiation. Front Immunol (2018) 9:1662. doi: 10.3389/FIMMU.2018.01662
63. Kragstrup TW, Adams M, Lomholt S, Nielsen MA, Heftdal LD, Schafer P, et al. IL-12/IL-23p40 Identified as a Downstream Target of Apremilast in Ex Vivo Models of Arthritis. Ther Adv Musculoskelet Dis (2019) 11:1759720X1982866. doi: 10.1177/1759720X19828669
64. Bing SJ, Shemesh I, Chong WP, Horai R, Jittayasothorn Y, Silver PB, et al. AS101 Ameliorates Experimental Autoimmune Uveitis by Regulating Th1 and Th17 Responses and Inducing Treg Cells. J Autoimmun (2019) 100:52–61. doi: 10.1016/J.JAUT.2019.02.006
65. Wang C, Zhou W, Su G, Hu J, Yang P. Progranulin Suppressed Autoimmune Uveitis and Autoimmune Neuroinflammation by Inhibiting Th1/Th17 Cells and Promoting Treg Cells and M2 Macrophages. Neurol - Neuroimmunol Neuroinflamm (2022) 9(2):e1133. doi: 10.1212/NXI.0000000000001133
66. Mei S, Huang Y, Li N, Xu Z, Xu J, Dai Q, et al. Aminooxy-Acetic Acid Inhibits Experimental Autoimmune Uveitis by Modulating the Balance Between Effector and Regulatory Lymphocyte Subsets. Curr Mol Med (2020) 20:624–32. doi: 10.2174/1566524020666200211112219
67. Dua B, Upadhyay R, Natrajan M, Arora M, Kithiganahalli Narayanaswamy B, Joshi B. Notch Signaling Induces Lymphoproliferation, T Helper Cell Activation and Th1/Th2 Differentiation in Leprosy. Immunol Lett (2019) 207:6–16. doi: 10.1016/J.IMLET.2019.01.003
68. Amsen D, Helbig C, Backer RA. Notch in T Cell Differentiation: All Things Considered. Trends Immunol (2015) 36:802–14. doi: 10.1016/J.IT.2015.10.007
69. Jiao WE, Wei JF, Kong YG, Xu Y, Tao ZZ, Chen SM. Notch Signaling Promotes Development of Allergic Rhinitis by Suppressing Foxp3 Expression and Treg Cell Differentiation. Int Arch Allergy Immunol (2019) 178:33–44. doi: 10.1159/000493328
70. Wei H, Yin X, Tang H, Gao Y, Liu B, Wu Q, et al. Hypomethylation of Notch1 DNA is Associated With the Occurrence of Uveitis. Clin Exp Immunol (2020) 201:317–27. doi: 10.1111/CEI.13471
71. Rong H, Shen H, Xu Y, Yang H. Notch Signalling Suppresses Regulatory T-Cell Function in Murine Experimental Autoimmune Uveitis. Immunology (2016) 149:447–59. doi: 10.1111/IMM.12663
72. Yin X, Wei H, Wu S, Wang Z, Liu B, Guo L, et al. DAPT Reverses the Th17/Treg Imbalance in Experimental Autoimmune Uveitis In Vitro via Inhibiting Notch Signaling Pathway. Int Immunopharmacol (2020) 79:106107. doi: 10.1016/j.intimp.2019.106107
73. Wang RX, Yu CR, Dambuza IM, Mahdi RM, Dolinska MB, Sergeev YV, et al. Interleukin-35 Induces Regulatory B Cells That Suppress Autoimmune Disease. Nat Med (2014) 20:633–41. doi: 10.1038/NM.3554
74. van Deemter M, Pas HH, Kuijer R, van der Worp RJ, Hooymans JMM, Los LI. Enzymatic Breakdown of Type II Collagen in the Human Vitreous. Invest Ophthalmol Vis Sci (2009) 50:4552–60. doi: 10.1167/IOVS.08-3125
75. Ihanamäki T, Salminen H, Säämänen AM, Pelliniemi LJ, Hartmann DJ, Sandberg-Lall M, et al. Age-Dependent Changes in the Expression of Matrix Components in the Mouse Eye. Exp Eye Res (2001) 72:423–31. doi: 10.1006/EXER.2000.0972
76. Foussat A, Gregoire S, Clerget-Chossat N, Terrada C, Asnagli H, Lemoine FM, et al. Regulatory T Cell Therapy for Uveitis: A New Promising Challenge. J Ocul Pharmacol Ther (2017) 33:278–84. doi: 10.1089/JOP.2016.0165
77. Asnagli H, Jacquin M, Belmonte N, Gertner-Dardenne J, Hubert MF, Sales A, et al. Inhibition of Noninfectious Uveitis Using Intravenous Administration of Collagen II-Specific Type 1 Regulatory T Cells. Invest Ophthalmol Vis Sci (2015) 56:6456–66. doi: 10.1167/IOVS.15-16883
78. Tasso R, Ilengo C, Quarto R, Cancedda R, Caspi RR, Pennesi G. Mesenchymal Stem Cells Induce Functionally Active T-Regulatory Lymphocytes in a Paracrine Fashion and Ameliorate Experimental Autoimmune Uveitis. Invest Ophthalmol Vis Sci (2012) 53:786–93. doi: 10.1167/IOVS.11-8211
79. Ma OKF, Chan KH. Immunomodulation by Mesenchymal Stem Cells: Interplay Between Mesenchymal Stem Cells and Regulatory Lymphocytes. World J Stem Cells (2016) 8:268–78. doi: 10.4252/WJSC.V8.I9.268
80. Ghannam S, Pene J, Torcy-Moquet G, Jorgensen C, Yssel H, Yssel H. Mesenchymal Stem Cells Inhibit Human Th17 Cell Differentiation and Function and Induce a T Regulatory Cell Phenotype. J Immunol (2010) 185:302–12. doi: 10.4049/jimmunol.0902007
81. Akle CA, Welsh KI, Adinolfi M, Leibowitz S, Mccoll I. Immunogenicity of Human Amniotic Epithelial Cells After Transplantation Into Volunteers. Lancet (London England) (1981) 2:1003–5. doi: 10.1016/S0140-6736(81)91212-5
82. Li H, Niederkorn JY, Neelam S, Mayhew E, Word RA, McCulley JP, et al. Immunosuppressive Factors Secreted by Human Amniotic Epithelial Cells. Invest Ophthalmol Vis Sci (2005) 46:900–7. doi: 10.1167/IOVS.04-0495
83. Strom SC, Gramignoli R. Human Amnion Epithelial Cells Expressing HLA-G as Novel Cell-Based Treatment for Liver Disease. Hum Immunol (2016) 77:734–9. doi: 10.1016/J.HUMIMM.2016.07.002
84. Jiang T-S, Cai L, Ji W-Y, Hui Y-N, Wang Y-S, Hu D, et al. Reconstruction of the Corneal Epithelium With Induced Marrow Mesenchymal Stem Cells in Rats. Mol Vis (2010) 16:1304–16.
85. Yang P-J, Yuan W-X, Liu J, Li J-Y, Tan B, Qiu C, et al. Biological Characterization of Human Amniotic Epithelial Cells in a Serum-Free System and Their Safety Evaluation. Acta Pharmacol Sin (2018) 39:1305–16. doi: 10.1038/aps.2018.22
86. Li J, Qiu C, Zhang Z, Yuan W, Ge Z, Tan B, et al. Subretinal Transplantation of Human Amniotic Epithelial Cells in the Treatment of Autoimmune Uveitis in Rats. Cell Transplant (2018) 27:1504–14. doi: 10.1177/0963689718796196
87. Ali MA, Liu YF, Arif S, Tatovic D, Shariff H, Gibson VB, et al. Metabolic and Immune Effects of Immunotherapy With Proinsulin Peptide in Human New-Onset Type 1 Diabetes. Sci Transl Med (2017) 9(402):eaaf7779. doi: 10.1126/scitranslmed.aaf7779
88. Hall JA, Cannons JL, Grainger JR, Dos Santos LM, Hand TW, Naik S, et al. Essential Role for Retinoic Acid in the Promotion of CD4+ T Cell Effector Responses via Retinoic Acid Receptor Alpha. Immunity (2011) 34:435. doi: 10.1016/J.IMMUNI.2011.03.003
89. Coombes JL, Siddiqui KRR, Arancibia-Cárcamo CV, Hall J, Sun CM, Belkaid Y, et al. A Functionally Specialized Population of Mucosal CD103+ DCs Induces Foxp3+ Regulatory T Cells via a TGF-Beta and Retinoic Acid-Dependent Mechanism. J Exp Med (2007) 204:1757–64. doi: 10.1084/JEM.20070590
90. Zhou X, Kong N, Wang J, Fan H, Zou H, Horwitz D, et al. Cutting Edge: All-Trans Retinoic Acid Sustains the Stability and Function of Natural Regulatory T Cells in an Inflammatory Milieu. J Immunol (2010) 185:2675–9. doi: 10.4049/JIMMUNOL.1000598
91. Lu L, Lan Q, Li Z, Zhou X, Gu J, Li Q, et al. Critical Role of All-Trans Retinoic Acid in Stabilizing Human Natural Regulatory T Cells Under Inflammatory Conditions. Proc Natl Acad Sci USA (2014) 111(33):E3432-40. doi: 10.1073/pnas.1408780111
92. Zhou R, Horai R, Mattapallil MJ, Caspi RR. A New Look at Immune Privilege of the Eye: Dual Role for the Vision-Related Molecule Retinoic Acid. J Immunol (2011) 187:4170–7. doi: 10.4049/JIMMUNOL.1101634
93. Raverdeau M, Christofi M, Malara A, Wilk MM, Misiak A, Kuffova L, et al. Retinoic Acid-Induced Autoantigen-Specific Type 1 Regulatory T Cells Suppress Autoimmunity. EMBO Rep (2019) 20(5):e47121. doi: 10.15252/embr.201847121
94. Dansokho C, Ait Ahmed D, Aid S, Toly-Ndour C, Chaigneau T, Calle V, et al. Regulatory T Cells Delay Disease Progression in Alzheimer-Like Pathology. Brain (2016) 139:1237–51. doi: 10.1093/BRAIN/AWV408
95. Grinberg-Bleyer Y, Baeyens A, You S, Elhage R, Fourcade G, Gregoire S, et al. IL-2 Reverses Established Type 1 Diabetes in NOD Mice by a Local Effect on Pancreatic Regulatory T Cells. J Exp Med (2010) 207:1871–8. doi: 10.1084/JEM.20100209
96. Bonnet B, Vigneron J, Levacher B, Vazquez T, Pitoiset F, Brimaud F, et al. Low-Dose IL-2 Induces Regulatory T Cell-Mediated Control of Experimental Food Allergy. J Immunol (2016) 197:188–98. doi: 10.4049/JIMMUNOL.1501271
97. Chen Z, Zhang T, Kam HT, Qiao D, Jin W, Zhong Y, et al. Induction of Antigen-Specific Treg Cells in Treating Autoimmune Uveitis via Bystander Suppressive Pathways Without Compromising Anti-Tumor Immunity. EBioMedicine (2021) 70:103496. doi: 10.1016/J.EBIOM.2021.103496
98. Dominguez-Villar M, Baecher-Allan CM, Hafler DA. Identification of T Helper Type 1-Like, Foxp3+ Regulatory T Cells in Human Autoimmune Disease. Nat Med (2011) 17:673–5. doi: 10.1038/NM.2389
99. Albayrak O, Oray M, Can F, Kirimli GU, Gul A, Tugal-Tutkun I, et al. Effect of Interferon Alfa-2a Treatment on Adaptive and Innate Immune Systems in Patients With Behçet Disease Uveitis. Invest Ophthalmol Vis Sci (2019) 60:52–63. doi: 10.1167/IOVS.18-25548
100. Chauhan SK, Saban DR, Lee HK, Dana R. Levels of Foxp3 in Regulatory T Cells Reflect Their Functional Status in Transplantation. J Immunol (2009) 182:148–53. doi: 10.4049/jimmunol.182.1.148
101. Miserocchi E, Giuffrè C, Cornalba M, Pontikaki I, Cimaz R. JAK Inhibitors in Refractory Juvenile Idiopathic Arthritis-Associated Uveitis. Clin Rheumatol (2020) 39:847–51. doi: 10.1007/S10067-019-04875-W
102. Yong C, Dan L, Chenhong L, Yan S, Jianfei C, Jianlong G. Efficacy and Safety of Metformin for Behcet’s Disease and Its Effect on Treg/Th17 Balance: A Single-Blinded, Before-After Study. Nan Fang Yi Ke Da Xue Xue Bao (2019) 39:127–33. doi: 10.12122/J.ISSN.1673-4254.2019.02.01
103. Liu X, Li W, Liu X, Luo J, Gao C, Li X. Low-Dose IL-2 Effectively Restored Decreased Regulatory T Cells in Patients With Behçet’s Disease. Clin Exp Rheumatol (2021) 39:746–52.
104. Sakaguchi S, Mikami N, Wing JB, Tanaka A, Ichiyama K, Ohkura N. Regulatory T Cells and Human Disease. Annu Rev Immunol (2020) 38:541–66. doi: 10.1146/ANNUREV-IMMUNOL-042718-041717
105. Caudy AA, Reddy ST, Chatila T, Atkinson JP, Verbsky JW. CD25 Deficiency Causes an Immune Dysregulation, Polyendocrinopathy, Enteropathy, X-Linked-Like Syndrome, and Defective IL-10 Expression From CD4 Lymphocytes. J Allergy Clin Immunol (2007) 119:482–7. doi: 10.1016/J.JACI.2006.10.007
106. Schubert D, Bode C, Kenefeck R, Hou TZ, Wing JB, Kennedy A, et al. Autosomal Dominant Immune Dysregulation Syndrome in Humans With CTLA4 Mutations. Nat Med (2014) 20:1410–6. doi: 10.1038/NM.3746
107. Knochelmann HM, Dwyer CJ, Bailey SR, Amaya SM, Elston DM, Mazza-McCrann JM, et al. When Worlds Collide: Th17 and Treg Cells in Cancer and Autoimmunity. Cell Mol Immunol (2018) 15:458–69. doi: 10.1038/S41423-018-0004-4
108. Viglietta V, Baecher-Allan C, Weiner HL, Hafler DA. Loss of Functional Suppression by CD4+CD25+ Regulatory T Cells in Patients With Multiple Sclerosis. J Exp Med (2004) 199:971–9. doi: 10.1084/jem.20031579
109. Sugiyama H, Gyulai R, Toichi E, Garaczi E, Shimada S, Stevens SR, et al. Dysfunctional Blood and Target Tissue CD4+CD25high Regulatory T Cells in Psoriasis: Mechanism Underlying Unrestrained Pathogenic Effector T Cell Proliferation. J Immunol (2005) 174:164. doi: 10.4049/JIMMUNOL.174.1.164
110. Jorn Bovenschen H, van de Kerkhof PC, van Erp PE, Woestenenk R, Joosten I, Koenen HJPM. Foxp3+ Regulatory T Cells of Psoriasis Patients Easily Differentiate Into IL-17a-Producing Cells and Are Found in Lesional Skin. J Invest Dermatol (2011) 131:1853–60. doi: 10.1038/jid.2011.139
111. Samson M, Audia S, Janikashvili N, Ciudad M, Trad M, Fraszczak J, et al. Brief Report: Inhibition of Interleukin-6 Function Corrects Th17/Treg Cell Imbalance in Patients With Rheumatoid Arthritis. Arthritis Rheum (2012) 64:2499–503. doi: 10.1002/ART.34477
112. Kikuchi J, Hashizume M, Kaneko Y, Yoshimoto K, Nishina N, Takeuchi T. Peripheral Blood CD4+CD25+CD127low Regulatory T Cells are Significantly Increased by Tocilizumab Treatment in Patients With Rheumatoid Arthritis: Increase in Regulatory T Cells Correlates With Clinical Response. Arthritis Res Ther (2015) 17:1–10. doi: 10.1186/S13075-015-0526-4/FIGURES/1
113. Grant CR, Liberal R, Mieli-Vergani G, Vergani D, Longhi MS. Regulatory T-Cells in Autoimmune Diseases: Challenges, Controversies and–Yet–Unanswered Questions. Autoimmun Rev (2015) 14:105–16. doi: 10.1016/J.AUTREV.2014.10.012
114. Miyara M, Gorochov G, Ehrenstein M, Musset L, Sakaguchi S, Amoura Z. Human FoxP3+ Regulatory T Cells in Systemic Autoimmune Diseases. Autoimmun Rev (2011) 10:744–55. doi: 10.1016/J.AUTREV.2011.05.004
115. Bonelli M, Savitskaya A, Steiner C-W, Rath E, Smolen JS, Scheinecker C. Phenotypic and Functional Analysis of CD4+CD25–Foxp3+ T Cells in Patients With Systemic Lupus Erythematosus. J Immunol (2009) 182:1689–95. doi: 10.4049/JIMMUNOL.182.3.1689
116. Ferreira RC, Simons HZ, Thompson WS, Rainbow DB, Yang X, Cutler AJ, et al. Cells With Treg-Specific FOXP3 Demethylation But Low CD25 are Prevalent in Autoimmunity. J Autoimmun (2017) 84:75. doi: 10.1016/J.JAUT.2017.07.009
117. Golding A, Hasni S, Illei G, Shevach EM. The Percentage of FoxP3+Helios+ Treg Cells Correlates Positively With Disease Activity in Systemic Lupus Erythematosus. Arthritis Rheum (2013) 65:2898–906. doi: 10.1002/ART.38119
118. Morita T, Shima Y, Wing JB, Sakaguchi S, Ogata A, Kumanogoh A. The Proportion of Regulatory T Cells in Patients With Rheumatoid Arthritis: A Meta-Analysis. PloS One (2016) 11(9):e0162306. doi: 10.1371/journal.pone.0162306
119. Spence A, Klementowicz JE, Bluestone JA, Tang Q. Targeting Treg Signaling for the Treatment of Autoimmune Diseases. Curr Opin Immunol (2015) 37:11–20. doi: 10.1016/J.COI.2015.09.002
120. Trotta E, Bessette PH, Silveria SL, Ely LK, Jude KM, Le DT, et al. A Human Anti-IL-2 Antibody That Potentiates Regulatory T Cells by a Structure-Based Mechanism. Nat Med (2018) 24:1005–14. doi: 10.1038/s41591-018-0070-2
121. Bluestone JA, Buckner JH, Fitch M, Gitelman SE, Gupta S, Hellerstein MK, et al. Type 1 Diabetes Immunotherapy Using Polyclonal Regulatory T Cells. Sci Transl Med (2015) 7:315ra189. doi: 10.1126/scitranslmed.aad4134
122. Brunstein CG, Miller JS, McKenna DH, Hippen KL, DeFor TE, Sumstad D, et al. Umbilical Cord Blood-Derived T Regulatory Cells to Prevent GVHD: Kinetics, Toxicity Profile, and Clinical Effect. Blood (2016) 127:1044–51. doi: 10.1182/BLOOD-2015-06-653667
123. Grégoire S, Terrada C, Martin GH, Fourcade G, Baeyens A, Marodon G, et al. Treatment of Uveitis by In Situ Administration of Ex Vivo-Activated Polyclonal Regulatory T Cells. J Immunol (2016) 196:2109–18. doi: 10.4049/JIMMUNOL.1501723
124. Terrada C, Fisson S, De Kozak Y, Kaddouri M, Lehoang P, Klatzmann D, et al. Regulatory T Cells Control Uveoretinitis Induced by Pathogenic Th1 Cells Reacting to a Specific Retinal Neoantigen. J Immunol (2006) 176:7171–9. doi: 10.4049/JIMMUNOL.176.12.7171
125. Iizuka-Koga M, Nakatsukasa H, Ito M, Akanuma T, Lu Q, Yoshimura A. Induction and Maintenance of Regulatory T Cells by Transcription Factors and Epigenetic Modifications. J Autoimmun (2017) 83:113–21. doi: 10.1016/J.JAUT.2017.07.002
126. Miyara M, Yoshioka Y, Kitoh A, Shima T, Wing K, Niwa A, et al. Functional Delineation and Differentiation Dynamics of Human CD4+ T Cells Expressing the FoxP3 Transcription Factor. Immunity (2009) 30:899–911. doi: 10.1016/j.immuni.2009.03.019
Keywords: regulatory T cells, Tregs, uveitis, inflammatory eye disease, T cell plasticity
Citation: Lee AYJ and Foulsham W (2022) Regulatory T Cells: Therapeutic Opportunities in Uveitis. Front. Ophthalmol. 2:901144. doi: 10.3389/fopht.2022.901144
Received: 21 March 2022; Accepted: 28 April 2022;
Published: 25 May 2022.
Edited by:
Daniel Vitor Vasconcelos-Santos, Universidade Federal de Minas Gerais, BrazilReviewed by:
Shilpa Kodati, National Institutes of Health (NIH), United StatesCopyright © 2022 Lee and Foulsham. This is an open-access article distributed under the terms of the Creative Commons Attribution License (CC BY). The use, distribution or reproduction in other forums is permitted, provided the original author(s) and the copyright owner(s) are credited and that the original publication in this journal is cited, in accordance with accepted academic practice. No use, distribution or reproduction is permitted which does not comply with these terms.
*Correspondence: William Foulsham, d3NmOTAwM0BueXAub3Jn